Abstract
The antifungal activity of thymol against Aspergillus awamori F23 and Botrytis aclada F15 in onions was examined through direct treatment with amended media and gaseous treatment with I-plates (plastic plates containing central partitions). The protective and curative control efficacy of thymol was examined 24 h before and after the inoculation of onion bulbs with the fungal isolates. Mycelial growth, sporulation, and spore germination of the isolates were inhibited on potato dextrose agar amended with various concentrations of thymol or acetic acid (positive control). Overall, thymol produced a stronger inhibitory effect on the mycelial growth and development of the isolates than acetic acid. Following gaseous treatment in I-plates, mycelial growth, sporulation, and spore germination of the isolates were inhibited at higher concentrations of thymol or acetic acid; however, acetic acid showed a little effect on the sporulation and spore germination of the isolates. Following the treatment of onion bulbs with 1000 mg L−1 of thymol 24 h before and after fungal inoculation, lesion diameter was greatly reduced compared with that following treatment with 0.5% ethanol (solvent control). Onion bulbs sprayed with thymol 24 h before fungal inoculation generally showed reduced lesion diameters by isolate F23 but not in isolate F15 compared with those sprayed 24 h after fungal inoculation. Collectively, thymol effectively inhibited the growth and development of A. awamori and B. aclada on amended media and in I-plates. In addition, spraying or fumigation of thymol is more desirable for effectively controlling these postharvest fungal pathogens during long-term storage conditions.
1. Introduction
Onions (Allium cepa L.) are primarily cultivated in temperate and sub-tropical regions and are consumed in almost every culture and country [Citation1]. In Korea, this crop is one of the most important and seasonal vegetables; thus, consistent supply to consumers must be ensured through long-term postharvest storage. However, onions are one of the most vulnerable vegetables to postharvest fungal pathogens, such as Aspergillus spp., Botrytis spp., Fusarium spp., and Penicillium spp., under storage conditions [Citation2–7]. These pathogens causing postharvest diseases often lead to the deterioration of vegetable quality and nutrient composition, eventually resulting in postharvest losses and shortening of storage duration.
In onions, conventional postharvest disease control relies on the use of chemical fungicides before or after harvest. However, consumers have been paying increasing attention toward safer food for consumption and prefer less harmful control measures to the environment and human health. To cope with this consumer trend, alternative control measures, such as the use of antifungal microorganisms or natural products, have been extensively explored [Citation8–11]. However, controlling postharvest diseases at the desirable level is challenging due to the lack of multiple options for disease control, although postharvest biocontrol has been explored for a long time [Citation12]. In this context, plant extracts and antagonistic microbes may be promising alternatives without further regulation of use as postharvest products [Citation13–15]. Therefore, studies on the biocontrol of the storage diseases of onions, including the practical use of plant-derived materials, may offer not only economic (in terms of crop loss) but also human health (in terms of safety) benefits [Citation16].
In particular, essential oils have garnered much attention due to their environmentally sound characteristics [Citation17,Citation18]. Plant-derived essential oils often contain diverse chemical components such as terpenes, aldehydes, ketones, and phenolic compounds [Citation19–22]. Previously, Maqbool et al. [Citation22] reported that cinnamon oil exhibited antifungal activity against Colletotrichum gloeosporioides in banana. Moreover, thyme oil exhibited control efficacy against C. gloeosporioides in avocado and Penicillium digitatum in citrus lemon [Citation23,Citation24]. Similarly, Arrebola et al. [Citation25] reported that lemongrass oil from Cymbopogon citratus inhibited the mycelial growth of Botrytis cinerea and Rhizopus stolonifera in peach fruit. In addition, Vitoratos et al. [Citation26] tested various plant essential oils against B. cinerea, Penicillium italicum, and P. digitatum, and observed that lemongrass essential oil from C. citratus and tea tree oil from Melaleuca plants showed antifungal activity against B. cinerea. Among these plant-derived compounds, thymol [a colorless crystalline monoterpene phenol (2-isopropyl-5-methylphenol) isolated from the essential oil of thyme (Thymus vulgaris L. thymoliferum)] inhibited the growth of many plant fungal pathogens, such as Alternaria alternata, B. cinerea, and C. gloeosporioides [Citation27–30]. Moreover, thymol vapor as fumigation treatment inhibited postharvest brown and gray mold rot in apricot [Citation31]. Occasionally, thymol exhibits synergistic antifungal activity with salicylic acid (an inducer of systemic resistance of the host) against Fusarium solani, a postharvest fungal pathogen of many fruits and vegetables [Citation32]. Another recent study showed that thymol protected tobacco under salt stress (abiotic stress) through increased sodium ion efflux and elevated nitric oxide and glutathione levels [Citation33]. Further, thymol has been regarded safe by the Food and Drug Administration and Environmental Protection Agency of the United States [Citation34].
To this end, in the present study, antifungal activity of thymol against Aspergillus awamori, which causes black mold rot, and Botrytis aclada, which causes gray mold rot of onions, was investigated through direct treatments with amended media and gaseous treatment with I-plates (plastic plates containing central partitions). Moreover, the protective and curative efficacy of thymol against the fungal isolates on onion bulbs was examined.
