Abstract
Gray mold (Botrytis cinerea) is one of the most common diseases of strawberries (Fragaria × ananassa Duchesne) worldwide. Although many chemical fungicides are used for controlling the growth of B. cinerea, the risk of the fungus developing chemical resistance together with consumer demand for reducing the use of chemical fungicides have necessitated an alternative method to control this pathogen. Various naturally occurring microbes aggressively attack plant pathogens and benefit plants by suppressing diseases; these microbes are referred to as biocontrol agents. However, screening of potent biocontrol agents is essential for their further development and commercialization. In this study, 24 strains of yeast with antagonistic ability against gray mold were isolated, and the antifungal activity of the volatile and diffusible metabolites was evaluated. Putative mechanisms of action associated with the biocontrol capacity of yeast strains against B. cinerea were studied through in vitro and in vivo assays. The volatile organic compounds produced by the Galactomyces candidum JYC1146 could be useful in the biological control of plant pathogens and therefore are potential alternative fungicides with low environmental impact.
1. Introduction
Strawberries (Fragaria × ananassa Duch.) are a rich source of essential nutrients, minerals, vitamins, and beneficial phytochemicals, which appear to exert useful biological effects on human health [Citation1,Citation2]. For example, the dietary fibers in strawberries help regulate blood sugar levels by slowing digestion and controlling calorie intake through its satiating effect [Citation3]. In addition, strawberries are a rich source of vitamin C (about 60 mg/100 g fresh fruit) and folate (24 µg/100 g fresh fruit) [Citation1]. Vitamin C is an antioxidant that plays a vital role in many physiological functions [Citation4]. Folate plays a central role in the synthesis of nucleic acids and in the remethylation of homocysteine into methionine [Citation5]. Therefore, consuming moderate amount of strawberries benefits human health. Because of its flavor and eutrophy, consumers worldwide purchase either fresh strawberries or processed strawberries products, such as yogurts, jams, and juices. Thus, in addition to their health benefits, strawberries are also economically and commercially important.
Botrytis rot is a serious disease that affects strawberry production worldwide and is caused by B. cinerea. Botrytis cinerea (teleomorph: Botryotinia fuckeliana), which is also known as gray mold. It is a ubiquitous filamentous fungal pathogen of a wide range of plant species that causes agricultural losses in more than 200 crop species worldwide [Citation6]. The fungus can infect the leaves [Citation7], flowers [Citation8], and fruits of straberry [Citation9]. This disease occurs not only in the field but also during storage, transportation, and marketing of strawberry due to the onset of severe rot as the fruits begin to ripen [Citation10]. Strawberries prefer cooler temperatures; therefore, the timing of their cultivation is crucial in the subtropical areas (e.g., Taiwan). However, B. cinerea prefers to grow at low temperature and high moisture, so the growing season suitable for strawberry harvest is prone to infection by gray mold. This renders B. cinerea a common and destructive disease of strawberries in many countries. Therefore, the management of gray mold is a crucial area of research in the strawberry industry.
The disease cycle starts with an airborne conidium landing on the host surface. Following attachment, the conidium germinates on the host surface and forms the appressorium that penetrates the host surface [Citation11,Citation12]. Botrytis cinerea induces programmed cell death of the host plant, which may be essential for successful infection [Citation13]. Thus, when a primary lesion is formed on the host, host defense responses are triggered [Citation14]. In some cases, the fungus enters a quiescence period of undefined length, during which fungal outgrowth is restricted [Citation15]. When the defense barriers of the plant collapse, the fungus grows vigorously, which leads to rapid maceration of plant tissue. The fungus ultimately sporulates on the tissue to produce inoculum for the next infection [Citation11].
Strawberries are also susceptible to several other fungal pathogens that require specific chemical control measures [Citation16,Citation17]. Antagonistic microorganisms as a biological control agents have been suggested as a practicable method to reduce the occurrence of various diseases on strawberries [Citation18,Citation19]. Microorganisms often used for developing biocontrol agents are yeasts, bacteria, and fungi. Compared with bacteria, the genome of yeasts is more stable. Some yeast species have been reported to be effective agents for the suppression of gray mold on strawberry [Citation20,Citation21]. Several mechanisms have been proposed for the biological control of pathogenic fungi [Citation22]. Biological control relies on multiple mechanisms that can be achieved by using either a single biocontrol agent exhibiting several mechanisms or applying more than one biocontrol agent in a mixture that exerts combined effects. With this information, in this study, we aimed to (a) screen and identify the species of yeast antagonistic to B. cinerea and (b) evaluate the putative mechanisms of action associated with the biocontrol capacity of yeast strains against B. cinerea and some other postharvest pathogens through in vitro and in vivo tests.
2. Materials and methods
2.1. Fungal inoculum
Botrytis cinerea (strain JYC2142) and other pathogens (Colletotrichum gloeosporioides, Fusarium incarnatum, and B. cinerea JYC2143) used in this study were isolated from rotten strawberries collected from northern and central regions of Taiwan. Yeast strains for screening of metabolites that contribute to antagonistic effects were isolated from various niches, including wine, flowers, soil, insects, and fruit etc. Both yeast and pathogens were grown on YPD agar plates containing 1% Yeast extract, 2% Peptone, 2% Dextrose (D-glucose), and 2% agar. The candidate yeasts and pathogens including Col. gloeosporioides, and F. incarnatum were incubated at 28 °C; B. cinerea was incubated at 22 °C.
2.2. In vitro screening of yeast isolates for antagonism to B. cinerea strain JYC2142
To evaluate the interactions between the antagonists and the pathogens in culture, gray mold was cocultured with each yeast isolate on YPD agar plates. Petri dishes containing 15 mL of YPD agar medium were inoculated in three replicates with 6 × 6 mm2 agar discs with mycelium of pathogens that had been obtained from the margins of young colonies of the fungus grown on the same medium. Approximately 20 μL of yeast suspension (OD660 = 0.8, ∼1.26 × 107 cells/mL) was inoculated in the middle of agar plate and the plate was sealed with parafilm. A control dish was prepared to compare only the inoculation of the pathogenic fungus. The cultures were incubated at 22 °C for 6 days. All cultures were kept in the dark.
