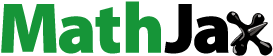
ABSTRACT
Strain CY-012 isolated from garden soil sprayed with pyrethroid pesticide was able to efficiently degrade fenvalerate. The bacterium was identified as Bacillus licheniformis based on its morphology, physiological and biochemical characteristics, and 16S ribosomal DNA gene analysis. Response surface methodology analysis showed that the optimum conditions for fenvalerate degradation were fenvalerate concentration of 44.04 mg L−1, pH 7.48, and ferric chloride concentration of 0.051% (w/v). Under these conditions, approximately 80.07% of fenvalerate was degraded within 60 h of incubation. Five metabolic compounds, including α-isopropyl-4-chlorobenzene acetic acid, 4-chlorobenzene acetic acid, 3-phenoxybenzyl alcohol, phenol and benzoic acid were detected during fenvalerate degradation and identified by gas chromatography–mass spectrometry. A possible degradation pathway of fenvalerate was proposed based on these identified metabolites. The results indicated that strain CY-012 could potentially be used to eliminate environmental contamination with pyrethroid insecticides.
Introduction
Fenvalerate, (RS)-α-cyano-3-phenoxyphenyl(RS)-2-(4-chlorophenyl)-3-methylbutyrate, is a synthetic pyrethroid pesticide that has been used in agriculture and forestry worldwide against pests and for livestock disease prevention because of its short half-life and relatively low level of toxicity [Citation1]. However, fenvalerate may have detrimental effects on non-target organisms such as fish and aquatic insects [Citation2], beetles [Citation3], bees [Citation4] and microorganisms [Citation5]. Moreover, long-term exposure to fenvalerate may cause chronic diseases [Citation6], while the increase of soil residues can cause serious environmental pollution and ecological destruction [Citation7,Citation8]. Therefore, it has become an important goal to eliminate or reduce fenvalerate residues in food and the environment.
Biodegradation refers to the use of various microorganisms to degrade toxic pesticide residues. It has received increasing attention because biodegradation is characterized by the use of mild conditions, high removal efficiency and environmentally friendly activity [Citation9]. Up to the present, including Bacillus [Citation10], Micrococcus [Citation11], Sphingomonas [Citation12], Aspergillus [Citation13] and Pseudomonas [Citation14], etc. have been reported to degrade synthetic pyrethroid pesticides. However, little information is available concerning the biodegradation of fenvalerate [Citation9,Citation15] and its degradation pathway. Yu et al. [Citation16] reported that Sphingomonas sp. F-7 was capable of completely degrading 100 mg L−1 fenvalerate within 72 h and 3-phenoxybenzoic acid (3-PBA) was detected as a major metabolite. However, the degradation pathway of fenvalerate and the continuous degradation of 3-PBA strain F-7 have not yet been studied.
Thus, in this study, further to Birolli et al. [Citation17], who only analyzed the degradation products of esfenvalerate, a novel fenvalerate-degrading bacterium that could efficiently degrade fenvalerate was isolated and identified as Bacillus licheniformis based on 16S ribosomal DNA (rDNA) sequence analysis. In addition, the optimum cultural conditions favouring the fenvalerate degradation were determined by the response surface methodology (RSM) using the Box–Behnken experimental design. Furthermore, based on the bacterial metabolic products, the biochemical degradation pathway of fenvalerate was proposed. The findings highlighted the potential of B. licheniformis in bioremediation of fenvalerate-contaminated environments.
Materials and methods
Chemicals and media
A fenvalerate standard (purity ≥ 99%) was obtained from the National Standard Centre. The original pesticide fenvalerate (purity 96%) was purchased from Nanjing Rongcheng Chemical Company, China. Chromatographic grade acetonitrile was obtained from Adamas Company. 3-PBA (purity 98%) was obtained from TCI Reagent Factory. Benzoic acid, 4-chlorobenzene acetic acid and phenol were purchased from Kelong Chemical Reagent Factory (Chengdu, China). All other chemicals, which were purchased from Kelong Chemical Reagent Factory, were of analytical grade.
