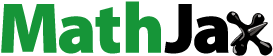
Abstract
In this study, the antioxidant, anti-xanthine oxidase, anti-melanogenic and anti-wrinkle effects of methanol (ME) and hot water (HE) extracts from the fruiting bodies of Phellinus vaninii were investigated. The 1,1-diphenyl-2-picryl-hydrazyl free radical scavenging activity of 2.0 mg/mL HE (95.38%) was comparable to that of butylated hydroxytoluene (96.97%), the reference standard. The hydroxyl radical scavenging activities of ME (98.19%) and HE (97.55%) were higher than that of butylated hydroxytoluene (92.66%) at 2.0 mg/mL. Neither ME nor HE was cytotoxic to murine melanoma B16-F10 cells at 25–750 µg/mL. Although the xanthine oxidase (XO) inhibitory effects of ME and HE were significantly lower than that of allopurinol, the values were higher than 84 percent. The in vitro tyrosinase inhibitory activities of ME and HE were comparable to kojic acid at 2.0 mg/mL. The cellular tyrosinase and melanin synthetic activities of ME and HE on B16-F10 melanoma cells at 500 µg/mL were higher than arbutin, indicating that the inhibitory effects of arbutin on the tyrosinase and melanin synthesis were higher than those of ME and HE. The collagenase inhibitory activity of HE was comparable to EGCG at 2.0 mg/mL, however, the elastase inhibitory activity of ME and HE was lower than EGCG at the concentration tested. The study results demonstrated that the fruiting bodies of Ph. vaninii possessed good antioxidant, anti-xanthine oxidase, cell-free anti-tyrosinase, cellular anti-tyrosinase, anti-collagenase, and moderate anti-elastase activities, which might be used for the development of novel anti-gout, skin-whitening, and skin anti-wrinkle agents.
1. Introduction
Free radicals and reactive oxygen species (ROS) are generally unstable and highly-reactive chemical species. They are mainly derived from enzymatic and non-enzymatic metabolic pathways in cells of the human body through the mitochondrial electron transport system of respiration, phagocytosis, prostaglandin synthesis, and the endoplasmic reticulum system [Citation1]. However, free radicals and ROS are also generated from exposure to exogenous sources, including heavy metals, tobacco smoke, chemicals, and industrial pollutants [Citation2]. They have been reported to cause various ailments, such as aging, cardiovascular diseases, respiratory disorders, diabetes mellitus, rheumatism, and liver diseases [Citation3].
Xanthine oxidase (XO) catalyzes the oxidation of hypoxanthine to xanthine and further converts xanthine to uric acid. Gout is characterized by an elevated concentration of uric acid (hyperuricemia) in the blood that leads to the deposition of uric acid crystals in the joints causing painful inflammation and 5–30% of the world population suffers from gout, which is considered to be an important risk factor for human health [Citation4,Citation5]. For the treatment of gout, XO inhibitors which lower the plasma uric acid concentration are generally employed [Citation6]. Although allopurinol, an XO inhibitor, has been used for the treatment of gout, a new XO inhibitor, febuxostat, was recently developed. However, the drug has many side effects, including hepatitis, nephropathy, and allergic reactions [Citation7]. Thus, the search for new XO inhibitors with good therapeutic activity and lower side effects has continued.
Tyrosinase is a rate-limiting copper-containing oxidative enzyme commonly present in plants, microorganisms and animals. Tyrosinase is involved in the melanin synthetic pathways by the hydroxylation of L-tyrosine to 3,4-dihydroxyphenylalanine (DOPA) and the conversion of DOPA to dopaquinone [Citation8]. Melanin is produced by the melanocytes of the melanosomes and plays an important role in defending the epidermis from the harmful effects of ultraviolet (UV) radiation from sunlight. Skin hyperpigmentation resulting from increased melanin synthetic enzyme activity by excessive exposure to UV radiation may cause dermatological disorders, including melasma, vitiligo, and lentigo [Citation9,Citation10]. Recently, tyrosinase inhibitors have become important for preventing pigmentation problems and other melanin-related medical disorders. Since many women prefer to lighten their dark skin patches, the medical and cosmetic industries have focused on tyrosinase inhibitors to treat skin hyperpigmentation [Citation11]. To deal with these problems, organic compounds originating from microorganisms and plants, such as kojic acid, arbutin, azelaic acid, gentisic acid, and others have been developed as skin-whitening agents [Citation12]. The efficacy of whitening compounds is determined by tyrosinase inhibition in cell-free systems or by the inhibition of cellular tyrosinase and new sources of tyrosine inhibitors from plants and microorganisms are continually being screened [Citation13].
Skin aging is a biologically inevitable process of living organisms and instigated by both intrinsic and extrinsic factors. Intrinsic skin aging, also called age-dependent aging or chronologic aging, is caused by internal physiological factors of the body, while extrinsic aging is caused by many external factors, including exposure of the skin to UV radiation, cigarette smoking, air pollution, etc. These factors can cause wrinkles, pigmentation, and changes in skin thickness [Citation14]. Skin is the soft outer tissue consists of epidermal, dermal and subcutaneous tissue. The outermost part of the skin is an extracellular matrix (ECM) and consists of collagen and elastin [Citation15]. Collagen provides elasticity, strength, and flexibility of the skin. It also maintains the structural framework of the skin and plays a pivotal role maintaining normal cellular functions in the morphogenesis of skin [Citation16]. Elastin is a key protein present in the connective tissue of the ECM and provides elasticity to the skin. Collagenase, a zinc-dependent endopeptidase, is capable of degrading ECM components, specifically, type 1 collagen. Elastase, a protease enzyme, breaks down elastin, and collagen, and determines the mechanical and structural properties of the connective tissue of the ECM [Citation17]. Therefore, it is desirable to develop pharmacological inhibitors to prevent skin aging, such as skin sagging and wrinkling.
Mushrooms have been used as good food sources and traditional folk medicine for thousands of years in many different countries. Their fruiting bodies contain biologically-active metabolites with high medicinal value, including β-glucans, polysaccharides, polyphenols, flavonoids, terpenoids, and other organic compounds, which provide immune stimulating, antitumor, anti-hyperglycemic, anti-hypercholesterolemic, hepatoprotective, and inflammation inhibitory activities [Citation18–20].
Phellinus vaninii, commonly known as ‘sanghuang’, belongs to Basidiomycota, Aphyllophorales, Hymenochaetacae and is distributed in East Asia and Russia [Citation21]. Several mushroom species belonging to the genus Phellinus, including Ph. baumi, Ph. gilvus, Ph. linteus, Ph. merrillii, and Ph. pini, have been studied for their potential medicinal antioxidant, anti-diabetic, anti-hyperlipidemic, anti-microbial, and antitumor activities [Citation22,Citation23].