2.3. Assays of diffusible metabolite and volatile compounds
After screening the antagonistic yeasts on agar plates, the inhibition compounds produced from them were further tested to understand whether they are diffusible or volatile substances. Production of diffusible substances was tested using the dual culture method in which 20 μL of yeast suspension (OD660 = 0.8, ∼1.26 × 107 cells/mL) was seeded onto plates containing YPD agar near the disk edge. Pathogenic mycelium (6 × 6 mm2) was inoculated at the opposite edge (modified from Lopes et al. [Citation23]; ). After incubating the pathogenic fungus and yeast antagonists at 22 °C for about 5 days, fungal growth was measured. The inhibition rate was calculated as [(Rc – Rexp)/Rc] × 100%, where Rc represents the longest diameter of fungal mycelium and Rexp is the horizontal diameter of the pathogenic fungus, which shows the inhibitory effect. Three replications were evaluated for each treatment and the experiment was repeated two times. All cultures were kept in the dark.
Figure 1. Diffusible metabolite assay in dual culture. Inhibition rate was calculated as [(Rc − Rexp)/Rc] × 100%, where Rc represents the longest diameter of the fungal mycelium and Rexp the horizontal diameter of the pathogenic fungus (which show the inhibitory effect).
![Figure 1. Diffusible metabolite assay in dual culture. Inhibition rate was calculated as [(Rc − Rexp)/Rc] × 100%, where Rc represents the longest diameter of the fungal mycelium and Rexp the horizontal diameter of the pathogenic fungus (which show the inhibitory effect).](/cms/asset/2b60f531-0665-42b8-af46-73457d0c0ffa/tmyb_a_1454013_f0001_c.jpg)
To assess the production of volatile compounds, 20 μL of yeast suspension (OD660 = 0.8, 1.26 × 107 cells/mL) was inoculated on one plate and a disk of pathogen mycelium (6 × 6 mm2) was inoculated on the other plate. The two plates were placed “mouth to mouth,” and wrapped together with parafilm. Mycelial growth was observed after incubating the plates at 22 °C for 5 days. To study the efficacy of the selected antagonist on the growth inhibition of the pathogenic fungus, radial mycelium growth in two vertical directions chosen randomly was measured [Rexp and Rc, which represent the diameter of fungal mycelium with yeast and in the absence of yeast (control), respectively]. The inhibition rate was calculated according to the following equation: [(Rc2 – Rexp2)/Rc2] × 100% (). Three replications were evaluated for each treatment and the experiment was repeated two times. All cultures were kept in the dark.
Figure 2. Detection of volatile compounds secreted from antagonistic yeasts according to the ‘‘mouth-to-mouth” method. To study the efficacy of selected antagonist on the growth inhibition of pathogenic fungus, radial mycelia growth in two vertical directions randomly chosen was measured (Rexp and Rc, which represents the diameter of fungal mycelium with yeast (upper panel) and in the absence of yeast (control, lower panel), respectively). Inhibition rate was calculated as [(Rc2 − Rexp2)/Rc2] × 100%.
![Figure 2. Detection of volatile compounds secreted from antagonistic yeasts according to the ‘‘mouth-to-mouth” method. To study the efficacy of selected antagonist on the growth inhibition of pathogenic fungus, radial mycelia growth in two vertical directions randomly chosen was measured (Rexp and Rc, which represents the diameter of fungal mycelium with yeast (upper panel) and in the absence of yeast (control, lower panel), respectively). Inhibition rate was calculated as [(Rc2 − Rexp2)/Rc2] × 100%.](/cms/asset/094e1677-4f00-44f0-adc3-a2aff497cce0/tmyb_a_1454013_f0002_c.jpg)
2.4. Production of hydrolytic enzymes (chitinase, protease, and cellulase)
The ability to synthesize fungal cell wall-degrading enzymes, which suppress the growth of fungal pathogens, can further enhance the biocontrol efficacy of VOC-producing antagonists. Thus, the capability of the selected yeasts to produce and secrete fungal cell wall lytic enzymes (chitinase, protease, and cellulase) was tested on solid media containing the corresponding substrates: (a) chitinase was tested on colloidal chitin (Sigma) pH = 4.7 amended with mineral salts [Citation24]; (2) protease on an agar plate containing skimmed milk power (28 g/L), tryptone (5 g/L), yeast extract (2.5 g/L), glucose (1 g/L), and bacterial agar (15 g/L; modified from Dunne et al. [Citation25]); (3) cellulase on an agar plate containing yeast extract (10 g/L), peptone (20 g/L), carboxymethyl cellulose (CMC) sodium salt (10 g/L; Alfa Aesar), and agar (20 g/L) [Citation26]. All prepared plates were inoculated with 3 μL of the respective yeast culture suspension for 24 h. All enzymatic activities were evaluated after incubation at 28 °C for 5 days. After incubating, the plates for the cellulase assay were stained with 0.1% Congo red (Sigma) solution for 30 min and then washed with 1 M NaCl solution for 15 min. Chitinase activity was detected by the presence of a purple zone () around yeast colonies. Protease () and CMCase () activities were detected by the presence of degradation halos around the colonies. The protease and CMCase activity among the isolates was compared based on a ratio calculated according to the following formula: R/r, where R is the diameter of the entire clear zone and r is the diameter of the zone of yeast colony ().
Figure 3. Detection of hydrolytic enzymes activity of antagonistic yeasts based on their reactions in a culture medium containing the substrate of the candidate enzymes. (A) Extracellular chitinase activity was detected on colloidal chitin media containing bromocresol purple (pH 4.7). (B) Detection of protease activity of antagonistic yeasts based on culturing yeast in a culture medium containing casein. (C) CMCase activity was detected on carboxymethylcellulose media. (D) Comparison of protease and CMCase was based on the following ratio: R/r, where R is the diameter of the entire clear zone and is the diameter of the zone with yeast colonies.
