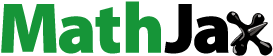
Abstract
We developed an efficient and rapid Agrobacterium-mediated tomato transformation protocol by using cotyledon and hypocotyl as explants. The transgenic nature of the regenerants was confirmed through β-glucuronidase activity staining, polymerase chain reaction (PCR), quantitative real-time PCR (qPCR) analysis and northern blot analysis. In the used protocol, the optimized experimental conditions of the tomato genetic transformation were Agrobacterium liquid concentration, equivalent to optical density (OD600) of 1.0, 20 min (cotyledon) or 30 min (hypocotyl) infection time and 2 d of co-culturing period. The optimized medium for shoot induction was Murashige & Skoog (MS) medium + 0.5 mg/L indole-3-acetic acid (IAA) + 0.5 mg/L 6-benzylaminopurine (BAP) for cotyledon and MS + 0.5 mg/L IAA + 2.0 mg/L BAP for hypocotyl. The root induction rate was the highest on the MS medium, supplemented with 0.1 mg/L IAA. The transformation frequency reached 40% per explant. The received results are a base for a larger scale development of transformation procedure.
Introduction
Tomato is an important vegetable and economic crop worldwide, and it is a model plant for fruit development research works.[Citation1] Considerable efforts have been exerted to improve the genetics of this valuable plant. Genetic transformation is a fundamental process in plant molecular genetics and plant improvement. Agrobacterium-mediated transformation is commonly employed to transfer a foreign gene into the genome of plants, such as tomato.[Citation2] Since the first transgenic tomato was produced nearly 30 years ago,[Citation3] significant progress in the genetic transformation of tomato has been achieved in recent years.[Citation4,Citation5] However, such transformation was confined to model cultivars, such as Moneymaker,[Citation6] Micro-Tom [Citation7] and Rio Grande.[Citation8] Efficient transformation protocols for different cultivars were established, but the factors that affect transformation must be optimized to realize efficient transformation in different genotype cultivars.
Tomato cv. Hezuo 908, which demonstrates inter determined growth type and high fruit-setting ability, is a commonly cultivated cultivar in several provinces in China.[Citation9] However, efficient Agrobacterium-mediated genetic transformation methods are limited for this cultivar, when compared with other major commercial tomato cultivars. Several investigators have focused themselves extensively on the genetic transformation of this cultivar, but have achieved limited success.[Citation10,Citation11]
In our previous study, we developed an Agrobacterium-mediated gene transient genetic transformation approach.[Citation9] However, no gene was integrated into the genome and transgenes were unstable and could become lost through environmental factors. In this study, we established an efficient Agrobacterium-mediated gene transformation system for cv. Hezuo 908 with four gene fragments (AV1, AC1, AC3 and AV1-AC1-AC3). Genes AV1, AC1 and AC3 encode coat protein, replication-related factor and replication accessory factor, respectively.[Citation9] Gene AV1-AC1-AC3 is a fusion gene that includes partial gene fragments of AV1 (151 bp), AC1 (150 bp) and AC3 (153 bp). Molecular characterization through the polymerase chain reaction (PCR) analysis, quantitative real-time PCR (qPCR) analysis, β-glucuronidase (GUS) activity staining and northern blot analysis revealed that 40% positive transgenic plants were successfully obtained. This study provides an alternative method for the molecular analysis of gene functions in this tomato cultivar.
Materials and methods
Plant materials
Seeds of tomato (Lycopersicon esculentum L. cv. Hezuo 908) were provided by the Institution of Biotechnology, Shanghai Academy of Agricultural Sciences. Tomato seeds were surface-sterilized with 75% ethanol for 1 min and 15% H2O2 for 15 min, rinsed 5 times with sterilized distilled water and then germinated on the Murashige & Skoog (MS) basic medium (Sigma-Aldrich, St. Louis, MO, USA) in a greenhouse, maintained at 22 °C under long-day conditions (16 h light / 8 h dark) with 60% humidity.