Luria–Bertani (LB) medium containing 10.0 g L−1 tryptone, 5.0 g L−1 yeast extract and 10.0 g L−1 NaCl, and mineral medium (MM) containing 1.5 g L−1 (NH4)2SO4, 1.5 g L−1 K2HPO4, 0.5 g L−1 KH2PO4, 0.2 g L−1 MgSO4 and 0.5 g L−1 NaCl were used in this study. Different amounts of fenvalerate were added to the media to obtain the desired concentrations.
Isolation and identification of fenvalerate-degrading strains
Soil samples used as inocula were collected from garden soil in Sichuan, China, which had been sprayed with pyrethroid pesticide. The samples were transferred into 100-mL Erlenmeyer flasks containing sterilized enrichment LB medium with fenvalerate at concentrations of 50, 100, 200, 400 and 800 mg L−1, and cultured at 30 °C on a rotary shaker at 180 rpm. After 6 days of incubation, the bacterial suspensions were diluted and spread on MM agar plates containing 100 mg L−1 fenvalerate. Then, bacteria were repeatedly purified on LB and MM agar plates with 100 mg L−1 fenvalerate to isolate individual colonies. The ability of the isolates to degrade fenvalerate was determined by high-performance liquid chromatography (HPLC). One of the pure isolates that showed the highest degradation activity was selected for further study and named strain CY-012.
Strain CY-012 was characterized by its morphology, physiological and biochemical characteristics, and genetic analysis based on a 16S rDNA gene sequence. Cell morphology was observed under an electron microscope. The physiological and biochemical characteristics corresponded to those provided in the second edition of Bergey's Manual of Systematic Bacteriology [Citation18]. Two polymerase chain reaction (PCR) primers, Eu27F (5′-AGAGTTTGATCCTGGCTCAG-3′, 10 μmol L−1) and 1490R (5′-GGTTACCTTGTTACGACTT-3′, 10 μmol L−1), were used to amplify the 16S rDNA gene. The PCR product was sent for sequencing to Invitrogen, and then the sequence was compared to sequences available in GenBank using the BLAST program. Sequence alignment was carried out using Clustal X, and a phylogenetic tree was constructed using the MEGA software version 6.0.
Extraction and determination of residual fenvalerate
Extraction of residual fenvalerate from the media was done according to the method described by Tang et al. [Citation19]. Take 1.0 mL of well-mixed liquid nutrient medium and an equal volume of acetonitrile to a 10 mL tube, followed by ultrasonic-assisted extraction for 60 min and centrifugation at 17,000 × g for 10 min, then diluted with acetonitrile to 10 mL. The supernatant was filtered through 0.45-μm organic filter membrane. The quantitative determination of fenvalerate was performed using the Waters 2695 HPLC system with an Inertsil ODS-3 (5.0 μm, 150 mm × 4.60 mm i.d.) chromatographic column and a mobile phase of acetonitrile–ultrapure water (90:10, v/v) at a flow rate of 1.0 mL min−1. The column temperature was 25 °C, the detection wavelength was 210 nm, and the sample volume was 10 μL. Fenvalerate was identified and quantified based on the retention time and peak area of the pure standard.
Optimization of fenvalerate-degradation conditions
RSM based on the Box–Behnken experimental design [Citation20] was used to determine the optimal conditions for degrading fenvalerate by strain CY-012. With single factor tests and the Plackett–Burman (PB) experimental design, three selected factors that significantly affected the fenvalerate degradation activity of strain CY-012 were represented at suboptimal (−1), optimal (0) and supraoptimal (+1) levels of performance and retested using full combinations of the factors as described in . All experiments were done in triplicate with uninoculated media used as controls. Residual concentrations were tested after 60 h of incubation. The results were then analyzed using RSM with the following quadratic regression model:(1)
(1) where Y is the predicted response (fenvalerate degradation rate, %), Xi and Xj are independent variables, b0 is a constant, bi is a linear coefficient, bij is an interaction coefficient, and bii is a quadratic coefficient.
Table 1. Results of Box–Behnken experimental design.
