ABSTRACT
Currently, Salmonella enterica is the most common bacterial foodborne pathogen, causing serious extraintestinal disease. Typing methods play an important role on pathogens’ source tracking, knowing the source(s) of bacteria in pharmaceutical sciences, preventing and controlling the diarrhea and food-poisoning outbreaks. The purpose of this study is to use different moleculer typing methods to determine the genetic variability of 38 foodborne Salmonella isolates that were previously identified by biochemical tests. The methods were evaluated by four molecular techniques including 16S rRNA sequencing, PFGE, PCR-RFLP and invA-spvC PCR. 16S rRNA sequencing results showed that four of the 38 isolates were Escherichia coli, Proteus mirabilis and Citrobacter murliniae, and the others were Salmonella enterica. Thirty-eight strains were subtyped by XbaI-PFGE into 20 profiles with different clusters, while they were subtyped by 16S rRNA-RFLP into 9 profiles with a single cluster. Out of two Salmonella isolates, the invasion gene (invA) was detected in all other Salmonella isolates (94%) and the virulence gene (spvC) was detected in 11% of Salmonella isolates. Our results suggested that the PFGE subtyping is the prominent method for the evaluation and benchmarking of molecular subtyping.
Introduction
Gram-negative foodborne pathogen Salmonella is a major worldwide health problem that causes typhoidal and non-typhoidal salmonellosis. Typhoidal and non-typhoidal illnesses cause millions of cases yearly with significant economic losses and even human deaths [Citation1–3]. Salmonella infections in humans are usually associated with food, such as poultry, eggs, meat and dairy products [Citation4]. Identifying and typing of Salmonella isolates are crucial for diagnosis, treatment, epidemiological surveillance of salmonellosis and tracking the source of an outbreak [Citation5]. Multiple typing methods, including phenotypic and genotypic, are still being used to discriminate microorganisms at the strain level. Bacterial isolates can be characterized based on phenotypic traits, by using biotyping, serotyping, phage typing, antibiotic susceptibility testing, mass spectrometry (MS) and sodium dodecyl sulphate-polyacrilamide gel electrophoresis (SDS-PAGE) of cellular-extracellular components, and based on nucleic acid, by polymerase chain reaction-restriction fragment length polymorphism (PCR-RFLP), pulsed field gel electrophoresis (PFGE), random amplified polymorphic DNA (RAPD), amplified fragment length polymorphism (AFLP), ribotyping, multilocus sequence typing and multiplex PCR. Characterization of strains by using phenotypic methods is not enough and even a trained person has to spend days or weeks to identify them. Various genetic methods have been developed since 1980 s [Citation6] and these methods have been preferred due to their high discriminatory powers [Citation7]. In this study, we have evaluated three molecular methods that are PCR-RFLP, PFGE and 16S rRNA sequencing. We also have investigated invasion gene (invA) and virulence plasmid borne gene (spvC) for Salmonella spp. Relatively conserved regions are known to be associated with 16S rRNA sequences. The analysis of sequences are important to identify the various bacterial species [Citation8]. Obtained restriction DNA fragments are separated on agarose gel and the band profiles are compared. Although the RFLP system is easy to perform, inexpensive and rapid [Citation9,Citation10], the analysis using single gene and enzyme usually provides limited discriminatory power [Citation11]. PFGE is an efficient sub-typing method and commonly used in outbreak investigations. PFGE produces comparable data of genotypic characteristics of Salmonella strains and it is accepted as the gold standard among molecular methods [Citation12,Citation13]. In addition to this, it has been used in typing Salmonella in human patients, animal sources and foods because of its discriminatory power and high reproducibility [Citation14–16]. Numerous reports have been documented that using the highly discriminatory technique of PFGE was successful to track the source of Salmonella infections in different serovars [Citation17–21]. However, PFGE does not display equal sensitivity in different serovars [Citation14,Citation21] and it has several limitations including changes in DNA concentration, percent of agarose in the gel, applied voltage and gel temperature. Beside these, it requires at least 3–4 days labour intensive to complete the test and the presence of expensive specialized equipment and high quality chemicals [Citation7,Citation22,Citation23]. For sequencing analysis, 16S rRNA region was chosen in this study. The 16S and 23S rRNA genes are the most widely used molecular chronometers toform microbial phylogeny studies [Citation24,Citation25]. 16S rRNA gene-based PCR primers have been described by Lin and Tsen [Citation26] in 1996, 16S rRNA provides specific detection for all bacteria and of course for Salmonella spp. [Citation26,Citation27]. PCR-based techniques are rapid, sensitive and reliable tools in identification of foodborne pathogens such as Salmonella [Citation28,Citation29]. Chromosomal virulence genes, such as invA, invE, himA and phoP, and plasmid genes, such as spvA, spvB and spvC, are PCR target genes that detect Salmonella species [Citation30–32].