Optimization of plantlet regeneration parameters
In our preliminary experiments, we selected the most suitable cotyledon and hypocotyl explants. Plant hormones indole-3-acetic acid (IAA) (0.1, 0.2 and 0.5 mg/L) and 6-benzylaminopurine (BAP) (0.5, 1.0 and 2.0 mg/L) were added in the MS basic medium to optimize the plantlet regeneration from the explants. The explants were plated on the plantlet regeneration medium, containing different concentrations (0, 10, 20, 30, 40, 50, 60, 70 and 80 mg/L) of kanamycin to assess their sensitivity. Elongated shoots (2–3 cm) were transferred to the MS basic medium, supplemented with IAA (0.05, 0.1, 0.2 and 0.5 mg/L) for rooting.
Bacterial strain and plant expression vector construction
Agrobacterium tumefaciens strain LBA4404 was also provided by the Institution of Biotechnology, Shanghai Academy of Agricultural Sciences and used for all experiments. Tomato expression vectors pBIN438-AV1(i/r), pBIN438-AC1(i/r), pBIN438-AC3(i/r) and pBIN438-AV1-AC1-AC3(i/r) were constructed previously in our laboratory.[Citation9] The expression of the gene of interest was under the control of the double constitutive CaMV 35S promoter (). The plasmid was transferred into the competent cells of A. tumefaciens LBA4404 through the freeze–thaw method. The engineering strain was grown in 50 mL of the yeast extract phosphate medium, containing 100 mg/L kanamycin in a 28 °C shaker at 220 rpm for 16 h. The bacterial suspension was centrifuged and the bacteria were resuspended in the MS medium to optical density (OD600) of 1.0, and used for bacterial infection.
Tomato transformation
After 10 d of tomato seeds culturing, the cotyledons completely opened, which made them suitable to be used as explants for the next operation. Cotyledon edges were cut into two halves at the midvein region, but only the distal and central cotyledons were selected as explants. Hypocotyl (one per seedling) was then excised with an acropetal cut made just 0.5 cm below the cotyledonary nodes. Sections of hypocotyl (7–10 mm) were used as explants. All explants were dipped into Agrobacterium suspensions and gently shaken for different time (10, 20 or 30 min) to ensure a full contact between the cut explant edges and the bacterial cells. The explants were blotted dry on sterilized Whatman No. 1 filter paper, transferred to the co-cultivation medium and then incubated for different time (1, 2 or 3 d) at 24 °C in the dark. The co-cultivation medium was the MS medium, containing 3% (w/v) sucrose, 0.2% (w/v) Gelzan (Sigma-Aldrich, St. Louis, MO, USA) and plant hormones –0.5 mg/L IAA and BAP (0.5 mg/L for cotyledon and 2.0 mg/L for hypocotyl). After co-culture, the explants were transferred to the shoot induction medium, namely, the MS medium containing 0.5 mg/L IAA and BAP (0.5 mg/L for cotyledon and 2.0 mg/L for hypocotyl), 0.2% (w/v) Gelzan, 3% (w/v) sucrose, 50 mg/L kanamycin and 500 mg/L cefotaxime. The plates were incubated at 28 °C with a 16 h photoperiod. After 30 d, the plantlets were excised from the original explants and transferred to the shoot proliferation medium, namely, the MS medium, containing 0.5 mg/L IAA and BAP (0.5 mg/L for cotyledon and 2.0 mg/L for hypocotyl), 0.2% (w/v) Gelzan, 3% (w/v) sucrose, 50 mg/L kanamycin and 250 mg/L cefotaxime. After 30 d of shoot proliferation, shoots, elongated to 2–3 cm, were cut off and transferred to a root induction medium, namely, the MS medium containing 0.1 mg/L IAA, 0.3% (w/v) Gelzan, 3% (w/v) sucrose and 50 mg/L kanamycin. After 20 d of rooting, the rooted tomatoes were subjected to different analyses, as putative transgenic plants. Transformation cycle, which was also assessed in this study, is the time from tomato seeds sterilization to rooted transgenic plants obtaining.
GUS histochemical assay
GUS expression was determined, as previously described, with minor modifications.[Citation12] Transgenic plant leaves were sampled and incubated overnight at 37 °C in X-Gluc buffer containing 1 mmol/L X-Gluc, 100 mmol/L sodium phosphate buffer, pH 7.0, 0.5 mmol/L potassium ferricyanide, 0.5 mmol/L potassium ferrocyanide and 0.1% (v/v) Triton X-100. Chlorophyll was then removed from the tissues by using ethanol at room temperature for 48 h.
