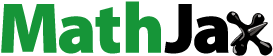
Abstract
Fungal ecological interactions play a key role in structuring community assemblages. These associations may involve both antagonistic and synergistic relationships, which are commonly influenced by abiotic factors such as nutrient conditions. However, information for extreme, oligotrophic systems remain poor. Herein, interactions among key members of the aquatic transient fungal community (Aspergillus niger, Cladosporium sp., and Coprinellus micaceus) of a low-nutrient freshwater system in the Cuatro Ciénegas Basin, Mexico were studied. Pairwise interaction bioassays were explored in vitro under different nutrient conditions, including carbohydrates-rich, carbohydrates and amino peptides-rich, and low nutrients. Our results indicated that antagonistic patterns prevail among the studied taxa. However, nutrient-dependent changes were observed in Cladosporium sp. shifting to synergy under carbohydrates-rich conditions, suggesting changes in the fungal community composition as a result of nutrient enrichment. Remarkably, our findings contrast with previous work demonstrating mainly synergistic interactions between our tested fungal isolates and co-occurring autochthonous bacteria (Aeromonas spp. and Vibrio sp.) under low-nutrient conditions. This observation may indicate that bacteria and fungi exhibit distinct community-level responses, driven by nutrient conditions. This contributes to the knowledge of fungal community dynamics and interspecific interactions in an oligotrophic ecosystem, highlighting the relevance of nutrient-based shifts and antagonistic interactions in ecosystem dynamics.
In nature microorganisms co-exist in close proximity, interacting and communicating with each other [Citation1,Citation2]. These polymicrobial associations occur at a microscale and represent important drivers of community structure and ecosystem dynamics. Two main ecological strategies have been proposed based on the co-aggregation (synergy) or segregation (antagonism) of organisms, where interactions may drive community function and diversity at larger geographical scales [Citation3]. Besides, a genetic basis has been proposed for these interactions (in prokaryotic systems), as similar genotypes tend to cooperate, whereas different genotypes tend to compete [Citation4].
The relationship between the severity of environmental stress and the relative importance of facilitation and competition has been conceptually formalized in the “stress-gradient hypothesis”, predicting that positive facilitative effects are more important under harsher condition and vice versa [Citation5]. Under this context, environmental conditions play an important role in the organization of communities [Citation6]. For instance, temperature-mediated changes have been documented in wood decay fungi [Citation7]. Specifically, nutrient conditions have been suggested to shape microbial interactions, with P and N concentrations as major determinants [Citation8,Citation9]. Nonetheless, the comprehension of microbial interactions is still at an early stage for non-model eukaryotic taxa such as saprotrophic fungi. Furthermore, information on fungal associations under extreme, nutrient-depleted ecosystems remains largely unknown.
Recent work has demonstrated that carbon and nitrogen sources affect the cross-kingdom interactions between fungi and bacteria. Consequently, the composition of organic inputs shapes nutrient turnover, due to its effect on the outcome of cross-kingdom interactions [Citation10]. This premise has been increasingly explored evidencing that nutrients (organic and inorganic) acquisition plays a central role in the interactions that fungi establish with the surrounding microbiota (reviewed in [Citation11]). Under this scope, nutrient-based fungal bacterial interactions have been described from an arid system characterized by significant low total phosphorus concentrations [Citation12], hereafter referred as oligotrophic [Citation13–15], suggesting that fungi may play a key role in promoting ecosystem functioning in nutrient-depleted, extreme habitats.
Antagonistic interactions have been reported as a common pattern in saprotrophic fungi [Citation16], influencing decomposition rates and carbon cycling [Citation17,Citation18]. Fungal antagonistic interactions are characterized by the secretion of assorted secondary metabolites of remarkable biological activities [Citation19], detoxification processes and the transformation of metabolites produced by other fungi as observed in Aspergillus niger [Citation20]. Overall, these investigations have evidenced that species-specific factors and/or effects are involved in pairwise interactions, and that A. niger may represent a highly competitive species dominating in dual cultures over competitors.