Although Ph. vaninii has become available in Korea, there are only a few reports of its medicinal effects [Citation24]. Therefore, the purpose of this study was to evaluate the antioxidant, anti-xanthine oxidase, anti-melanogenic and anti-wrinkle effects of the methanol and hot water extracts of Ph. vaninii fruiting bodies.
2. Materials and methods
2.1. Chemicals and reagents
Butylated hydroxytoluene (BHT), 1,1-diphenyl-2-picryl-hydrazy (DPPH), dimethyl sulfoxide, (DMSO) xanthine oxidase, tyrosinase, L-3,4-dihydroxyphenylalanine (DOPA), xanthine oxidase, allopurinol, kojic acid, arbutin, collagenase, and porcine pancreatic elastase were purchased from Sigma-Aldrich Co. (St. Louis, MO, USA), and all other chemicals and solvents used for the study experiments were of analytical grade.
2.2. Mushroom extract
The fruiting bodies of Ph. vaninii were obtained from Mushroom Research Institute of Gyeonggi Agricultural Technology Institute in Korea. The fruiting bodies were air-dried (45 °C for 48 h) and finely pulverized. To prepare the methanol extract (ME), 20 g of the powder in 400 mL of 80% methanol was kept in an orbital shaker (125 rpm) for 24 h at 25 °C. The solution was filtered through filter paper. To obtain the hot water extract (HE), the same amount of the powder was boiled for 3 h with 400 mL of deionized distilled water and filtered through filter paper. Then, the remaining residue was extracted with 400 mL of 80% methanol or hot water twice, as described above, respectively. Then, the combined extracts were evaporated to dryness at 40 °C, and the remaining water was removed with a freeze-drier.
2.3. Total phenolic and flavonoids content
The total phenolic content of ME and HE was determined using a slight modification of the Folin-Ciocalteu assay [Citation25]. A 1 mL aliquot of ME and HE was added to 1 mL of 10% Folin-Ciocalteu solution. The solution was vortexed and left for 3 min at 25 °C. Then, 3 mL of 2% sodium carbonate (Na2CO3) solution was added and kept for 2 h at 25 °C. The absorbance was measured with a spectrometer at 760 nm. The total phenolic content was expressed as mg gallic acid equivalents (GAE).
The total flavonoid content was measured by a slightly modified aluminum chloride (AlCl3) colorimetric assay [Citation26]. An aliquot (1 mL) of ME and HE was dissolved in 1 mL deionized water. This solution (1 mL) was added to 3.0 mL of 95% alcohol, 0.2 mL of 10% aluminum chloride, 0.2 mL of potassium acetate (1 M), and 5.6 mL of deionized water. Then, the reaction mixture was incubated at 25 °C for 40 min, and the absorbance was measured with a spectrometer at 415 nm. The total flavonoid content was expressed as mg quercetin equivalents (QE).
2.4. Cell viability assay
2.4.1. Cell culture
The murine melanoma B16-F10 cell line was obtained from the Korean Cell Line Bank of the Korean Cell Line Research Foundation, Seoul, Korea. The cells were cultured in Dulbecco’s Modified Eagle’s Media (DMEM) supplemented with 10% heat-inactivated fetal bovine serum (FBS), and 1% penicillin-streptomycin at 37 °C in a humidified incubator with 5% atmospheric CO2.
2.4.2. Cell viability
The effect of ME and HE on the cell viability of B16-F10 cells was estimated by MTT assay [Citation27]. The cells (5 × 104) were cultured in a 24-well plate and incubated for 24 h at 37 °C. Then, the cells were treated with varying concentrations of ME and HE (25–750 µg/mL) and incubated for 48 h. Then, each well was supplemented with 200 µL of MTT solution (0.5 mg/mL) and incubated for 4 h in the dark. The resulting colored formazan crystals were solubilized in 200 µL of DMSO and the absorbance was measured with a microplate reader at 570 nm.
2.5. Antioxidant assay
2.5.1. DPPH scavenging activity
The radical scavenging effect of ME and HE from Ph. vaninii fruiting bodies was determined using the DPPH assay [Citation28] with minor modifications. Briefly, 1 mL of different concentrations of ME and HE (0.125–2.0 mg/mL) was mixed with I mL of DPPH (0.1 mM) in methanol. The mixture was shaken and kept for 30 min at 25 °C. The absorbance was measured with a spectrophotometer at 517 nm. The DPPH radical scavenging effect was calculated using the following formula:
where Absc represents the absorbance of the vehicle control and Abss represents the absorbance of the sample. BHT was used as a reference standard.
2.5.2. Inhibition of lipid peroxidation
The lipid peroxidation inhibitory activity of ME and HE from Ph. vaninii fruiting bodies was assessed using a previously described method [Citation29] with minor modification. Briefly, 250 µL of egg yolk homogenate (10% in distilled water), 50 µL of each ME and HE, and 200 µL of distilled water were added to a test tube. Twenty-five milliliters of FeSO4 (0.07 M) was added and incubated for 30 min at 25 °C. Then, 750 µL of 20% acetic acid (pH 3.5) and 750 µL of 0.8% thiobarbituric acid (TB) in 1.1% sodium dodecyl sulfate (SDS) with 25 µL of 20% trichloroacetic acid (TCA) were added and the mixture was vortexed and incubated in a water bath for 60 min at 100 °C. After cooling to 25 °C, 3.0 mL of 1-butanol was added to each tube and they were centrifuged for 10 min at 3000 rpm. The absorbance of the solution was measured with a spectrophotometer at 532 nm. The inhibitory effect of lipid peroxidation was calculated using the following formula:
Lipid peroxidation inhibition (%) = [(Absc – Abss)/Absc] × 100,
where Absc represents the absorbance of the vehicle control and Abss represents the absorbance of the test sample. BHT was used as a reference standard.
2.5.3. Hydroxyl radical scavenging activity
The hydroxyl radical scavenging effect of ME and HE from Ph. vaninii fruiting bodies was measured using a published method [Citation30] with minor modifications. In brief, 0.5 mL of different concentration of ME and HE (0.125–2.0 mg/mL) were incubated in a solution containing 100 µL of 2.8 mM 2-deoxyribose in phosphate buffer (10 mM, pH 7.4), 200 µL of FeCl3 (200 microM)-EDTA (1.04 microM) (1:1 v/v), 100 µL of H2O2 (1.0 mM), and 100 µL of ascorbic acid (1.0 mM). The amount of deoxyribose degradation was measured after the addition of 1.0 mL of 1% TBA, followed by incubation at 100 °C for 20 min. The absorbance was measured on a spectrophotometer at 532 nm. The inhibitory effect of hydroxyl radical scavenging was calculated using the following formula:
Hydroxyl radical scavenging (%) =[(Absc – Abss)/Absc] × 100
where Absc represents the absorbance of the vehicle control and Abss represents the absorbance of the test sample. BHT was used as a reference standard.