Isolation and identification of fenvalerate metabolites
Metabolites of fenvalerate, produced in the optimized culture medium, were extracted by the method described by Chen et al. [Citation21]. Sterile supernatant, with 2 mol L−1 HCl used to adjust the pH to 2.0, was obtained by centrifugation of 10 mL of the degradation mixture at 4 °C, 10,062 × g. Then, an equal volume of ethyl acetate was added, and the mixture was vortexed for 30 s and let stand for 10 min. The organic phase was passed through anhydrous Na2SO4, evaporated to dryness on a rotary evaporator at 50 °C, and then dissolved in 1 mL of methanol.
Metabolites of fenvalerate were analyzed by gas chromatography–mass spectrometry (GC–MS). GC–MS analyses were performed in an electron ionization (EI) mode (70 eV) using a gas chromatographer equipped with an MS detector and a DB-5 column (30.0 m × 0.25 mm × 0.25 mm). Nitrogen was used as the carrier gas at a constant flow of 1.5 mL/min. The extracts were analyzed at an injection temperature of 250 °C and EI source temperature of 280 °C. The extract volume was 1 μL, and the sample was injected into the column with a temperature program of 90 °C (2 min), 6 °C min−1 to 150 °C (1 min), 10 °C min−1 to 180 °C (4 min), and 20 °C min−1 to 260 °C (10 min). Metabolites were identified and quantified based on the retention times and peak areas of the pure standards [Citation16].
Identification and sustainable degradation of intermediate fenvalerate metabolites
The fenvalerate-degrading bacterium, strain CY-012, was inoculated into the optimized liquid culture medium. After 48 h of shake flask cultivation, intermediate metabolites were extracted and analyzed by the method described above. Then, strain CY-012 was inoculated into liquid MM, each flask containing a different intermediate metabolite at a concentration of 20 mg L−1 and the inoculum size of 5%. Its metabolites were extracted and analyzed after 48 h of cultivation. All experiments were done in triplicate with the uninoculated medium used as a control.
Results and discussion
Isolation and identification of a fenvalerate-degrading bacterium
Strain CY-012, which was selected for further studies, showed a superior degrading ability on LB agar plates containing 100 mg L−1 of fenvalerate. The strain was a gram-positive, aerobic, spore-shaped, single or chain arrangement bacterium, could grow well in the range of 20–41 °C. Colonies grown on LB agar plates for 24 h were red, opaque and flat, with a rough surface and border irregularity. Strain CY-012 was positive in tests for glycerol and glucose fermentation, catalase production, starch hydrolysis, gelatin liquefaction, Voges–Proskauer, citrate utilization, indole, motility, methyl red; and was negative in tests for ethanol and lactose fermentation. Phylogenetic analysis of the 16S rDNA gene sequence revealed that strain CY-012 (GenBank accession number KR349358) was grouped among Bacillus species and closely clustered with B. licheniformis CICC 10037 (GenBank accession number AY871102) and B. licheniformis BCRC 11702 (GenBank accession number NR116023), with 100% sequence identity (). Based on the morphology, physiological and biochemical characteristics and 16S rDNA gene analysis, strain CY-012 was identified as B. licheniformis.
It has been generally considered that the enrichment and screening conditions of environmental microorganisms are crucial for the selection of desired degrading bacteria [Citation22,Citation23]. In this study, members of the genus Bacillus were considered potential pesticide bioremediation agents based on previous studies [Citation24,Citation25]. In particular, Sundaram et al. [Citation25] have studied the degradation of cypermethrin in a soil microcosm by Bacillus sp. strain ISTS2, which could completely mineralize the insecticide. Liu et al. [Citation26] have found that a co-culture of B. licheniformis B-1 and Sphingomonas sp. SC-1 had a good degradation ability for cypermethrin and 3-PBA and could degrade 75.6% of cypermethrin in 24 h and 10.31 mg L−1 of 3-PBA in 72 h. However, the degradation of fenvalerate by B. licheniformis has remained largely unexplored.
