Abstract
A traditional karyomorphological approach was carried out in order to investigate the cytotaxonomic relationships among the species of the Santolina chamaecyparissus complex. All the 14 species included in this species complex were analysed for a total of 39 populations. Metaphase plates were obtained by squashing root tips stained with Feulgen technique. Measurable metaphase plates available in literature were also re-analysed. Chromosome number (2n), total haploid (monoploid) length (THL), and karyotype asymmetry indices (CVCL and MCA) were calculated for each of the 14 species. Chromosome data are provided for the first time for S. benthamiana and S. vedranensis, while a new hexaploid cytotype is reported for S. villosa. Five different ploidy levels are detected: diploid, triploid (a single individual of the otherwise diploid S. virens), tetraploid, pentaploid, and hexaploid. Our analyses reveal that polyploids are characterized by a more asymmetric karyotype with respect to diploids. The possible origin of polyploids, and in particular of the cultivated pentaploid S. chamaecyparissus, is briefly discussed.
Introduction
The Santolina chamaecyparissus complex includes 14 species that are endemic to the western portion of the Mediterranean Basin (Greuter Citation2008). These plants are dwarf aromatic shrubs occurring in arid habitats and preferably on limestone, where they usually form garigues (Arrigoni Citation1982a; Angiolini and Bacchetta Citation2003; Carbajal et al. Citation2019). Four different ploidy levels are currently known in this complex. The continental species, except S. villosa Mill. that is reported as tetraploid (2n = 4x = 36), are diploid with 2x = 2n = 18 chromosomes (Arrigoni Citation1977; Valdés-Bermejo and Antúnez Citation1981; Kyohs Citation1982; Afzal-Rafii et al. Citation1985). Santolina corsica Jord. & Fourr., a species endemic to Corsica and Sardinia, is tetraploid, and S. insularis (Gennari ex Fiori) Arrigoni, which is endemic to Sardinia only, is hexaploid (2n = 6x = 54) (Marchi and D’Amato Citation1973; Marchi et al. Citation1979). Santolina chamaecyparissus L., the nomenclatural type of the genus Santolina L. (Greuter Citation2008), is pentaploid with 2n = 5x = 45 chromosomes (Marchi and D’Amato Citation1973). The latter is a commonly cultivated species of unknown origin, very appreciated as ornamental in gardens and hedges (Giacò et al. Citation2021). According to Marchi and D’Amato (Citation1973), it may have been originated by a crossing between S. insularis and another tetraploid Santolina. According to qualitative cytogenetic studies, polyploid species of the S. chamaecyparissus complex seemingly show a higher number of subterminal chromosomes (Marchi and D’Amato Citation1973; Marchi et al. Citation1979; Valdés-Bermejo and Antúnez Citation1981). Previous studies on the S. rosmarinifolia L. complex (Rivero-Guerra Citation2008a, Citation2008b, Citation2010) highlighted that, in Iberian Peninsula, polyploidy and chromosome rearrangements played an important role in the segregation of taxa. Based on the current knowledge, we hypothesize that these phenomena played an important role in the evolution of the S. chamaecyparissus complex as well. In order to investigate the diversity at a cytogenetic level among the species of this complex, we carried out a karyomorphological study (Paszko Citation2006; Peruzzi and Eroğlu Citation2013; Peruzzi and Altınordu Citation2014).
Karyomorphology, i.e. the study of the general morphology of a chromosome complement, is a cheap and relatively easy method to investigate plant diversity at different taxonomic levels (Peruzzi et al. Citation2009; Astuti et al. Citation2017). With Feulgen or similar staining techniques, the most common studied parameters are the chromosome number (2n), the basic chromosome number (x), the Total Haploid (monoploid) Length (THL), and the karyotype asymmetry (Astuti et al. Citation2017). The first two parameters provide basic and fundamental information to understand relationships among taxa, especially when studying groups showing dysploidy and/or polyploidy (Levin Citation2002). THL is a good proxy for genome size (Peruzzi et al. Citation2009; Carta and Peruzzi Citation2016). Karyotype asymmetry depends on the heterogeneity of chromosome sizes in a complement (inter-chromosomal asymmetry), and on the deviation degree of centromeres from the median position in each chromosome (intra-chromosomal asymmetry) (Levitsky Citation1931; Stebbins Citation1971). Albeit the limitations of the traditional karyomorphological approach are well known (Guerra Citation2012; Astuti et al. Citation2017), it can provide valuable information to better understand evolutionary trends and to infer taxonomic relationships, especially when integrated with other approaches, such as molecular and morphological analyses (Caputo et al. Citation2013; Nardi et al. Citation2018; Bernardo et al. Citation2020; Ghosh et al. Citation2021).