Polymerase chain reaction (PCR) analysis
Tomato genomic DNA was extracted from putative transgenic tomato leaves by using the cetyltrimethylammonium bromide method, as previously described.[Citation13] The non-transformed plant genomic DNA was used as a negative control. PCR was carried out to amplify specific fragments from 12 putative transformed plants and 1 untransformed plant, in order to confirm the presence or absence of transgenes in the tomato genome. The specific primers listed in were used during the PCR. In these primers, AV1-F and AV1-R amplified transgene AV1, AC1-F and AC1-R amplified transgene AC1, AC3-F and AC3-R amplified transgene AC3, AV1-AC1-AC3-F and AV1-AC1-AC3-R amplified transgene AV1-AC1-AC3. The approximate product lengths of AV1, AC1, AC3 and AV1-AC1-AC3 were 415, 310, 241 and 200 bp, respectively. The PCR programme was conducted with 1 cycle of denaturation at 95 °C for 1 min, followed by 27 cycles of 95 °C for 30 s, 55 °C for 30 s, 72 °C for 30 s and 72 °C for 10 min. The amplified products were analysed through electrophoresis in 1% (w/v) agarose gels. The DNA Marker was DL2000.
Table 1. Sequences of primer pairs for PCR amplification.
Quantitative real-time PCR analysis
SYBR Green I real-time PCR was developed as a fast and convenient approach to determine the copy number of transgenes in transgenic plants.[Citation14,Citation15] In this approach, the sucrose phosphate synthase (SPS) gene served as an endogenous reference gene.[Citation16] The following specific primers, LeSPS-F and LeSPS-R, were designed for tomato SPS gene (LeSPS) (GenBank accession No. AB051216) and the approximate product length was 100 bp. The following specific primers, RTM1-F and RTM1-R, were designed for the exogenous gene restricted TEV movement 1 (RTM1) and the approximate product length was 102 bp (). qPCR was conducted in a Rotor-Gene 3000 Real-time thermal cycler (Corbett Research, Australia) in a 20 μL reaction mixture system that contained 4 μL DNA template, 3 μL of each primer and 10 μL SYBR Green Real-time PCR Master Mix (TOYOBO Biotechnology). With a series of dilutions, the standard curves of the threshold cycle (CT), relative to the log of each initial template copy of RTM1 and LeSPS, were obtained. Each sample was quantified in triplicate with the same volume of DNA template.
Northern blot analysis
For the northern blot analysis, 10 μg of total RNA extract from putative transgenic tomato with Trizol reagent (Invitrogen) was diluted in 1 × 3-morpholinopropanesulfonic acid (MOPS) / 50% (v/v) formamide / 10% (v/v) formaldehyde and denatured for 10 min at 65 °C and then separated on 1% agarose-formaldehyde denaturing gels.[Citation9] Untransformed tomato was selected as a negative control, whereas miRNA 166 was applied as a loading control.[Citation9] The gels were blotted overnight on Hybond-N + membrane (Roche). The membrane was hybridized with PCR-labelled gene-specific Digoxin (DIG) probes (Roche). For each tested small inference RNA (siRNA), RNA probes were amplified from the plant expression vector with the same primers, adopted for the PCR analysis. The hybridization was performed overnight at 42 °C in 50% (v/v) formamide buffer, followed by post-hybridization washes performed sequentially with 2 × saline sodium citrate (SSC) buffer and twice with 0.1 × SSC along with 0.1% (w/v) sodium dodecyl sulphate (SDS) at 65 °C. Radioactively labelled bands were detected through autoradiography.
Statistical analysis
Experiments were set up in a completely randomized design and repeated twice, with approximately 90 explants per treatment for both regeneration and transformation experiments. Regeneration frequency (RF) was calculated by using EquationEquation (1)(1)
(1) ,
(1)
(1) where Nroot is the number of independent explants producing rooted plant and Nexplants is the total number of surviving explants. RF was calculated for the cultured explants until rooted plants were obtained. Transformation frequency (TF) was calculated by using EquationEquation (2)
(2)
(2) ,
(2)
(2) where Nplant is the number of independent explants producing rooted transgenic plant and Nexplants is the total number of surviving explants. TF was also scored after the rooted plants were obtained. One-way analysis of variance was used to test the statistical significance of the effects of different concentrations of IAA and BAP on the plantlet regeneration and the effects of co-cultivation time and Agrobacterium infection time on the transformation efficiency. Significant differences among means were considered at the 5% probability level. A one-tailed Student's t-test was also performed to compare the effects of different concentrations of kanamycin on the plantlet regeneration. For each treatment, the standard error of the mean was calculated on the basis of at least three biological replicates.
