Abstract
In the present study, a thermophilic anaerobic bacterial consortium YTY-70 that produced thermostable β-galactosidase was isolated from a hot spring in Yongtai (Fujian, China). Based on 16S rRNA sequences, we concluded that Caldicellulosiruptor was the predominant genus in the studied bacterial consortium. Subsequently, a thermostable β-galactosidase gene was cloned and expressed in Escherichia coli as a fusion protein with glutathione S-transferase. The recombinant enzyme exhibited a maximum activity at an optimum pH of 7.0 and an optimum temperature of 75 °C. It had a high activity across a broad pH range (between pH 5.0 and pH 9.0) and high thermal stability at temperatures up to 90 °C. The activity of the recombinant β-galactosidase was enhanced by TritonX-100, Tween-20 and 1 mmol/L 2-mercaptoethanol, while it was inhibited by ethylenediaminetetraacetic acid, sodium dodecyl sulphate, phenylmethylsulphonyl fluoride and 10 mmol/L 2-Me. The enzyme's catalytic function was enhanced by the following metal ions: Na+, K+, Mg2+, Ba2+, Ca2+, Fe2+, Zn2+, Mn2+, Al3+, Cu2+, Fe3+ and Li+. The thermostable β-galactosidase might be a promising candidate for use in food industries, particularly for the dairy industry and biosynthesis of galacto-oligosaccharides, due to its high thermostability and broad pH range.
Introduction
β-Galactosidase (β-D-galactohydrolase, EC3.2.1.23), also known by the trade name lactase,[Citation1] catalyses both hydrolysis and transgalactosylation reactions.[Citation2–4] It is widely distributed in microorganisms, plants and animal tissues.[Citation5,Citation6] Commercialized β-galactosidase mainly derives from Escherichia coli, Aspergillus niger, Aspergillus oryzae, yeasts, some species from the Lactobacillus genus and others.[Citation7] β-Galactosidases from microorganisms have attracted considerable attention because of their use in biotechnological applications. As it can hydrolyse lactose into glucose and galactose in nature, this enzyme has potential application in food processing industry, especially in dairy industry. Approximately 80% of Asian people suffer from lactose intolerance, because they have low levels of intestinal β-galactosidase.[Citation8] This enzyme can also be used in the biosynthesis of galacto-oligosaccharides (GOSs). GOSs are widely recognized as non-digestible oligosaccharides and can promote the growth of beneficial intestinal bacteria.[Citation8,Citation9] Hence, more and more reports have been published on the characterizations of microbial β-galactosidases.[Citation10,Citation11]
β-Galactosidase can be categorized as either an intracellular or an extracellular enzyme based on its origin. For example, those derived from lactic yeast, Aspergillus niger, Aspergillus oryzae and Rhizopus oryzae, are extracellular enzymes, whereas those derived from Kluyveromyces fragilis and some bacteria, which exist in humans, are considered as intracellular enzymes.[Citation12] The origin of β- galactosidase can cause great differences in its properties.[Citation13]
Generally speaking, most industrial enzymes have limited application in the dairy industry due to low reaction temperatures and poor thermal stability. In contrast, a heat-resistant β-galactosidase has a good thermal stability and is active at higher temperatures. Thereby, it can be used to enhance the concentration of the substrate and reduce the chance for production of other microorganisms. β-Galactosidases of microbial origin possess a wide range of optimal reaction temperatures. They range from approximately 35 °C for β-galactosidases, derived from yeasts, to 50–60 °C for mould-derived β-galactosidases, and from 65 to 80 °C for those derived from Bacillus genus and Thermus aquaticus, respectively.[Citation14]
In the present study, a thermophilic anaerobic bacterial consortium YTY-70 that produced thermostable β-galactosidase was isolated from a hot spring in Yongtai (Fujian, China). Using a metagenomic strategy, a 16S rDNA gene library was constructed in order to characterize the bacterial diversity of YTY-70. The Caldicellulosiruptor genus, which could produce thermostable β-galactosidase, was demonstrated to be the predominant genus in the YTY-70. The β-galactosidase gene was, therefore, cloned and the recombinant β-galactosidase was further characterized.