The aim of this study is to have a complete picture about the chromosome number and karyotype structure of the species belonging to S. chamaecyparissus complex. Moreover, we want to quantitatively check if there are significant karyomorphological differences among diploid and polyploid karyotypes, as suggested by previous scholars (Marchi and D’Amato Citation1973; Marchi et al. Citation1979; Valdés-Bermejo and Antúnez Citation1981). This study is a further step forward in the framework of an ongoing study of integrative taxonomy of this complex (Giacò et al. Citation2021).
Materials and methods
Collection of material
Material for karyological analyses was collected for all the 14 species belonging to the complex, for a total of 39 populations (Table S1). It includes material newly collected for this study and measurable metaphase plates already published in previous studies (Marchi and D’Amato Citation1973; Marchi et al. Citation1979; Valdés-Bermejo and Antúnez Citation1981). Fieldwork for seed collection was conducted from June to September 2020 and 2021 on 21 populations. For S. chamaecyparissus, given the unavailability of viable seeds in the sampled population, we prepared stem cuttings from two different individuals. The cuttings were planted in the Botanic Garden of Pisa, and root tips for karyological analyses were collected in winter. Seeds for six Sardinian populations were already available at Cagliari’s seed bank (Banca del Germoplasma della Sardegna, BG-SAR).
Table 1. Karyological data in the Santolina chamaecyparissus complex.
Karyological analyses
Metaphase plates were obtained by squashing root-tip cells according to the following protocol: pre-treatment in 0.4% colchicine solution for 3 hours; 3:1 ethanol/acetic-acid fixing solution for one hour; hydrolysis in HCl 1 N for seven to eight minutes at 60 °C; staining with leucobasic fuchsine for at least two hours. Metaphase plates were observed with a Leitz Diaplan microscope, were photographed with a Leica MC170 HD camera and related software (LAS EZ 3.4.0), and were measured using the software KaryoType (Altınordu et al. Citation2016). Images of metaphase plates that were already available in literature (Marchi and D’Amato Citation1973; Marchi et al. Citation1979; Valdés-Bermejo and Antúnez Citation1981) were digitized and measured using the same software. As regards the populations sampled in the field and those coming from BG-SAR, four to seven metaphase plates from different individuals for each population were measured.
THL (Total Haploid Length, i.e. the length of the monoploid chromosome complement) and the indices CVCL and MCA were calculated for each metaphase plate. CVCL (Coefficient of Variation of Chromosome Length) quantifies the inter-chromosomal asymmetry, and it is calculated by the ratio between standard deviation and mean of chromosome length × 100 (Paszko Citation2006). MCA (Mean Centromeric Asymmetry) quantifies the intra-chromosomal asymmetry, and it is calculated as the mean of (L − S)/(L + S) × 100, where “L” stands for long arm and “S” stands for short arm of each chromosome in the complement (Peruzzi and Eroğlu Citation2013). Chromosomes are classified based on Levan’s nomenclature (Levan et al. Citation1964). Differences among ploidy levels were investigated with Kruskal-Wallis test followed by pairwise Mann-Whitney U-Test with Bonferroni correction. Differences were considered significant when p < 0.01. Statistical analyses were conducted using R software (R: A language and environment for statistical URL: https://www.R-project.org/.).
Idiograms presented in were obtained based on the average measures reported in Tables S2 and S3, respectively. Chromosomes of different metaphase plates were grouped following the graphical method proposed by Plummer et al. (Citation2003). In this method, New Relative Length (100 × chromosome length of the single chromosome/total length of all chromosomes) is plotted against arm ratio. In this representation, putatively homologous chromosomes are displayed close each other.
Figure 1. Monoploid idiograms (x = 9) of the studied species of the Santolina chamaecyparissus complex, based on the mean values reported in Table S2. For Santolina chamaecyparissus a holoploid idiogram is presented, based on the mean values reported in Table S3. The last 15 chromosomes of S. chamaecyparissus cannot be grouped by five on morphological grounds. Consequently, according to their mean arm ratio, they have been grouped in one group of seven submetacentric chromosomes, and one group of eight subtelocentric chromosomes. Metacentric and submetacentric chromosomes are coloured in blue, while subtelocentric chromosomes are coloured in red.