Salmonella enterica is currently the most common bacterial foodborne pathogen, causing serious extraintestinal diseases, and misrepresentation might lead to consequences that can affect many people's health. The aim of the present study was to compare four different molecular techniques commonly used in the identification of Salmonella. We show that PFGE subtyping is a prominent method for the evaluation and benchmarking of molecular subtyping, compared to three other commonly used typing methods and, to the best of our knowledge, this is the first report comparing these typing methods.
Materials and methods
Bacterial strains, media and growth conditions
In this study, 38 Salmonella strains [Citation33] were obtained from Prokaryote Genetic Laboratory Culture Collection of Ankara University. A total of 38 different Gram-negative strains (34 - Salmonella enterica subsp. enterica, 2 - Proteus mirabilis, 1- Escherichia coli and 1- Citrobacter murliniae) were isolated from chicken and red meat samples, randomly collected from city markets. Luria-Bertani broth (LB) was used to make strains grow and the samples were taken from glycerol stocks. Salmonella strains were grown overnight (18 h) under aerobic conditions at 37 °C (Merck, US).
Preparation of DNA: making and casting agarose plugs
PFGE was carried out using CDC PulseNet protocol for Salmonella [Citation34] with minor modifications. Overnight Salmonella cultures were diluted between 0.8 and 1 at OD610, and centrifuged at +4 °C, 9000 × g for 5 min. Pellets were washed with 500 µL sterile cell suspension buffer (CSB) (100 mmol/L Tris pH:8.0, 100 mmol/L ethylenediaminetetraacetic acid (EDTA) pH:8.0). The supernatant was discarded, centrifuged at +4 °C, 10.000 rpm for 5 mins and resuspended in 100 μL of the same buffer. Resuspension was mixed with 2% low melting grade agarose (BioShop, Canada); 100 μL mixture was transferred into plug molds (10 mm × 5 mm × 1.5 mm, BioRad, USA) and stored until polymerization at 4 °C. Cells, embedded in agarose, were lysed in situ with Lysis-Proteinase K Buffer (50 mmol/L Tris pH: 8.0, 50 mmol/L EDTA pH: 8.0, 10% sarcosyl, proteinase K 0.1 mg/mL) for 2 h at 50 °C under shaking. After lysing, buffer was discarded and agarose plugs were put into the new sterile tubes. Agarose plugs containing genomic DNA were washed at 54 °C for 15 min: twice with 5 mL dH2O (pre-heated to 54 °C) to remove the residual lysis buffer coating the plugs and four times with 5 mL 0.5 mol/L TE buffer (pre-heated to 54 °C). The agarose plugs including the pure DNA were obtained after washing them and the plugs were put into 1.5 mL microcentrifuge tubes containing 1 mL 0.5 mol/L TE buffer. The plugs were restricted immediately or stored in TE buffer at 4 °C until needed.