2.6. Xanthine oxidase inhibition
The xanthine oxidase (XO) inhibitory activity of ME and HE from the fruiting bodies of Ph. vaninii was measured using xanthine as the substrate by a published method with minor modifications [Citation31]. A 1 mL aliquot of various concentrations of ME and HE (0.5–8.0 mg/mL) was added to 2.9 mL of phosphate buffer (pH 7.5) and 0.1 mL of xanthine oxidase enzyme solution (0.1 units/mL in phosphate buffer pH 7.5) and pre-incubated at 25 °C for 15 min. Then, the reaction was initiated by the addition of 2 mL of the substrate solution (150 µM xanthine in the buffer). The mixture was incubated for 30 min at 25°C and the reaction was terminated by the addition of 1 mL 1 N HCl. The absorbance was measured with a spectrophotometer at 290 nm. Allopurinol was used as a reference standard. Xanthine oxidase inhibition was calculated using the following formula:
where A is the enzyme activity without the extract, B is the control of A without the extract and enzyme; C is the activity of extract with XO, D is the activity of the extract without XO.
2.7. Anti-melanogenic effects
2.7.1. In vitro inhibition of tyrosinase
The tyrosinase inhibitory activity of the mushroom extracts was determined using a published method [Citation32] with slight modification. Briefly, the reaction mixtures were prepared by the addition of 40 µL of tyrosinase (31 units/mL) to 40 µL of varying concentrations of ME and HE (0.125–2.0 mg/mL), 40 µL of 1.5 mM L-tyrosine, and 80 µL of 0.1 M phosphate buffer (pH 6.8). Then, the mixture was incubated for 10 min at 37 °C. The absorbance was measured with a microplate reader at 475 nm. The tyrosinase inhibition was calculated using the following formula:
Inhibition of tyrosinase (%) = [(Absc – Abss)/Absc] × 100,
where Absc represents the absorbance of the vehicle control and Abss represents the absorbance of the test sample. BHT was used as a reference standard.
2.7.2. In vitro inhibition of DOPA auto-oxidation
The L-DOPA auto-oxidation inhibitory activity of the mushroom extracts was determined using a slightly modified method [Citation33]. Briefly, the reaction mixtures were prepared by the addition of 40 µL tyrosinase (31 units/mL) to 40 µL of varying concentrations of ME and HE (0.125–2.0 mg/mL), and pre-incubated for 10 min at 37 °C. Then, the reaction was initiated by the addition of 40 µL of 2.5 mM L-DOPA solution (in phosphate buffer) and 80 µL of phosphate buffer (0.1 M, pH 6.8) and incubated for 10 min at 37 °C in the dark. The absorbance was measured with a microplate reader at 475 nm. The percentage of L-DOPA auto-oxidation inhibition was calculated using the following formula:
Inhibition of DOPA auto-oxidation (%) = [(Absc – Abss)/Absc] × 100,
where Absc represents the absorbance of the vehicle control and Abss represents the absorbance of the test sample. Kojic acid was used as a reference standard.
2.7.3. Cellular tyrosinase activity
The cellular tyrosinase activity in B16-F10 cells was measured using a slight modification of a published method [Citation34]. The cells (5 × 105 cells/well) were cultured in 96-well plates. After treatment with various concentrations of samples (25–500 µg/mL) for 24 h, the melanoma cells were washed with PBS, and lysed with 0.1 M phosphate buffer (pH 6.8) containing 1% Triton X-100. The cells were kept on ice for 10 min and the lysates were centrifuged at 10,000 ×g for 10 min. Then, the protein content in the supernatant was determined by the Braford method, with bovine serum albumin (BSA) as a standard. To measure the tyrosinase activity, 100 µL of cell lysate solution and 100 µL of 2.5 mM L-DOPA (in the same phosphate buffer) and was added to each well of a 96-well plate. After incubation at 37 °C for 1 h, the absorbance was measured at 475 nm using a microplate reader. The cellular tyrosinase activity was calculated using the following formula:
Cellular tyrosinase activity (%) = (Abss/Absc) × 100,
where Abss represents the absorbance of the sample and Absc represents the absorbance of the control. Arbutin was used as a reference standard.
2.7.4. Cellular melanin synthesis
Measurement of melanin content in B16-F10 melanoma cells treated with varying concentrations of the mushroom extracts was performed using the method published by Hosoi et al. [Citation35]. The cells (4 × 104 cells/well) were cultured in a 96-well plate in DMEM. After 24 h of incubation, the cells were treated with ME and HE (25–500 µg/mL) for 72 h containing 0.4 µM melanocyte-stimulating hormone (MSH). The cells were harvested by the addition of 300 µL of Triton ×100 (1%, PBS), centrifuged for 5 min at 3000 rpm, and the supernatant removed. Then, 100 µL of 1 N NaOH and 200 µL of DMSO (10%) were added to the cell pellets and kept for 1 h at 60 °C. The absorbance of the solution was measured using a microplate reader at 405 nm. The effect of ME and HE on melanin synthesis was determined. Arbutin was used as a reference standard.
2.8. Anti-wrinkle effects
2.8.1. Elastase inhibition
The elastase inhibitory effect of ME and HE was assessed by previously reported methods [Citation36]. Porcine pancreatic elastase (PE–EC. 3.4.21.36) was dissolved in sterile water to make a 3.33 mg/mL stock solution. The substrate, N-succinyl-Ala-Ala-Ala-p-nitroanilide (AAAPVN), was dissolved in 0.2 M Tris-HCl buffer (pH 8.0). The reaction was carried out in a 96-well microplate. Each well contained 120 µL of 0.2 M Tris-HCl buffer, 50 µL of mushroom extract (0.125–2.0 mg/mL) in Tris-HCl buffer, 30 µL of PE, and 50 µL of 1.6 mM AAAPVN. The test extract was pre-incubated at 25 °C for 15 min before addition of the substrate. The absorbance was measured with a microplate reader at 402 nm. The percent inhibition of esterase was calculated as follows:
Esterase inhibition (%) = [(Absc – Abss)/Absc] × 100,
where Absc represents the absorbance of the vehicle control and Abss represents the absorbance of the test sample. EGCG was used as a reference standard, while distilled water was used as a negative control.
2.8.2. Collagenase inhibition
The collagenase inhibitory effect of ME and HE from Ph. vaninii fruiting bodies was investigated using previously reported methods [Citation37] with some modifications. Collagenase from Clostridium histolyticum (ChC–EC.3.4.23.3) was solubilized in 50 mM of 1.44 U/mL Tricine buffer (pH 7.8). The substrate, 4-phenylazobenzyloxycarbonyl-Pro-Leu-Gly-Pro-D-Arg trifluoroacetate salt (PZ-peptide), was dissolved in 2.5 mM Tricine buffer. Twenty-five microliters of mushrooms extracts (0.125 to 2.0 mg/mL) were incubated with 20 µL of enzyme in 155 µL of buffer for 15 min at 37 °C before the addition of 50 µL of PZ-peptide to initiate the reaction for 60 min at 37 °C. The reaction was stopped by the addition of 1 mL of citric acid (3%). Then, 5 mL of ethyl acetate was added and the solution was vigorously shaken and allowed to stand for 10 min. The absorbance of the supernatant was measured at 320 nm using a microplate reader. EGCG was used as a reference standard. The inhibition of collagenase was calculated using the following formula:
Collagenase inhibition (%) = [(Absc – Abss)/Absc] × 100,
where Absc represents the absorbance of the vehicle control and Abss represents the absorbance of the test sample. EGCG was used as a reference standard.