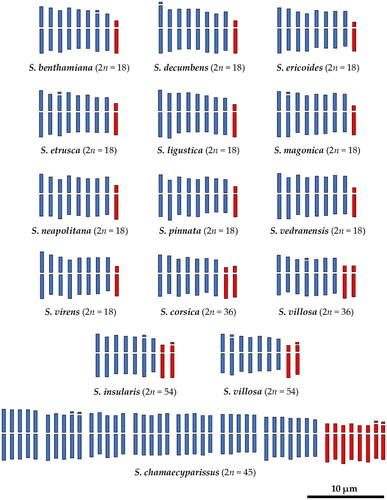
Results
Ploidy levels
Five different ploidy levels were detected: diploid, triploid, tetraploid, pentaploid, and hexaploid. The triploid level is referred to a single individual detected in the studied population of S. virens, that is otherwise diploid. Chromosome number is provided for the first time for S. benthamiana and S. vedranensis, that are both diploid. A new hexaploid cytotype was detected for S. villosa in southern Spain. A summary of ploidy levels and mean values of THL, CVCL, and MCA is reported in .
Karyomorphology and karyotype asymmetry
The two studied populations of S. benthamiana are diploid (2n = 2x = 18 chromosomes) and show 8 pairs of metacentric (m)/submetacentric (sm) chromosomes and one pair of subtelocentric (st) chromosomes ( and , Table S2). The longest chromosome of the complement is 6.3 μm ± 1.0 μm long on average, and the shortest chromosome is 4.3 μm ± 0.8 μm long on average. THL, CVCL, and MCA mean values are reported in . Secondary constrictions were occasionally observed in the population from Le Roumenga (Occitanie, France) on a m chromosome pair and on a sm chromosome pair (–C).
Figure 2. Selected metaphase plates in species of the Santolina chamaecyparissus complex. Santolina benthamiana (A, 2n = 2x = 18, Prats-de-Mollo-la-Preste, Occitanie, France), S. chamaecyparissus (B, 2n = 5x = 45, Le Luc, Provence-Alpes-Côte d’Azur, France), S. corsica (C, 2n = 4x = 36, Mont Pigno, Corsica, France), S. decumbens (D, 2n = 2x = 18, Mont Caume, Provence-Alpes-Côte d’Azur, France), S. ericoides (E, 2n = 2x = 18, Béziers, Occitanie, France), S. etrusca (F, 2n = 2x = 18, Radicofani, Tuscany, Italy), S. insularis (G, 2n = 6x = 54, Iglesias, Sardinia, Italy), S. ligustica (H, 2n = 2x = 18, Levanto, Liguria Italy), S. magonica (I, 2n = 2x = 18, Tirant [Menorca], Balearic Islands, Spain), S. neapolitana (J, 2n = 2x = 18, Monte Sant’Angelo, Campania, Italy), S. pinnata (K, 2n = 2x = 18, Forno [Apuan Alps], Tuscany, Italy), S. vedranensis (L, 2n = 2x = 18, Es Vedrà, Balearic Islands, Spain), S. villosa (M, 2n = 6x = 54, Gor, Granada, Spain), S. villosa (N, 2n = 4x = 36, Arganda, Madrid, Spain), S. virens (O, 2n = 2x = 18, Fuentenebro, Castile and León, Spain). Individual with an additional metacentric chromosome (P) and a triploid individual (Q, 2n = 3x = 27) of the same population of S. virens. Black arrows point at st chromosomes, green arrows point at m and sm chromosomes showing secondary constrictions, and purple arrows point at st chromosomes showing secondary constrictions. Scale bar = 10 μm.
The studied naturalized population of S. chamaecyparissus is pentaploid (2n = 5x = 45 chromosomes), and it shows 37 m/sm chromosomes and 8 st chromosomes ( and , Table S3). The karyotype of this species is irregular since not all chromosomes can be grouped by five on morphological grounds (). The longest chromosome of the complement is 6.5 μm ± 1.0 μm long on average, and the shortest chromosome is 4.3 μm ± 1.0 μm long on average. THL, CVCL, and MCA mean values are reported in . Secondary constrictions were observed on three sm chromosomes and on a single st chromosome ().
The two studied populations of S. corsica are tetraploid (2n = 4x = 36 chromosomes) and chromosomes can be easily grouped by four on morphological grounds. This species shows 7 groups of four m/sm chromosomes and 2 groups of four st chromosomes ( and , Table S2). THL, CVCL, and MCA mean values are reported in . The longest chromosome of the complement is 5.7 μm ± 1.8 μm long on average, and the shortest chromosome is 4.1 μm ± 0.9 μm long on average. Secondary constrictions were occasionally observed in both populations on sm and st chromosome pairs (–F).
The three studied populations of S. decumbens are diploid (2n = 2x = 18 chromosomes) and show 8 pairs of m/sm chromosomes and one pair of st chromosomes ( and , Table S2). The longest chromosome of the complement is 6.0 μm ± 1.0 μm long on average, and the shortest chromosome is 4.0 μm ± 0.7 μm long on average. THL, CVCL, and MCA mean values are reported in . Secondary constrictions were observed in a single metaphase plate (La Fare-les-Oliviers, Provence-Alpes-Côte d’Azur, France) on a pair of m chromosomes ().