Restriction digestion and PFGE analysis
Each DNA embedded agarose plug was cut into four slices (approximately 2-mm-wide) with surgical blades and placed in a sterile microcentrifuge tube that contains 100 µL of a dilution of the appropriate restriction buffer and BSA for the enzyme. Chromosomal DNA was subjected to enzymatic digestion in microcentrifuge tubes with 20 U of XbaI (New England BioLabs, Massachusetts, USA) according to the instructions of the manufacturer. After digestions, slices were taken into 0.5 × TBE buffer for 30 min (175 rpm under shaking condition at room temperature) to stop the enzyme activities. DNA containing agarose plugs of Salmonella serovar Braenderup H9812 were cut by the same restriction buffer solution as described above and XbaI-digested Salmonella Braenderup strain H9812 was used as a ‘universal’ molecular weight standard marker and normalization reference [Citation35,Citation36]. Electrophoresis of restricted DNA fragments was achieved through a contour-clamped homogenous electric field method on CHEF DR-III system (Bio-Rad Laboratories, Hercules, CA) by using 1% pulsed-field certified agarose gel and 0.5 × Tris-borate–EDTA (TBE) buffer. 1% pulsed-field certified agarose gel was prepared using a 15-well comb (Bio-Rad) in the casting stand. DNA containing agarose plug slices were loaded into wells. Electrophoresis was performed at 14 °C and at 120° angle. Electrophoresis conditions were as follows: initial switch time value of 2.2 sec, final switch time of 63.8 sec at a gradient of 6 V/cm and electrophoresis run time of 18 h. Results of PFGE profiles were visualized under UV after staining with ethidium bromide (10 μg/mL). Then, the gels were de-stained with 500 mL of deionized water for 30 min. The pulsotypes (PFPs) were recorded digitally using Kodak Gel Logic 200 Imaging System and documented as TIFF files. The normalization process is carried out with the standard strain of S. Braenderup H9812 in each gel. Band sizes were calculated by comparing them with the standard strains.
Genomic DNA isolation, PCR-RFLP, invA, spvC, 16S rRNA gene amplifications and sequencing
Salmonella DNA was extracted with CTAB method as described by Wilson [Citation37]. PCR was performed using a primer set targeted to region 16S rRNA of Salmonella genome as described by Lagatolla et al. [Citation38] for RFLP. All isolates were examined for the presence of genes encoding virulence (spvC) and invasion (invA). shows the primers used for PCR amplification of genes. PCR amplifications were performed in a ThermoCycler (Techne TC-512, Staffordshire, UK) in 0.2 mL reaction tubes each with 50 µL reaction mixtures composed of the primer 0.2 µmol/L (Ella Biotech GmbH, Martinsried, Germany), 0.25 mmol/L dNTP mix (Thermo Scientific, USA), 1 X reaction buffer, 2 mmol/L MgCl2, 0.025 U/mL Taq polymerase (Thermo Scientific, USA) and 1 µL extracted DNA.
Table 1. Polymerase chain reaction primers.
16S rRNA PCR amplicons were enzymatically digested with five restriction enzymes including AluI, BglI, HindIII, EcoRI, BgIII (Thermo Scientific, USA) according to instructions of the manufacturer. A 100 bp marker (Thermo Scientific, USA) was used as a molecular weight standard. The digested DNA materials were run on 2% agarose gel electrophoresis.
Sizes of 16S rRNA amplicons were verified by sequence analysis by MEDSANTEK, a biotechnology company. The sequences were checked on BLAST for homology (data not shown).
Statistical analysis
Digital images, obtained from PFGE assay, were analyzed using NTSYSpc software (Numerical taxonomy and multivariate analysis system, version 2.2, State University of New York, Stony Brook, New York, USA). Cluster analysis of the Dice similarity co-efficient was based on the unweighted pair group method with arithmetic averages (UPGMA) and at the end of the analysis the dendrogram was generated to describe the relationship among isolates [Citation37,Citation38,Citation42,Citation43].
Result and discussion
All tested strains were typed using different molecular methods for the purpose of obtaining a common molecular profile. First of all, 34 isolates of Salmonella spp. and other Gram-negative strains which were identified with biochemical methods handled to obtain their genotypes by PFGE using XbaI as macro-restriction enzyme ( (A), (B) and (C)). Cluster analysis of the PFGE profiles sub-typed all the 38 strains into 20 different PFPs according to sizes from 1,135 to 2 kb. The similarity levels in two main clusters (A and B) ranged from 35%–100%. A minor cluster (10,5% of all isolates) was obtained by considering homology of about 45% and the isolates numbered as IS2, IS46, IS73 and IS174 were classified in this minor group. All the other isolates (out of these 4 isolates) were included in a major cluster B (89,5% of all isolates), which was subdivided into six subgroups (). Four groups of strains: the first group (IS53, IS58), the second group (IS80, IS81, IS83), the third group (IS96, IS97) and the fourth group (IS117, IS124) had the same band patterns and these strains showed that the pattern was 100% identical with others according to the PFGE profile. The genetic relation of the PFGE profiles with Salmonella spp. and the other Gram-negative strains were only found in clusters B2, B3 and B4. Surprisingly, Proteus mirabilis strain (IS96) had 100% similarity ratio with S. enterica (IS97). Citrobacter murliniae (IS23), P. mirabilis (IS96), P. mirabilis (IS98) and E. coli (IS148) strains had 45%, 81%, 67% and 63% similarity ratio with other S. enterica strains, respectively. It is possible to see that the genetic variability among Salmonella strains and other Gram-negative strains is due to harbouring linear plasmids and transposones, which can be integrated into chromosome by horizontal gene transfer between the strains in the same environment. This causes a difference in the fragment patterns and chromosomal diversity among the strains.