2.9. Statistical analysis
All data were expressed as means ± standard deviations (SD) and SPSS V.13 (SPSS Inc., Chicago, IL, USA) was used for statistical analysis. One-way analysis of variance followed by Duncan’s multiple range tests were used to compare means among groups. Differences between means at the 5% (p < .05) level were considered statistically significant.
3. Results and discussion
3.1. Total phenolics and flavonoids contents
The total phenolic and flavonoid contents of ME and HE are presented in . The results revealed that the total phenolic and flavonoid contents of ME were 31.67 mg GAE/g and 6.83 mg QE/g, respectively, whereas those for HE were 16.33 mg GAE/g and 4.50 mg QE/g, respectively. Vamanu and Nita [Citation38] reported that the total phenolic contents of methanol and hot water extracts from the fruiting bodies of Boletus edulis were 18.96 mg GAE/g and were 17.22 mg GAE/g, respectively, while flavonoid content of methanol extract from Boletus aestivalis and Leccinum carpini fruiting bodies was 1.53 mg CE/g and 1.48 mg CE/g, respectively [Citation39]. Our results suggest that the total phenolic and flavonoid content of the mushroom extracts was higher than those of the mushrooms mentioned above, and may have contributed to the improvement of the antioxidant related anti-xanthine oxidase, anti-melanogenic and skin anti-wrinkle activities.
Table 1. Total polyphenol and flavonoid contents of methanol and hot water extract from fruiting bodies of Phellinus vaninii.
3.2. Cell viability
To determine whether the extracts from Ph. vaninii fruiting bodies exhibited a cytotoxic effect on B16-F10 murine melanoma cells, the cells were treated with varying concentrations of ME and HE for 72 h, and the cell viability was determined by MTT assay. The cell viability of the ME- and HE-treated cells ranged from 99.74 to 85.33% and 100.67 to 88.33% at 50 to 750 µg/mL, respectively (). The cell viabilities decreased with increasing concentrations of the mushroom extracts. Since the viabilities of cells treated with 500 µg/mL ME and HE were over 90.33% and 91%, respectively, and the IC50 values of ME and HE were 4,410 µg/mL and 5,385 µg/mL, respectively, the ME and HE were not cytotoxic to B16-F10 melanoma cells at the concentrations tested. Therefore, the cellular tyrosinase and melanin synthesis activities of B16-F10 melanoma cells treated with different concentrations of the mushroom extracts were performed.
3.3. Antioxidant activity
3.3.1. DPPH radical scavenging effect
DPPH radical scavenging effects of ME and HE from the fruiting bodies of Ph. vaninii increased with increasing extract concentrations. The radical scavenging activities of ME and HE at 0.125–2.0 mg/mL ranged from 75.83% to 95.17%, and 73.33% to 94.33%, respectively, and BHT, the positive control, demonstrated good scavenging activity (96.19–96.97%) (). Although the DPPH scavenging activity of ME and HE were lower than BHT at concentrations ranging from 0.125 to 0.5 mg/mL, the percentage of inhibition observed in ME and HE at 1.0–2.0 mg/mL was statistically insignificant compared to BHT. These results suggest that the methanol and hot water extracts possessed good radical scavenging activities at the higher concentrations tested.
Figure 2. Antioxidant activities of methanol and hot water extracts from fruiting bodies of Phellinus vaninii. (A) DPPH radical scavenging activity; (B) Lipid peroxidation inhibitory activity; (C) Hydroxyl radical (OH−) scavenging activity. Values are means ± SD (n = 3). BHT, butylated hydroxytoluene; DPPH, 1,1-Diphenyl-2-picryl-hydrazy. ***p < .001, **p < .01, *p < .05 vs. BHT group; ##p < .01, #p < .05 vs. Methanol extract group.
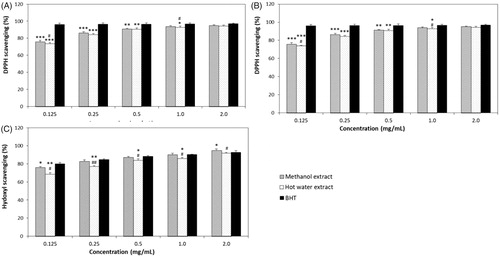
DPPH scavenging activities of the methanol extract from Pleurotus pulmonarius fruiting bodies have been reported to range from 25.67% to 92.73% at 0.125 to 2.0 mg/mL [Citation40]. Mau et al. [Citation41] found that the radical scavenging effects of the methanol extracts from Tricholoma giganteum, Grifola frondosa, and Hericium erinaceus mushrooms ranged from 63.2% to 67.8% at 6.4 mg/mL concentration. Therefore, our experimental results suggest that the DPPH scavenging activity of ME and HE (0.125–2.0 mg/mL) from Ph. vaninii fruiting bodies can provide an adequate defense from free radical and ROS injuries.
3.3.2. Lipid peroxidation inhibition
The lipid peroxidation inhibitory activity of ME and HE from the fruiting bodies of P. vaninii ranged from 44.99 to 66.08% and from 41.26 to 64.00% at 0.125-2.0 mg/mL, respectively (), indicating that increasing concentrations of mushroom extracts increased lipid peroxidation inhibition. However, the lipid peroxidation inhibitory effect of BHT was 90.30% at 2.0 mg/mL, which was significantly higher (p < .001) than those of ME and HE. The IC50 values of ME against lipid peroxidation from wild and cultivated mushrooms including Pleurotus ostreatus, Termitomyces robustus, Pleurotus sajor-caju, and Auricularia auricula were 0.15, 0.43, 0.75, and 1.51 mg/mL, respectively [Citation42]. The inhibitory effects of hot water extracts on lipid peroxidation of 14 different edible and medicinal mushroom species collected from Malaysia ranged from 33.33% to 57.18% at the concentration of 10 mg/mL [Citation43]. In this study, the lipid peroxidation inhibitory activity of ME and HE from Ph. vaninii ranged from 65.87% to 78% at 2.0 mg/mL and their IC50 values were 0.19 mg/mL and 0.17 mg/mL, respectively. These results suggest that ME and HE from Ph. vaninii fruiting bodies possessed good inhibitory activity toward lipid peroxidation compared to the mushrooms described above. Therefore, P. vaninii fruiting bodies could be a valuable antioxidant source.