The three studied populations of S. ericoides are diploid (2n = 2x = 18 chromosomes). This species shows 8 pairs of m/sm chromosomes and one pair of st chromosomes ( and , Table S2). The longest chromosome of the complement is 5.6 μm ± 0.9 μm long on average, and the shortest chromosome is 3.7 μm ± 0.6 μm long on average. THL, CVCL, and MCA mean values are reported in . Secondary constrictions were occasionally observed on sm and st chromosome pairs (–J).
The two studied populations of S. etrusca are diploid (2n = 2x = 18 chromosomes) and show 8 pairs of m/sm chromosomes and one pair of st chromosomes ( and , Table S2). The longest chromosome of the complement is 6.8 μm ± 2.0 μm long on average, and the shortest chromosome is 4.5 μm ± 1.0 μm long on average. THL, CVCL, and MCA mean values are reported in . Secondary constrictions were observed in a single metaphase plate (Radicofani, Tuscany, Italy) on a m chromosome and on a pair of sm chromosomes ().
The five studied populations of S. insularis are hexaploid (2n = 6x = 54 chromosomes). The chromosomes of this species can be easily grouped by six on morphological grounds, indeed this species shows 7 groups of m/sm chromosomes and 2 groups of st chromosomes ( and , Table S2). The longest chromosome of the complement is 6.1 μm ± 1.4 μm long on average, and the shortest chromosome is 4.8 μm ± 1.0 μm long on average. THL, CVCL, and MCA mean values are reported in . Secondary constrictions were occasionally observed on sm and st chromosome pairs (–N).
The studied population of S. ligustica is diploid (2n = 2x = 18 chromosomes) and shows eight pairs of m/sm chromosomes and one pair of st chromosomes ( and , Table S2). The longest chromosome of the complement is 5.5 μm ± 0.6 μm on average, and the shortest chromosome is 3.8 μm ± 0.4 μm on average. THL, CVCL, and MCA mean values are reported in . We did not observe secondary constrictions in this species.
The two studied populations of S. magonica are diploid (2n = 2x = 18 chromosomes) and show eight pairs of m/sm chromosomes and one pair of st chromosomes ( and , Table S2). The longest chromosome of the complement is 6.5 μm ± 1.9 μm on average, and the shortest chromosome is 4.6 μm ± 1.5 μm on average. THL, CVCL, and MCA mean values are reported in . Secondary constrictions were detected in two metaphase plates of the population from Tirant (Menorca, Balearic Islands) on a pair of sm chromosomes ( and ).
Figure 3. Karyotype asymmetry in the Santolina chamaecyparissus complex. The scatter plot shows the karyotype asymmetry of each measured metaphase plate (see also Table S1), expressed by Mean Centromeric Asymmetry (on x-axis) and Coefficient of Variation of Chromosome Length (on y-axis) values.
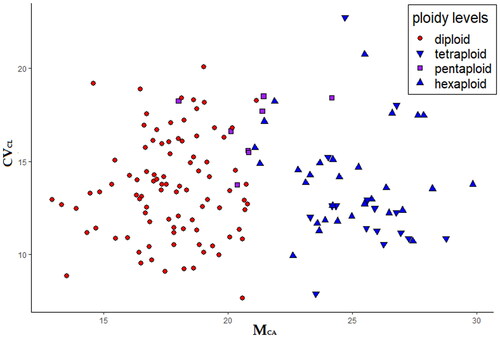
The studied population of S. neapolitana is diploid (2n = 2x = 18 chromosomes) and shows eight pairs of m/sm chromosomes and one pair of st chromosomes ( and , Table S2). The longest chromosome of the complement is 6.2 μm ± 1.0 on average, and the shortest chromosome is 4.6 μm ± 0.5 μm on average. THL, CVCL, and MCA mean values are reported in . We did not observe secondary constrictions in this species.
The studied population of S. pinnata is diploid (2n = 2x = 18 chromosomes) and shows eight pairs of m/sm chromosomes and one pair of st chromosomes ( and , Table S2). The longest chromosome of the complement is 6.1 μm ± 1.0 on average, and the shortest chromosome is 4.4 μm ± 0.6 μm on average. THL, CVCL, and MCA mean values are reported in . We did not observe secondary constrictions in this species.
The studied population of S. vedranensis is diploid (2n = 2x = 18 chromosomes) and shows eight pairs of m/sm chromosomes and one pair of st chromosomes ( and , Table S2). The longest chromosome of the complement is 7.4 μm ± 1.3 on average, and the shortest chromosome is 4.8 μm ± 0.6 μm on average. THL, CVCL, and MCA mean values are reported in . We did not observe secondary constrictions in this species.