As natural communities represent a complex array of species, disentangling polymicrobial interactions under different nutrient scenarios is central to understanding ecosystem dynamics and energy fluxes in response to perturbations [Citation21]. The aim of this work was to evaluate pairwise in vitro interactions among fungal isolates obtained from a phosphorous-poor desert oasis, under different nutrient conditions to explore their inter- and intraspecific interactions. The experiments were conducted using isolates from the Churince system, in the Chihuahuan desert, an oligotrophic oasis, now mostly dry [Citation12] in order to increase baseline knowledge on the functioning of this endangered region. We hypothesize that beneficial interactions will dominate under low-nutrients media in accordance with the stress-gradient hypothesis.
The Cuatro Ciénegas Basin (CCB) is an inter-mountain closed basin with arid climate that harbors an extensive system of springs, streams and pools fed by subterranean water deposited by the end of the late Quaternary [Citation22,Citation23]. It has two marked seasons with temperatures fluctuating intensively: from November to April it is cold and dry (4–31 °C), and from May to October it is hot (15–35 °C) [Citation24]. It is characterized by low phosphorus levels, both in the water and soil, in comparison to other similar environments [Citation14]. Particularly, the Churince aquatic system is the oldest marsh in the CCB, yet in recent years sudden decreases in the water level have turned this unique ecosystem into a desolate space full of gypsum.
Interaction experiments were implemented with fungal isolates from the culture collection of the Laboratorio de Ecología Molecular de Micromicetes en Ecosistemas Amenazados (C-121), Instituto de Biología, Universidad Nacional Autónoma de México (available for research upon request). These fungi were isolated from the same water sample collected in the Churince freshwater system, CCB, Coahuila, Mexico, during an expedition in 2015, and have been tested for cross-kingdom interactions with bacterial isolates from the same site showing predominant synergistic patterns [Citation12]. It should be noted that plating procedures in the before-mentioned work, yielded to the sole isolation of four fungal taxa (Cladosporium sp., Coprinellus micaceus 1 and 2, and A. niger), herein tested.
Pairwise fungal interactions among A. niger, Cladosporium sp., and C. micaceus () were assessed on solid plate co-cultures using a modified agar plate antagonism bioassay [Citation25–28]. Three days old actively growing fungal plugs (circa 5 mm of diameter) were spot-inoculated on Petri dishes. Controls (mono-cultures) and test plates (co-cultures where inocula were placed at 3 mm from each other) were tested on four different agar media: carbohydrates-rich PDA (Fluka Analytical, Sigma-Aldrich, St. Louis, MO, USA), carbohydrates and amino peptides-rich (CP) medium (containing 10 g yeast extract, 11 g D(+)-glucose, 10 g NaCl, 15 g agar, 1000 mL distilled water, pH 6.2), and low-nutrient marine agar medium that resembles nutrient conditions in the CCB (MM, containing 5 g peptone, 1 g yeast extract, 0.08 g KBr, 0.034 g SrCl2, 0.022 g H3BO3, 0.024 NaF, 0.016 g NH4NO3, 0.08 g Na2HPO4, 0.004 g Na2SiO3, 5 g NaCl, 2.2 g MgCl2, 1 g Na2SO4, 0.4 g CaCl2, 15 g agar, 1000 mL distilled water).
Table 1. Information of the analyzed fungi isolated from a water sample collected in an oligotrophic desert oasis (N 26° 50′ 55.2′′, W 102° 08′ 34.8′′).
Fungal development and macroscopic morphological characteristics were inspected daily for 7 d. All the bioassays (both controls and test plates) were run in triplicate and incubated at 28 °C with a 12 h photoperiod. Photographic record of the experiment was registered using a Nikon D3000 digital SLR camera (Nikon Inc., Tokyo, Japan) at 72, 120, and 168 h after inoculation, using identical camera settings and light conditions. Fungal growth (area) was determined through image analysis using the software IMAGEJ 1.52a [Citation29].