Results and discussion
Optimization of regeneration parameters
To date, many studies have established efficient Agrobacterium-mediated transformation protocols for different tomato cultivars; the transformation frequencies in these protocols vary depending on the tomato genotypes.[Citation7,Citation8,Citation17,Citation18] There is not any protocol applicable for all cultivars. Considerable efforts have also been devoted to optimize the transformation of specific tomato cultivars. In preliminary experiments, different factors that affect the efficiency of shoot regeneration were optimized to provide the most suitable conditions for the plantlet regeneration.[Citation19] Commonly examined factors include explant type, medium composition and selective agent.
Tomato cotyledon and hypocotyl are common explants used in tomato transformation. In 2014, tomato hairy root was used as a new explant to assess gene expression and function.[Citation20] The effect of explant type on RF varies, depending on the genotypes of the tested tomatoes. Cotyledons and hypocotyls were used to measure the RF. The most suitable explant for plant regeneration and genetic transformation was identified. As indicated in , the highest RF of cotyledon (68%) was slightly lower than that of hypocotyl (70%). Both explants had similar regeneration frequencies, which were influenced by different hormone concentrations.
Table 2. Effects of different IAA and BAP concentrations in the regeneration medium on frequency of regeneration.
also shows the effects of the concentrations of IAA and BAP on plant regeneration. The RF of cotyledon reached its maximum percentage on the regeneration medium supplemented with 0.5 mg/L IAA and 0.5 mg/L BAP. The RF of hypocotyl, on the same regeneration medium, was 56%; after changing the BAP concentration to 2 mg/L, the RF of cotyledon decreased to 47%, whereas that of hypocotyl reached its highest percentage (70%). Therefore, the optimized media for the shoot induction of cotyledon and hypocotyl were MS + 0.5 mg/L IAA + 0.5 mg/L BAP and MS + 0.5 mg/L IAA + 2.0 mg/L BAP, respectively.
The sensitivity of the explants to kanamycin was also tested. Kanamycin, widely used in plant transformation, is phytotoxic and inhibits the regeneration of untransformed plantlets.[Citation21] After assessing the sensitivity of the explants, 50 mg/L kanamycin concentration was screened and selected for both explants (data not shown).
The effect of the IAA concentration in the root induction medium on rooting was tested. The survival of the transgenic plantlet depended on the quality and quantity of the roots. Vigorous, thick and fibrous roots were observed on the root induction medium, supplemented with 0.1 mg/L IAA after 30 d of culture ((c)).
Figure 2. A. tumefaciens-mediated transformation of tomato (L. esculentum L. cv. Hezuo 908) by using cotyledon and hypocotyl as explants. (a) Regenerated kanamycin-resistant shoots from hypocotyl; (b) regenerated kanamycin-resistant shoots from cotyledon; (c) rooted kanamycin-resistant plantlet; (d) histochemical GUS staining of kanamycin-resistant plantlet leaf; (e) growth of transgenic lines in the greenhouse.
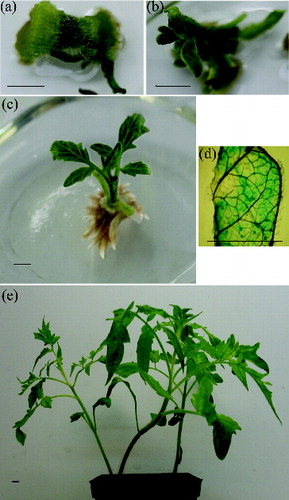
Optimization of transformation parameters
The effect of Agrobacterium infection time and co-cultivation time on tomato transformation efficiency was evaluated to obtain high plant transformation efficiency. presents the effect of the above-mentioned factors on tomato transformation efficiency. The highest transformation efficiency was shown by the cotyledon (45%) and hypocotyl (33%) infected with bacterial suspension for 20 and 30 min, respectively and co-cultivated for 2 d.
Table 3. Factors affecting the transformation frequency.