3.3.3. Hydroxyl radical scavenging effect
The hydroxyl radical scavenging effects of ME and HE from Ph. vaninii ranged from 75.60 to 98.19% and 65.21 to 97.55% at 0.125 to 2.0 mg/mL concentrations, respectively, whereas those of BHT ranged from 79.89 to 92.66% (). However, the IC50 values of ME and HE from Ph. vaninii were 0.115 mg/mL and 0.119, respectively, whereas the IC50 value of BHT was 0.111 mg/mL, indicating that the hydroxyl radical scavenging effects of ME and HE were a little lower than that of BHT. Thus, ME and HE from Ph. vaninii possessed relatively good and comparable hydroxyl radical scavenging activities at the concentrations tested. The higher hydroxyl radical scavenging activities found in ME and HE in this experiment indicated that the extracts effectively prevented the decomposition of 2-deoxyribose by removing hydroxyl radicals in the test solution. The methanol extract from the fruiting bodies of Pleurotus pulmonarius has been reported to have a 95.13% hydroxyl radical scavenging effect at 2.0 mg/mL [Citation40], which was lower than those of ME (98.18%) and HE (97.55%) from Ph. vaninii at the concentration tested. The hydroxyl scavenging effect of the methanol extract from fruiting bodies of Hypsizigus ulmarius mushrooms has been reported to be 76.67% at 1.0 mg/mL [Citation44], whereas those of ME and HE were 83.00% and 77.17% at 0.25 mg/mL. Taken together, our results suggest that the hydroxyl radical scavenging effect of ME and HE from Ph. vaninii were better than those of the mushroom extracts mentioned above. Therefore, ME and HE from Ph. vaninii are potent hydroxyl radical scavengers that may be used to prevent ROS-related problems.
3.4. Xanthine oxidase inhibition
The xanthine oxidase inhibitory activities of ME and HE from Ph. vaninii increased with increasing concentrations. The xanthine oxidase inhibition of ME and HE at 0.5 to 8.0 mg/mL ranged from 61.15% to 88.99%, and 34.13% to 84.56%, respectively. However, allopurinol, the reference standard, demonstrated excellent XO inhibitory activities of 89.73 to 97.23% at the same concentrations tested (). Although ME and HE exhibited moderate XO inhibitory activities at lower concentrations, the mushroom extracts significantly inhibited XO at higher concentrations. The XO inhibition by the methanol and hot water extracts from Ph. gilvus fruiting bodies have been reported to range from 28.60% to 86.20% and from 36.53% to 97.07% at 0.5 to 8.0 mg/mL, respectively [Citation45], indicating that the inhibitory effect of ME and HE toward XO were comparable with those of Ph. gilvus.
Figure 3. Xanthine oxidase inhibitory activity of methanol and hot water extracts from fruiting body of Phellinus vaninii. Methanol extract was extracted with 80% methanol; Hot water extract was extracted with hot water. Values are means ± standard deviation (SD, n = 3). ***p < .001, **p < .01, *p < .001 vs. allopurinol group; ###p < .001, ##p < .01, #p < .05 vs. Methanol extract group.
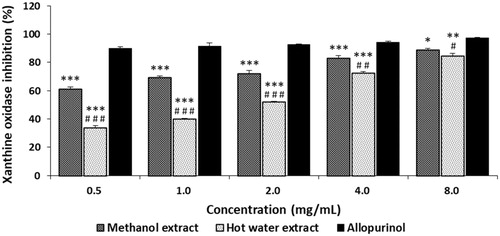
Flavonoids, a class of polyphenolic compounds, are the most common secondary metabolites of plants and fungi, and have been reported to possess xanthine oxidase inhibitory activity [Citation46]. Hence, higher phenolic and flavonoid concentrations detected in the ME and HE may have contributed to the inhibition of xanthine oxidase.
3.5. Skin-whitening effect
3.5.1. In vitro tyrosinase inhibition
The tyrosinase inhibitory activities of ME and HE from the fruiting bodies of Ph. vaninii ranged from 55.83% to 96.16% and from 30.29% to 92.74% at 0.125–2.0 mg/mL, respectively (), and increased with increasing concentrations. However, kojic acid, the reference standard, demonstrated excellent tyrosinase inhibitory activities between 98.74 and 99.61% at 0.125–2.0 mg/mL. The results suggest that the ME exhibited a good effect, while HE showed a moderate effect, at the concentrations tested. Alam et al. [Citation47] reported that the tyrosinase inhibition by methanol and hot water extracts from the fruiting bodies of P. salmoneostramineus ranged from 20.90% to 63.29% and from 15.25% to 49.25% at 0.125–1.0 mg/mL, respectively, indicating that the inhibitory activities of Ph. vaninii on tyrosinase were higher than those of P. salmoneostramineus fruiting bodies.
Figure 4. Tyrosinase and DOPA auto-oxidation inhibitory activities of methanol and hot water extracts from fruiting bodies of Phellinus vaninii. (A) Tyrosinase inhibitory activity; (B) DOPA auto-oxidation inhibitory activity. Values are means ± standard deviation (SD, n = 4). ***p < .001, *p < .05 vs. Kojic acid group; ###p < .001, #p < .05 vs. Methanol extract group.
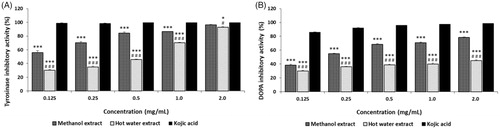
Phenolic compounds are aromatic acid compounds containing phenolic rings and carboxylic acid. Flavonoids are a group of polyphenolic compounds. They are found in many plant and mushroom species. Polyphenols are regarded as substrates for tyrosinase and are the largest group of tyrosinase inhibitors. Several polyphenols have been reported to inhibit tyrosinase by binding to the active site of the tyrosinase enzyme, resulting in irreversible inactivation of the enzyme by a substrate-like interaction [Citation48]. Some flavonoids have also demonstrated an inhibitory effect on tyrosinase by chelation of the copper ions in tyrosinase [Citation49].
Therefore, the inhibitory effect of ME and HE toward tyrosinase might be due to the polyphenolic compounds and flavonoids present in the mushroom extracts. The antioxidant effect of ME and HE may also contribute to the inhibition of tyrosinase activity.
3.5.2. Inhibition of DOPA auto-oxidation
L-DOPA is one of the precursors of melanin during melanogenesis. L-DOPA is synthesized from L-tyrosine by tyrosinase hydroxylase. Tyrosinase can also convert L-DOPA to dopaquinone, eventually leading to melanin. The in vitro, L-DOPA auto-oxidation inhibitory activities of P. vaninii are summarized in . The inhibition of L-DOPA auto-oxidation by ME and HE ranged from 38.25% to 78.46% and from 29.68% to 44.77% at 0.125 to 2.0 mg/mL, respectively, whereas kojic acid demonstrated excellent L-DOPA inhibitory activities of 86.14 to 98.72% at the concentration tested. The results showed that ME and HE moderately inhibited L-DOPA auto-oxidation. However, ME and HE exhibited less inhibitory activity on L-DOPA auto-oxidation when L-DOPA was used as a substrate than when L-tyrosine was used as a substrate of tyrosinase. Since tyrosinase uses two different binding sites for tyrosine hydroxylase and L-DOPA oxidase, our experimental results suggest that selective effectiveness of tyrosinase on the two different substrates during melanogenesis.