Materials and methods
Isolation and identification of the thermophilic anaerobic bacterial consortium YTY-70
A water sample (approximately 100 mL) was collected from a hot spring in Yongtai at a depth of 3 m and was sealed in an anaerobic jar. The water sample was cultured at 70 °C for three days in medium, which consisted of the following ingredients: 0.75 g/L KH2PO4; 1.5 g/L K2HPO4; 0.9 g/L NH4Cl; 0.4 g/L MgCl2·6H2O; 0.9 g/L NaCl; 0.6 g/L yeast extract; 2.0 g/L tryptone; 0.5 g/L L-cystein; 10 μL 10% FeSO4 and 9 mL trace mineral solution. The trace mineral solution contained per litre of distilled water: 1.50 g nitrilotriacetic acid; 3.00 g MgSO4·7H2O; 0.50 g MnSO4·2H2O; 1.00 g NaCl; 0.10 g FeSO4·7H2O; 0.10 g CoCl2; 0.10 g CaCl2·2H2O; 0.10 g ZnSO4; 0.01 g CuSO4·5H2O; 0.01 g AlK(SO4)2; 0.01 g H3BO3; 0.01 g Na2MoO4·2H2O. Screening of the thermophilic anaerobic bacterial consortium that produced the thermostable β-galactosidase was conducted at pH 7.0. The bacterial consortium went through three rounds of growth and purification in the liquid culture medium mentioned above.
The genomic DNA of the bacterial consortium was extracted by using the Bacterial Genomic DNA Extraction Kit (Sangon, China) according to the manufacturer's instructions. The conserved sequence was amplified from the genomic DNA of the bacterial consortium by polymerase chain reaction (PCR), using primers 27F (5′-AGAGTTTGATCCTGGCTCAG-3′) and 1492R (5′-GGTTACCTTGTTACGACTT-3′).[Citation15] The PCR conditions were as follows: 95 °C for 3 min, 30 cycles at 94 °C for 1 min, 55 °C for 1 min and 72 °C for 1.5 min, followed by 1 cycle at 72 °C for 10 min. Homology was analysed in GenBank with the BLAST programme. The 16S rRNA gene was cloned into pMD-18T vector (Takara, China) and sequenced at the Shanghai Sangon Biological Engineering Technology & Service Company (China).
Cloning of the gene encoding β-galactosidase
By analysing the available published sequences of β-galactosidase genes (GenBank accession numbers CP002219.1, CP001393.1, CP000679.1 and CP002164.1), the following degenerate primers were synthesized: sense primer GlaF [5′-ATGAGTTTT(A/C)CCAAAAGGATT-3′] and antisense primer GlaR [5′-TTAC(T)GA G(A)TTTTCCTTTATAT-3′]. The β-galactosidase gene was amplified from the genomic DNA of the bacterial consortium YTY-70 by PCR using the degenerate primers. The PCR conditions were as follows: 95 °C for 5 min, 30 cycles at 95 °C for 1 min, 57 °C for 1 min and 72 °C for 2 min, followed by 1 cycle at 72 °C for 10 min. The PCR products were purified from agarose gels. The purified DNA fragment was cloned into the pMD-18T vector and sequenced by the Shanghai Sangon Biological Engineering Technology & Service Company (Sangon).
Gene expression and purification of GST-β-galactosidase fusion protein
The β-galactosidase gene was amplified using the forward primer GlaF-BamHI, 5′-CGCGGATCCATGAGTTTTCCAAAAGGATT-3′ and the reverse primer GlaR-XhoI, 5′-CCGCTCGAGTTATGAGTTTTCCTTTATAT-3′, with the underlined nucleotides representing the BamHI and XhoI sites, respectively. The PCR conditions were as follows: 95 °C for 5 min; 30 cycles at 95 °C for 1 min, 57 °C for 1 min and 72 °C for 2 min; followed by one cycle at 72 °C for 10 min. The amplified DNA fragment was purified from an agarose gel and digested with BamHI and XhoI, then ligated into the BamHI/XhoI sites of the pGEX-4T-2 vector (Amersham, Sweden) downstream of glutathione S-transferase (GST) gene. The recombinant plasmid containing the thermostable β-galactosidase gene was confirmed by sequencing.