2. Materials and methods
2.1. Fungal isolates
The A. awamori isolate F23 and B. aclada isolate F15 derived from diseased onion bulbs, which were maintained on potato dextrose agar (PDA) at 4 °C for long-term storage in our laboratory, were used in this study. The A. awamori F23 (KACC 47249) and B. aclada F15 (KACC 47250) have been deposited to the Korean Agricultural Culture Collection (KACC) (Wanju, Korea). Isolate F23 was initially deposited to KACC as Aspergillus niger; subsequently, it was re-identified as A. awamori as observed in our other work [Citation7]. These isolates of A. awamori and B. aclada were cultured on PDA for 5 days at 28 °C and 20 °C, respectively. Spores of the cultures were harvested using sterile distilled water with 0.03% Tween 20, and spore concentration was adjusted to 1 × 106 spores mL−1 using a hemocytometer for use as inocula.
2.2. Ethanol concentration for thymol-amended media
Various concentrations of absolute ethanol as the solvent for thymol were tested to determine the maximum concentration that does not affect fungal growth. The final ethanol concentration in PDA was adjusted to 0%, 0.01%, 0.1%, 0.2%, 0.5%, and 1% of the total medium volume. Then, 2 µL L−1 of the spore suspension (1 × 106 spores mL−1) of A. awamori F23 and B. aclada F15 was drop-inoculated on PDA and further incubated at 28 °C and 20 °C, respectively. Mycelial growth (mm) of isolates F23 and F15 was determined 6 and 7 days after incubation, respectively. The experiment was conducted two times with three replicates each.
2.3. Fugal growth and development on thymol-amended medium
Various concentrations of thymol [0 (unamended control), 1, 10, 50, 100, 200, and 250 mg L−1] or glacial acetic acid (GAA; positive control) [0 (unamended control), 1, 10, 100, 200, 500, and 1000 µL L−1] were added to the PDA medium. Thymol dissolved in absolute ethanol was added to the medium (final ethanol concentration = 0.1% of the total medium volume). Spore suspensions (2 µL of 1 × 106 spores mL−1 of 0.03% Tween 20) of A. awamori F23 and B. aclada F15 were drop-inoculated at the center of the culture plate containing PDA amended with various concentrations of thymol or acetic acid and incubated at 28 °C and 20 °C, respectively. Mycelial growth (mm) of isolates F23 and F15 was determined 6 and 7 days after incubation, respectively. Relative mycelial growth was determined as mycelial diameter on the thymol- or acetic acid-amended medium divided by mycelial diameter on the unamended control medium. Quadratic regression analysis of relative mycelial growth on log10-transformed thymol or acetic acid concentrations was applied to fit the dose-response curves. From the dose-response curves, the EC50 values and 95% fiducial limit for the fungal isolates were determined. The experiment was conducted two times with three replicates each.
Next, the spores of A. awamori and B. aclada isolates on thymol or acetic acid-amended PDA were harvested using 10 mL of 0.03% Tween 20 per plate. Mycelial debris was removed after passing through two layers of sterile cheesecloth. Harvested spores were counted using a hemocytometer after adjusting to proper spore concentration. To evaluate the germination rate of the harvested spores of isolates F23 and F15, spore suspension (2 µL of 1 × 106 spores mL−1 of 0.03% Tween 20) was dropped on a sterile glass slide. The glass slide with two drops (sub-replication) of spore suspensions deposited at a distance was placed on a wet filter paper in a petri plate to avoid drying of the spore drops. Germination (%) of the fungal spores was determined 18 h after incubation at 28 °C for A. awamori F23 and 20 °C for B. aclada F15. The experiment was conducted twice with three replicates (glass slide) each.
2.4. Fungal growth and development in I-plates containing volatile thymol
Various concentrations of thymol (5 mL) [0 (unamended control), 10, 100, 200, 500, and 1000 mg L−1] (final ethanol concentration = 0.1% of the total medium volume) or GAA (positive control) [0 (unamended control), 10, 100, 200, 500, and 1000 µL L−1] were applied to one side of the I-plates (plastic plates containing central partitions) (Fisher Scientific, Pittsburgh, PA, USA) without medium. On the other side (PDA) of the plates, spore suspensions (2 µL of 1 × 106 spores mL−1 of 0.03% Tween 20) of A. awamori F23 and B. aclada F15 were drop-inoculated. The plates were incubated for 6 days at 28 °C for A. awamori F23 and for 7 days at 20 °C for B. aclada F15. Mycelial growth, sporulation, and germination rate of the fungal isolates were evaluated as described above. Determination of relative mycelial growth, quadratic regression analysis, and determination of the EC50 values and 95% fiducial limit were also conducted as described above. The experiment was conducted two times with three replicates each.
2.5. Disease evaluation on thymol-treated onion bulbs
To test the control efficacy of thymol against A. awamori F23 and B. aclada F15, the fungal isolates were inoculated on half-cut onion bulbs originating from Muan, Jeonnam Province, Korea, which were purchased from the Gyeongdong Market, Seoul, Korea [Citation5]. Briefly, the half-cut onion bulbs were treated with 0.5% ethanol (solvent control), GAA (2000 µL L−1) (positive control), and thymol (1000 mg L−1) at concentrations determined based on EC50 values from previous tests. In addition, fluazinam (400 µL L−1, commercial concentration) was used as the positive fungicide control. The test chemicals were sprayed on the inner layers of the half-cut onion bulbs 24 h before (i.e., protective efficacy) and after (i.e., curative efficacy) inoculation with the fungal isolates. For inoculation, the isolates were grown on PDA as described above, and mycelial plugs (5 mm in diameter) were placed on the inner layers of the bulbs [three points (sub-replicates) per bulb]. Agar plugs without mycelia were used as uninoculated controls. Next, the inoculated bulbs were placed on mesh screens in plastic containers (16.0 × 23.0 × 12.5 cm3) with three layers of wet paper towels. The containers were tightly closed and incubated at 28 °C for A. awamori F23 and 20 °C for B. aclada F15. At 5 days after incubation, lesion diameters (mm) on the inner layers of the inoculated onion bulbs were determined. The experiment was conducted two times with three replicates (bulbs) each.