3.5.3. Cellular tyrosinase activity
Intracellular tyrosinase activity was determined after treating the cells with varying concentrations of ME and HE from the fruiting bodies of Ph. vaninii. The effect of ME and HE on the tyrosinase activity of B10-F10 melanoma cells ranged from 96.94% to 61.14% and from 98.12% to 84.16% at 25–500 ug/mL, respectively, whereas arbutin showed tyrosinase activities of 98.52 to 58.12% at the concentration tested (), indicating that the tyrosinase inhibition of arbutin was higher than those of ME and HE. The results also showed that the tyrosinase activity of ME was higher than that of HE and that increasing concentrations of ME and HE resulted in decreased intracellular tyrosinase activity. Although in vitro tyrosinase inhibitory effects of ME and HE at 500 ug/mL were 84.39% and 45.64%, respectively, cellular tyrosinase inhibition in B16-F10 melanoma cells were 38.86% and 15.24%, respectively at the same concentration tested, indicating that the mushroom extract factors responsible for tyrosinase inhibition were not able to penetrate the cells effectively. Huang et al. [Citation50] reported that cellular tyrosinase activity of methanol extract from fruiting bodies of Agaricus brasiliensis were 95.75%–67.12% at 100–500 ug/mL in B16-F10 melanoma cells, suggesting that cellular tyrosinase activity of Ph. vaninii were little higher than that of A. brasiliensis at the same concentration tested.
Figure 5. Cellular tyrosinase and melanin synthesis activities of methanol and hot water extracts from fruiting bodies of Phellinus vaninii. (A) Tyrosinase activity in B16-F10 melanoma cells; (B) Melanin synthesis activity in B16-F10 melanoma cells. Values are means ± standard deviation (SD, n = 4). ***p < .001, **p < .01, *p < .05 vs. Arbutin group; ###p < .001, ##p < .01 vs. Methanol extract group.
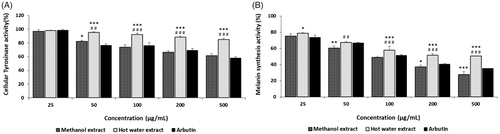
To assess whether the decreased intracellular tyrosinase activity and reduced melanin production observed in B16-F10 melanoma cells were due to cytotoxicity of the mushroom extracts, an MTT assay was performed. In the MTT assay, B16-F10 melanoma cells treated with ME and HE exhibited cell viabilities of 105% to 90.33%, and 107% to 91% at 50–500 µg/mL, respectively, indicating that the mushroom extracts were not toxic to B16-F10 melanoma cells at the concentrations tested ().
3.5.4. Melanin synthesis activity
To confirm whether the good cellular tyrosinase inhibitory activities detected in the previous experiment were related to melanin synthesis inhibition in B16-F10 melanoma cells, melanin production by the melanoma cells was further investigated. The melanin synthesis of B16-F10 melanoma cells treated with ME and HE ranged from 71.18% to 27.61% and from 78.54% to 50.53%, respectively, at 25–500 µg/mL, while the melanin synthesis by arbutin ranged from 73.50% to 35.16% at the concentration tested (). The study results suggest that inhibition of the melanin synthesis by arbutin was higher than that of HE but lower than that of ME. Increased concentrations of the mushroom extracts resulted in decreased melanin synthesis in B16-F10 melanoma cells. The results suggested that the inhibition of melanin synthesis in B16-F10 melanoma cells by ME was significantly higher than that of arbutin, while the inhibition of melanin production by HE was significantly lower than that of arbutin. Cha and Kim [Citation51] demonstrated that fermented extract of Cordyceps militaris exhibited 66–47% melanin synthetic activity at 1.0–3.0 mg/mL in B16-F10 melanoma cells, suggesting that inhibitory activity of melanin synthesis of fruiting body extracts of Ph. vaninii were higher than that of fermented extract of Cordyceps militaris. Therefore, the study results demonstrated that the mushroom extracts not only inhibited in vitro tyrosinase activity and L-DOPA auto-oxidation but also decreased tyrosinase activity and melanin production in B16-F10 melanoma cells. Taken together, our findings suggest that ME and HE from Ph. vaninii fruiting bodies may be regarded as potential novel anti-melanogenic sources of protection against skin pigmentation from the ultraviolet radiation of sunlight.
3.6. Skin anti-wrinkle activity
3.6.1. Elastase inhibition
Elastase, a protease, is responsible for the breakdown of elastin which is found in the ECM.
Elastases can cleave elastin, as well as other substrates, such as collagen, fibronectin and ECM proteins. However, under natural conditions, elastase activity is essential for the degradation of foreign proteins after wounding [Citation52]. To delay skin aging, such as wrinkles, sagging, and freckles, it is necessary to prevent the degradation of ECM-related proteins and thereby protect the loss of skin elasticity. The esterase inhibitory rate of ME and HE from the fruiting bodies of Ph. vaninii ranged from 37.23% to 71.24% and from 28.99% to 64.65% at 0.125–2.0 mg/mL, respectively, whereas EGCG demonstrated esterase inhibitions of 17.93 to 45.31% at the concentration tested (). The elastase inhibition rate of ME and HE might be considered excellent compared to EGCG. Kim et al. [Citation53] reported that mycelia extract of Tricholoma matsutake demonstrated that elastase inhibitory activity by 81.4% at 100 µg/mL, indicating that the inhibitory effects of ME and HE from Ph. vaninii on elastase were lower than that of T. matsutake extract. Choi and Lee [Citation54] demonstrated elastase inhibition by the methanol and hot water extracts from Coriolus versicolor fruiting bodies ranged from 21.5% to 35.47% and from 17.57% to 25.86% at 1.0 to 3.0 mg/mL, respectively, suggesting that the elastase inhibition of ME and HE were higher than those of C. versicolor fruiting bodies. The results suggest that the mushroom extracts possess anti-wrinkle potential to prevent elastin and collagen from degradation.
Figure 6. Elastase and collagenase inhibitory activities of methanol and hot water extracts from fruiting bodies of Phellinus vaninii. Values are means ± standard deviation (SD, n = 4). (A) Collagenase inhibitory activity; (B) Elastase inhibitory activity. EGCG, epigallocathechin-3-gallate. ***p < .001 vs. EGCG group; ###p < .001, ##p < .01, #p < .05 vs. Methanol extract group.