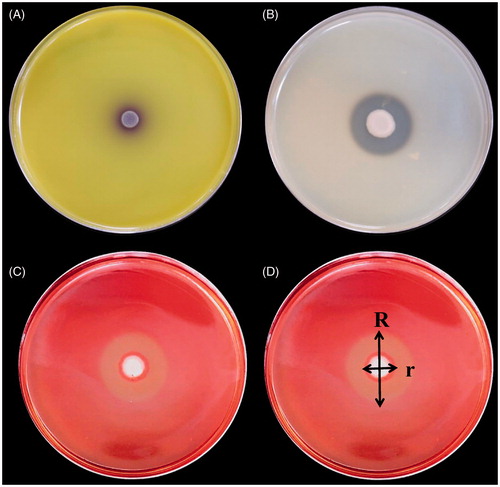
2.5. Biofilm-forming capacity
The ability to form biofilms has been proposed as a possible mechanism of biocontrol in some antagonist yeasts [Citation27]. One of the most important aspects of biofilm formation is the initial attachment of microorganisms because this is a necessary first step. Thus, the biofilm-forming capacity was tested by measuring yeast adherence to a polystyrene surface according to the method reported by Giobbe et al. [Citation28], but with slight modifications. The method involved the following steps: the yeast was grown overnight at 28 °C in a tube containing 3-mL YPD medium. The yeast suspension (1 mL) was transferred to an Eppendorf tube and brought to an OD660 nm of 0.2. The yeast culture was then centrifuged to remove supernatants. Cells of each yeast strain were then resuspended in 1-mL YPD medium, and 200-μL aliquots were inoculated in triplicates into 96-well polystyrene plate. A well with only the YPD medium was used as the control. The plate was incubated at 22 °C for 2 days. After incubation, the wells were washed with sterile water to remove the cells that were not attached to the wells. Moreover, 200 μL aliquots of 0.1% (w/v) crystal violet (Panreac AppliChem, Germany) were then added. After 30 min, the wells were washed with sterile water for about eight times to remove the redundant dye. The adherence of cells was quantified by solubilizing the retained crystal violet with 200 μL of 10% sodium dodecyl sulfate (SDS) for 30 min. Finally, the OD570 nm of the solution was measured using a Multiskan GO Microplate Spectrophotometer (Thermo Scientific).
2.6. Detection of inhibition of mycelia growth on strawberry fruit
The efficacy of the volatiles produced by antagonistic yeast in the postharvest control of B. cinerea on strawberry was determined according to the method suggested by Huang et al. with some modifications [Citation20]. Aliquots (100 μL) of the yeast cell suspension (approximately 1 × 107 yeast cells/mL) were spread on YPD agar plates (9 cm in diameter). The covers of the Petri dishes were removed and two (or four) plates with the yeast cultures were placed in each plastic box. For the control treatment, two or four plates with YPD agar only were placed in a box. Mature and healthy strawberry fruits were purchased from a market. Five unwounded strawberry fruits of similar size were surface sterilized in 70% ethanol for 5 s, washed two times with sterile distilled water, and blotted on paper towels to remove the water. A conidial suspension (100 μL) of B. cinerea at approximately 1 × 107 conidia/mL was spread on each strawberry. Both the fruit and yeast culture plates were then placed into a plastic box (). The experiment was conducted with a completely randomized design with three replicates per treatment (two or four dishes of yeast culture), five fruits in each replicate, and the experiment was repeated two times. The boxes were covered to maintain high relative humidity, which favors the postharvest onset of the disease. The boxes were stored in an incubator for 4 or 8 days at 22 °C or for 20 or 30 days at 4 °C. Strawberry fruits showing symptoms of gray mold in each box were individually rated for disease severity using a scale of 0–8, where 0 represents healthy and 1, 2, 3, 4, 5, 6, 7, and 8 represent less than 12.5, 12.6–25.0%, 25.1–37.5%, 37.6–50.0%, 50.1–62.5%, 62.6–75.0%, 75.1–87.5%, and 87.6–100% of the area rotted, respectively.
Figure 4. Efficacy of the volatiles produced by antagonistic yeast in postharvest control of B. cinerea on strawberry. Yeast cultures and strawberries with B. cinerea spores on the surface were placed into a sealed plastic box at high relative humidity to ensure favorable conditions for the postharvest onset of the disease.
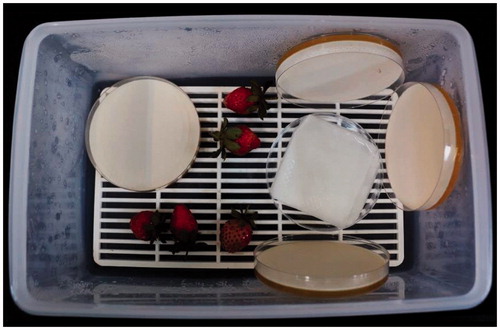
2.7. Fungal genomic DNA extraction
Young fungal cultures (1 mL) were transferred to a 1.5-mL tube and centrifuged at 13,000 g–16,000 g for 1 min. The supernatant was discarded, and the cell pellet was suspended in 200 µL of a lysis buffer [2% Triton X-100, 1% SDS, 100 mM sodium chloride, 10 mM Tris (pH 8.0), and 1 mM ethylenediaminetetraacetic acid (EDTA)] to which 200 µL of phenol–chloroform–isoamyl alcohol (25:24:1; isoamyl alcohol is optional) and 0.3 g of acid-washed glass beads (0.45–0.52 mm) were added and gently mixed. The samples were vortexed for 5 min to disrupt the cells and then centrifuged at 13,000 g–16,000 g for 5 min. The aqueous layer of each sample was then transferred to a clean tube, followed by the addition of 400 µL of 95% ethanol and 16 µL of 3 M sodium acetate (pH 5.2). The samples were mixed through inversion and centrifuged at 13,000 g–16,000 g for 5 min. The pellets were then washed with 300 µL of 70% ethanol, and the samples were centrifuged at 13,000 g–16,000 g for 2 min before the supernatant was discarded. Subsequently, the ethanol solution was aspirated with air for 30 min to dry the pellets. Finally, genomic DNA from each sample was suspended in 100 µL of a Tris–EDTA buffer (pH 8.0).
2.8. Fungal identification
Sequences of the ribosomal DNA (rDNA) were determined from polymerase chain reaction (PCR) products from genomic DNA extracted from the yeast cells. The LSU rDNA, including the D1/D2 domain, 5.8S rDNA, and internal transcribed spacer (ITS) regions, were amplified using PCR with the universal primers ITS-1 (5′-TCCGTAGGTGAACCTGCG-3′) and NL-4 (5′-GGTCCGTGTTTCAAGACGG-3′) [Citation29]. PCR was performed as follows: initial denaturation at 95 °C for 5 min and repeated denaturation at 95 °C for 1 min (annealing temperature, 48–55 °C for 30 s), and elongation at 72 °C for 1 min and 40 s for 35 cycles. The final elongation lasted for 5 min. The DNA sequencing of these samples (∼650 base pair product) was performed at Tri-I Biotech, Inc. A BLAST search of the nucleotide sequences was performed against the National Center for Biotechnology Information database (http://www.ncbi.nlm.nih.gov). Our identification of fungi were also made by using a combination of morphological, cultural, and molecular characters. The voucher specimens of pathogenic fungi are deposited in the Bioresource Collection and Research Center, Hsinchu City, Taiwan (http://www.bcrc.firdi.org.tw/).