2.6. Statistical analysis
All experiments were arranged in a completely randomized design, and the data were analyzed using Statistical Analysis Systems software (SAS Institute, Cary, NC, USA). Quadratic regression analysis of the relative mycelial diameters of A. awamori F23 and B. aclada F15 was performed using polynomial regression in SigmaPlot® 2001 for Windows (SPSS Inc., Chicago, USA) to obtain the best fit equation for thymol and acetyl acid. The EC50 values and 95% fiducial limit of the isolates to thymol and acetyl acid was determined using probit analysis. The log10-transformation of sporulation data and arcsine-transformation of spore germination data were applied for statistical analysis. For the data of lesion diameters on onion bulbs subjected to thymol and other treatments, contrast analysis was used to compare the means before and after inoculation with the fungal isolates. General linear model procedures were used for the analysis of variance and the means were separated using the least significant difference test at p < 0.05.
3. Results
3.1. Ethanol concentration for thymol-amended media
In experiments 1 and 2, mycelial growth of A. awamori F23 and B. aclada F15 on PDA amended with various concentrations (0.01%, 0.1%, 0.2%, 0.5%, and 1% in the total medium volume) of ethanol slightly differed compared with that on ethanol-unamended control medium (0%; Supplementary Table S1). However, mycelial growth of A. awamori differed between PDA amended with 0.01 and 1% and ethanol-unamended control medium in experiment 2. Similarly, B. aclada also showed slight differences in mycelial growth between ethanol-amended PDA and ethanol-unamended control medium (Supplementary Table S1). Therefore, <0.5% ethanol of the total medium volume was considered appropriate as the solvent concentration for thymol and applied in further in vitro and in vivo tests on the antifungal effect of thymol.
3.2. In vitro antifungal effect of thymol on PDA
To test the in vitro antifungal effect of thymol, mycelial growth, sporulation, and spore germination of A. awamori F23 and B. aclada F15 were examined on the thymol- or GAA (positive control)-amended PDA (). Mycelial growth of these isolates was inhibited on media amended with various concentrations of thymol or acetic acid, as shown in dose-response curves in . On thymol-amended media, the EC50 values against A. awamori F23 were 2.2 and 2.3 (log10 value) mg L−1 in experiments 1 and 2, respectively. Meanwhile, on acetic acid-amended media, the EC50 values against the isolate were 6.0 and 8.8 (log10 value) µL L−1 in experiments 1 and 2, respectively. Notably, B. aclada F15 was effectively inhibited by both thymol and acetic acid, with the EC50 values of 1.4 and 1.2 (log10 value) mg L−1 for thymol and of 2.7 and 2.6 (log10 value) µL L−1 for acetic acid in experiments 1 and 2, respectively ().
Figure 1. Mycelial growth of (A) Aspergillus awamori F23 and (B) Botrytis aclada F15 on PDA amended with various concentrations of glacial acetic acid (GAA, positive control; 0, 1, 10, 100, 200, 500, and 1000 μL L−1) or thymol (0, 1, 10, 50, 100, 200, and 250 mg L−1). Spore suspensions (2 μL of 1 × 106 spores mL−1) of isolates F23 and F15 were drop-inoculated on potato dextrose agar (PDA) and mycelial growth (mm) was measured 6 and 7 days after incubation at 28 °C for A. awamori F23 and at 20 °C for B. aclada F15, respectively. A: GAA; T: thymol.
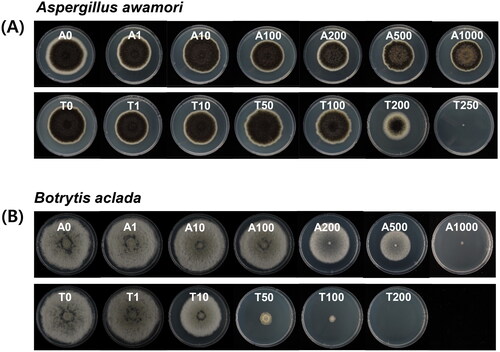
Figure 2. EC50 values and 95% fiducial limits for Aspergillus awamori F23 and Botrytis aclada F15 based on relative mycelial growth on PDA amended with various concentrations of (A) GAA (positive control; 0, 1, 10, 100, 200, 500, and 1000 μL L−1) or (B) thymol (0, 1, 10, 50, 100, 200, and 250 mg L−1). Spore suspensions (2 μL of 1 × 106 spores mL−1) of the isolates were drop-inoculated on PDA and then mycelial growth (mm) was measured 6 and 7 days after inoculation at 28 °C for A. awamori F23 and at 20 °C for B. aclada F15, respectively. The experiment was conducted with three replicates each.