Optimization of fenvalerate degradation conditions
The degradation of fenvalerate depends on various factors such as culture conditions, the inoculum amount and the presence of chemical compounds [Citation27,Citation28]. Three independent variables, including the fenvalerate concentration (X1), pH (X2) and ferric chloride concentration (X3), were demonstrated to play significant roles in the degradation of fenvalerate by B. licheniformis CY-012 in a single-factor experiment and PB experiment. Using the RSM analysis, the following quadratic polynomial model EquationEquation (2)(2)
(2) was fitted to calculate the percentage of fenvalerate degradation using coded process variables. The results of the Box–Behnken experimental design and variance analysis are presented in . The overall model was highly significant, with a regression coefficient of 0.99 (P < 0.01):
(2)
(2) where Y is the predicted degradation rate of fenvalerate by B. licheniformis CY-012, while X1, X2 and X3 are the coded values of the fenvalerate concentration, pH and ferric chloride concentration, respectively.
Table 2. Variance analysis of the results from the Box–Behnken test.
Three-dimensional response surfaces were plotted to examine the optimal conditions under which B. licheniformis CY-012 could degrade fenvalerate. As shown in , the theoretical maximum fenvalerate degradation rates were relatively similar for the three graphs. The biodegradation test of fenvalerate confirmed that the degradation rate of fenvalerate reached 80.07% after 60 h of incubation at a fenvalerate concentration of 44.04 mg L−1, pH of 7.48, and a ferric chloride concentration of 0.051% (w/v).
Figure 2. Three-dimensional plots and contours of the quadratic model for fenvalerate degradation by strain CY-012. [Citation1] Response surface (A-1) and contour map (A-2) of the fenvalerate degradation rate (Y, %) as a function of the fenvalerate concentration (X1) and pH (X2) at a fixed concentration of ferric chloride (X3) of 0.051% (w/v). [Citation2] Response surface (B-1) and contour map (B-2) of the fenvalerate degradation rate (Y, %) as a function of the concentrations of ferric chloride (X3) and fenvalerate (X1) at a fixed pH value (X2) of 7.48. [Citation3] Response surface (C-1) and contour map (C-2) of the fenvalerate degradation rate (Y, %) as a function of the concentration of ferric chloride (X3) and pH (X2) at a fixed fenvalerate concentration (X1) of 44.04 mg L−1.
![Figure 2. Three-dimensional plots and contours of the quadratic model for fenvalerate degradation by strain CY-012. [Citation1] Response surface (A-1) and contour map (A-2) of the fenvalerate degradation rate (Y, %) as a function of the fenvalerate concentration (X1) and pH (X2) at a fixed concentration of ferric chloride (X3) of 0.051% (w/v). [Citation2] Response surface (B-1) and contour map (B-2) of the fenvalerate degradation rate (Y, %) as a function of the concentrations of ferric chloride (X3) and fenvalerate (X1) at a fixed pH value (X2) of 7.48. [Citation3] Response surface (C-1) and contour map (C-2) of the fenvalerate degradation rate (Y, %) as a function of the concentration of ferric chloride (X3) and pH (X2) at a fixed fenvalerate concentration (X1) of 44.04 mg L−1.](/cms/asset/bd117a0a-6b1d-40df-ab6c-7b89e1ee588e/tbeq_a_1438210_f0002_b.gif)
Identification of metabolites of fenvalerate degradation
Metabolites were extracted and characterized by GC–MS to reveal the pathway of fenvalerate degradation by strain CY-012. After 48 h of cultivation, six compounds were produced in the fenvalerate degradation process ( and ). Compound S with a retention time of 24.925 min was identified as fenvalerate, which indicated that the substrate could not be completely degraded by B. licheniformis CY-012 after 2 days. Compounds A–E were identified as phenol (A), benzoic acid (B), 4-chlorobenzene acetic acid (C), α-isopropyl-4-chlorobenzene acetic acid (D) and 3-phenoxybenzyl alcohol (E), respectively. Among them, 3-phenoxybenzyl alcohol is easily oxidized to 3-PBA in a degradation system according to the research of Yang and Ji [Citation29] and Xiao et al. [Citation30]. The similarities of compounds B and C with their standards were only 68% and 65%, respectively, which might have been caused by their smaller chromatographic peaks and ion interference in the background, and the results indicated that compounds B and C were difficult to extract and their content in the degradation mixture was low.
Table 3. Chromatographic characteristics of the products of fenvalerate degradation by Bacillus licheniformis CY-012.