The quantitative assessment of interactions was scored by calculating the Percent Inhibition Index (PII, Equation (1)) [Citation30], and the Overall Antagonism Index (OAI or resistance estimate, Equation (2)) [Citation31]. The PII denotes growth differences in mono-cultures in relation to co-cultures, whereas the OAI represents the overall ability of a given species to grow and to resist the presence of other fungi. High PII and OAI values may indicate competition/antagonism (−/−), while low values suggest a neutral interaction (0/0), and negative values designate a relation of cooperation/symbiosis (+/+). Both indexes were calculated for days 5 and 7, since growth in co-culture for day 3 was insufficient (no physical contact) to detect patent interactions. Statistical analyses and plots were carried out with MATLAB version R2017b. The statistical significance of interactions was tested with a one-way ANOVA (p < .05) for the fifth and seventh days using software PAST version 2.17c [Citation32].
Equation (1) PII, where Gc is the total growth area in controls; Gi is the total growth area of interacting species; Ic is the inoculated area in controls; and Ii is the inoculated area of interacting species
(1)
(1)
Equation (2) OAI, which refers to the summation of mean fungal growth during interaction for days 5 and 7
(2)
(2)
Our results indicated that fungal growth varied among the tested taxa in response to nutrient conditions (Supplementary Data 1). Globally, in carbohydrates-rich PDA medium the overall radial growth of the three tested fungal isolates was lower compared to CP and MM (). Morphological differences were also detected in the isolates across the different tested culture media (). Mycelia of A. niger and C. micaceus grew profusely on CP, reaching the biggest colony area. Whereas, on MM these two fungi showed rapid radial growth rates, yet hyphal lateral branching was poor. On this nutrient-poor medium, poor sporulation was observed for A. niger. Moreover, on CP and PDA Cladosporium sp. formed crater-like, radially furrowed, olivaceous green colonies. In contrast to MM, where fluffy colonies were observed. On the reverse side, colonies were black (PDA), green (CP), and light maroon color (MM).
Figure 1. Fungal growth in test plates (co-cultures) and controls (mono-cultures) on three different culture media, x-axis represents days and y-axis signifies fungal radial growth (cm2). Controls (A, B, and C) and test plates on: PDA (D–F), CP (G–I), and MM (J–L); where D, G, and J show A. niger in co-culture; E, H, and K show Cladosporium sp. in co-culture; F, I, and L show C. micaceus in co-culture.
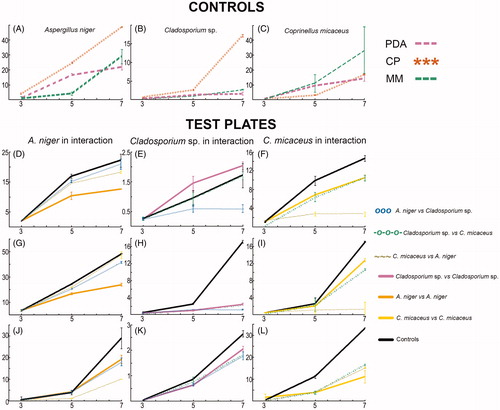
Figure 2. Culture characteristics during intra- and interspecific microfungal pairwise interactions on different culture media: PDA, CP, and MM. Controls: Aspergillus niger (1, 10, 19), Coprinellus micaceus (2, 11, 20), Cladosporium sp. (3, 12, 21). Interactions: A. niger vs A. niger (4, 13, 22), C. micaceus vs. C. micaceus (5, 14, 23), Cladosporium sp. vs. Cladosporium sp. (6, 15, 24), A. niger vs. Cladosporium sp. (7, 16, 25), A. niger vs. C. micaceus (8, 17, 26), C. micaceus vs Cladosporium sp. (9, 18, 27). Front (a) and back (b) of the petri dish.