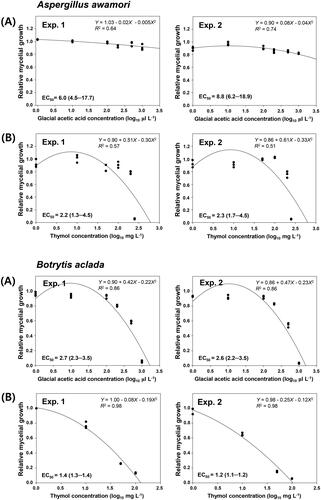
Figure 3. (A) Sporulation (log10 spores cm−2) and (B) spore germination (%) of Aspergillus awamori F23 and Botrytis aclada F15 on PDA amended with various concentrations of GAA (positive control) or thymol. Spore suspensions (2 μL of 1 × 106 spores mL−1) of the isolates were drop-inoculated on PDA, and sporulation was determined 6 and 7 days after inoculation on PDA amended with GAA or thymol at 28 °C for A. awamori F23 and at 20 °C for B. aclada F15, respectively. Spore germination was determined 18 h after incubation at the same temperatures. Bars represent mean ± standard deviation of three replicates. Values followed by the same letters are not significantly different (p < 0.05) according to the least significant difference test following arcsine transformation. *Uppercase and lowercase letters indicate significant differences between GAA and thymol for A. awamori F23 and B. aclada F15, respectively.
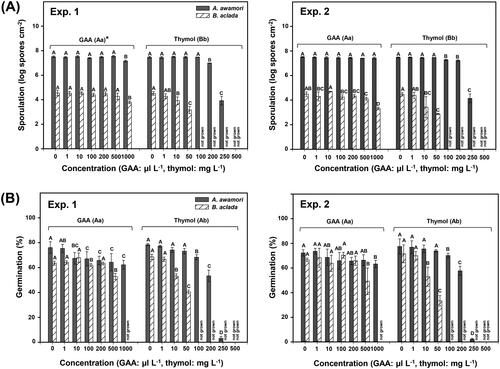
Furthermore, in A. awamori F23, thymol significantly (p < 0.05) inhibited sporulation at the concentrations of 200 and 250 mg L−1 () and spore germination at the concentrations of 100 to 250 mg L−1 () in experiments 1 and 2. Acetic acid as a positive control slightly affected sporulation of isolate F23 but reduced spore germination to some extent at the concentrations of 10 to 1000 µL L−1 in experiment 1 and of 1000 µL L−1 in experiment 2. Similarly, the sporulation and spore germination of B. aclada F15 were inhibited at 10 and 50 mg L−1 of thymol in both experiments (). In addition, acetic acid affected sporulation of isolate F15 at 1000 µL L−1 in experiment 1 and at 500 and 1000 µL L−1 in experiment 2. Furthermore, acetic acid affected the germination of isolate F15 only at 500 µL L−1 in repeated experiments (). Overall, thymol regardless of test concentrations more significantly (p < 0.05) inhibited the sporulation and spore germination of A. awamori F23 and B. aclada F15 than acetic acid ().
3.3. In vitro antifungal effect of volatile thymol in I-plates
Various concentrations of thymol or GAA in the gaseous form were applied in I-plates, and dose-response curves between the relative mycelial growth of A. awamori F23 and B. aclada F15 and volatile thymol or acetic acid were described ( and ). The EC50 value of volatile (gaseous form) thymol against A. awamori F23 was 3.0 (log10 value) mg L−1 in experiments 1 and 2. Meanwhile, the EC50 values of volatile acetic acid against isolate F23 were 7.0 and 6.3 (log10 value) µL L−1 in experiments 1 and 2, respectively (). Likewise, the EC50 values of volatile thymol against B. aclada F15 were 2.2 and 2.4 (log10 value) mg L−1 in experiments 1 and 2, respectively; however, the EC50 values of volatile acetic acid against isolate F15 were 5.5 and 5.3 (log10 value) µL L−1 in experiments 1 and 2, respectively ().
Figure 4. Effect of gaseous treatment at various concentrations of GAA (positive control; 0, 10, 100, 200, 500, and 1000 μL L−1) or thymol (0, 10, 100, 200, 500, and 1000 mg L−1) on the mycelial growth of (A) Aspergillus awamori F23 and (B) Botrytis aclada F15 on PDA in I-plates. Spore suspensions (2 μL of 1 × 106 spores mL−1) of the isolates were drop-inoculated on PDA and mycelial growth (mm) was measured 6 and 7 days after incubation at 28 °C for A. awamori F23 and at 20 °C for B. aclada F15, respectively. A: GAA; T: thymol.
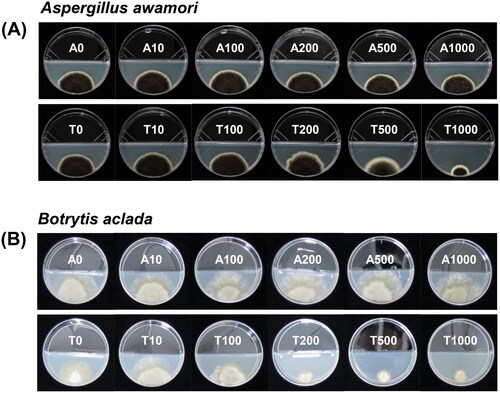
Figure 5. EC50 values and 95% fiducial limits for Aspergillus awamori F23 and Botrytis aclada F15 based on relative mycelial growth on PDA after gaseous treatment at various concentrations of (A) GAA (positive control; 0, 10, 100, 200, 500, and 1000 μL L−1) or (B) thymol (0, 10, 100, 200, 500, and 1000 mg L−1) in I-plates. Spore suspensions (2 μL of 1 × 106 spores mL−1) of the isolates were drop-inoculated on PDA and mycelial growth (mm) was measured 6 and 7 days after incubation at 28 °C for A. awamori F23 and at 20 °C for B. aclada F15, respectively.