After co-cultivation in the dark, the explants were transferred to the shoot induction medium with 16 h photoperiod per day. At 10 d after culture, some buds turned green, whereas others turned white. At 30 d after culture under light illumination, green buds proliferated and elongated ((a) and 2(b)). Green buds were cut and transferred to the shoot proliferation medium and then transferred to the root induction medium. After rooting ((c)), the plantlets were transferred to greenhouse and grown in soil ((e)). At this time, the transformation efficiency was evaluated for further analysis.
We introduced four genes to evaluate the transformation efficiency of our approach. As shown in , the transformation efficiency of cotyledon ranged from 33.3% to 45.6%, whereas that of hypocotyl ranged from 35.6% to 44.4%. Although the transformation efficiency fluctuated by nearly 10%, when compared with different gene transformation experiments, the efficiency was still sufficiently high to obtain transgenic plants in a small transformation experiment. In particular, the transformation efficiency of tomato cv. Hezuo 908 reached 40%, an achievement, similar to previous work.[Citation11]
Table 4. Transformation frequency of tomato with different explants and transgenes.
Aside from TF, transformation cycle is another critical parameter in evaluating the transformation efficiency. In previous studies, the total transformation cycle may take four months [Citation7] or more.[Citation18] In contrast, a complete transformation cycle in our protocol () only needs approximately 90 d, including explants preparation (10 d), co-cultivation (2 d), shoot induction (30 d), shoot proliferation (30 d) and rooting (20 d). The main reason for this short transformation cycle was the direct transgenic shoot induction from the explants and not from the callus.
Figure 3. Protocol used for A. tumefaciens-mediated tomato (L. esculentum L. cv. Hezuo 908) transformation.
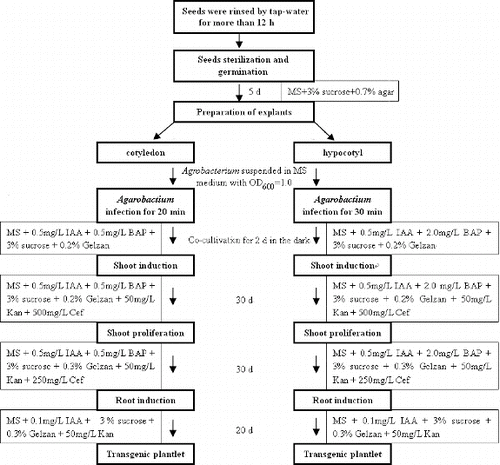
This study presents a useful protocol for Agrobacterium-mediated tomato (L. esculentum L. cv. Hezuo 908) transformation. We introduced genetic materials into the host genome and sustained transgene expression, even after the replication of host cells. Foreign genetic materials (DNAs and RNAs) are introduced in plant genomes either stably or transiently, depending on the nature of the genetic materials and the objective of the experiment.[Citation22–24] Transformation is important for studying the function of genes [Citation25] or gene products, by enhancing or inhibiting a specific gene expression in cells.[Citation26] It also aids the production of recombinant siRNAs [Citation9] or proteins [Citation27] in cells, to ensure that the cells possess novel functions [Citation28] or act as plant bioreactors.[Citation29,Citation30] The establishment of this protocol complements previous studies and improves tomato cultivar transformation.
Histochemical GUS assay
Histochemical analysis of the GUS activity was conducted by using kanamycin-resistant lines. GUS-positive blue coloration was visibly detected in the leaves of some transformed lines stained with X-Gluc ((d)).
PCR analysis of transformed plants
To confirm the presence of the transgene in the kanamycin-resistant plants, the genomic DNA of these plants and untransformed plants were isolated. Twelve putative transformed plants were assayed through PCR amplification, by using specific primers for transgene. PCR analysis indicated that the expected sizes 415, 310, 241 and 200 bp for AV1, AC1, AC3 and AV1-AC1-AC3, respectively ((a)), were obtained as expected. No other amplified products were detected in the untransformed control plants. This result indicated that all of the test plants were positive transgenic plants.
Figure 4. PCR and northern blot analyses of transgenic plants. (a) PCR analysis for the detection of specific gene fragment from four transgenic lines (AV1, AC1, AC3 and AV1-AC1-AC3). Lanes: positive control (plant expression vector) (1); transgenic plants (2–4); negative control (untransformed plant) (5); DNA marker DL2000 (M; from top to bottom 2000 bp, 1000 bp, 750 bp, 500 bp, 200 bp, 100 bp). (b) Northern blot analysis for the detection of siRNA accumulation in four transgenic lines (AV1, AC1, AC3 and AV1-AC1-AC3). Lanes: transgenic plants from (a) lanes 2–4, respectively (2–4); negative control (untransformed plant) from (a) lane 5 (5).