2.9. Statistical analysis
Data are expressed as mean ± standard deviation (SD). The significance of differences between each treatment and control was determined using one-tailed Student t tests and analysis of variance. p < .05 was considered statistically significant.
3. Results
3.1. Production of diffusible and volatile substances on agar plates
In our study, 918 yeast strains were isolated from different natural sources and screened for antagonistic activity. Among these, 24 isolates were antagonistic to B. cinerea JYC2142 (). We further evaluated the inhibition compounds from yeast strains to understand whether they are diffusible or volatile. If an inhibition gap was noted between the gray mold and yeast colonies, the yeast strain was recognized as having the ability to produce diffusible inhibition compounds (). The inhibition of mycelial growth of B. cinerea JYC2142 in dual cultures due to the production of diffusible antifungal compounds was observed for six antagonistic yeasts. The inhibition rate varied from 18.7 to 36.5%, with Aureobasidium sp. JYC1525, Saccharomyces cerevisiae JYC137, and Candida stellimalicola JYC2120 having the best ability for diffusion inhibition (36.5, 26.8, and 24.5%; ). Production of volatile antifungal compounds was observed in almost all of these 24 antagonistic yeast strains (from 2.7 to 69.6%, with 14 isolates having statistical significance), with Galactomyces candidum JYC1146, Aureobasidium pullulans JYC1278, and A. pullulans JYC1291 having the best ability for volatile inhibition (69.6, 33.7, and 23.6%; ).
Figure 5. Evaluation of in vitro antagonism by production of diffusible substances by yeasts against the B. cinerea JYC2142 on YPD plate. (A) B. cinerea alone as the control group. (B) Yeasts (right) were antagonistic to B. cinerea (left). (C) Yeasts (right) were not antagonistic to B. cinerea (left).
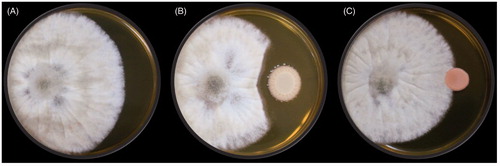
Table 1. Antagonistic yeasts with the accession numbers of large subunit (LSU) of ribosomal DNA sequences.
Table 2. In vitro antagonistic activity assays of antagonistic yeast.
On the basis of the results of antagonistic assays against B. cinerea JYC2142, we chose three antagonistic yeast strains (Gal. candidum JYC1146, A. pullulans JYC1278, and A. pullulans JYC1291) that had the best antagonistic ability for volatile inhibition and three strains (Aureobasidium sp. JYC1525, S. cerevisiae JYC137, and Can. stellimalicola JYC2120) that had the best antagonistic ability for diffusible inhibition to test whether they could also inhibit other pathogens isolated from rotten strawberries (). Diffusible compounds from all six strains did not inhibit the mycelial growth of F. incarnatum; however, five of these except S. cerevisiae JYC137 did inhibit the mycelial growth of Col. gloeosporioides by secreting diffusible compounds. Moreover, the mycelial growth inhibition of B. cinerea JYC2143 by diffusible antifungal compounds from yeasts was noted in S. cerevisiae JYC137, Aureobasidium sp. JYC1525, and Can. stellimalicola JYC2120 (). In the antagonistic activity assays of antagonistic yeast against other pathogenic fungi by volatile compounds in vitro, volatile antifungal compounds from Gal. candidum JYC1146, A. pullulans JYC1278, and A. pullulans JYC1291 exhibited broad inhibitory activity against various pathogenic fungi. The volatile compounds from S. cerevisiae JYC137 inhibited the growth of F. incarnatum and B. cinerea JYC2143. The volatile compounds from Aureobasidium sp. JYC1525 and Can. stellimalicola JYC2120 showed the narrowest inhibitory activity of all. Their volatile compounds inhibited the growth of only B. cinerea JYC2143 ().
Table 3. Pathogens of strawberry with the accession numbers of ITS1-5.8S-ITS2 and partial large subunit (LSU) of ribosomal DNA sequences.
Table 4. In vitro antagonistic activity assays of antagonistic yeast against other pathogenic fungi by diffusible compounds.
Table 5. In vitro antagonistic activity assays of antagonistic yeast against other pathogenic fungi by volatile compounds.
3.2. Production of cell wall-degrading enzymes (chitinase, protease, and cellulase)
Extracellular chitinase activity was detected on colloidal chitin media containing bromocresol purple (pH 4.7). When inoculated with chitinolytic yeasts, the color of zone the surrounding the inoculated fresh culture plugs in the region of chitin utilization changed from yellow to purple (). The color changed because of breakdown of chitin into N-acetyl glucosamine, which caused a corresponding shift in pH toward alkalinity, changing the color of the pH indicator dye (BCP). In our study, nine strains exhibited chitinase activity (). Extracellular protease activity was detected on skimmed milk agar, which was indicated by the appearance of clearing zones due to casein degradation. The activity unit (AU) of enzymatic production was defined as the diameter of the entire clearing zone divided by the diameter of yeast colonies. Six yeast strains produced protease, with the AU ranging from 1.25 to 1.98; A. pullulans JYC1524 exhibited the strongest activity ().
The cell wall of most fungi consists of complex contents, such as chitin, glucan, and mannoprotein. However, the cell walls of oomycetes are composed of cellulose and glucan. Oomycetes are a group of several hundred species of organisms containing some of the most devastating plant pathogens [Citation30]. The oomycete pathogens associated with crown and root diseases of strawberry have been reported in several studies [Citation31,Citation32]. Many species in the genus Phytophthora of oomycetes are specialized to infect strawberry [Citation32–34]. Therefore, the cellulase-producing ability of yeasts may be the mechanism responsible for the antagonistic activity of these strains against these plant pathogens. In our study, the carboxymethyl cellulase (CMCase) activity was observed in eight strains, with the AU ranging from 1.69 to 2.81; A. pullulans JYC1061 exhibited the strongest activity ().