To express the β-galactosidase gene, the bacterial transformant was first grown overnight in Luria Broth (LB) at 37 °C. Then, the bacteria containing the β-galactosidase gene and the control (bacteria, containing only vector) were inoculated into a new media at a ratio of 1:100. When an optical density of 0.6 at 600 nm was reached, the bacteria were induced with 0.5 mmol/L isopropyl-β-D-thiogalactopyranoside (IPTG) (Bio Basic Inc., BBI, Canada) and grown for additional 4 hours at 37 °C. The whole-cell protein of the induced and non-induced bacteria was analysed by sodium dodecyl sulphate–polyacrylamide gel electrophoresis (SDS–PAGE). The GST-β-galactosidase fusion protein was purified by the usage of Glutathione Sepharose 4B (GE Healthcare) following the manufacturer's instructions. The cells were harvested and resuspended in 50 mL of phosphate-buffered saline (PBS), which contained 140 mmol/L NaCl, 2.7 mmol/L KCl, 10 mmol/L Na2HPO4, 1.8 mmol/L KH2PO4, pH 7.3 and then lysed by sonication. The lysis was performed by adding Triton X-100 to a final concentration of 1% (w/v) and mixing gently for 30 min. Insoluble particles were removed by centrifugation at 14,000 × g for 30 min at 4 °C. A 5-mL suspension of Glutathione Sepharose 4B was then added to the supernatant and incubated for 1 hour at a room temperature (22–25 °C). After incubation, the Glutathione Sepharose 4B was packed in a mini-column and washed with 100 mL of PBS, and the GST-β-galactosidase fusion protein was eluted with 5 mL of elution buffer (50 mmol/L Tris-HCl, 10 mmol/L reduced glutathione, pH 8.0). The protein concentration was determined by the Bradford method [Citation16] using bovine serum albumin (Sigma) as a standard protein. The purity of the fusion protein was confirmed by SDS–PAGE.
Assay of β-galactosidase activity
The procedure for determining the β-galactosidase activity followed the description in the Food Chemicals Codex–National Academy of Sciences, with o-nitrophenol-β-D-galactopyranoside (ONPG) (8.3 × 10−3 mol/L) dissolved in 0.05 mol/L sodium phosphate buffer (pH 7.0), used as a substrate. The purified fusion protein was diluted 100 × 0.4 mL of the enzyme dilution was added to 1.6 mL of substrate solution and incubated at 75 °C for 10 min. The assay was stopped by the addition of 2 mL of sodium carbonate (1 mol/L), and the absorbance was determined at 420 nm. One unit of enzyme activity was defined as the quantity of enzyme that would liberate 1 mmol/L of o-nitrophenol from ONPG per minute under the assay conditions.[Citation17] Specific activity was expressed as U/mg of protein.
Characterization of recombinant β-galactosidase
The temperature effect on the activity of β-galactosidase was evaluated in a 0.05 mol/L sodium phosphate buffer (pH 7.0) at various temperatures (35, 45, 55, 65, 75, 85 and 95 °C). To evaluate the effect of temperature on the thermostability of β-galactosidase, the enzyme solution was preincubated in 0.05 mol/L sodium phosphate buffer (pH 7.0) at 75, 80, 85, 90 and 95 °C for 30 min. Subsequently, the residual enzymatic activity was determined using a β-galactosidase activity assay. The assayed activity under the conditions of 75 °C and pH 7.0 was defined as 100% relative activity.
The pH effect on the activity of the enzyme was evaluated at 75 °C in buffer solutions with various pH values, ranging from 4.0 to 10.0. The following buffers (100 mmol/L) were used: phosphate-citrate buffer (pH 4.0–6.0), phosphate buffer (pH 6.0–8.0) and glycine–sodium hydroxide buffer (pH 8.0–10.0). To evaluate the effect of pH on the stability of β-galactosidase, the recombinant β-galactosidase was incubated in different pH buffers (pH 4.0–10.0) at 75 °C for 30 min. The stability of the enzyme was assessed with an enzyme activity assay. The assayed activity under the conditions of 75 °C and pH 7.0 was defined as 100% relative activity.