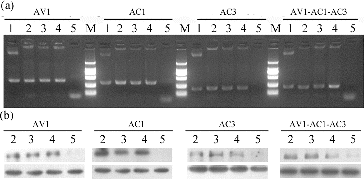
qPCR analysis of transformed plants
In transgenic plants, the number of transgene copies is a significant factor that greatly influences transgene expression level and genetic stability.[Citation16] The correlation coefficients of CT relative to the log of each initial template copy of RTM1 and LeSPS was 0.9912 and 0.9957, respectively. The initial copy number calculation equation for LeSPS and RTM1 was 10(−0.293 × CT + 10.674) and 10(−0.312 × CT + 11.351), respectively. As shown in , all transgenic lines had single transgene copy number in the tomato genome.
Table 5. Estimation of transgene copy number in each transgenic line.
Northern blot analysis of transformed plants
siRNA is an effective tool in plant anti-virus breeding; thus, an efficient method of delivering siRNA is urgently needed.[Citation31] Expressing siRNA through stable gene transformation is a candidate approach. In our experiment, transgenic tomato was considered successful on the basis of its capability to generate siRNAs. Northern blot was applied to investigate siRNA production in transgenic plants. As shown in (b), the transgenic plants accumulated some siRNAs, whereas untransformed plants did not produce detectable amounts of siRNAs. These results indicated that the transgenic tomato plants can express siRNAs.
Conclusions
In this study, an Agrobacterium-mediated tomato (Lycopersicon esculentum L. cv. Hezuo 908) transformation system was established, which provides a baseline for an efficient insertion of gene into this cultivars. In our protocol, tomato cotyledon and hypocotyl were selected as explants. In Agrobacterium infection section, bacterial liquid concentration equivalent to OD600 of 1.0, 20 min (cotyledon) or 30 min (hypocotyl) infection time and 2 d co-culturing periods. The shoot induction and proliferation medium was MS + 0.5 mg/L IAA + 0.5 mg/L BAP for cotyledon and MS + 0.5 mg/L IAA + 2.0 mg/L BAP for hypocotyl. The root induction medium was MS + 0.1 mg/L IAA. The TF reached 40% per explant.
Disclosure statement
No potential conflict of interest was reported by the authors.
Additional information
Funding
References
- Barone A, Di Matteo A, Carputo D, et al. High-throughput genomics enhances tomato breeding efficiency. Curr Genomics. 2009;10:1–9.
- Garcia D, Narvaez-Vasquez J, Orozco-Cardenas ML. Tomato (Solanum lycopersicum). Methods Mol Biol. 2015;1223:349–361.
- McCormick S, Niedermeyer J, Fry J, et al. Leaf disc transformation of cultivated tomato (L. esculentum) using Agrobacterium tumefaciens. Plant Cell Rep. 1986;5:81–84.
- McBride KE, Summerfelt KR. Improved binary vectors for Agrobacterium-mediated plant transformation. Plant Mol Biol. 1990;14:269–276.
- Kimura S, Sinha N. Tomato transformation. CSH Protoc. 2008; 2008:pdb prot5084.
- van Roekel JS, Damm B, Melchers LS, et al. Factors influencing transformation frequency of tomato (Lycopersicon esculentum). Plant Cell Rep. 1993;12:644–647.
- Sun HJ, Uchii S, Watanabe S, et al. A highly efficient transformation protocol for Micro-Tom, a model cultivar for tomato functional genomics. Plant Cell Physiol. 2006;47:426–431.
- Yasmeen A. An improved protocol for the regeneration and transformation of tomato (cv Rio Grande). Acta Physiologiae Plantarum. 2009;31:1271–1277.
- Sun S, Kang XP, Xing XJ, et al. Transient expression of siRNA targeted against the TYLCV AV1, AC1 and AC3 genes for high resistance in tomato. Scientia Horticulturae. 2014;179:321–327.
- Yang ZA, Zhang YH, Ding YM, et al. Study on genetic transformation of tomato using AGPase anti-sense gene. J Yunnan Agric Univ (Nat Sci Edition). 2008;23:158–161.