The karyotype structure of the two cytotypes of S. villosa is very similar. The chromosomes of this species can be easily grouped by four in tetraploids and by six in hexaploids. In both cases, the monoploid set shows seven m/sm chromosomes and two st chromosomes ( and , Table S2). As regards the tetraploid cytotype, the longest chromosome of the complement is 5.9 μm ± 1.1 μm on average, and the shortest chromosome is 4.5 μm ± 0.6 μm on average. In the studied hexaploid population, the longest chromosome of the complement is 5.9 μm ± 1.4 μm on average, and the shortest chromosome is 4.6 μm ± 0.9 μm on average. Secondary constrictions were occasionally observed on sm and st chromosome pairs (). THL, CVCL, and MCA mean values for both cytotypes are reported in . Mann-Whitney U-Test suggests that there is no significant difference in THL (statistic = 19.5, p = 0.34), CVCL (statistic = 20, p = 0.32), and MCA (statistic = 26, p = 0.03) between the two cytotypes of S. villosa.
The studied population of S. virens shows mostly diploid (2n = 2x = 18 chromosomes) individuals, with a karyotype made by eight pairs of m/sm chromosomes and one pair of st chromosomes ( and , Table S2). The longest chromosome of the complement is 5.8 μm ± 0.8 on average, and the shortest chromosome is 3.5 μm ± 0.7 μm on average. THL, CVCL, and MCA mean values are reported in . We detected a single individual with an additional metacentric chromosome (2n = 19; ) and a single triploid individual with 2n = 3x = 27 chromosomes (). Unfortunately, the three metaphase plates obtained from the triploid individual were good enough for counting chromosomes but not for karyotype reconstruction. We did not observe secondary constrictions in this species.
The scatter plot represented in suggests differences in MCA values among ploidy levels. Overall differences among the four ploidy levels are significant according to Kruskal-Wallis test (statistic = 100.4, p ≪ 0.01). Pairwise Mann-Whitney U-Test with Bonferroni correction suggests that diploids show significantly (p ≪ 0.01) lower MCA values with respect to tetraploids and hexaploids. Santolina chamaecyparissus, the only pentaploid species, shows significantly (p < 0.01) lower MCA values when compared to hexaploids, while there is no significant difference between S. chamaecyparissus and both diploids (p = 0.01, value on threshold) and tetraploids (p = 0.02). There is no significant difference in MCA values between tetraploids and hexaploids. Also, there is no significant difference among ploidy levels concerning CVCL and THL.
Discussion
The chromosome numbers detected so far in the S. chamaecyparissus complex (2n = 18 [19], 27, 36 [37], 45, and 54) point to a basic chromosome number x = 9. These findings confirm the previous studies concerning the genus Santolina (Marchi and D’Amato Citation1973; Marchi et al. Citation1979; Valdés-Bermejo and Antúnez Citation1981) and the subtribe Santolininae (Oberprieler et al. Citation2007); x = 9 is also the most likely ancestral chromosome number inferred for Asteraceae as a whole (Carta et al. Citation2020).
All the continental species except S. villosa are diploid, as also the two species endemic to the Balearic Islands, S. magonica (Mallorca and Menorca) and S. vedranensis (islet of Es Vedrà). Chromosome number was provided for the first time for S. benthamiana and S. vedranensis, that are both diploids. Moreover, a new hexaploid cytotype was found in S. villosa. For all the other species, previously published chromosome numbers are confirmed (see also the distribution map of the studied populations, ) (Marchi and D’Amato Citation1973; Arrigoni Citation1977; Marchi et al. Citation1979; Valdés-Bermejo and Antúnez Citation1981).
With Feulgen staining, secondary constrictions are not always visible. For this reason, we consider with caution the putative differences observed among species in this respect.
Diploid species show a rather homogeneous karyotype structure, despite some (not statistically tested) qualitative difference concerning the visibility/position of secondary constrictions. Polyploids also share a homogeneous karyotype structure, but different from that detected in diploids. Indeed, a significant difference in intra-chromosomal asymmetry (MCA values) is apparent between diploids and polyploids, except for the pentaploid S. chamaecyparissus, which shows a somehow intermediate karyotype structure (). Indeed, considering the haploid (monoploid) chromosome set, all tetraploids and hexaploids show two subtelocentric chromosomes (st), while diploids only one. A similar difference was already documented in the two cytotypes (diploid and tetraploid) of S. pectinata Lag., a member of the S. rosmarinifolia L. complex, endemic to Spain (Rivero-Guerra Citation2008b). The different karyotype structure between diploids and polyploids in the S. chamaecyparissus complex may suggest an allopolyploid origin of the polyploids, involving also other diploid Santolina species, possibly extinct, likely having a different karyotype structure with respect to diploids studied here. However, autopolyploidy cannot be ruled out, since chromosome rearrangements may have occurred after polyploidization. Several studies demonstrated that translocations occur frequently in newly formed polyploids (Lim et al. Citation2008; Wang et al. Citation2012). These chromosomal changes are often involved in the diploidization process of polyploids (Dodsworth et al. Citation2016), to reduce sterility due to abnormal chromosome behavior during meiosis (Weiss and Maluszynska Citation2000; Levin Citation2002).