To study the effect of various metal ions, additives and detergents on the activity of β-galactosidase, the enzyme samples were assayed with ONPG in 0.05 mol/L sodium phosphate buffer (pH 7.0) at 75 °C with 1 mmol/L or 10 mmol/L of the following metal ion sources: NaCl, KCl, MgCl2, BaCl2, CaCl2, FeSO4, ZnSO4, MnCl2, AlCl3, CuCl2, FeCl3 and LiCl. The used additives and detergents were 1 mmol/L or 10 mmol/L SDS, 1 mmol/L or 10 mmol/L EDTA, 1 mmol/L or 10 mmol/L PMSF, also known as phenylmethanesulfonyl fluoride, TritonX-100, Tween-20 or 2-mercaptoethanol (2-Me), all with final concentrations of 0.1% or 1% (w/v).[Citation18] The measured activity was compared with the activity of the enzyme solution without the added metal ions, additives and detergents under the same incubation conditions. The assayed activity under the conditions of 75 °C and pH 7.0 without the added metal ions, additives and detergents was defined as 100% relative activity.
Results and discussion
Cloning of β-galactosidase genes from bacterial consortium YTY-70 and nucleotide sequence analysis
The thermophilic anaerobic bacterial consortium YTY-70, which was isolated from hot spring, could grow within a temperature range of 50–85 °C with an optimum temperature of 75 °C. On the basis of 16S rRNA sequence analysis, we concluded that the vast majority of bacteria in YTY-70 belonged to Caldicellulosiruptor genus (data not shown). The thermostable enzymes that are being used industrially are mainly isolated from microorganisms and thermophiles, which are the most important sources. Many thermostable enzymes have been obtained from thermophilic bacteria and have been used in various industries like chemical, food, pharmaceutical, paper, textile and others.[Citation19–21] Although thermophilic bacteria can be good candidates for the development of thermostable β-galactosidase, most native thermostable enzymes give very low yield and are therefore difficult to purify.[Citation22]
A 1359 bp fragment was obtained from the genomic DNA of the bacterial consortium YTY-70 by using degenerate PCR primers, designed from the conserved regions of various thermostable β-galactosidase sequences. This fragment contained an open reading frame (ORF) encoding 452 amino acids (GenBank accession number JX531650). The results of the GenBank BLAST analysis indicated that the deduced amino acid sequence was homologous to a known β-galactosidase and that the ORF was most likely the β-galactosidase gene of Caldicellulosiruptor genus. The amino acid sequence of Caldicellulosiruptor β-galactosidase shared 96%, 95%, 93%, 54% and 53% identities with the thermostable β-galactosidase from Caldicellulosiruptor hydrothermalis 108, Caldicellulosiruptor kristjanssonii 177R1B, Caldicellulosiruptor obsidiansis OB47, Dictyoglomus thermophilum H-6-12 and Clostridium cellulovorans 743B, respectively ().
Figure 1. Multiple alignments of representative β-galactosidases from thermophiles.
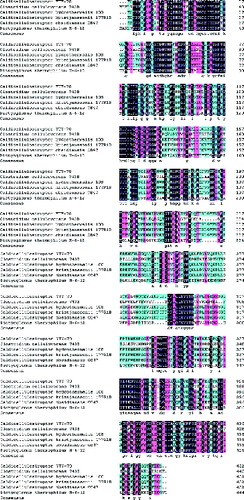
Expression and purification of recombinant β-galactosidase
The pGEX-4T-2 expression system was used for the expression of the recombinant β-galactosidase. The ORF was expressed as a GST fusion protein in E. coli. To express the β-galactosidase-encoding gene, the bacterial transformant was induced with IPTG. The total protein from the induced and non-induced bacterial transformant containing the β-galactosidase-encoding gene and from the induced and non-induced control bacteria (containing only vector) were analysed by SDS–PAGE. A band at approximately 70 kDa, which corresponded to the GST-β-galactosidase fusion protein, was observed in the induced bacterial transformant (, lane 4). No protein was observed at that position in the induced and non-induced controls. After purification with Glutathione Sepharose 4B, 5 mL of the fusion protein was collected. Determined by the Bradford method,[Citation16] the result showed that the protein concentration was 0.438 mg/mL. The use of recombinant technologies is an effective way to reduce production costs and to increase the yield of these processes. In recent decades, many types of thermostable enzymes were cloned from thermophilic bacteria and expressed at high levels in mesophilic hosts, such as E. coli,[Citation23] Pichia pastoris,[Citation24] Kluyveromyces lactis [Citation25] and Bacillus subtilis.[Citation26]
Figure 2. Purification of the recombinant β-galactosidase.