Figure 2. PFGE dendrogram obtained by unweighted pair group method using average linkage of dice correlation coefficients.
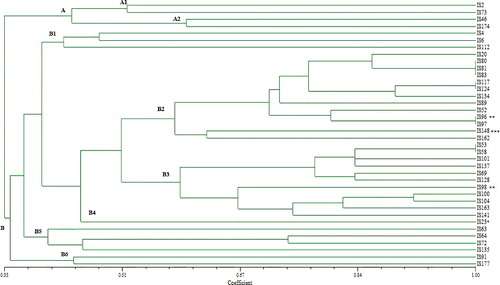
All identified isolates were subjected to an analysis of PCR-RFLP of the 16S rRNA genes, while several restriction endonucleases were separately used to digest the PCR products. The genotyping pattern of strains with enzymes including AluI, BglI, HindIII, EcoRI and BgIII (Thermo Scientific, USA) demonstrated that they can be classified into two main groups. PFGE-based assay segregated the most of the strains into different clusters, PCR-RFLP grouped these strains into a single cluster and produced 9 different restriction patterns. Although these strains displayed genetic variations in their PFGE subtypes, the PCR-RFLP patterns showed an identity among them. The similarity level in two main branches ranged from 46%–100% according to cluster analysis of PCR-RFLP profiles. 95% of isolates showed 70% similar homology in major cluster C and 5% of the isolates in minor cluster D showed lower similarity. This minor cluster was comprised by P. mirabilis strains which had 46% similarity ratio with S. enterica strains. E. coli (IS148) and Citrobacter murliniae (IS23) had 70% similarity and 94% ratio with S. enterica strains, respectively (). Since it is fast, accurate and economical, PCR-RFLP could be an alternative approach for serotyping of Salmonella spp. Certain researchers used this method on different genes using different restriction endonuclease enzymes and they obtained different results [Citation44]. Sumithra et al. [Citation45] used RFLP to analyse the typing and according to their results, PCR-RFLP was used with four endonucleases which digested 16S rRNA, fliC and fimH genes. This gave a good typing result but had a low discriminatory power. PCR- RFLP assay using the fliC/fljB genes (encoding phase-1 and phase-2 flagellin of Salmonella enterica) and two restriction endonucleases (MboI, HhaI) showed that PCR-RFLP was not able to differentiate Salmonella serotypes [Citation46]. They demonstrated that the PCR-based RFLP test could not take a place of serotyping assay. Zaki et al. [Citation47] studied on the nine Salmonella isolates for the purpose of discrimination through PCR-RFLP by using 16S and 23S rRNA genes. They showed significant discrimination between the studied isolates at the genus level. And also, they proved that 23S rRNA PCR-RFLP was more discriminative than 16S rRNA PCR-RFLP. Importantly, the discriminatory power of the method also depends on the type of restriction endonuclease and the chosen gene [Citation48]. Our results demonstrated that PCR-RFLP using AluI, BglI, HindIII, EcoRI, BgIII restriction endonucleases and 16S rRNA gene didn't have enough discriminatory power for this study.
Figure 3. PCR-RFLP dendrogram obtained by unweighted pair group method using average linkage of dice correlation coefficients.