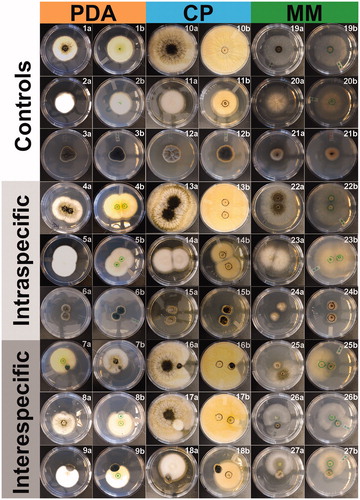
The quantitative evaluation of interspecific interactions evidenced that C. micaceus showed the highest OAI values in all the tested media (), representing a predominantly competitive species irrespective of nutrient conditions. In addition, pairwise comparisons (PII) revealed the following antagonist patterns: C. micaceus > Cladosporium sp. > A. niger on all media. However, on MM nutrient-dependent changes were observed, favoring A. niger over C. micaceus. We also observed nutrient-mediated changes in the interactions between isolates from the same species (same genet). These differences were evident under nutrient-depleted conditions for A. niger and C. micaceus fungi, as the highest PIIs were observed in MM (). Considering that fungal growth may determine fitness (extent of reproduction or survival) during adverse conditions [Citation33], our results exposed the key role of nutrient conditions in modeling interspecific interactions, particularly for aggressive species such as A. niger (with considerable demands for nutrients and space).
Table 2. Overall antagonism index for each fungal species on the three tested culture media: carbohydrates-rich potato dextrose agar medium (PDA), carbohydrates and amino peptides-rich medium (CP), low-nutrient marine agar medium (MM).
Table 3. Fungal percentage of inhibition index (PII) on the three tested culture media: carbohydrates-rich potato dextrose agar medium (PDA), carbohydrates and amino peptides-rich medium (CP), low-nutrient marine agar medium (MM). Values are presented in the same order as the interacting organisms.
All of the evaluated taxa inhibited the growth of their counterpart to some degree. Remarkably, the detected prevailing antagonistic interactions (especially under low-nutrient conditions, ) agree with further observations on aquatic fungi [Citation34,Citation35] including marine taxa [Citation36,Citation37]. However, our results were unexpected given previous observations on the cross-kingdom interactions with Aeromonas and Vibrio isolates (obtained from the same site and sampling), where beneficial interactions dominated in low-nutrients media, suggesting predominant synergistic interactions under low-nutrient conditions [Citation12] in accordance with the stress-gradient hypothesis [Citation5].
Figure 3. Schematic representation of fungal interactions in co-culture on PDA (A), CP (B), and MM (C), where Aspergillus niger is represented as AS, Cladosporium sp. as CL, and Coprinellus micaceus as CO. Red lines indicate antagonism, black lines neutral interactions, and green lines synergistic interactions. Line width is proportional to the intensity of interactions.
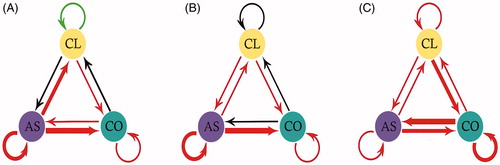
Predominant antagonistic interactions among the tested fungi also suggest a niche overlap that increases competition under limited resources as has been observed in CCB bacterial community, where interactions with “foreigners” or nonmembers of the community are epitomized by a fierce competition [Citation38–40]. Globally, interspecific competitive interactions among members of the microbial community in the CCB may be associated with “The Red Queen Model” [Citation41]. This model proposes a coevolution process driven by constant competitive interactions between species to gain reproductive advantage and proliferate under fluctuating environmental conditions and interacting with opposing organisms, as seen in the association among Cladosporium sp., A. niger, and C. micaceus. Furthermore, the observed antagonistic patterns under low-nutrient conditions (MM) may be associated with niche overlapping between fungal species, in contrast to previously reported synergistic fungal–bacterial interactions [Citation12].