Sustainable degradation of intermediate metabolites
The intermediate metabolites, including 4-chlorobenzene acetic acid, 3-PBA, benzoic acid and phenol, were added to the optimized liquid culture medium as substrates, and the media were inoculated with B. licheniformis CY-012. After 48 h of cultivation, these substrates were extracted and characterized by GC–MS. In the degradation system of 3-PBA, phenol (a) and benzoic alcohol (b) were detected ( and ), which indicated that 3-PBA could be degraded by B. licheniformis CY-012. Based on the differences in the peak areas between the control and experimental groups, 4-chlorobenzene acetic acid, benzoic acid and phenol could apparently be degraded by B. licheniformis CY-012 (). The results indicated that B. licheniformis CY-012 was capable of sustainably degrading the intermediate metabolites, which showed that the metabolites of fenvalerate were difficult to accumulate, providing a solution for its complete degradation.
Table 4. Chromatographic characteristics of the degradation products of 3-PBA generated by Bacillus licheniformis CY-012.
Table 5. Degradation of intermediate metabolites.
Proposed metabolic pathway of fenvalerate degradation by B. licheniformis CY-012
Based on the metabolites formed, we propose a possible pathway of fenvalerate degradation by B. licheniformis CY-012 (). The parent fenvalerate [S] was first degraded into α-isopropyl-4-chlorobenzene acetic acid [D] and 3-phenoxybenzyl alcohol [E] with the breakage of the carboxyl ester bond. The main degradation pathway of pyrethroids is the hydrolysis of the ester linkage, which has been generally acknowledged to operate in a multitude of microorganisms to destroy the insecticidal activity of pyrethroids, leading to their detoxification [Citation11,Citation31,Citation32]. This also occurred in the initial steps of fenvalerate degradation. α-Isopropyl-4-chlorobenzene acetic acid [D] was transformed to 4-chlorobenzene acetic acid [C] further in the reaction. On the other hand, 3-phenoxybenzyl alcohol [E] is easily oxidized to 3-PBA [Citation11,Citation29,Citation30] and then further metabolized via diphenyl ether cleavage to yield phenol [A] [Citation33,Citation34] and benzoic acid [B]. Furthermore, phenol [A] [Citation35,Citation36], benzoic acid [B] and 4-chlorobenzene acetic acid [C] [Citation37] were found to be further degraded by B. licheniformis CY-012 when added to the optimized liquid culture medium at a concentration of 20 mg L−1.
Conclusions
In this study, strain CY-012, which was identified as B. licheniformis, was selected for its high fenvalerate-degrading potential. The optimal pH value, fenvalerate concentration and that of ferric chloride for fenvalerate degradation were 7.48, 44.04 mg L−1 and 0.051% (w/v), respectively. The degradation rate of fenvalerate could reach 80.07%, which agreed with the model predicted value (80.41%) in an optimized degradation system. The results enrich the bacterial resource of fenvalerate degradation and provide a basis for degradation pathway research. During the fenvalerate degradation process, α-isopropyl-4-chlorobenzene acetic acid and 4-chlorobenzene acetic acid were detected, which was a new discovery. Moreover, 3-phenoxybenzyl alcohol was oxidized to 3-PBA, which was further degraded into phenol and benzoic acid. Thus, the biodegradation pathway of fenvalerate was predicted, and that provided theoretical support for the degradation mechanism of fenvalerate. In addition, 4-chlorobenzene acetic acid, 3-PBA, phenol and benzoic acid were further degraded by B. licheniformis CY-012, indicating that fenvalerate could be completely degraded. Generally, this research has an important reference value and application prospect, which would contribute to the degradation studies of other pesticides and lays a foundation for bioremediation.
Disclosure statement
The authors declare no competing financial interest.
Additional information
Funding
References
- Giri S, Sharma GD, Giri A, et al. Fenvalerate-induced chromosome aberrations and sister chromatid exchanges in bone marrow cells of mice in vivo. Mutat Res. 2002;520:125–132.
- Antwi FB, Reddy GVP. Toxicological effects of pyrethroids onnon-target aquatic insects. Environ Toxicol Pharmacol. 2015;40:915–923.