Among the three strains that had best diffusible inhibition ability, Aureobasidium sp. JYC1525 had the ability to produce chitinase and CMCase but S. cerevisiae JYC137 and Can. stellimalicola JYC2120 did not have the ability to secreting cell wall-degrading enzymes we tested. Thus, in these two yeast isolates, other substances might be responsible for diffusible inhibition against gray mold. Furthermore, among the three strains that had best volatile inhibition ability, Gal. candidum JYC1146 had the ability to secrete chitinase, A. pullulans JYC1278 had the ability to secrete protease and CMCase, and A. pullulans JYC1291 had the ability to secrete all cell wall-degrading enzymes we tested ().
3.3. Biofilm-forming capacity
We chose three antagonistic yeast strains (Gal. candidum JYC1146, A. pullulans JYC1278, and A. pullulans JYC1291) that had the best volatile inhibition ability and six strains (Aureobasidium sp. JYC1525, S. cerevisiae JYC137, and Can. stellimalicola JYC2120, S. cerevisiae JYC138, A. pullulans JYC2041, and A. pullulans JYC1061) that had the best diffusible inhibition ability to test whether they had biofilm-forming capacity. Experiments of formation of biofilms demonstrated that Aureobasidium sp. JYC1525 had the best film-forming capacity, as the concentration of crystal violet solubilized by the yeast adhering to the well was higher than 0.002. Gal. candidum JYC1146, A. pullulans JYC1278, A. pullulans JYC1291, A. pullulans JYC2041, and A. pullulans JYC1061 had moderate film-forming ability, as the concentration of crystal violet was higher than 0.0005 but less than 0.002. S. cerevisiae JYC137, S. cerevisiae JYC138, and Can. stellimalicola JYC2120 almost did not have the ability to form biofilm, as the concentration of crystal violet was less than 0.0005 ().
Figure 6. Extracellular chitinase activity was detected on colloidal chitin media containing bromocresol purple (pH 4.7). Three antagonistic yeast (JYC1146, JYC1278, and JYC1291) that had best ability of volatile inhibition and other six strains (JYC1525, JYC137, JYC2120, JYC138, JYC2041, and JYC1061) that had ability of diffusible inhibition to test if they had biofilm-forming capacity. Ability evaluated as the concentration of crystal violet solubilizing from the retained yeast adhered to the well.
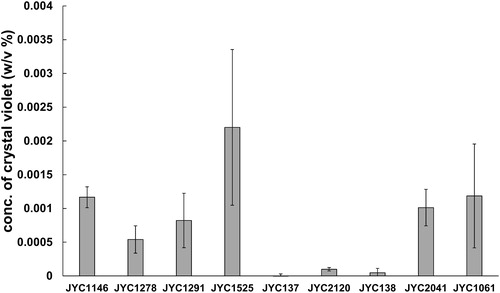
3.4. Efficacy of the antagonistic yeast for the control of B. cinerea on strawberry
To evaluate the biocontrol capacity of each yeast strain in vivo, four petri dishes with yeast culture and five strawberries with spores of B. cinerea on the surface were incubated together in a sealed plastic box. We chose three antagonistic yeasts (Gal. candidum JYC1146, A. pullulans JYC1278, and A. pullulans JYC1291) that had the best volatile inhibition ability to test their efficacy in controlling B. cinerea growth on strawberry. Gal. candidum JYC1146 was the most efficient strain that significantly reduced the disease severity (). To evaluate the effective concentration, the number of petri dishes was reduced to two. Comparing the results of two-plate and four-plate treatments (), the inhibitory effect was shown to decline as the number of petri dishes decreased. Because the strain Gal. candidum JYC1146 shows the best efficacy of gray mold inhibition in vivo, we further tested its inhibitory effect against B. cinerea on strawberries at 4 °C, which is the temperature of cold storage of strawberry. However, the yeast did not exhibit obvious effects when incubated at 4 °C (data not shown).
Figure 7. Biocontrol efficacy of the three selected antagonistic yeasts that had best ability of volatile inhibition on the decay of strawberries caused by B. cinerea. (A–C) evaluating the efficacy of inhibition of gray mold infection on strawberries by four petri dishes with strain Galactomyces candidum JYC1146, Aureobasidium pullulans JYC1278, and A. pullulans JYC1291 cultures, respectively. (D–F) evaluating the efficacy of inhibition of gray mold infection on strawberries by two petri dishes with strain Gal. candidum JYC1146, A. pullulans JYC1278 and A. pullulans JYC1291 cultures, respectively. E1 and E2 represents two repeats of the experiment set with yeast treatment. C1 and C2 represents two repeats of the experiment set without yeast treatment.
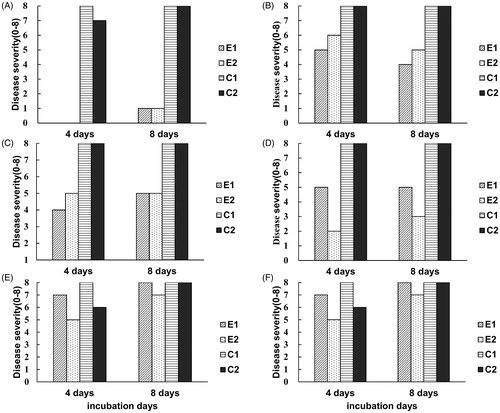
4. Discussion
Because of concerns of chemical resistance and environmental contamination, consumer demand for biological control agents is increasing. Biological control refers to the use of natural enemies (i.e., biological control agents) to reduce populations of pests and plant pathogens. In this present study, we demonstrated that some yeast strains can inhibit the growth of B. cinerea. The inhibitory effects of yeast strains on the mycelial growth were tested in vitro for detecting the potential production of diffusible metabolites and VOCs.