Irrespective of their allo- or autopolyploid origin, the karyotype structure of the polyploids S. corsica (tetraploid), S. insularis (hexaploid), and S. villosa (both tetraploid and hexaploid) is strikingly similar, since they show 7 metacentric/submetacentric chromosomes and 2 subtelocentric chromosomes in their monoploid complement ( and ). However, despite these polyploid species show a similar karyotype structure, preliminary molecular phylogenetic analyses (Varaldo et al. Citation2021) suggest that S. corsica/S. insularis (endemic to Corsica and Sardinia) and S. villosa (endemic to continental Spain) belong to two distinct clades. As regards this latter species, a tetraploid cytotype was already recorded in four different localities of central-eastern Spain by Valdés-Bermejo and Antúnez (Citation1981), and a new hexaploid cytotype was recorded by us in southern Spain. There is no obvious morphological difference among these cytotypes, which both clearly agree with the descriptions provided by Carbajal et al. (Citation2019) and Giacò et al. (Citation2021). However, the lectotype of S. villosa lacks a precise geographical location (Ferrer-Gallego et al. Citation2021) so that, at the current state of knowledge, it is not possible to establish if this name applies to tetraploid or hexaploid plants. Accordingly, molecular and more detailed morphological studies are needed to clarify the possible taxonomic value of these cytotypes. Despite that, S. villosa was previously misidentified with S. ericoides (Carbajal et al. Citation2019), and both species are very common in central and eastern Spain (). However, they show obvious differences on both morphological (Carbajal et al. Citation2019) and karyological grounds.
Santolina insularis is endemic to southern and central-eastern Sardinia, and all the studied populations are invariably hexaploid. On the contrary, S. corsica, which is endemic to Corsica and north-eastern Sardinia, is invariably tetraploid, fully confirming previous literature data (Marchi and D’Amato Citation1973; Marchi et al. Citation1979). The populations of these two species are allopatric in Sardinia, where S. corsica is reported only for Monte Albo (Angiolini and Bacchetta Citation2003). Although Arrigoni (Arrigoni Citation1982b) suggested that the two species may co-occur in central-eastern Sardinia, our chromosome counts do not support this hypothesis. Preliminary morphometric, seed morpho-colorimetric, and molecular analyses highlight a strong affinity between these two taxa, suggesting that S. corsica and S. insularis could even be considered as two cytotypes of the same species (A. Giacò and collaborators, in preparation).
Santolina chamaecyparissus is confirmed as pentaploid, and this explains the production of aborted pollen grains, as observed by Arrigoni (Arrigoni Citation1977). Its karyotype structure () and MCA values () are intermediate between the diploid and the other polyploid (artioploid) species. Santolina chamaecyparissus is of unknown, likely anthropic, origin and is commonly cultivated, albeit sometimes escapes from cultivation (Greuter Citation2008). According to our results, it is very likely an allopolyploid unit, and its morphology (Arrigoni Citation1977, Citation1982b) and karyotype suggest that at least one of the parental species is related to the lineage of S. corsica/S. insularis. However, the peculiar karyotype structure of S. chamaecyparissus cannot be explained by hypothesizing a simple crossing between any of the existing species in this complex.
Santolina virens is a commonly cultivated plant that is known as native only in Spain (Carbajal et al. Citation2019). According to Pau (Citation1907) and Marcos and Burgaz (Citation1990), S. virens may be a species with hybrid origin involving one species of the S. chamaecyparissus complex and a species of the S. rosmarinifolia complex as putative parental taxa. This hypothesis is strengthened by the karyotype instability suggested by the documentation, in an otherwise diploid species (see also Kyohs, 1982), of a triploid individual and a diploid individual with an additional metacentric chromosome. In addition, in the same collection site of S. virens we also recorded the occurrence of S. ericoides and S. rosmarinifolia, that could represent the putative parental species. Future studies are needed to investigate the putative hybrid status of this species, also in relation with the two sympatric taxa mentioned above.