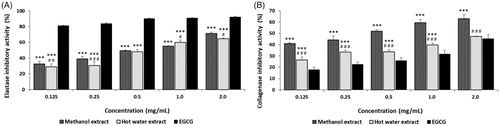
3.6.2. Collagenase inhibition
Collagenases are metalloproteinases capable of cleaving various molecules found within the cells and ECM, such as collagen, elastin, fibronectin, gelatin, and laminin. The collagenase used in this experiment, bacterial collagenase (Clostridium histolyticum, ChC), also degrades the ECM [Citation55]. Therefore, the bacterial collagenase was used to test the anti-collagenase activity of the mushroom extracts. The collagenase inhibitory activities of ME and HE from the fruiting bodies of Ph. vaninii ranged from 41.04% to 63.02% and from 26.40% to 47.33% at 0.125–2.0 mg/mL, respectively (), while EGCG inhibited collagenase 17.93 to 45.31% at the concentration tested. The results suggest that ME was an excellent inhibitor, whereas HE revealed moderate inhibitory effects at the concentrations tested. Cheon et al. [Citation56] reported that the collagenase inhibition by ethanol and hot water extracts from the fruiting bodies of Phellinus linteus ranged from 3.4% to 6.8% and from 33.4% to 89.6% at 0.05–1.0 mg/mL, respectively, indicating that collagenase inhibition by ME from Ph. vaninii was higher than that from hot water extract of Ph. linteus fruiting bodies Since ME and HE exhibited good inhibition of collagenase, the extracts may protect the ECM and collagen from degradation caused by photo-aging. Although most elastase and collagenase inhibitory activities have been demonstrated in plants, including white tea, green tea, mulberry bark, and ginkgo fruits [Citation57,Citation58], this is the first report of elastase and collagenase inhibitory activities from the fruiting body extracts from Ph. vaninii, and may represent a new anti-wrinkle agent source.
In conclusion, the fruiting body extracts from Ph. vaninii exhibited good antioxidant, anti-xanthine oxidase, tyrosinase, melanin synthesis, elastase, and collagenase inhibitory activities. The xanthine oxidase, tyrosinase, and melanin synthesis inhibitory activities could be used for natural sources to control gout and skin pigmentation agents, and inhibition of elastase and collagenase might be good sources for treating skin wrinkle-related disorders. Further research is necessary to determine the factors providing the anti-xanthine oxidase, anti-tyrosinase, and anti-wrinkle activities in the extracts from Ph. vaninii fruiting bodies.
Disclosure statement
No potential conflict of interest was reported by the authors.
Additional information
Funding
References
- Valko M, Leibfritz D, Moncol J. Free radicals and antioxidants in normal physiological functions and human disease. Int J Biochem Cell Biol. 2007;39(1):44–84.
- Aseervatham GSB, Sivasudha T, Jeyadevi R, et al. Environmental factors and unhealthy lifestyle influence oxidative stress in humans–an overview. Environ Sci Pollut Res. 2013;20(7):4356–4369.
- Spector A. Oxidative stress and disease. J Ocul Pharmacol Ther. 2000;16(2):193–201.
- Dawson J, Walters M. Uric acid and xanthine oxidase: future therapeutic targets in the prevention of cardiovascular disease? Br J Clin Pharmacol. 2006;62(6):633–644.
- Song JS. New classification criteria and guideline for management of gout. Kor J Med. 2018;93(4):344–350.
- Suresh E, Das P. Recent advances in management of gout. Q J Med. 2012;105(5):407–417.
- Kim S, Kim HJ, Ahn HS, et al. Renoprotective effects of febuxostat compared with allopurinol in patients with hyperuricemia: a systematic review and meta-analysis. Kidney Res Clin Pract. 2017;36(3):274–281.
- Nerya O, Vaya J, Musa R, et al. Glabrene and isoliquiritigenin as tyrosinase inhibitors from licorice roots. J Agric Food Chem. 2003;51(5):1201–1207.
- Hearing VJ. Biogenesis of pigment granules: a sensitive way to regulate melanocyte function. J Dermatol Sci. 2005;37(1):3–14.
- Briganti S, Camera E, Picardo M. Chemical and instrumental approaches to treat hyperpigmentation. Pigment Cell Res. 2003;16(2):101–118.
- Pillaiyar T, Manickam M, Namasivayam V. Skin whitening agents: medicinal chemistry perspective of tyrosinase inhibitors. J Enzyme Inhib Med Chem. 2017;32(1):403–425.
- Smit N, Vicanova J, Pavel S. The hunt for natural skin whitening agents. Int J Mol Sci. 2009;10(12):5326–5349.
- Sjerobabski-Masnec I, Šitum M. Skin aging. Acta Clin Croat. 2010;49(4):515–519.
- Muiznieks LD, Keeley FW. Molecular assembly and mechanical properties of the extracellular matrix: a fibrous protein perspective. Biochim Biophys Acta. 2013;1832(7):866–875.
- Shoulders MD, Raines RT. Collagen structure and stability. Annu Rev Biochem. 2009;78(1):929–958.
- Kielty CM, Sherratt MJ, Shuttleworth CA. Elastic fibres. J Cell Sci. 2002;115(Pt 14):2817–2828.
- Feeney MJ, Miller AM, Roupas P. Mushrooms–biologically distinct and nutritionally unique exploring a “third food kingdom. Nutr Today. 2014;49(6):301–307.
- Lindequist U, Niedermeyer THJ, Julich WD. The pharmacological potential of mushrooms. Evid based Complement Alternat Med. 2005;2(3):285–299.
- Rathee S, Rathee D, Rathee D, et al. Mushrooms as therapeutic agents. Rev Bras Farmacogn. 2012; 22:457–474.
- Dai YC. A new kind of medicinal fungi-Phellinus vaninii Ljup (Phellinus spp). Chin Edible Fungi. 2003;22:7–8.
- Hsieh PW, Wu JB, Wu YC. Chemistry and biology of Phellinus linteus. Biomed. 2013;3(3):105–113.
- Azeem U, Dhingra GS, Shri R. Pharmacological potential of wood inhabiting fungi of genus Phellinus Quél.: an overview. J Pharmacogn Phytochem. 2018;7(2):1161–1171.
- Cheng XY, Bao HY, Ding Y, et al. Free radical scavenging activities of phenolic and flavonoid compounds from fruiting body of Phellinus vaninii. Mycosystema. 2011;30:281–287.
- Hu W, Liu S, Zhang Y, et al. Mycelial fermentation characteristics and antiproliferative activity of Phellinus vaninii Ljup. Pharmacogn Mag. 2014;10:430–434.
- Singleton VL, Rossi JA. Jr. Colorimetry of total phenolics with phosphomolybdic phosphotungstic acid reagents. Amer J Enol Viticult. 1965;16:144–158.