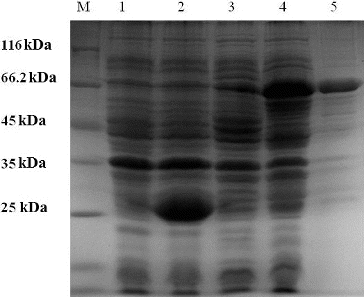
Characterization of the recombinant β-galactosidase
ONPG was used as a substrate for the recombinant β-galactosidase to evaluate the enzyme's activity. The recombinant enzyme exhibited a maximum activity at an optimum temperature of 75 °C ((A)). The specific activity at the optimal conditions was 260 U/mg (taken to be 100%). The optimum temperature was found to be similar to several thermostable β-galactosidases from Thermus sp. (70 °C),[Citation27] Sulfolobus solfataricus (75 °C) [Citation28] and Thermotoga maritima (80 °C to 85 °C).[Citation29,Citation30] Moreover, the recombinant enzyme had a high thermal stability at temperatures up to 90 °C ((B)). The activity level of β-galactosidases, produced from Caldicellulosiruptor, was greater than that of the majority of enzymes produced from other sources.The activity of β-galactosidase from K. lactis lost half of its maximum value for 17.5 min at 30 °C and for less than 1 min at temperatures above 45 °C.[Citation31] In another study, β-galactosidase, produced from K. lactis decreased its catalytic activity by 73% at 60 °C.[Citation32] β-Galactosidase from Aspergillus alliaceus retained 82% of its enzyme activity at 70 °C, compared to the maximum value.[Citation33] Compared to other thermostable β-galactosidases, this recombinant β-galactosidase showed considerable thermal stability over a broad temperature range (between 50 and 90 °C). As we know, there are many benefits when reactions are catalysed at elevated temperatures, such as improved reaction kinetics and increased substrate solubility.[Citation8]
Figure 3. Effects of temperature on recombinant β-galactosidase activity (A) and stability (B).
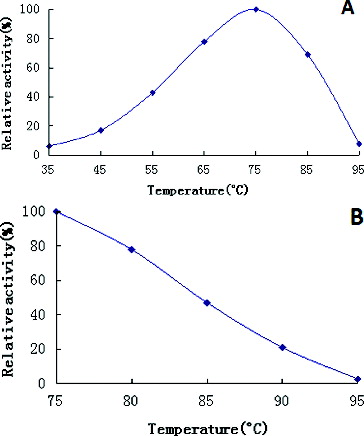
The effects of pH on enzymatic activity were investigated at a pH range from pH 4.0 to pH 10.0. The recombinant β-galactosidase achieved a maximum activity at pH 7.0 in 100 mmol/L phosphate buffer, with a good activity across a broad pH range – from pH 5.0 to pH 9.0 ((A)). Furthermore, the enzyme displayed more than 54% of its maximum activity after incubating for 30 min in the pH range of 5.0–8.0 ((B)). In addition, the activity of this enzyme was almost unchanged after incubation for 30 min in pH 7.0 ((B)). The optimum pH for β-galactosidase activity is also a very important consideration for industrial applications. The thermostable β-galactosidase, isolated during this study, displayed a maximum activity at pH 7.0, which would be advantageous for applications in the dairy industry, having in mind that the pH of milk is 6.8.[Citation34]
Figure 4. Effects of pH on β-galactosidase activity (A) and stability (B).