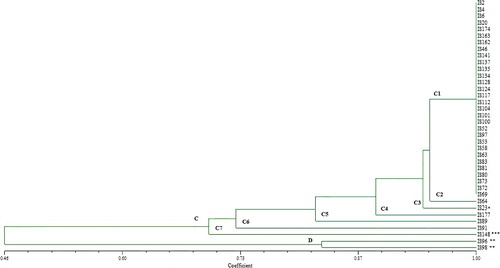
In order to confirm Salmonella strains and prove virulence of the food borne strains, the prevalence of invasion (invA) and virulence (spvC) genes were determined by conventional PCR. The invA gene was detected in all Salmonella isolates (94%) with the exception of two of them (IS124 and IS128) (). Some studies reported the detection of this gene in all tested Salmonella spp. isolates [Citation49–58]. On the other side, Moussa et al. [Citation59] and Maysa and Abd-Elall [Citation60] couldn't find invA gene in all isolates, same as our study. InvA gene was found in 47.3% and 50% in S. Enteritidis and S. Typhimurium, respectively [Citation59], and 50% in S. Newport [Citation60]. According to some researchers, detection of Salmonella by invA PCR is an international standard procedure because the gene is genus-specific gene and the technique has some advantages such as being simple, inexpensive and rapid. However, it is possible that mutations occur in invasion genes in the chromosome of wild-type Salmonella strains and therefore, we certainly cannot say that invA negative strain is not Salmonella.
Table 2. Salmonella strains used in this study, their sequence analysis and presence of inva, spvc
The spvC gene was detected on 11% of S. enterica isolates (). Das et al. [Citation53] amplified spvC gene in 42.85% of food-borne Salmonella isolates. Osman et al. [Citation56] found spvC gene in 27.8% of Salmonella strains, isolated from chicken. None of all 37 Salmonella isolated from pork and slaughterhouse by Chaudhary et al. [Citation57] possessed the spvC gene. This variable state suggested that the prevalence of Salmonella virulence plasmids is restricted within the isolates of some defined origin of sources. It seems that spvC cannot be used for identification of all Salmonella strains.
We also detected invA gene in C. murliniae (IS23), P. mirabilis (IS96, IS98) and E. coli (IS148) strains. Studies on the detection of invA gene showed that the gene has not been found in Klebsiella pneumoniae, Shigella sp., E. coli, Citrobacter freundii and Proteusmirabilis sp. [Citation49,Citation51,Citation61]. Bacterial type III secretion systems evolved by multiple horizontal transfer systems [Citation62] and invA is a prominent inner membrane component of Salmonella type III secretion system (T3SS) apparatus [Citation63]. These lateral transfers have effectively changed the pathogenic characters of bacterial species [Citation64]. Unlike the mentioned studies, in this study, the detection of invA for E. coli, Citrobacter murliniae and Proteus mirabilisis is evidence of horizontal gene transfer between members of the Enterobacteriaceae family.
Conclusions
Salmonella, an important food pathogen, infects millions of people every year, causing significant health problems and economic losses. Therefore, researchers have high-accuracy results, especially while examining the food and the food sources in terms of the pathogen aspect, which directly affects the health of many people and animals. For detection of such pathogens, it is often desirable to perform a rapid analysis by using molecular techniques. In this study, we used four molecular techniques, which are widely used in the investigation of salmonella pathogenesis. With the aim of increasing the safety of our results, these studies were conducted with salmonella strains, which are food isolates. To the best of our knowledge, this is the first report comparing these four commonly used typing methods. It is possible to say that the XbaI-PFGE technique identifies Salmonella isolates more successfully than the other three molecular techniques. However, PFGE is not sufficient enough to characterise Salmonella because it cannot be performed at serotyping level. In conclusion, the complexity and diversity of Salmonella serovars present a significant challenge to molecular approaches. With the recent improvement in sequencing technologies, full genome sequencing could be developed into a promising molecular approach to serotype Salmonella. The technology can be a major benefit in public health specifically for rapid diagnosis, epidemiological investigations, ideal vaccine, development of treatment and prophylactic strategies for salmonellosis in the near future.
Disclosure statement
The authors declare that they have no conflict of interest.
References
- Crump JA, Luby SP, Mintz ED. The global burden of typhoid fever. Bull World Health Org. 2004;82:346–353.
- Majowicz SE, Musto J, Scallan E, et al. The global burden of nontyphoidal Salmonella gastroenteritis. Clin Infec Dis. 2010;50:882–889.