Various mechanisms, such as the enzyme degradation of cell walls, have been proposed for biological suppression of infection and inoculum potential of plant pathogens. In our study, among the selected strains that had the best diffusible inhibition ability, Aureobasidium sp. JYC1525 had the ability to produce chitinase and CMCase. Furthermore, among the selected strains that had the best volatile inhibition ability, Gal. candidum JYC1146 showed the ability to secrete chitinase, A. pullulans JYC1278 the ability to secrete protease and CMCase, and A. pullulans JYC1291 the ability to secrete all cell wall-degrading enzymes tested in this work. Parafati et al. [Citation35] demonstrated that some fungal isolates, namely, S. cerevisiae, Wickerhamomyces anomalus, Metschnikowia pulcherrima, and A. pullulans, isolated from different food sources have the ability to inhibit the growth of B. cinerea. In these species, the competition for iron and the ability to form biofilm and to colonize fruit wounds were hypothesized as the main action mechanisms for M. pulcherrima. Furthermore, all A. pullulans and W. anomalus strains studied could hydrolyze laminarin (b-1,3-glucanase activity); all A. pullulans strains also showed pectinolytic and proteolytic (skim milk and gelatin) activities. Thus, the production of hydrolytic enzymes was one of the important mechanisms for biocontrol activity exhibited by A. pullulans and W. anomalus, which also showed an increased ability to form biofilm.
In agriculture, fungal VOCs have been as part of biological control strategies to prevent the growth of plant pathogens [Citation36]. In our work, volatile antifungal compounds were produced by almost all of the 24 antagonistic yeast strains studied. The strain Gal. candidum JYC1146 inhibited the growth of B. cinerea most evidently, and it could even inhibit several fungal pathogens, including F. incarnatum and Col. gloeosporioides. In Parafati et al. [Citation35], the production of VOCs with in vitro and in vivo inhibitory effect on pathogen growth was observed for the antagonistic species W. anomalus, S. cerevisiae, and M. pulcherrima. Huang et al. [Citation20] showed that the incidence and severity of Botrytis fruit rot of strawberry was significantly reduced by exposure of the strawberry to the volatiles from Can. intermedia cultures or Can. intermedia-infested strawberry fruit. Emerging evidence suggests that bacterial VOCs can directly inhibit fungal growth. Volatiles generated by Bacillus subtilis JA have been demonstrated to significantly inhibit both spore germination and the elongation of germ tubes in B. cinerea [Citation37]. Li et al. [Citation38] showed that the volatile substances produced by Streptomyces globisporus JK-1 can exert significant inhibitory effects on mycelial growth, sporulation, and conidial germination of B. cinerea. These studies on the antagonistic activity of fungi and bacteria to fungal pathogens continue highlight their potential as biobased fumigants. On the other hand, the VOCs released by microorganisms may act directly or indirectly to activate plant immunity or regulate plant growth and morphogenesis [Citation39]. In Su et al. [Citation40], they indicated that rhizomorphs of Marasmius crinisequi are able to induce defoliation of tea twigs by releasing volatile substances. It means plants are able to recognize microbe-derived compounds and adjust their defense and growth responses according to the type of microorganism encountered.
In our study, we found that some antagonistic yeasts have the ability to produce volatile compounds, some have diffusible inhibitory compounds, some have secret hydrolytic enzymes (chitinase, protease and CMCase), and others have the ability to form biofilm. However, no yeast possessed all the mechanisms we assayed. Zangoei et al. analyzed the effects of yeast biocontrol agents Can. membraniciens A4 and A5 and Pic. guilliermondii A6 as well as bacteria biocontrol agents Bacillus subtilis B2 and B6 individually or in combination on gray mold of apple [Citation41]. The combinations of B2 + A5 and B6 + A6 was more effective in control of gray mold of apple in vivo than their individual applications. Moreover, the proportions of the antagonist yeast in the mixture influenced the biocontrol effect. However, the biocontrol effect was weak when using B2 + A6 compared with their individual application. These results suggest that yeast or bacterial antagonists are more effective in biological control of apple gray mold when used in appropriate combinations than when used alone. Hence, a potential method to enhance the effect of biocontrol is through combining the antagonistic yeast in our study with other bacteria or yeast. However, the biocontrol effect should be further tested to determine whether the mixture can exhibit improved biocontrol efficacy.
We found that S. cerevisiae JYC137, S. cerevisiae JYC138, and Can. stellimalicola JYC2120 can secret diffusible compounds to inhibit the growth of gray mold; however, they could not secret any hydrolytic enzymes we had assayed, indicating that there might be other substances involved in diffusible inhibition against gray mold by these yeasts. The production of killer toxins is a well-established phenomenon in yeasts, and the killer phenomenon was first discovered in S. cerevisiae in 1963 [Citation42]. Killer toxins are proteinaceous substances produced by some groups of yeast called “killer yeasts.” Some yeast strains that have the killer ability could secret killer toxin to inhibit the growth of other microbes that were sensitive to the toxin. Santos and Marquina [Citation43] demonstrated the protection of Vitis vinifera plant against B. cinerea by the killer toxin of Pichia membranifaciens and its possible use as a biocontrol agent against gray mold disease of grapevine. The toxins from S. cerevisiae are of five types: K1, K2, K3, K28, and Klus [Citation44]. No studies have yet investigated the use of the purified toxin of killer yeast against gray mold; nevertheless, such use might be a possible strategy involved in diffusible inhibition by the yeast. In our previous study, Can. stellimalicola was found to not only suppress the growth of gray mold but also inhibit the growth of human pathogen Pseudozyma aphidis [Citation45]. However, Can. stellimalicola showed the antagonistic behavior to P. aphidis only at pH 4.7 and pH 5, indicating that this antagonistic activity has a narrow optimal pH range; this likely shows the application limits of this strain.
Biofilms are a consortium of microorganisms encased in an exopolymeric matrix and attached to a surface. Biofilm formation is considered an important attribute for antagonist microbes, as it helps to successfully colonize and protect both the wound site and the intact fruit. In our study, S. cerevisiae JYC137, S. cerevisiae JYC138, and Can. stellimalicola JYC2120 showed weak capability to form biofilms. Mass production of antagonistic microbes and its formulation with an appropriate carrier are the critical steps to develop biofungicides with extended shelf life and prolonged activity. This means that the formulation types must be optimized to improve the adherence of the antagonistic yeast. Fungicides can be formulated in several ways depending on their physical characteristics and application methods [Citation46]. Kim et al. [Citation47], various formulations of antagonist B. licheniformis N1 were generated and evaluated for their activity to control strawberry gray mold. Per their study, the wettable powder-type formulation N1E (a laboratory-made formulation) was used in pot experiments with remarkable disease control activity on both strawberry leaves and flowers. Alternatively, the use of mixed biofilms containing both fungi (filamentous or non-filamentous) or bacteria can be considered a practical strategy to improve the attachment. This arrangement differs from mixed communities, as in a biofilm, in that the microorganisms form structured communities held together by an extracellular matrix of microorganism-derived macromolecules that have physical and physiological properties distinct from those of free-living cells. The interactions between microbes are crucial for mutual metabolic cooperation. Bacteria and fungi can form a range of physical interaction that depend on different modes of molecular communication for their development and functioning. The formation of fungal–bacterial biofilms by bacterial colonization on fungal surface has been hypothesized to give the biofilm enhanced metabolic activities compared with monocultures [Citation48]. Seneviratne et al. [Citation49] reviewed relevant studies and summarized that fungal–bacterial/fungal–rhizobial biofilms are more effective in their biological performance than are monocultures.