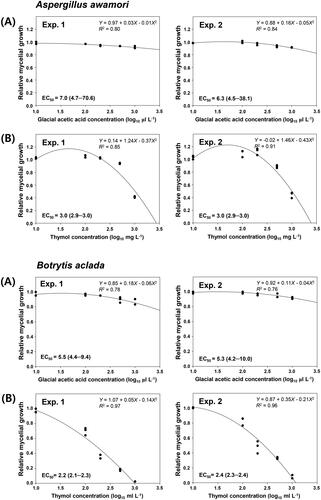
In A. awamori F23, volatile thymol significantly (p < 0.05) inhibited sporulation at 200 to 1000 mg L−1 () and spore germination at 500 and 1000 mg L−1 in at least one of two experiments (). However, volatile GAA slightly affected the sporulation of isolate F23 in repeated experiments, except at 1000 µL L−1 in experiment 2. Likewise, the spore germination of isolate F23 was somewhat inhibited at the tested concentrations in both experiments (). Similarly, the sporulation of B. aclada F15 was significantly (p < 0.05) reduced at 200 and 1000 mg L−1 of volatile thymol compared with untreated controls in experiments 1 and 2 (). However, the germination of isolate F15 was greatly reduced at 10 to 1000 mg L−1 in experiment 1 and at 1000 mg L−1 in experiment 2, compared with the untreated controls. In contrast, volatile (gaseous form) acetic acid did not significantly (p > 0.05) affect the sporulation and spore germination of isolate F15 at all tested concentrations in repeated experiments ().
Figure 6. (A) Sporulation (log10 spores cm−2) and (B) spore germination (%) of Aspergillus awamori F23 and Botrytis aclada F15 on PDA after gaseous treatment at various concentrations of (A) GAA (positive control; 0, 10, 100, 200, 500, and 1000 μL L−1) or (B) thymol (0, 10, 100, 200, 500, and 1000 mg L−1) in I-plates. Spore suspensions (2 μL of 1 × 106 spores mL−1) of the isolates were drop-inoculated on PDA, and sporulation was determined at 6 and 7 days after inoculation on PDA amended with GAA or thymol at 28 °C for A. awamori F23 and at 20 °C for B. aclada F15, respectively. Spore germination of the isolates was determined 18 h after incubation at the same temperature. Bars represent mean ± standard deviation of three replicates. Values followed by the same letters are not significantly different (p < 0.05) according to the least significant difference test following arcsine transformation. *Uppercase and lowercase letters indicate significant differences between GAA and thymol for A. awamori F23 and B. aclada F15, respectively.
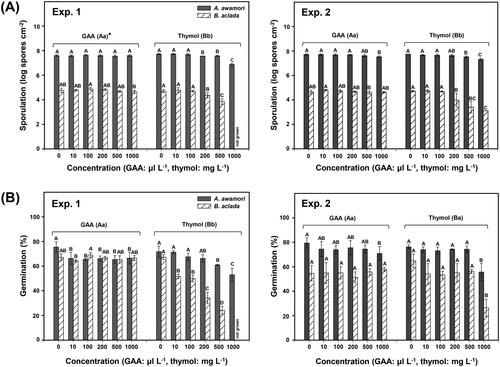
3.4. In vivo disease inhibitory effect of thymol on onion bulbs
To evaluate the disease inhibitory effect of thymol on onion, half-cut bulbs were treated with 1000 mg L−1 thymol 24 h before (i.e., protective efficacy) and after (i.e., curative efficacy of thymol) inoculation with A. awamori F23 and B. aclada F15 (). The bulbs sprayed with tymol 24 h before (pre-treatment) fungal inoculation produced greatly reduced lesion diameters by both isolates, compared with those sprayed with 0.5% ethanol (solvent control) in experiments 1 and 2. Further, GAA (2000 µL L−1) (positive control) and fluazinam (400 µL L−1) (fungicide control) significantly (p < 0.05) reduced lesion diameters in both experiments. Thymol showed comparable disease control efficacy against A. awamori F23 and B. aclada F15 to fluazinam, except against isolate F15 in experiment 1 ().
Table 1. Lesion diameters on the inner layers of onion bulbs sprayed with thymol, glacial acetic acid (GAA, positive control), ethanol (solvent control), and fluazinam (fungicide control) 24 h before and after inoculation with Aspergillus awamori F23 and Botrytis aclada F15.
Meanwhile, bulbs sprayed with thymol 24 h after (post-treatment of thymol) fungal inoculation showed significantly (p < 0.05) reduced lesion diameters caused by B. aclada F15 but not by A. awamori F23 compared with those sprayed with ethanol in experiments 1 and 2 (). As observed above, post-treatments with acetic acid (positive control) and fluazinam (fungicide control) significantly (p < 0.05) reduced lesion diameters caused by isolate F15 in both experiments and by isolate F23 in experiment 1 ().
Furthermore, bulbs sprayed with thymol 24 h before fungal inoculation (i.e., protective efficacy), produced significantly (p < 0.05) reduced lesion diameters caused by A. awamori F23 compared with those sprayed with thymol 24 h after inoculation (i.e., curative efficacy) in experiments 1 and 2 (). However, unlike that in A. awamori F23, in B. aclada F15, thymol treatment showed no significant (p > 0.05) effect on lesion diameter between 24 h before and after fungal inoculation in repeated experiments. Acetic acid significantly (p < 0.05) reduced lesion diameters by isolate F23 but not by isolate F15 when applied 24 h before inoculation compared with those when applied 24 h after inoculation in repeated experiments. Fluazinam treatment before fungal inoculation showed better disease inhibition against isolates F23 and F15 than treatment after inoculation in experiment 2. Regardless of the pre- or post-treatment, acetic acid and fluazinam produced overall potent inhibitory effects against diseases caused by B. aclada F15, as observed with thymol treatment ().