- Su CX, Huo XW, Tan QH, et al. The establishment of plant regeneration from cotyledon and hypocotol explants of tomato (Lycopersicon esculentum Mill.). J Inner Mongolia Agric Univ. 2006;4:91–95.
- Jefferson RA, Kavanagh TA, Bevan MW. GUS fusions: beta-glucuronidase as a sensitive and versatile gene fusion marker in higher plants. EMBO J. 1987;6:3901–3907.
- Chang L, Zhang Z, Yang H, et al. Detection of strawberry RNA and DNA viruses by RT-PCR using total nucleic acid as a template. J Phytopathol. 2007;155:431–436.
- Ingham DJ, Beer S, Money S, et al. Quantitative real-time PCR assay for determining transgene copy number in transformed plants. Biotech. 2001;31:132–140.
- Mason G, Provero P, Vaira AM, et al. Estimating the number of integrations in transformed plants by quantitative real-time PCR. BMC Biotechnol. 2002;2:20.
- Wang YH, Zhao S, Chen F, et al. Estimation of the copy number of exogenous gene in transgenic rice by real-time fluorescence quantitative PCR. Life Sci Res. 2007;11:301–305.
- Arshad W, Haq IU, Waheed MT, et al. Agrobacterium-mediated transformation of tomato with rolB gene results in enhancement of fruit quality and foliar resistance against fungal pathogens. PLOS One. 2014;9:e96979.
- Park SH, Morris JL, Park JE, et al. Efficient and genotype-independent Agrobacterium-mediated tomato transformation. J Plant Physiol. 2003;160:1253–1257.
- Vinoth S, Gurusaravanan P, Jayabalan N. Optimization of factors influencing microinjection method for Agrobacterium tumefaciens-mediated transformation of tomato. Appl Biochem Biotechnol. 2013;169:1173–1187.
- Ron M, Kajala K, Pauluzzi G, et al. Hairy root transformation using Agrobacterium rhizogenes as a tool for exploring cell type-specific gene expression and function using tomato as a model. Plant Physiol. 2014;166:455–469.
- Satyavathi VV, Prasad V, Lakshmi BG, et al. High efficiency transformation protocol for three Indian cotton varieties via Agrobacterium tumefaciens. Plant Sci. 2002;162:215–223.
- Recillas-Targa F. Multiple strategies for gene transfer, expression, knockdown, and chromatin influence in mammalian cell lines and transgenic animals. Mol Biotechnol. 2006;34:337–354.
- Kim TK, Eberwine JH. Mammalian cell transfection: the present and the future. Anal Bioanal Chem. 2010;397:3173–3178.
- Dan Y, Yan H, Munyikwa T, et al. MicroTom-a high-throughput model transformation system for functional genomics. Plant Cell Rep. 2006;25:432–441.
- Koul B, Srivastava S, Sanyal I, et al. Transgenic tomato line expressing modified Bacillus thuringiensis cry1Ab gene showing complete resistance to two lepidopteran pests. Springerplus. 2014;3:84.
- Gao D, Huibers RP, Loonen AE, et al. Down-regulation of acetolactate synthase compromises Ol-1- mediated resistance to powdery mildew in tomato. BMC Plant Biol. 2014;14:32.
- Gong B, Li X, VandenLangenberg KM, et al. Overexpression of S-adenosyl-L-methionine synthetase increased tomato tolerance to alkali stress through polyamine metabolism. Plant Biotechnol J. 2014;12:694–708.
- Gisbert C, Rus AM, Bolarin MC, et al. The yeast HAL1 gene improves salt tolerance of transgenic tomato. Plant Physiol. 2000;123:393–402.
- Zhang H, Zhao L, Chen Y, et al. Expression of human coagulation Factor IX in transgenic tomato (Lycopersicon esculentum). Biotechnol Appl Biochem. 2007;48:101–107.
- Wurbs D, Ruf S, Bock R. Contained metabolic engineering in tomatoes by expression of carotenoid biosynthesis genes from the plastid genome. Plant J. 2007;49:276–288.
- Mao YB, Cai WJ, Wang JW, et al. Silencing a cotton bollworm P450 monooxygenase gene by plant-mediated RNAi impairs larval tolerance of gossypol. Nat Biotechnol. 2007;25:1307–1313.