However, nutrient-based shifts leading to positive intraspecific interactions were observed for Cladosporium sp. on PDA, a carbohydrate-rich culture medium. This cooperative association (PDA, , green arrow) agrees with the inclusive fitness theory, which postulates that cooperative interactions should be restricted to similar genotypes [Citation4]. In contrast, A. niger and C. micaceus exhibited an antagonistic behavior when paired with themselves in mono-cultures on all the tested media, resembling the classic competitive exclusion principle [Citation42]. Moreover, this theory also predicts opposition between different genotypes when competing for resources [Citation4]; accordingly, the three tested fungi displayed the highest OAI on MM (low-nutrients, ; ), suggesting a stronger confrontation when resources are scarce.
Diverse interspecific associations have been reported for Cladosporium members, which depend on the media and the co-cultured microorganism. These interactions range from moderate to low antagonistic patterns by Cladosporium cladosporioides [Citation43,Citation44], to growth inhibition by fungi such as Trichoderma and Ralstonia pickettii on rich media [Citation45]. In addition, a neutral association with aquatic bacteria such as Chryseobacterium sp. has been reported [Citation46,Citation47]. Our results agree with observations on the growth inhibition of Cladosporium by other fungi on rich media, yet this fungus may resist repression from other species on poor media as suggested by the presence of an inhibition halo ( and ).
Similarly, a number of ecological interactions have been described for A. niger on rich media. For instance, this species may inhibit the growth of pathogenic species such as Colletotrichum gloeosporioides and Pestalotia psidii [Citation43]. Nevertheless, strong antagonism by bacteria such as Bacillus and Pseudomonas chlororaphis has been observed as well [Citation48,Citation49]. Furthermore, compatibility between A. niger and Penicillium sp. has been described under rich nutrient conditions [Citation50]. Our findings agree with the antagonistic behavior of A. niger against other fungi on rich media. However, we observed that on poor media this fungus represents a mild competitor.
The interspecific interactions of C. micaceus have been extensively investigated on rich media, leading to the documentation of inhibition patterns toward pathogenic fungi such as Fusarium culmorum, Bipolaris sorokiniana, Gaumenomyces graminis var. tritici, Verticillium dahlae, Rhizoctonia solani, Rhizoctonia cerealis, Gliocladium roseum, Trichoderma asperellum, Trichoderma harzianum, Trichoderma pseudokoningii, Trichoderma viride, Alternaria alternata, Alternaria wentii, Fusarium tricinctum, Microsporum gypseum, Penicillium griseofulvum [Citation51]. Also, neutral patterns (no interaction) have been described with bacteria (Pectobacterium carotovorum, Pectobacterium syringae, and Xanthomonas campestris) and the fungus Stereum hirsutum [Citation52,Citation53]. Lastly, synergistic interactions have been documented with fungi such as Fomes fomentarius and Eutypa spinosa [Citation52]. Our CCB strain of C. micaceus is a poor competitor on rich as well as on poor media, being susceptible to inhibition by A. niger and Cladosporium sp. ().
Carbohydrates-rich PDA is frequently used to culture fungi, since this medium encourages fungal sporulation, pigment production, and luxuriant growth [Citation54]. However, the tested fungi from the Churince, an oligotrophic system (low in P), showed the best growth rates on CP (amino peptides-rich) and MM (low-nutrient) suggesting an adaptation to their environment. Considering the characteristics of CP and MM media, it is possible C does not represent a growth-limiting nutrient for fungi in this site, and instead N and P are major drivers of community assemblage.
The investigation of processes and mechanisms underlying fungal interactions is crucial to understand community dynamics and the maintenance of microbial biodiversity, especially in nutrient-poor systems. The idea that facilitative interactions increase as environmental conditions become more stressful has become a ruling paradigm in ecology [Citation55]. However, our results suggest that in the CCB (characterized by oligotrophic conditions) interspecific fungal competition for nutrients and space may lead to prevailing antagonistic interactions, where the intensity of this relation depends on the species or genotype, and the culture media (carbon and nitrogen concentrations).