4. Discussion
In our previous study [Citation7], we reported that Aspergillus spp. (63.9%, occurrence frequency), Fusarium spp. (6.4%), Penicillium spp. (15.5%), Rhizopus spp. (5.2%), and others (9.0%) were the predominant fungal species of diseased onion bulbs originating from Muan, Korea, which were purchased at the Garak Market, Seoul, Korea. In that study, A. awamori, Fusarium oxysporum, Penicillium brasilianum, and Rhyzoctonia oryzae were the predominant fungi in onion bulbs. In another study [Citation2], B. aclada (44%) was the most commonly isolated fungal pathogen from onions at cold storage houses in Muan, Korea. Since these species are important postharvest fungal pathogens of onions in Korea, they should be controlled through appropriate measures under long-term storage conditions. Therefore, we attempted to establish an environmentally sound and effective control method and tested the antifungal activity of thymol against A. awamori, the causal agent of black mold rot, and B. aclada, the causal agent of gray mold rot, using in vitro amended media and I-plates and in vivo artificial inoculation trials. We demonstrated that thymol effectively inhibited the growth and development of A. awamori F23 and B. aclada F15 on amended medium and in I-plates. In addition, spraying thymol on onions efficiently controlled these postharvest fungal pathogens under cold storage conditions.
Essential oils from various host plants have been tested against several plant pathogens because plant-derived essential oils exhibit environmentally friendly control characteristics [Citation17,Citation18,Citation20,Citation35]. In a previous study, Rasooli et al. [Citation36] reported that essential oil extracts from Thymus eriocalyx and Thymus xporlock exhibited antifungal effects against A. niger. In addition, the mycelia of A. niger treated with essential oils exhibited irreversibly damaged cell walls and membranes and organelles in transmission electron microscopy. Similarly, Mossini et al. [Citation37] conducted in vitro trials to examine the effects of Azadirachta indica (neem) extracts against Penicillium verrucosum and Penicillium brevicompactum and observed significant reductions in mycelial growth and sporulation but no inhibition of ochratoxin A production by P. verrucosum and P. brevicompactum. Recently, De Melo et al. [Citation35] reported that essential oils of Lippia gracilis and Lippia sidoides exhibited significant, antifungal activity against Lasiodiplodia theobromae, the causal fungus of diplodia pod rot of cocoa.
Based on many studies on the antifungal effects of plant-derived essential oils, we tested various concentrations of thymol for antifungal activity against A. awamori F23 and B. aclada F15 in the present study. High concentrations of thymol as amendment in culture media or in gaseous form in I-plates significantly inhibited the mycelial growth, sporulation, and spore germination of both isolates. Overall, thymol produced a stronger inhibitory effect on the mycelial growth and development of the isolates than acetic acid (positive control). This antifungal activity of thymol may be attributed to its phenolic components that can disrupt fungal cell wall or membrane integrity as well as interfere with ergosterol biosynthesis [Citation38,Citation39]. In a previous study, Ahmad et al. [Citation38] reported fungistatic and fungicidal effects of thymol against 111 isolates of Candida and demonstrated that the mode of action of this compound is closely related to the mechanism described above. In another recent study, Ranjbar et al. [Citation40] assessed the antifungal effects of thymol against A. niger and Penicillium commune causing postharvest rot on pomegranate fruits. Scanning electron microscopy revealed cell deformation of A. niger, resulting from cell membrane destruction and cell wall strength loss. Interestingly, fungal growth was inhibited due to reduced cellulase and pectinase activities following thymol treatment [Citation40]. Furthermore, Chen et al. [Citation41] reported, for the first time, the anti-oomycete activity of a sulfonate derivative of thymol against Phytophthora capsici, the causal agent of Phytophthora blight of pepper. Therefore, thymol may serve as an environmentally sound alternative control measure against postharvest storage fungal pathogens on onions.
In the present study, we used acetic acid as the positive control against A. awamori F23 and B. aclada F15 because it has been reported to effectively control various storage fungi [Citation8,Citation42,Citation43]. In a previous study, Liu et al. [Citation42] reported that acetic acid vapors reduced the colony diameter and germination of Monilinia fructicola. However, we observed weak effect of acetic acid on the mycelial growth, sporulation, or spore germination of A. awamori F23 and B. aclada F15 when applied as the gaseous form in I-plates. Chu et al. [Citation8] have reported similar results in sweet cherries. They fumigated M. fructicola and Penicillium expansum-infected sweet cherries with acetic acid and noted that acetic acid effectively reduced blue mold rot but not brown rot. Thus, acetic acid treatment in the gaseous form may be not greatly effective against certain fungal pathogens.
In the case of postharvest diseases, primary inoculum is typically already present, as derived through exposure to contaminated soil, transported vehicle, storage house atmosphere or wounds during transportation and packaging [Citation44–46]. El-Mougy et al. [Citation47] treated garlic cloves with essential oils before storage, which produce desirable protective effects against black mold rot. Raju and Naik [Citation48] sprayed onion bulbs with various systemic and non-systemic fungicides before storage and observed protective effects, as evidenced by reduced mycelial growth and disease incidence of A. niger. In the present study, spraying onion bulbs with 1000 mg L−1 thymol 24 h before and after fungal inoculation greatly reduced lesion diameters compared with spraying 0.5% ethanol (solvent control). Onion bulbs sprayed with thymol 24 h before fungal inoculation (i.e., protective efficacy) generally showed reduced lesion diameters by both A. awamori F23 and B. aclada F15. However, post-treatment of the pathogen-inoculated onion bulbs with thymol only provided a curative effect against B. aclada F15, but not against A. awamori F23. Here, the reason why thymol failed to show a curative effect against A. awamori F23 might be the rapid penetration of the fungal isolate into onion tissues after inoculation.