- Desneux N, Decourtye A, Delpuech JM. The sublethal effects of pesticides on beneficial arthropods. Ann Rev Entomol. 2007;52:81–106.
- Decourtye A, Devillers J, Genecque E, et al. Comparative sublethal toxicity of nine pesticides on olfactory learning performances of the honeybee Apis mellifera. Arch Environ Contam Toxicol. 2005;48:242–250.
- Das R, Das SJ, Das AC. Effect of synthetic pyrethroid insecticides on N2-fixation and its mineralization in tea soil. Eur J Soil Biol. 2016;74:9–15.
- Kolaczinski JH, Curtis CF. Chronic illness as a result of low-level exposure to synthetic pyrethroid insecticides: A review of the debate. Food Chem Toxicol. 2004;42:697–706.
- Ye XQ, Xiong K, Liu J. Comparative toxicity and bioaccumulation of fenvalerate and esfenvalerate to earthworm Eisenia fetida. J Hazard Mater. 2016;310:82–88.
- Nesser GAA, Abdelbagi AO, Hammad AMA, et al. Levels of pesticides residues in the White Nile water in the Sudan. Environ Monit Assess. 2016 [cited 2018 Feb 02];188:374. DOI:10.1007/s10661-016-5367-3
- Cycoń M, Piotrowska-Seget Z. Pyrethroid-degrading microorganisms and their potential for the bioremediation of contaminated soils: a review. Front Microbiol. 2016 [cited 2018 Feb 02];7:1463. DOI:10.3389/fmicb.2016.01463
- Bhatt P, Sharma A, Gangola S, et al. Novel pathway of cypermethrin biodegradation in a Bacillus sp. strain SG2 isolated from cypermethrin-contaminated agriculture field. 3 Biotech. 2016 [cited 2018 Feb 02];6:45. DOI:10.1007/s13205-016-0372-3
- Tallur PN, Megadi VB, Ninnekar HZ. Biodegradation of cypermethrin by Micrococcus sp. strain CPN1. Biodegradation. 2008;19:77–82.
- Guo P, Wang BZ, Hang BJ, et al. Pyrethroid degrading Sphingobium sp. JZ-2 and the purification and characterization of a novel pyrethroid hydrolase. Int Biodeterior Biodegradation. 2009;63:1107–1112.
- Zhu YT, Li JL, Yao K, et al. Degradation of 3-phenoxybenzoic acid by a filamentous fungus Aspergillus oryzae M-4 strain with self-protection transformation. Appl Microbiol Biot. 2016;100:9773–9786.
- Tang AX, Wang BW, Liu YY, et al. Biodegradation and extracellular enzymatic activities of Pseudomonas aeruginosa strain GF31 on β-cypermethrin. Environ Sci Pollut R. 2015;22:13049–13057.
- Gill KK, Sandhu HS, Kaur R. Evaluation of lipid peroxidation and antioxidant status on fenvalerate, nitrate and their co-exposure in Bubalus bubalis. Pesticide Biochem Physiol. 2015;123:19–23.
- Yu FB, Shan SD, Luo LP, et al. Isolation and characterization of a Sphingomonas sp. strain F-7 degrading fenvalerate and its use in bioremediation of contaminated soil. J Environ Sci Heal B. 2013;48:198–207.
- Birolli WG, Borges EM, Nitschke M, et al. Biodegradation pathway of the pyrethroid pesticide esfenvalerate by bacteria from different biomes. Water Air Soil Poll. 2016 [cited 2018 Feb 02];227:271. DOI:10.1007/s11270-016-2968-y
- Garrity GM, Bell JA, Lilburn TG. Taxonomic outline of the prokaryotes. Bergey's manual of systematic bacteriology. 2nd ed. New York (NY): Elsevier Academic Press; 2003.
- Tang J, Yao K, Liu SL, et al. Biodegradation of 3-phenoxybenzoic acid by a novel Sphingomonas sp. SC-1. Fresenius Environ Bull. 2013;22:1564–1572.
- Ghevariya CM, Bhatt JK, Dave BP. Enhanced chrysene degradation by halotolerant Achromobacter xylosoxidans using response surface methodology. Bioresour Technol. 2011;102:9668–9674.