Although several factors such as inoculum potential, conidia germination efficiency, growth rate, and substrate utilization may affect in vitro experiments, our findings indicated that paired interactions between Cladosporium sp. isolates may shift to synergy as a result of nutrient enrichment. This information has important implications in view of the continued threats to the endangered CCB, which suffers from increasing human impacts due to the aquifer overexploitation, agriculture and cattle settlements, which may be associated with nutrient increments [Citation56,Citation57]. These activities are causing the collapse of aquatic environments in the region, leading to changes in the fungal community assemblage. According to our results, these changes may be characterized by the proliferation of species such as Cladosporium sp. (presenting synergistic intraspecific interactions) and strong competitors (e.g. Coprinellus micaceus). Results presented here act as a guide to the outcome of the fungal interactions in nature under fluctuating nutrient conditions. However, further in situ detailed studies are encouraged to confirm this hypothesis.
Supplemental Material
Download MS Excel (45.9 KB)Acknowledgements
The authors thank anonymous reviewers for valuable comments on earlier versions of the manuscript, Erika Aguirre-Planter and Laura Espinosa-Asuar for assistance in the laboratory. This paper was written during a sabbatical leave of LEE and VSS in the University of Minnesota in Peter Tiffin and Michael Travisano laboratories, respectively, with support by scholarships from PASPA, DGAPA, UNAM.
Disclosure statement
No potential conflict of interest was reported by the author(s).
Additional information
Funding
References
- Strobel G, Daisy B. Bioprospecting for microbial endophytes and their natural products. Microbiol Mol Biol Rev. 2003;67(4):491–502.
- Aly AH, Debbab A, Proksch P. Fungal endophytes: unique plant inhabitants with great promises. Appl Microbiol Biotechnol. 2011;90(6):1829–1845.
- Cordero OX, Datta MS. Microbial interactions and community assembly at microscales. Curr Opin Microbiol. 2016;31:227–234.
- Mitri S, Richard Foster K. The genotypic view of social interactions in microbial communities. Annu Rev Genet. 2013;47:247–273.
- Kawai T, Tokeshi M. Testing the facilitation–competition paradigm under the stress-gradient hypothesis: decoupling multiple stress factors. Proc Biol Sci. 2007;274(1624):2503–2508.
- Choudhury AJA, Trevelyn PMJ, Boswell GP. A mathematical model of nutrient influence on fungal competition. J Theor Biol. 2017;438:9–20.
- Hiscox J, Clarkson G, Savoury M, et al. Effects of pre-colonisation and temperature on interspecific fungal interactions in wood. Fungal Ecol. 2016;21:32–42.
- Grattan RM, Suberkropp K. Effects of nutrient enrichment on yellow poplar leaf decomposition and fungal activity in streams. J N Am Benthol Soc. 2001;20(1):33–43.
- Gulis V, Suberkropp K. Interactions between stream fungi and bacteria associated with decomposing leaf litter at different levels of nutrient availability. Aquat Microb Ecol. 2003;30:149–157.
- Lohberger A, Spangenberg JE, Ventura Y, et al. Effect of organic carbon and nitrogen on the interactions of Morchella spp. and bacteria dispersing on their mycelium. Front Microbiol. 2019;10:124.
- Deveau A, Bonito G, Uehling J, et al. Bacterial–fungal interactions: ecology, mechanisms and challenges. FEMS Microbiol Rev. 2018;42(3):335–352.
- Velez P, Espinosa-Asuar L, Figueroa M, et al. Nutrient dependent cross-kingdom interactions: microfungi and bacteria from an oligotrophic desert oasis. Front Microbiol. 2018;9:1755.
- Mckee JW, Jones NW, Long LE. Stratigraphy and provenance of strata along the San Marcos fault, central Coahuila, Mexico. Geol Soc Am Bull. 1990;102(5):593–561.
- Elser JJ, Schampel JH, Garcia‐Pichel F, et al. Effects of phosphorus enrichment and grazing snails on modern stromatolitic microbial communities. Freshwater Biol. 2005;50(11):1808–1825.
- Lee ZM, Steger L, Corman JR, et al. Response of a stoichiometrically imbalanced ecosystem to manipulation of nutrient supplies and ratios. PloS One. 2015;10(4):e0123949.