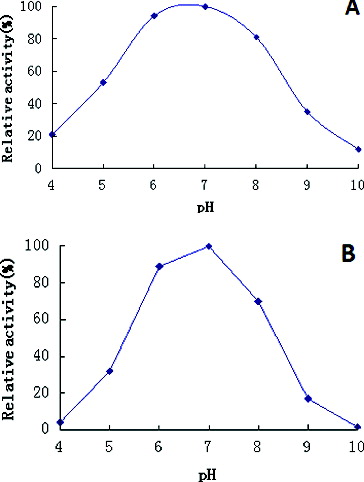
The results from the activity assay, following exposure to metal ions, indicated that the catalytic function of the enzyme was promoted with the presence of Na+, K+, Mg2+, Ba2+, Ca2+, Fe2+, Zn2+, Mn2+, Al3+, Cu2+, Fe3+ and Li+ ((A)). The effects of various cations on the enzyme activity were consistent with earlier reports.[Citation35–37] However, metal ions, such as Ca2+, Zn2+, Mn2+ and Cu2+, have also been reported to decrease the activity of thermostable β-galactosidase from Thermotoga naphthophila and Lactobacillus delbrueckii.[Citation8,Citation38] In some cases, the addition of metal ions have no effect on the activity of thermostable β-galactosidase. Such examples are the results obtained from Bacillus coagulans [Citation39] and S.solfataricus.[Citation28] The thermostable β-galactosidase also proved to be resistant to additives and detergents. As demonstrated in (B), the recombinant β-galactosidase had a good activity when SDS (1 mmol/L or 10 mmol/L), EDTA (1 mmol/L or 10 mmol/L), PMSF (1 mmol/L or 10 mmol/L), TritonX-100 (0.1%, w/v or 1%, w/v), Tween 20 (0.1%, w/v or 1%, w/v) and 2-Me (1 mmol/L or 10 mmol/L) were used. The enzymatic activity was enhanced by TritonX-100, Tween-20 and 1 mmol/L 2-Me, but it was inhibited by EDTA, SDS, PMSF and 10 mmol/L 2-Me.
Figure 5. Effects of metal ions (A) and additives, and detergents (B) on β-galactosidase activity.
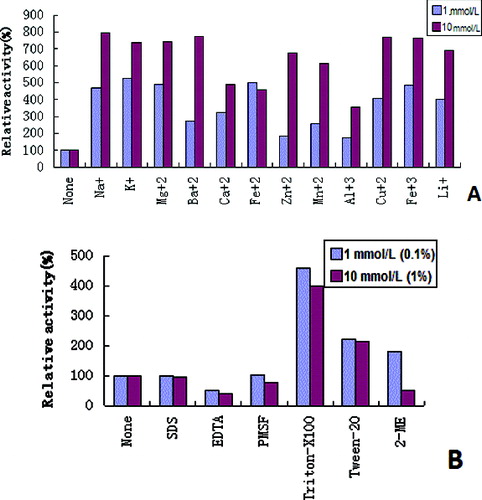
Conclusion
The obtained results from this study demonstrated that the recombinant thermostable β-galactosidase expressed in a mesophilic host was stable (i.e., kept its correct folding structure) and was suitable for commercial applications due to its high thermostability and broad pH range. The recombinant enzyme exhibited maximum activity at an optimum pH of 7.0 and an optimum temperature of 75 °C. The enzyme had a considerable stability over a broad temperature range (between 50 and 90 °C) and a broad pH range (between pH 5.0 and 9.0). The activity of this thermostable β-galactosidase can be significantly promoted with all of the metal ions, which participated in the assay and the enzyme was proved to be resistant to additives and detergents. The excellent characteristics of this enzyme suggest that it may have potential applications in food processing industry, especially in the dairy industry and biosynthesis of GOSs. Furthermore, our research also implies that hot spring thermophiles are very important sources of thermostable enzymes.
Disclosure statement
No potential conflict of interest was reported by the authors.
Additional information
Funding
References
- Reuter S, Nygaard AR, Zimmermann W. β-Galacto-oligosacharide synthesis with β-galactosidases from Sulfolobus solfataricus, Aspergillus oryzae, and Escherichia coli. Enzyme Microb Technol. 1999;25:509–516.
- Kim YS, Park CS, Oh DK. Lactulose production from lactose and fructose by a thermostable-galactosidase from Sulfolobus solfataricus. Enzyme Microb Technol. 2006;39:903–908.
- Gaur R, Pant H, Jain R, Khare SK. Galacto-oligosaccharide synthesis by immobilized Aspergillus oryzae β-galactosidase. Food Chem. 2006;97(3):426–430.