- Barrow PA, Methner U. Salmonella in domestic animals. UK: CABI; 2013.
- Yan SS, Pendrak ML, Abela-Ridder B, et al. An overview of Salmonella typing public health perspectives. Clin Appl Immunol Rev. 2003;4:189–204.
- Turki Y, Mehri I, Fhoula I, et al. Comparison of five molecular subtyping methods for differentiation of Salmonella Kentucky isolates in Tunisia. World J Microbiol Biotechnol. 2014;30:87–98.
- Cai HY, Archambault M, Gyles CL, et al. Molecular genetic methods in veterinary clinical bacteriology laboratory: current usage and future applications. Animal Health Res. 2003;4:73–93.
- Li W, Raoult D, Fournier P. Bacterial strain typing in the genomic era. FEMS Microbiol Rev. 2009;33:892–916.
- Levin RE. The use of molecular methods for detecting and discriminating Salmonella associated with foods-a review. Food Biotechnol. 2009;23:313–367.
- Gallegos-Robles MA, Morales-Loredo A, Alvarez-Ojeda G, et al. Identification of Salmonella serotypes isolated from cantaloupe and chile pepper production systems in Mexico by PCR–restriction fragment length polymorphism. J Food Protect. 2008;71:2217–2222.
- Khaki P, Bidhendi SM, Ezatpanah E. PCR–RFLP of isolated Salmonella from poultry with Sau3AI and HhaI restriction endonucleases in Arak. Int J Mol Clin Microbiol. 2013;1:255–260.
- Chen Y, Son I. DNA methods in food safety: molecular typing of foodborne and waterborne bacterial pathogens: polymerase chain reaction-based subtyping methods. UK: Wiley; 2014.
- Gunel E, Kilic GP, Bulut E, et al. Salmonella surveillance on fresh produce in retail in Turkey. Int J of Food Microbiol. 2015;199:72–77.
- Oliveira SD, Bessa MC, Santos LR, et al. Phenotypic and genotypic characterization of Salmonella Enteritidis isolates. B J Microbiol. 2007;38:720–728.
- Kerouanton A, Marault M, Lailler R, et al. Pulsedfield gel electrophoresis subtyping database for foodborne Salmonella enterica serotype discrimination. Foodborne Pathog Dis. 2007;4:293–303.
- Zou W, Chen HC, Hise KB, et al. Meta-Analysis of pulsed-field gel electrophoresis fingerprints based on a constructed Salmonella database. PLoS ONE. 2013;8:e59224.
- Ozdemir K, Acar S. Plasmid profile and pulsed–field gel electrophoresis analysis of Salmonella enterica isolates from humans in Turkey. PLoS ONE. 2014;9:e95976.
- Threlfall EJ, Hampton MD, Ward LR, et al. Pulsed field gel electrophoresis identifies an outbreak of Salmonella enterica serotype Montevideo infection associated with a supermarket hot food outlet. Commun Dis Public Health. 1999;2:207–209.
- Sivapalasingam S, Barrett E, Kimura A, et al. A multistate outbreak of Salmonella enterica serotype Newport infection linked to mango consumption: impact of water-dip disinfestation technology. Clin Infect Dis. 2003;37:1585–1590.
- Dionisi AM, Carattoli A, Luzzi I, et al. Molecular genotyping of Salmonella enterica Abortusovis by pulsed field gel electrophoresis. Vet Microbiol. 2006;116:217–223.
- Best EL, Hampton MD, Ethelberg S, et al. Drug-resistant Salmonella Typhimurium DT 120: use of PFGE and MLVA in a putative international outbreak investigation. Microb Drug Resist. 2009;15:133–138.
- Wattiau P, Boland C, Bertrand S. Methodologies for Salmonella enterica subsp. Enterica subtyping: gold standards and alternatives. App Environ Microbiol. 2011;77:7877–7885.
- Herschleb J, Ananiev G, Schwartz DC. Pulsed-field gel electrophoresis. Nat Protoc. 2007;2:677–684.
- Yıldırım IH, Yıldırım SC, Koçak N. Molecular methods for bacterial genotyping and analyzed gene regions. J Microbiol Infect Dis. 2011;1:42–46.