- Zhishen J, Mengcheng T, Jianming W. The determination of flavonoid contents in mulberry and their scavenging effect on superoxide radicals. Food Chem. 1999;64(4):555–559.
- Mosmann T. Rapid colorimetric assay for cellular growth and survival: application to proliferation and cytotoxicity assays. Immunol Meth. 1983;65(1–2):55–63.
- Blois MS. Antioxidant determination by the use of a stable free radical. Nature. 1958;181(4617):1199–1200.
- Ruberto G, Baratta MT, Deans SG, et al. Antioxidant and antimicrobial activity of Foeniculum vulgare and Crithmum maritimum essential oils. Planta Med. 2000;66(8):687–693.
- Halliwell B, Gutteridge JM, Aruoma OI. The deoxyribose method: a simple “test-tube” assay for the determination of rate constants for reactions of hydroxyl radicals. Anal Biochem. 1987;165(1):215–219.
- Owen PL, Johns T. Xanthine oxidase inhibitory activity of north-eastern North American plant remedies used for gout. J Ethnopharmacol. 1999;64:149–160.
- Kim MJ, Ryu MJ. Inhibition of melanogenesis and anti-UV properties of Reynoutri eppitica. Kor J Aesthet Cosmotol. 2012;10:961–968.
- Masuda T, Yamashita D, Takeda Y, et al. Screening for tyrosinase inhibitors among extracts of seashore plants and identification of potent inhibitors from Garcinia subelliptica. Biosci Bitotechnol Biochem. 2005;69(1):197–201.
- Nagata H, Takekoshi S, Takeyama R, et al. Quercetin enhances melanogenesis by increasing the activity and synthesis of tyrosinase in human melanoma cells and normal human melanocytes. Pigment Cell Res. 2004;17(1):66–73.
- Hosoi J, Abe E, Suda T, et al. Regulation of melanin synthesis of B16 mouse melanoma cells by 1 alpha, 25-dihyfroxyvitamin D3 and retinoic acid. Cancer Res. 1985;45(4):1474–1478.
- Kim Y, Uyama H, Kobayashi S. Inhibition effects of (+)-catechinaldehyde polycondensates on proteinase causing proteolytic degradation of extracellular matrix. Biochem Biophys Res Commun. 2004;320(1):256–261.
- Van Wart HE, Steinbrink DR. A continuous spectrophotometric assay for Clostridium histolyticum collagenase. Anal Biochem. 1981;113(2):356–365.
- Vamanu E, Nita S. Antioxidant capacity and the correlation with major phenolic compounds, anthocyanin, and tocopherol content in various extracts from the wild edible Boletus edulis mushroom. BioMed Res Int. 2013;2013:313905.
- Kosanić M, Ranković B, Dašić M. Mushrooms as possible antioxidant and antimicrobial agents. Iranian J Pharmaceut Res. 2012;11(4):1095–1102.
- Nguyen TK, Im KH, Choi JH, et al. Evaluation of antioxidant, anti-cholinesterase, and anti-inflammatory effects of culinary mushroom Pleurotus pulmonarius. Mycobiology. 2016;44(4):291–301.
- Mau JL, Lin HC, Song SF. Antioxidant properties of several specialty mushrooms. Food Res Int. 2002;35(6):519–526.
- Obodai M, Ferreira I, Fernandes A, et al. The chemical and antioxidant properties of wild and cultivated mushrooms of Ghana. Molecules. 2014;19(12):19532–19548.
- Abdullah N, Ismail ST, Aminudin N, et al. Evaluation of selected culinary-medicinalmushrooms for antioxidant and ace inhibitory activities. Evid based Complement Alternat Med. 2012;2012:464238.
- Premkumari B, Shivashankar M. Study on in vitro free radical scavenging activity of Hypsizygus ulmarius mushroom. J Chem Pharmaceut Res. 2014;6(6):501–507.
- Yoon KN, Jang HS. Anti-xanthine oxidase, anti-cholinesterase, and anti-inflammatory activities of fruiting bodies of Phellinus gilvus. Kor J Clin Lab Sci. 2018;50(3):225–235.
- Nagano A, Seki M, Kobayashi H. Inhibition of xanthine oxidase by flavonoids. Biosci Biotechnol Biochem. 1999;63(10):1787–1790.
- Alam N, Yoon KN, Cha YJ, et al. Appraisal of the antioxidant, phenolic compounds concentration, xanthine oxidase and tyrosinase inhibitory activities of Pleurotus salmoneostramineus. Afr J Agric Res. 2011;6(6):1555–1563.
- Wang Y, Curtis-Long MJ, Lee BW, et al. Inhibition of tyrosinase activity by polyphenol compounds from Flemingia philipinensis root. Bioorganic Med Chem. 2014;22(3):1115–1120.
- Kim DH, Park JY, Kim JH, et al. Flavonoids as mushroom tyrosinase inhibitors: a fluorescence quenching study. J Agric Food Chem. 2006;54 (3):935–941.
- Huang HC, Hsu TF, Chao HL, et al. Inhibition of melanogenesis in murine melanoma cells by Agaricus brasiliensis methanol extract and anti-reactive oxygen species (ROS) activity. Afr J Microbiol Res. 2014;8:519–524.
- Cha JY, Kim SY. Anti-melanogenesis in B16F0 melanoma cells by extract of fermented Cordyceps militaris containing high cordycepin. J Life Sci. 2013;23(12):1516–1524.
- Tyagi SC, Simon SR. Regulation of neutrophil elastase activity by elastin-derived peptide. J Biol Chem. 1993;268(22):16513–16518.
- Kim SY, Go KC, Song YS, et al. Extract of the mycelium of T. matsutake inhibits elastase activity and TPA-induced MMP-1 expression in human fibroblasts. Int J Mol Med. 2014;34(6):1613–1621.
- Choi BY, Lee HH. Antioxidant and physiological activities of Coriolus versicolor fruit body crude extracts. J Kor Acad-Indust Coop Soc. 2016;17(8):415–422.
- Pruteanu M, Hyland NP, Clarke DJ, et al. Degradation of the extracellular matrix components by bacterial-derived metalloproteases: implications for inflammatory bowel diseases. Inflamm Bowel Dis. 2011;17(5):1189–1200.
- Cheon SJ, Jang MJ, Jang YA, et al. Anti-wrinkle effect of Cambodian Phellinus linteus extracts. J Life Sci. 2008;18(12):1718–1722.
- Thring TSA, Hili P, Naughton DP. Anti-collagenase, anti-elastase and anti-oxidant activities of extracts from 21 plants. BMC Complement Altern Med. 2009;9(1):1–11.
- Ghimeray AK, Jung US, Lee HY, et al. In vitro antioxidant, collagenase inhibition, and in vivo anti-wrinkle effects of combined formulation containing Punica granatum, Ginkgo biloba, Ficus carica, and Morus alba fruits extract collagenase. Clin Cosmet Investig Dermatol. 2015;8:389–396.