Finally, in the assay of the efficacy of the antagonistic yeast in control of B. cinerea on strawberry, Gal. candidum JYC1146 showed the best ability to reduce the infection. Gal. candidum was a filamentous yeast-like fungus and was reported to be an pathogen that caused sour rot on numerous fruit and vegetable [Citation50]. Although the fungi was not obviously pathogenic to strawberry, the risk of applying this fungi as biocontrol agent should be considered.
5. Conclusions
Botrytis rot, caused by the wide-spread fungus B. cinerea, affects most vegetable and fruit crops, as well numerous shrubs, trees, flowers, and weeds. Biological control by antagonistic yeasts is a promising strategy for reducing the common use of synthetic fungicides to control this pathogen. In total, 918 yeast strains, isolated from different natural sources were screened in vitro for their potential antifungal activity against B. cinerea. The inhibitory effects of the yeast strains on the mycelial growth were tested in vitro for detecting the potential production of diffusible metabolites and VOCs. Furthermore, some strains among the most effective ones were selected and evaluated in vivo to test the postharvest biological control of gray mold decay of strawberry with B. cinerea. Some of the yeast strains strongly inhibited B. cinerea mycelial growth under in vitro conditions and significantly reduced rot severity in vivo. This study demonstrated that antagonistic yeast strains inhibited B. cinerea and that they have high potential for use in sustainable strawberry production. It would be interesting to develop the most effective strains for the protection not only of fruits but also leaves or whole plants of strawberry against pathogens in future studies.
Acknowledgments
The authors would like to thank the referees for their valuable comments which helped to improve the manuscript. The authors would also like to thank Prof. Pi-Han Wang (Tunghai University, Taiwan) and Prof. Shih-Feng Fu (National Changhua University of Education, Taiwan) for their detailed comments and suggestions for the manuscript. We thank the members of the Chou Lab for their contribution to the manuscript. This manuscript was edited by Wallace Academic Editing.
Disclosure statement
No potential conflict of interest was reported by the authors.
Additional information
Funding
References
- Giampieri F, Tulipani S, Alvarez-Suarez JM, et al. The strawberry: composition, nutritional quality, and impact on human health. Nutrition. 2012;28:9–19.
- Giampieri F, Forbes-Hernandez TY, Gasparrini M, et al. Strawberry as a health promoter: an evidence based review. Food Funct. 2015;6:1386–1398.
- Otles S, Ozgoz S. Health effects of dietary fiber. Acta Sci Pol Technol Aliment. 2014;13:191–202.
- Naidu KA. Vitamin C in human health and disease is still a mystery? An overview. Nutr J. 2003;2:7.
- Tulipani S, Romandini S, Alvarez Suarez JM, et al. Folate content in different strawberry genotypes and folate status in healthy subjects after strawberry consumption. Biofactors. 2008;34:47–55.
- Elad Y, Williamson B, Tudzynski P, Delen N. Botrytis spp. and diseases they cause in agricultural systems–an introduction. In: Elad Y, Williamson B, Tudzynski P, et al., editors. Botrytis: biology, pathology and control. The Netherlands: Springer; 2007. p. 1–8.
- Braun P, Sutton J. Infection cycles and population dynamics of Botrytis cinerea in strawberry leaves. Can J Plant Pathol. 1988;10:133–141.
- Xu X, Harris DC, Berrie AM. Modeling infection of strawberry flowers by Botrytis cinerea using field data. Phytopathology. 2000;90:1367–1374.
- Xiao C, Chandler C, Price J, et al. Comparison of epidemics of Botrytis fruit rot and powdery mildew of strawberry in large plastic tunnel and field production systems. Plant Disease. 2001;85:901–909.
- Droby S, Lichter A. Post-harvest Botrytis infection: etiology, development and management. In: Elad Y, Williamson B, Tudzynski P, et al., editors. Botrytis: Biology, pathology and control. The Netherlands: Springer; 2007. p. 349–367.
- van Kan JA. Infection strategies of Botrytis cinerea. In: VIII International Symposium on Postharvest Physiology of Ornamental Plants; 2003. p. 77–90.
- Gourgues M, Brunet‐Simon A, Lebrun MH, et al. The tetraspanin BcPls1 is required for appressorium‐mediated penetration of Botrytis cinerea into host plant leaves. Mol Microbiol. 2004;51:619–629.
- Govrin EM, Levine A. The hypersensitive response facilitates plant infection by the necrotrophic pathogen Botrytis cinerea. Curr Biol. 2000;10:751–757.
- Bézier A, Lambert B, Baillieul F. Study of defense-related gene expression in grapevine leaves and berries infected with Botrytis cinerea. Eur J Plant Pathol. 2002;108:111–120.
- Puhl I, Treutter D. Ontogenetic variation of catechin biosynthesis as basis for infection and quiescence of Botrytis cinerea in developing strawberry fruits. J Plant Dis Prot. 2008;115:247–251.
- Maas JL. Compendium of strawberry diseases. St. Paul (MN): APS press; 1998.
- Agrios GN. Plant Pathology. 5th ed. Department of Plant Pathology. University of Florida; 2005.
- Magnin-Robert M, Quantinet D, Couderchet M, et al. Differential induction of grapevine resistance and defense reactions against Botrytis cinerea by bacterial mixtures in vineyards. BioControl. 2013;58:117–131.
- Spadaro D, Droby S. Development of biocontrol products for postharvest diseases of fruit: the importance of elucidating the mechanisms of action of yeast antagonists. Trends Food Sci Technol. 2016;47:39–49.
- Huang R, Li G, Zhang J, et al. Control of postharvest Botrytis fruit rot of strawberry by volatile organic compounds of Candida intermedia. Phytopathology. 2011;101:859–869.