- Martínez-Villaluenga C, Cardelle-Cobas A, Corzo N, Olano A, Villamiel M. Optimization of conditions for galactooligosaccharide synthesis during lactose hydrolysis by β-galactosidase from Kluyveromyces lactis (Lactozym 3000L HPG). Food Chem. 2008;107:258–264.
- Shukla TP. Beta-galactosidase technology: a solution to the lactose problem. Crit Rev Food Technol. 1975;5:325–356.
- Covan DA, Daniel RM, Morgan HW. Some properties of β-galactosidase from an extremely thermophilic bacterium. Biotechnol Bioeng. 1984;26:1141–1145.
- Shen LM. Basic Biochemistry. Beijing: China Forestry Publishing House; 1996.
- Kong F, Wang Y, Cao S, Gao R, Xie G. Cloning, purification and characterization of a thermostable β-galactosidase from Thermotoga naphthophila RUK-10. Process Biochem. 2014;49:775–782.
- Panesar PS, Kumari S, Panesar R. Potential applications of immobilized β-galactosidase in food processing industries. Enzyme Res. 2010;2010:1–16.
- Rajakala P, Karthigai PS. The effect of pH, temperature and alkali metal ions on the hydrolysis of whey lactose catalysed by β-galactosidase from Kluyveromyces marxianus. Int J Dairy Sci. 2006;1:167–172.
- Halbmayr E, Mathiesen G, Nguyen TH, Maischberger T, Peterbauer CK, Eijsink VG, Haltric D. High-level expression of recombinant β-galactosidases in Lactobacillus plantarum and Lactobacillus sakei using a sakacin P-based expression system. J Agric Food Chem. 2008;56:4710–4719.
- Gekas V, Lopez-leiva M. Hydrolysis of lactose: a literature review. Process Biochem. 1985;2:2–12.
- Palumbo MS, Smith PW, Strange ED, Van Hekken DL, Tunick MH, Holsinger VH. Stability of beta-galactosidase from Aspergillus oryzae and Kluyveromyces lactis in dry milk powders. J Food Sci. 1995;60(1):117–119.
- Gao XR, Ma L, Ye H. Research advancement of β-galactosidase. Biotechnol Bull. 2005;3:18–19.
- Liu B, Zhou FF, Wu SJ, Xu Y, Zhang XB. Genomic and proteomic characterization of a thermophilic Geobacillus bacteriophage GBSV1. Res Microbiol. 2009;160(2):166–171.
- Bradford MM. A rapid and sensitive method for the quantitation of microgram quantities of protein utilizing the principle of protein-dye binding. Anal Biochem. 1976;72(1):248–254.
- Ustok FI, Tari C, Harsa S. Biochemical and thermal properties of β-galactosidase enzymes produced by artisanal yoghurt cultures. Food Chem. 2010;119:1114–1120.
- Liu B, Wang YQ, Zhang XB. Characterization of a recombinant maltogenic amylase from deep sea thermophilic Bacillus sp. WPD616. Enzyme Microb Technol. 2006;39:805–810.
- Demirjian DC, Moris-Varas F, Cassidy CS. Enzymes from extremophiles. Curr Opin Chem Biol. 2001;5:144–151.
- Zaks A. Industrial biocatalysis. Curr Opin Chem Biol. 2001;5:130–136.
- Gomes J, Steiner W. The biocatalytic potential of extremophiles and extremozymes. Food Technol Biotech. 2004;42:223–235.
- Daabrowski S, Sobiewska G, Maciuńska J, Synowiecki J, Kur J. Cloning, expression, and purification of the His6-tagged thermostable β-galactosidase from Pyrococcus woesei in Escherichia coli and some properties of the isolated enzyme. Protein Expr Purif. 2000;19:107–112.
- Ramchuran SO, Nordberg Karlsson E, Velut S, Maré de L, Hagander P, Holst O. Production of heterologous thermostable glycoside hydrolases and the presence of host-cell proteases in substrate limited fed-batch cultures of Escherichia coli BL21 (DE3). Appl Microbiol Biotechnol. 2002;60:408–416.