- Gutell RR, Larsen N, Woese CR. Lessons from an evolving rRNA: 16S and 23S rRNA structures from a comparative perspective. Microbiol Rev. 1994;58:10–26.
- Luz SP, Rodríguez-Valera F, Lan R, et al. Variation of the ribosomal operon 16S-23S gene spacer region in representatives of Salmonellaenterica subspecies. J Bacteriol. 1998;180:2144–2151.
- Lin CK, Tsen HY. Use of two 16S DNA targeted oligonucleotides as PCR primers for the specific detection of Salmonella in foods. J Appl Bacteriol. 1996;80:659–666.
- Trkov M, Avguštin G. An improved 16S rRNA based PCR method for the specific detection of Salmonella enterica. Int J Food Microbiol. 2003;80:67–75.
- Malorny B, Hoorfar J, Bunge C, et al. Multicenter validation of the analytical accuracy of Salmonella PCR: towards an international standard. Appl Environ Microb. 2003;69:290–296.
- Kim T-H, Hwang HJ, Kim JH. Development of a novel, rapid multiplex polymerase chain reaction assay for the detection and differentiation of Salmonella enterica Serovars Enteritidis and Typhimurium using ultra-fast convection polymerase chain reaction. Foodborne Pathog Dis. 2017;14:580–586.
- Anukampa, Shagufta B, Sivakumar M. Antimicrobial resistance and typing of Salmonella isolated from street vended foods and associated environment. J Food Sci Technol. 2017;54:2532–2539.
- van Asten Alphons JAM, Dijk JE. Distribution of “classic” virulence factors among Salmonella spp. FEMS Immunol Med Microbiol. 2005;44:251–259.
- Jamshidi A, Bassami MR, Afshari NS. Identification of Salmonella spp. and Salmonella Typhimurium by a multiplex PCR-based assay from poultry carcasses in Mashhad-Iran. Iran J Vet Med. 2009;3:43–48.
- Taskale N, Akcelik M. Use of RAPD-PCR, plasmid profiling, class 1 integron analysis, and antimicrobial resistance for molecular characterization of Salmonella strains isolated from Turkey. Acta Aliment. 2012;41:56–66.
- Ribot EM, Fair MA, Gautom R, et al. Standardization of pulsed-field gel electrophoresis protocols for the subtyping of Escherichia coli O157:H7, Salmonella and Shigella for PulseNet. Foodborne Pathog Dis. 2006;3:59–67.
- Hunter SB, Vauterin P, Lambert-Fair MA, et al. Establishment of a universal size standard strain for use with the PulseNet standardized pulsed-field gel electrophoresis protocols: converting the national databases to the new size standard. J Clin Microbiol. 2005;43:1045–1050.
- Ai ZX, Mei BX, Yun YC, et al. Establishment and comparison of pulsed-field gel electrophoresis, multiple-locus variable number tandem repeat analysis and automated ribotyping methods for subtyping of Citrobacter strains. Biomed Environ Sci. 2012;25:653–662.
- Wilson K. Currents protocol of molecular biology: preparation of genomic DNA from bacteria. UK: Wiley; 1987.
- Lagatolla C, Dolzani L, Tonin E, et al. PCR ribotyping for characterizing Salmonella isolates of different serotypes. J Clin Microbiol. 1996;34:2440–2443.
- Goodfellow M, Stackebrandt E. Nucleic acid techniques in bacterial systematics: 16S/23S rRNA sequencing. USA: Wiley; 1991.
- Swamy SC, Barnhart HM, Lee MD, et al. Virulence determinants invA and spvC in Salmonellae isolated from poultry products, wastewater and human sources. Appl Environ Microbiol. 1996;62:3768–3771.
- Cortez AL, Carvalho AC, Ikuno AA, et al. Identification of Salmonella spp. isolates from chicken abattoirs by multiplex-PCR. Res Vet Sci. 2006;81:340–344.
- Sneath PHA, Sokal RR. Numerical taxonomy: the principles and practice of numerical classification. USA: Freeman & Co.; 1973.
- Rohlf FJ. NTSYS-pc. Numerical taxonomy and multivariate analysis system, version 1.8. Setauket, NY, USA: Exeter Publishing; 1993.