Conclusion
Karyomorphological studies are still useful to investigate the diversity among species for systematic and taxonomic purposes. In this study, a complete picture of chromosome numbers and karyotype structure within the S. chamaecyparissus complex was presented, relying on 39 populations from all the 14 species of the complex (Table S1 and ). The main result of this study concerns the significant differences in the intra-chromosomal asymmetry (MCA) between diploid and polyploid species (). Indeed, all diploids invariably show only one subterminal chromosome while tetraploid and hexaploid species two. This feature suggests a possible allopolyploid origin of the polyploids, involving also other, possibly extinct, taxa. More evidence for an allopolyploid origin is provided by the peculiar karyotype structure of the pentaploid S. chamaecyparissus.
Supplemental Material
Download PDF (1.4 MB)Acknowledgements
We thank J. Franzoni, S. Chiletti, S. Cambria, and D. Dolci, who helped in the sampling of Italian species; J. Serapio, E. Guasp, and P. Fraga, who helped in the sampling of Spanish species. We also gratefully acknowledge J.-M Tison, who provided detailed information about occurrence localities of French species.
Disclosure statement
The authors declare no conflict of interest.
Additional information
Funding
References
- Afzal-Rafii Z, Vianot J, Ramade M, Bourreil P. 1985. Analyse des caractères caryologiques et écologiques de quelques taxons dans les massifs du Luberon, de Lure et du Mont Ventoux. Rev Cytol Biol Veg Botaniste. 8:33–62.
- Altınordu F, Peruzzi L, Yu Y, He X. 2016. A tool for the analysis of chromosomes: KaryoType. taxon. 65(3):586–592.
- Angiolini C, Bacchetta G. 2003. Analisi distributiva e studio fitosociologico delle comunità a Santolina insularis (Gennari ex Fiori) Arrigoni della Sardegna meridionale (Italia). Fitosociologia. 40:109–127.
- Arrigoni PV. 1977. Santoline italiche nuove: some new Italian santolinas. Webbia. 32(1):129–134.
- Arrigoni PV. 1982a. Santolina L. In: Pignatti S, editor. Flora d’Italia. 1st ed. Bologna, Italy: Edagricole. p. 64–65.
- Arrigoni PV. 1982b. Le piante endemiche della Sardegna. Boll Soc Sarda Sci Nat. 21:338–348.
- Astuti G, Roma-Marzio F, Peruzzi L. 2017. Traditional karyomorphological studies: can they still provide a solid basis in plant systematics? Fl Medit. 27:91–98.
- Bernardo L, Maiorca G, Franzoni J, Roma-Marzio F, Peruzzi L. 2020. A morphometric and karyological study of Onobrychis calabrica (Fabaceae), a neglected species endemic to Calabria, S Italy. Willdenowia. 50(2):217–224.
- Caputo P, Frediani M, Gelati MT, Venora G, Cremonini R, Ruffini Castiglione M. 2013. Karyological and molecular characterisation of subgenus Vicia (Fabaceae). Pl Biosystems. 147(4):1242–1252.
- Carbajal R, Ortiz S, Sáez L, et al. 2019. Santolina L. In: Castroviejo SB, Benedí C, Buira A, editors. Flora iberica. Madrid: Real Jardín Botánico, CSIC.
- Carta A, Bedini G, Peruzzi L. 2020. A deep dive into the ancestral chromosome number and genome size of flowering plants. New Phytol. 228(3):1097–1106.
- Carta A, Peruzzi L. 2016. Testing the large genome constraint hypothesis: plant traits, habitat and climate seasonality in Liliaceae. New Phytol. 210(2):709–716.
- Dodsworth S, Chase MW, Leitch AR. 2016. Is post-polyploidization diploidization the key to the evolutionary success of angiosperms? Bot J Linn Soc. 180(1):1–5.
- Ferrer-Gallego P, Sáez L, Wajer J, Giacò A, Peruzzi L. 2021. Typification of the names Santolina ericoides and S. villosa (Asteraceae) revisited. Phytotaxa. 509(2):233–240.
- Ghosh I, Saha PS, Bhowmick BKJ. Sumita 2021. A phylogenetic analysis of Momordica (Cucurbitaceae) in India based on karyo-morphology, nuclear DNA content and rDNA ITS1-5.8S-ITS2 sequences. Protoplasma. 258(2):347–360.
- Giacò A, Astuti G, Peruzzi L. 2021. Typification and nomenclature of the names in the Santolina chamaecyparissus species complex (Asteraceae). Taxon. 70(1):189–271.
- Greuter W. 2008. Santolina L. In: Greuter W, von Raab-Straube E, editors. Med-checklist: a critical inventory of vascular plants of the circum-mediterranean countries. Geneva, Switzerland: Organization for the Phyto Taxonomic Investigation of the Mediterranean Area (OPTIMA). p. 696–698.