Taken together, thymol, a plant-derived essential oil, can effectively inhibit the mycelial growth and development of A. awamori, the causal agent of black mold rot, and of B. aclada, the causal agent of gray mold rot, on in vitro amended media and I-plates as well as in in vivo artificially inoculated onion bulbs. In particular, spraying or fumigating thymol on onion bulbs can efficiently control these postharvest fungal pathogens. Additional studies on the gaseous treatment of thymol in onions to control various other fungal pathogens under long-term storage conditions are warranted.
Supplemental Material
Download MS Word (58.5 KB)Disclosure statement
No potential conflict of interest was reported by the authors.
Additional information
Funding
References
- McDonald MR, Jaime M, Hovius MHY. Management of diseases of onions and garlic. In: Naqvi SAMH, editor. Diseases of fruits and vegetables: diagnosis and management, Vol II. Dordrecht, Netherlands: Kluwer Academic Publishers; 2004. p. 149–200.
- Ji SH, Kim TK, Keum YS, et al. The major postharvest disease of onion and its control with thymol fumigation during low-temperature storage. Mycobiology. 2018;46(3):242–253.
- Lee JT, Bae DW, Park SH, et al. Occurrence and biological control of post-harvest decay in onion caused by fungi. Plant Pathol J. 2001;17:141–148.
- Oh JY, Kim KD. Control strategies for pathogenic fungi on stored onion (Allium cepa) and garlic (Allium sativum): a review. Life Sci Nat Resour Res. 2016;24:31–40.
- Oh JY, Han GD, Jeong JJ, et al. First report of Penicillium georgiense as a fungal pathogen of onion (Allium cepa L.). Crop Prot. 2015;72:83–89.
- Özer N, Köycü DN. Seed-borne fungal diseases of onion, and their control. In: Mukerji KG, editor. Disease management of fruits and vegetables: fruit and vegetable diseases, Vol. I. Dordrecht, Netherlands: Kluwer Academic Publishers; 2004. p. 281–306.
- Sang MK, Han GD, Oh JY, et al. Penicillium brasilianum as a novel pathogen of onion (Allium cepa L.) and other fungi predominant on market onion in Korea. Crop Prot. 2014;65:138–142.
- Chu CL, Liu WT, Zhou T. Fumigation of sweet cherries with thymol and acetic acid to reduce postharvest brown rot and blue mold rot. Fruits. 2001;56(2):123–130.
- Mari M, Bautista-Baños S, Sivakumar D. Decay control in the postharvest system: role of microbial and plant volatile organic compounds. Postharvest Biol Technol. 2016;122:70–81.
- Romanazzi G, Lichter A, Gabler FM, et al. Recent advances on the use of natural and safe alternatives to conventional methods to control postharvest gray mold of table grapes. Postharvest Biol Technol. 2012;63(1):141–147.
- Tripathi P, Dubey NK. Exploitation of natural products as an alternative strategy to control postharvest fungal rotting of fruit and vegetables. Postharvest Biol Technol. 2004;32(3):235–245.
- Droby S, Wisniewski M, Macarisin D, et al. Twenty years of postharvest biocontrol research: is it time for a new paradigm? Postharvest Biol Technol. 2009;52(2):137–145.
- Burt S. Essential oils: their antibacterial properties and potential applications in foods—a review. Int J Food Microbiol. 2004;94(3):223–253.
- Negi PS. Plant extracts for the control of bacterial growth: efficacy, stability and safety issues for food application. Int J Food Microbiol. 2012;156(1):7–17.
- Schillinger U, Geisen R, Holzapfel WH. Potential of antagonistic microorganisms and bacteriocins for the biological preservation of foods. Trends Food Sci Technol. 1996;7(5):158–164.
- Isman MB. Plant essential oils for pest and disease management. Crop Prot. 2000;19(8-10):603–608.
- Antunes MDC, Cavaco AM. The use of essential oils for postharvest decay control. A review. Flavour Fragr. J. 2010;25(5):351–366.
- Sivakumar D, Bautista-Baños S. A review on the use of essential oils for postharvest decay control and maintenance of fruit quality during storage. Crop Prot. 2014;64:27–37.
- Garcia R, Alves ES, Santos MP, et al. Antimicrobial activity and potential use of monoterpenes as tropical fruits preservatives. Braz J Microbiol. 2008;39(1):163–168.
- Kumar P, Mishra S, Malik A, et al. Biocontrol potential of essential oil monoterpenes against housefly, Musca domestica (Diptera: Muscidae). Ecotoxicol Environ Saf. 2014;100:1–6.
- Sartoratto A, Machado ALM, Delarmelina C, et al. Composition and antimicrobial activity of essential oils from aromatic plants used in Brazil. Braz J Microbiol. 2004;35(4):275–280.
- Maqbool M, Ali A, Alderson PG. Effect of cinnamon oil on incidence of anthracnose disease and postharvest quality of bananas during storage. Int J Agric Biol. 2010;12:516–520.