- Chen SH, Luo JJ, Hu MY, et al. Enhancement of cypermethrin degradation by a coculture of Bacillus cereus ZH-3 and Streptomyces aureus HP-S-01. Bioresour Technol. 2012;110:97–104.
- Gao Y, Chen SH, Hu MY, et al. Purification and characterization of a novel chlorpyrifos hydrolase from Cladosporium cladosporioides Hu-01. PLoS One. 2012 [cited 2018 Feb 02];7:e38137. DOI:10.1371/journal.pone.0038137
- Zhai Y, Li K, Song JL, et al. Molecular cloning, purification and biochemical characterization of a novel pyrethroid-hydrolyzing carboxylesterase gene from Ochrobactrum anthropi YZ-1. J Hazard Mater. 2012;221–222:206–212.
- Zu L, Li GY, An TC, et al. Biodegradation kinetics and mechanism of 2,4,6-tribromophenol by Bacillus sp. GZT: a phenomenon of xenobiotic methylation during debromination. Bioresour Technol. 2012;110:153–159.
- Sundaram S, Das MT, Thakur IS. Biodegradation of cypermethrin by Bacillus sp. in soil microcosm and in-vitro toxicity evaluation on human cell line. Int Biodeterior Biodegradation. 2013;77:39–44.
- Liu FF, Chi YL, Wu S, et al. Simultaneous degradation of cypermethrin and its metabolite, 3-phenoxybenzoic acid, by the cooperation of Bacillus licheniformis B-1 and Sphingomonas sp. SC-1. Agric Food Chem. 2014;62:8256–8262.
- Chen S, Dong YH, Chang C, et al. Characterization of a novel cyfluthrin-degrading bacterial strain Brevibacterium aureum and its biochemical degradation pathway. Bioresour Technol. 2013;132:16–23.
- Wan SG, Li GY, An TC, et al. Biodegradation of ethanethiol in aqueous medium by a new Lysinibacillus sphaericus strain RG-1 isolated from activated sludge. Biodegradation. 2010;21:1057–1066.
- Yang ZH, Ji GD. Quantitative response relationships between degradation rates and functional genes during the degradation of beta-cypermethrin in soil. J Hazard Mater. 2015;299:719–724.
- Xiao Y, Chen SH, Gao YQ, et al. Isolation of a novel beta-cypermethrin degrading strain Bacillus subtilis BSF01 and its biodegradation pathway. Appl Microbiol Biot. 2014;99:2849–2859.
- Wang BZ, Guo P, Hang BJ, et al. Cloning of a novel pyrethroid-hydrolyzing carboxylesterase gene from Sphingobium sp. strain JZ-1 and characterization of the gene product. Appl Environ Microbiol. 2009;75:5496–5500.
- Lin QS, Chen SH, Hu MY, et al. Biodegradation of cypermethrin by a newly isolated actinomycetes HU-S-01 from wastewater sludge. Int J Environ Sci Technol. 2011;8:45–56.
- Zhao JY, Chi YL, Xu YC, et al. Co-metabolic degradation of β-cypermethrin and 3-phenoxybenzoic acid by co-culture of Bacillus licheniformis B-1 and Aspergillus oryzae M-4. Plos One. 2016 [cited 2018 Feb 02];11:e0166796. DOI:10.1371/journal.pone.0166796
- Topp E, Akhtar MH. Identification and characterization of a Pseudomonas strain capable of metabolizing phenoxybenzoates. Appl Environ Microbiol. 1991;57:1294–1300.
- Zhou WG, Guo WB, Zhou HB, et al. Phenol degradation by Sulfobacillus acidophilus TPY via the meta-pathway. Microbiol Res. 2016;190:37–45.
- Wang Y, Chen H, Liu YX, et al. An adsorption-release-biodegradation system for simultaneous biodegradation of phenol and ammonium in phenol-rich wastewater. Bioresource Technol. 2016;211:711–719.
- Wang GY, Wen JP, Yu GH, et al. Anaerobic biodegradation of phenol by Candida albicans PDY-07 in the presence of 4-chlorophenol. World J Microbiol Biotechnol. 2008;24:2685–2691.