- Boddy L. Interspecific combative interactions between wood-decaying basidiomycetes. FEMS Microbiol Ecol. 2000;31(3):185–194.
- Hiscox J, O'leary J, Boddy L. Fungus wars: basidiomycete battles in wood decay. Stud Mycol. 2018;89:117–124.
- Hiscox J, Savoury M, Vaughan IP, et al. Antagonistic fungal interactions influence carbon dioxide evolution from decomposing wood. Fungal Ecol. 2015;14:24–32.
- Keller NP, TurneR G, Bennett JW. Fungal secondary metabolism – from biochemistry to genomics. Nat Rev Microbiol. 2005;3(12):937–947.
- Chatterjee S, Kuang Y, Splivallo R, et al. Interactions among filamentous fungi Aspergillus niger, Fusarium verticillioides and Clonostachys rosea: fungal biomass, diversity of secreted metabolites and fumonisin production. BMC Microbiol. 2016;16:83.
- Ingham RE, Trofymow JA, Ingham ER, et al. Interactions of bacteria, fungi, and their nematode grazers: effects on nutrient cycling and plant growth. Ecol Monogr. 1985;55(1):119–140.
- Meyer ER. Late‐quarternary paleoecology of the Cuatro Cienegas Basin, Coahuila, Mexico. Ecology. 1973;54(5):982–995.
- Wolaver BD, Sharp JM, Jr Tidwell VC, et al. An integrative approach to sustainable groundwater and associated groundwater-dependent system management in arid karst aquifers: Cuatrociénegas Basin, Mexico. International Association Hydrogeologists. 2008.
- CONAGUA. Normales climatológicas por estación. Ciudad de México: Servicio Meteorológico Nacional, CONAGUA; 2015.
- Reddi GS, Rao AS. Antagonism of soil actinomycetes to some soil-borne plant pathogenic fungi. Indian Phytopathol. 1971;24:649–657.
- Rothrock CS, Gottlieb D. Role of antibiosis in antagonism of Streptomyces hygroscopicus var. geldanus to Rhizoctonia solani in soil. Can J Microbiol. 1984;30(12):1440–1447.
- Crawford DL, Lynch JM, Whipps JM, et al. Isolation and characterization of actinomycete antagonists of a fungal root pathogen. Appl Environ Microbiol. 1993;59(11):3899–38905.
- Chamberlain K, Crawford DL. In vitro and in vivo antagonism of pathogenic turfgrass fungi by Streptomyces hygroscopicus strains YCED9 and WYE53. J Ind Microbiol Biotechnol. 1999;23(1):641–646.
- Schneider CA, Rasband WS, Eliceiri KW. NIH Image to ImageJ: 25 years of image analysis. Nat Methods. 2012;9(7):671–675.
- Mishra VK. In vitro antagonism of Trichoderma species against Pythium aphanidermatum. J Phytol. 2010;2:28–35.
- Asthana A, Shearer CA. Antagonistic activity of Pseudohalonectria and Ophioceras. Mycologia. 1990;82(5):554–561.
- Hammer Ø, Harper DA, Ryan PD. PAST-PAlaeontological Statistics. 2001. http://palaeo-electronica.org/2001_1/past/pastrog/past.pdf
- Skidmore AM, Dickinson CH. Colony interactions and hyphal interference between Septoria nodorum and phylloplane fungi. Trans Brit Mycol Soc. 1976;66(1):57–64.
- Shearer CA, Zare-Maivan H. In vitro hyphal interactions among wood- and leaf-inhabiting Ascomycetes and Fungi Imperfecti from freshwater habitats. Mycologia. 1988;80(1):31–37.
- Yuen TK, Hyde KD, Hodgkiss IJ. Interspecific interactions among tropical and subtropical freshwater fungi. Microb Ecol. 1999;37(4):257–262.
- Miller JD, Jones EBG, Moharir YE, et al. Colonization of wood blocks by marine fungi in Langstone Harbour. Bot Mar. 1985;28:251–257.