- Zhang H, Wang L, Dong Y, et al. Postharvest biological control of gray mold decay of strawberry with Rhodotorula glutinis. Biol Control. 2007;40:287–292.
- Compant S, Duffy B, Nowak J, et al. Use of plant growth-promoting bacteria for biocontrol of plant diseases: principles, mechanisms of action, and future prospects. Appl Environ Microbiol. 2005;71:4951–4959.
- Lopes MR, Klein MN, Ferraz LP, et al. Saccharomyces cerevisiae: a novel and efficient biological control agent for Colletotrichum acutatum during pre-harvest. Microbiol Res. 2015;175:93–99.
- Agrawal T, Kotasthane AS. Chitinolytic assay of indigenous Trichoderma isolates collected from different geographical locations of Chhattisgarh in Central India. SpringerPlus. 2012;1:73.
- Dunne C, Crowley JJ, Moënne-Loccoz Y, et al. Biological control of Pythium ultimum by Stenotrophomonas maltophilia W81 is mediated by an extracellular proteolytic activity. Microbiology. 1997;143:3921–3931.
- Mangunwardoyo W, Aprilismulan A, Oetari A, Sjamsuridzal W. Screening cellulose activity of yeast isolated from soil, sediment and water river from Taman Nasional Gunung Halimun, West Java, Indonesia. Malays J Microbiol. 2011;7:e216.
- Vero S, Garmendia G, González MB, et al. Evaluation of yeasts obtained from Antarctic soil samples as biocontrol agents for the management of postharvest diseases of apple (Malus × domestica). FEMS Yeast Res. 2013;13:189–199.
- Giobbe S, Marceddu S, Scherm B, et al. The strange case of a biofilm-forming strain of Pichia fermentans, which controls Monilinia brown rot on apple but is pathogenic on peach fruit. FEMS Yeast Res. 2007;7:1389–1398.
- Kurtzman CP, Robnett CJ. Identification of clinically important ascomycetous yeasts based on nucleotide divergence in the 5' end of the large-subunit (26S) ribosomal DNA gene. J Clin Microbiol. 1997;35:1216–1223.
- Morgan W, Kamoun S. RXLR effectors of plant pathogenic oomycetes. Curr Opin Microbiol. 2007;10:332–338.
- Fang XL, Phillips D, Li H, et al. Severity of crown and root diseases of strawberry and associated fungal and oomycete pathogens in Western Australia. Australasian Plant Pathol. 2011;40:109–119.
- Golzar H, Phillips D, Mack S. Occurrence of strawberry root and crown rot in Western Australia. Austral Plant Disease Notes. 2007;2:145–147.
- Eikemo H, Klemsdal SS, Riisberg I, et al. Genetic variation between Phytophthora cactorum isolates differing in their ability to cause crown rot in strawberry. Mycol Res. 2004;108:317–324.
- Abad ZG, Abad JA, Coffey MD, et al. Phytophthora bisheria sp. nov., a new species identified in isolates from the Rosaceous raspberry, rose and strawberry in three continents. Mycologia. 2008;100:99–110.
- Parafati L, Vitale A, Restuccia C, et al. Biocontrol ability and action mechanism of food-isolated yeast strains against Botrytis cinerea causing post-harvest bunch rot of table grape. Food Microbiol. 2015;47:85–92.
- Morath SU, Hung R, Bennett JW. Fungal volatile organic compounds: a review with emphasis on their biotechnological potential. Fungal Biol Rev. 2012;26:73–83.
- Chen H, Xiao X, Wang J, et al. Antagonistic effects of volatiles generated by Bacillus subtilis on spore germination and hyphal growth of the plant pathogen, Botrytis cinerea. Biotechnol Lett. 2008;30:919–923.
- Li Q, Ning P, Zheng L, et al. Effects of volatile substances of Streptomyces globisporus JK-1 on control of Botrytis cinerea on tomato fruit. Biol Control. 2012;61:113–120.
- Ortíz-Castro R, Contreras-Cornejo HA, Macías-Rodríguez L, et al. The role of microbial signals in plant growth and development. Plant Signal Behav. 2009;4:701–712.
- Su HJ, Thseng FM, Chen JS, et al. Production of volatile substances by rhizomorphs of Marasmius crinisequi and its significance in nature. Fungal Divers. 2011;49:199–202.
- Etebarian H ZE, Sahebani N. Biological control of apple gray mold by mixtures of Bacillus Subtilis and yeast isolates. Afr J Food Sci. 2014;8:155–163.
- Makower M, Bevan E. The inheritance of a killer character in yeast (Saccharomyces cerevisiae). In: Geerts SJ, editor. Genetics today: Proceedings of the XI international congress of genetics; 1963. p. 235–244.
- Santos A, Marquina D. Killer toxin of Pichia membranifaciens and its possible use as a biocontrol agent against grey mould disease of grapevine. Microbiology (Reading, Engl). 2004;150:2527–2534.
- Marquina D, Santos A, Peinado JM. Biology of killer yeasts. Int Microbiol. 2002;5:65–71.
- Chen PH, Chou JY. Screening and identification of yeasts antagonistic to pathogenic fungi show a narrow optimal pH range for antagonistic activity. Pol J Microbiol. 2017;66:101–106.
- Backman P. Fungicide formulation: relationship to biological activity. Annu Rev Phytopathol. 1978;16:211–237.
- Kim J, Lee SH, Kim CS, et al. Biological control of strawberry gray mold caused by Botrytis cinerea using Bacillus licheniformis N1 formulation. J Microbiol Biotechnol. 2007;17:438–444.
- Frey-Klett P, Burlinson P, Deveau A, et al. Bacterial-fungal interactions: hyphens between agricultural, clinical, environmental, and food microbiologists. Microbiol Mol Biol Rev. 2011;75:583–609.
- Seneviratne G, Zavahir J, Bandara W, et al. Fungal-bacterial biofilms: their development for novel biotechnological applications. World J Microbiol Biotechnol. 2008;24:739.
- Brown GE. Biology and control of Geotrichum candidum, the cause of citrus sour rot. Proc Flo State Hort Soc. 1984;92:186–189.
- Liti G, Carter DM, Moses AM, et al. Population genomics of domestic and wild yeasts. Nature. 2009;458:337–341.
- Chou JY, Chen HW, Lin CC, et al. Yeast diversity associated with the biting midge Forcipomyia taiwana in Taiwan. Nova Hedw. 2015;101:519–527.