- Pérez-Alfonso CO, Martínez-Romero D, Zapata PJ, et al. The effects of essential oils carvacrol and thymol on growth of Penicillium digitatum and P. italicum involved in lemon decay. Int J Food Microbiol. 2012;158(2):101–106.
- Sellamuthu PS, Mafune M, Sivakumar D, et al. Thyme oil vapour and modified atmosphere packaging reduce anthracnose incidence and maintain fruit quality in avocado. J Sci Food Agric. 2013;93(12):3024–3031.
- Arrebola E, Sivakumar D, Bacigalupo R, et al. Combined application of antagonist Bacillus amyloliquefaciens and essential oils for the control of peach postharvest diseases. Crop Prot. 2010;29(4):369–377.
- Vitoratos A, Bilalis D, Karkanis A, et al. Antifungal activity of plant essential oils against Botrytis cinerea, Penicillium italicum and Penicillium digitatum. Not Bot Hort Agrobot Cluj. 2013;41(1):86.
- Chillet M, Minier J, Hoarau M, et al. Potential use of thymol to control anthracnose development in mango. Eur J Plant Pathol. 2019;155(3):943–952.
- Hudaib M, Speroni E, Di Pietra AM, et al. GC/MS evaluation of thyme (Thymus vulgaris L.) oil composition and variations during the vegetative cycle. J Pharm Biomed Anal. 2002;29(4):691–700.
- Meeran MFN, Javed H, Taee HA, et al. Pharmacological properties and molecular mechanisms of thymol: prospects for its therapeutic potential and pharmaceutical development. Front Pharmacol. 2017;8:380. Article 380.
- Nickavar B, Mojab F, Dolat-Abadi R. Analysis of the essential oils of two Thymus species from Iran. Food Chem. 2005;90(4):609–611.
- Hassani A, Fathi Z, Ghosta Y, et al. Evaluation of plant essential oils for control of postharvest brown and gray mold rots on apricot. J Food Saf. 2012;32(1):94–101.
- Kong J, Xie Y, Yu H, et al. Synergistic antifungal mechanism of thymol and salicylic acid on Fusarium solani. Food Sci Technol. 2021;140:110787.
- Xu L, Song J-Q, Wang Y-L, et al. Thymol improves salinity tolerance of tobacco by increasing the sodium ion efflux and enhancing the content of nitric oxide and glutathione. BMC Plant Biol. 2022;22(1):31.
- Ji P, Momol MT, Olson SM, et al. Evaluation of thymol as biofumigant for control of bacterial wilt of tomato under field conditions. Plant Dis. 2005;89(5):497–500.
- De Melo JO, Blank AF, Nunes RDS, et al. Essential oils of Lippia gracilis and Lippia sidoides chemotypes and their major compounds carvacrol and thymol: nanoemulsions and antifungal activity against Lasiodiplodia theobromae. Res Soc Dev. 2022;11(3):e36511326715.
- Rasooli I, Rezaei MB, Allameh A. Growth inhibition and morphological alterations of Aspergillus Niger by essential oils from Thymus eriocalyx and Thymus x-porlock. Food Cont. 2006;17(5):359–364.
- Mossini SAG, Arrotéia CC, Kemmelmeier C. Effect of neem leaf extract and neem oil on Penicillium growth, sporulation, morphology and ochratoxin a production. Toxins (Basel). 2009;1(1):3–13.
- Ahmad A, Khan A, Akhtar F, et al. Fungicidal activity of thymol and carvacrol by disrupting ergosterol biosynthesis and membrane integrity against Candida. Eur J Clin Microbiol Infect Dis. 2011;30(1):41–50.
- Shcherbakova L, Mikirtyuk O, Arslanova L, et al. Studying the ability of thymol to improve fungicidal effects of tebuconazole and difenoconazole against some plant pathogenic fungi in seed or foliar treatments. Front Microbiol. 2021;12:629429.
- Ranjbar A, Ramezanian A, Shekarforoush S, et al. Antifungal activity of thymol against the main fungai causing pomegranate fruit rot by suppressing the activity of cell wall degrading enzymes. Food Sci Technol. 2022;161:113303.
- Chen G-Q, Sun D, Yang J-M, et al. Synthesis of sulfonate derivatives of carvacrol and thymol as anti-oomycetes agents. J Asian Nat Prod Res. 2021;23(7):692–702.
- Liu WT, Chu CL, Zhou T. Thymol and acetic acid vapors reduce postharvest brown rot of apricots and plums. Hort Sci. 2002;37(1):151–156.
- Peláez AL, Cataño CS, Yepes EQ, et al. Inhibitory activity of lactic and acetic acid on Aspergillus flavus growth for food preservation. Food Cont. 2012;24:177–183.
- Cho J, Bae RN, Lee SK. Current research status of post-harvest technology of onion (Allium cepa L.). Korean J Hort Sci Tech. 2010;28:522–527.
- Kim YK, Lee SB, Lee SS, et al. Cultural and chemical approaches for controlling postharvest diseases of garlics. Korean J Pest Sci. 2003;7:139–148.
- Tyson JL, Fullerton RA. Effect of soil-borne inoculum on incidence of onion black mould (Aspergillus niger). New Zealand Plant Prot. 2004;57:138–141.
- El-Mougy NS, El-Gamal NG, Abdel-Kader MM. Pre-storage application of some essential oils and food preservatives against black mould incidence of garlic cloves during storage. Arch Phytopathol Plant Prot. 2009;42(11):1059–1068.
- Raju K, Naik MK. Effect of pre-harvest spray of fungicides and botanicals on storage diseases of onion. Indian Phytopathol. 2006;59:133–141.