- Strongman DB, Miller JD, Calhoun L, et al. The biochemical basis for interference competition among some lignicolous marine fungi. Bot Mar. 1987;30:21–26.
- Pérez-Gutiérrez RA, López-Ramírez V, Islas A, et al. Antagonism influences assembly of a Bacillus guild in a local community and is depicted as a food-chain network. ISME J. 2013;7(3):487–497.
- Ponce-Soto GY, Aguirre-Von-Wobeser E, Eguiarte LE, et al. Enrichment experiment changes microbial interactions in an ultra-oligotrophic environment. Front Microbiol. 2015;6:246.
- Zapién-Campos R, Olmedo-Álvarez G, Santillán M. Antagonistic interactions are sufficient to explain self-assemblage of bacterial communities in a homogeneous environment: a computational modeling approach. Front Microbiol. 2015;6:489.
- Van Valen L. A new evolutionary law. Evol Theory. 1973;1:1–30.
- Gause CF. The struggle for existence. Baltimore: Williams & Wilkins; 1971.
- Pandey RR, Arora DK, Dubey RC. Antagonistic interactions between fungal pathogens and phylloplane fungi of guava. Mycopathologia. 1993;124(1):31–39.
- Morón-Ríos A, Gómez-Cornelio S, Ortega-Morales BO, et al. Interactions between abundant fungal species influence the fungal community assemblage on limestone. PloS One. 2017;12(12):e0188443.
- Barbosa MA, Rehn KG, Menezes M, et al. Antagonism of Trichoderma species on Cladosporium herbarum and their enzimatic characterization. Braz J Microbiol. 2001;32:98–104.
- Mille‐Lindblom C, Fischer H, Tranvik L. Antagonism between bacteria and fungi: substrate competition and a possible tradeoff between fungal growth and tolerance towards bacteria. Oikos. 2006;113(2):233–242.
- Baschien C, Rode G, Böckelmann U, et al. Interactions between hyphosphere-associated bacteria and the fungus Cladosporium herbarum on aquatic leaf litter. Microb Ecol. 2009;58(3):642–650.
- Palumbo JD, Baker JL, Mahoney NE. Microbial ecology isolation of bacterial antagonists of Aspergillus flavus from almonds. Microb Ecol. 2006;52(1):45–52.
- Ranjbariyan A, Shams-Ghahfarokhi M, Kalantari S, et al. Molecular identification of antagonistic bacteria from Tehran soils and evaluation of their inhibitory activities toward pathogenic fungi. Iran J Microbiol. 2011;3(3):140–146.
- Saber W, Ghanem KM, El-Hersh MS. Rock phosphate solubilization by two isolates of Aspergillus niger and Penicillium sp. and their promotion to mung bean plants. Res J Microbiol. 2009;4(7):235–250.
- Melikyan LR. Antifungal activity of several xylotrophic coprinoid mushrooms against filamentous fungi. Electron J Nat Sci. 2015;25:12–15.
- Heilmann-Clausen J, Boddy L. Inhibition and stimulation effects in communities of wood decay fungi: exudates from colonized wood influence growth by other species. Microb Ecol. 2005;49(3):399–406.
- Miles LA, Lopera CA, González S, et al. Exploring the biocontrol potential of fungal endophytes from an Andean Colombian Paramo ecosystem. BioControl. 2012;57(5):697–710.
- Booth C. Chapter II. Fungal culture media. In: Booth C, editor. Methods in microbiology. London: Academic Press; 1971.
- Holmgren M, Scheffer M. Strong facilitation in mild environments: the stress gradient hypothesis revisited. J Ecol. 2010;98(6):1269–1275.
- Contreras-Balderas S. Environmental impacts in Cuatro Ciénegas, Coahuila, México: a commentary. J Arizona-Nevada Acad Sci. 1984;19:85–88.
- Souza V, Espinosa-Asuar L, Escalante AE, Eguiarte LE, et al. An endangered oasis of aquatic microbial biodiversity in the Chihuahuan desert. Proc Natl Acad Sci USA. 2006;103(17):6565–6570.