- Moradi Bidhendi S, Alaei F, Khaki P, et al. Identification of avian Salmonella isolates by PCR-RFLP analysis of a fliC gene fragment. Arch Razi Inst. 2015;70:1–6.
- Sumithra TG, Chaturvedi VK, Gupta PK, et al. PCR-RFLP analysis of fliC, fimH and 16S rRNA genes in Salmonella Typhimurium isolates of varied origin. Ann Microbiol. 2014;64:177–183.
- Jong HY, Pak TS, Pannatee S, et al. PCR-based restriction fragment length polymorphism for subtyping of Salmonella from chicken isolates. Nat Sci. 2010;44:79–83.
- Zakı S, Abd-El-Haleem D, El-Helow E, et al. Molecular and biochemical diagnosis of Salmonella in wastewater. J Appl Sci Environ Manage. 2009;13:83–92.
- Wassenaar TM, Newell DG. Genotyping of Campylobacter spp. Appl Environ Microbiol. 2000;66:1–9.
- Salehi TZ, Mahzounieh M, Saeedzadeh A. Detection of invA gene in isolated Salmonella from broilers by PCR method. Int J Poult Sci. 2005;4:557–559.
- Amini K, Salehi TZ, Nikbakht G, et al. Molecular detection of invA and spv virulence genes in Salmonella enteritidis isolated from human and animals in Iran. Afr J Microbiol Res. 2010;4:2202–2210.
- Jeníková G, Pazlarová J, Demnerová K. Detection of Salmonella in food samples by the combination of immunomagnetic separation and PCR assay. Int Microbiol. 2010;3:225–229.
- Shanmugasamy M, Velayutham T, Rajeswar J. InvA gene specific PCR for detection of Salmonella from broilers. Vet World. 2011;4:562–564.
- Das A, Hari SS, Shalini U, et al. Molecular screening of virulence genes from Salmonella enterica isolated from commercial food stuffs. Biosci Biotechnol Res Asia. 2012;9:363–369.
- Ashraf AAET, Ahmed MA, Aisha RA, et al. Detection of common (invA) gene in Salmonella isolated from poultry using polymerase chain rection technique. Benha Vet Med J. 2013;25:70–77.
- El-Feky MA, Hassan MA, Mohamed WA, et al. Detection of InvA gene in non typhoidal Salmonella isolated from food products and clinical cases. Egypt J Med Microbiol. 2014;23:33–41.
- Osman KM, Elhariri M, Amin ZM, et al. Consequences to international trade of chicken hatchlings: Salmonella enterica and its public health implications. Int J Adv Res. 2014;2:45–63.
- Chaudhary JH, Nayak JB, Brahmbhatt MN, et al. Virulence genes detection of Salmonella serovars isolated from pork and slaughterhouse environment in Ahmedabad, Gujarat. Vet World. 2015;8:121–124.
- Makwana PP, Nayak JB, Brahmbhatt MN, et al. Detection of Salmonella spp. from chevon, mutton and its environment in retail meat shops in Anand city (Gujarat), India Vet World. 2015;8:388–392.
- Moussa IM, Aleslamboly YS, Al-Arfaj AA, et al. Molecular characterization of Salmonella virulence genes isolated from different sources relevant to human health. J Food Agric Environ. 2013;11:197–201.
- Maysa AIA, Abd-Elall AMM. Diversity and virulence associated genes of Salmonella enterica serovars isolated from wastewater agricultural drains, leafy green producing farms, cattle and human along their courses. Rev Medecine Vet-Toulouse. 2015;166:96–106.
- Ziemer CJ, Steadham SR. Evaluation of the specificity of Salmonella PCR primers using various intestinal bacterial species. Lett Appl Microbiol. 2003;37:463–469.
- Gophna U, Ron EZ, Graur D. Bacterial type III secretion systems are ancient and evolved by multiple horizontal-transfer events. Gene. 2003;312:151–163.
- Worrall LJ, Vuckovic M, Strynadka NC. Crystal structure of the C-terminal domain of the Salmonella type III secretion system export apparatus protein InvA. Protein Sci. 2010;19:1091–1096.
- Ochman H, Lawrence JG, Groisman, EA. Lateral gene transfer and the natüre of bacterial innovation. Nature. 2000;405:299–304.