- Guerra M. 2012. Cytotaxonomy: the end of childhood. Pl Biosystems. 146:703–710.
- Kyohs D. 1982. Miscellaneous chromosome numbers in Asteraceae. Madroño. 29(1):62.
- Levan A, Fredga KARL, Sandberg AA. 1964. Nomenclature for centromeric position on chromosomes. Hereditas. 52(2):201–220.
- Levin D. 2002. The role of chromosomal change in plant evolution. New York (NY): Oxford University Press.
- Levitsky GA. 1931. The karyotype in systematics. Bull Appl Bot Genet Pl Breed. 27:220–240.
- Lim KY, Soltis DES, Pamela S, Tate J, Matyasek R, Srubarova H, Kovarik A, Pires JC, Xiong Z, Leitch AR. 2008. Rapid chromosome evolution in recently formed polyploids in Tragopogon (Asteraceae). PLoS One. 3(10):e3353.
- Marchi P, Capineri R, D’Amato G. 1979. Il cariotipo di Santolina corsica Jord. & Fourr. (Compositae) proveniente da Bastia (Corsica) ed altre osservazioni. Ann Bot (Rome). 38:1–13.
- Marchi P, D’Amato G. 1973. Numeri cromosomici per la flora italiana: 145. Inform Bot Ital. 5(150):93–100.
- Marcos N, Burgaz AR. 1990. Santolina virens Miller (= S. viridis Willd.): a plant belonging to the Spanish flora. Anales Jard Bot Madrid. 47:513–516.
- Nardi FD, Pustahija F, Andreucci A, Ciccarelli D, Siljak-Yakovlev S, Peruzzi L. 2018. Does Solidago litoralis (Asteraceae) merit specific rank? Insights from cytogenetic, molecular and ecological data. Phytotaxa. 346(2):121–140.
- Oberprieler C, Himmelreich S, Vogt R. 2007. A new subtribal classification of the tribe Anthemideae (Compositae). Willdenowia. 37(1):89–114.
- Paszko B. 2006. A critical review and a new proposal of karyotype asymmetry indices. Plant Syst Evol. 258(1–2):39–48.
- Pau DC. 1907. Formas nuevas de plantas. Bol Soc Aragonesa Ci Nat. 6:23–30.
- Peruzzi L, Altınordu F. 2014. A proposal for a multivariate quantitative approach to infer karyological relationships among taxa. Comp Cytogenet. 8(4):337–349.
- Peruzzi L, Eroğlu HE. 2013. Karyotype asymmetry: again, how to measure and what to measure? Comp Cytogenet. 7(1):1–9.
- Peruzzi L, Leitch IJ, Caparelli KF. 2009. Chromosome diversity and evolution in Liliaceae. Ann Bot. 103(3):459–475.
- Plummer JA, Shan F, Galwey N, Yan G, Plummer JA, Galwey N. 2003. New methods for comparison of chromosomes within and between species. Caryologia. 56(2):227–231.
- Rivero-Guerra AO. 2008a. Cytogenetics, biogeography and biology of Santolina ageratifolia Barnades ex Asso (Asteraceae: Anthemideae). Bot J Linn Soc. 157(4):797–807.
- Rivero-Guerra AO. 2008b. Cytogenetics, geographical distribution, and pollen fertility of diploid and tetraploid cytotypes of Santolina pectinata Lag. (Asteraceae: Anthemideae). Botan J Linn Soc. 156(4):657–667.
- Rivero-Guerra AO. 2010. Cytogenetics, geographical distribution, pollen stainability and fecundity of Santolina impressa (Asteraceae: Anthemideae). Folia Geobot. 45(1):95–109.
- Stebbins GI. 1971. Chromosomal evolution in higher plants. London: Edward Arnold (Publishers) Ltd.
- Valdés-Bermejo E, Antúnez C. 1981. Estudios caríológicos en especies españolas del género "Santolina" L. ("Compositae"). An Jard Bot Madr. 38:127–144.
- Varaldo L, Casazza G, Guerrina M, Baumel A, Giacò A, Peruzzi L, Carballal R, Sáez L, Domina G, Minuto L. 2021. Prime osservazioni sulla filogenesi e la biogeografia del genere Santolina, con particolare riferimento all’aggregato di S. chamaecyparissus. Notiz Soc Bot Ital. 5 (in press).
- Wang Q, Liu H, Gao A, Yang X, Liu W, Li X, Li L. 2012. Intergenomic rearrangements after polyploidization of Kengyilia thoroldiana (poaceae: Triticeae) affected by environmental factors. PLoS One. 7(2):e31033.
- Weiss H, Maluszynska J. 2000. Chromosomal rearrangement in autotetraploid plants of Arabidopsis thaliana. Hereditas. 133(3):255–261.