- Ramchuran SO, Vargas V, Hatti-Kaul R, Nordberg Karlsson E. Production of a lipolytic enzyme originating from Bacillus halodurans LBB2 in the methylotrophic yeast Pichia pastoris. Appl Microbiol Biotechnol. 2006;71:463–472.
- Walsh DJ, Gibbs MD, Bergquist PL. Expression and secretion of a xylanase from the extreme thermophile, Thermotoga strain FjSS3B.1, in Kluyveromyces lactis. Extremophiles. 1998;2:2–16.
- Soutschek-Bauer E, Staudenbauer WL. Synthesis and secretion of a heat-stable carboxymethyl cellulase from Clostridium thermocellum in Bacillus subtilis and Bacillus stearothermophilus. Mol Gen Genet. 1987;208:537–541.
- Ladero M, Santos A, Garcia J, Carrascosa A, Pessela B, Garcia-Ochoa F. Studies on the activity and the stability of β-galactosidases from Thermus sp. strain T2 and from Kluyveromyces fragilis. Enzyme Microb Technol. 2002;30:392–405.
- Pisani FM, Rella R, Raia CA, Rozzo C, Nucci R, Gambacorta A, De Rosa M, Rossi M. Thermostable β-galactosidase from the archaebacterium Sulfolobus solfataricus: purification and properties. Eur J Biochem. 1990;187(2):321–328.
- Kim CS, Ji ES, Oh DK. Characterization of a thermostable recombinant β-galactosidase from Thermotoga maritima. J Appl Microbiol. 2004;97(5):1006–1014.
- Katrolia P, Zhang M, Yan QJ, Jiang ZQ, Song CL, Li L. Characterisation of a thermostable family 42 β-galactosidase (BgalC) family from Thermotoga maritima showing efficient lactose hydrolysis. Food Chem. 2011;125:614–621.
- Adalberto PR, Massabni AC, Carmona EC, Goulart AJ, Marques DP, Monti R. Effect of divalent metal ions on the activity and stability of β-galactosidase isolated from Kluyveromyces lactis. Rev Ciênc Farm Básica Apl. 2010;31(3):143–150.
- Ansari SA, Husain Q. Immobilization of Kluyveromyces lactis beta galactosidase onconcanavalin A layered aluminium oxide nanoparticles-Its future aspects in biosensor applications. J Mol Catal B. 2011;70(3–4):119–126.
- Sen S, Ray L, Chattopadhyay P. Production, purification, immobilization, and characterization of a thermostable β-galactosidase from Aspergillus alliaceus. Appl Biochem Biotechnol. 2012;167(7):1938–1953.
- Bowen WH, Lawrence RA. Comparison of the cariogenicity of cola, honey, cow milk, human milk, and sucrose. Pediatrics. 2005;116(4):921–926.
- Nguyen TH, Splechtna B, Steinböck M, Kneifel W, Lettner PH, Kulbe KD, Haltrich D. Purification and characterization of two novel β-galactosidases from Lactobacillus reuteri. J Agric Food Chem. 2006;54:4989–4998.
- Nguyen TH, Splechtna B, Krasteva S, Kneifel W, Kulbe KD, Divne C, Haltrich D. Characterization and molecular cloning of a heterodimeric β-galactosidase from the probiotic strain Lactobacillus acidophilus R22. FEMS Microbiol Lett. 2007;269:136–144.
- Juajun O, Nguyen TH, Maischberger T, Iqbal S, Haltrich D, Yamabhai M. Cloning, purification, and characterization of β-galactosidase from Bacillus licheniformis DSM 13. Appl Microbiol Biotechnol. 2011;89:645–654.
- Nguyen TT, Nguyen HA, Arreola SL, Mlynek G, Djinović-Carugo K, Mathiesen G, Haltrich D. Homodimeric β-galactosidase from Lactobacillus delbrueckii subsp. bulgaricus DSM 20081: expression in Lactobacillus plantarum and biochemical characterization. J Agric Food Chem. 2012;60:1713–1721.
- Batra N, Singh J, Banerjee UC, Patnaik PR, Sobti RC. Production and characterization of a thermostable β-galactosidase from Bacillus coagulans RCS3. Biotechnol Appl Biochem. 2002;36:1–6.