Abstract
Cancer pain treatment is a big challenge for healthcare providers and patients as well. The wide range of non-steroidal anti-inflammatory drugs (NSAIDs) used as painkillers in cancer patients, requires in-depth characterization of their effect on the disease process. The effects of NSAIDs have been widely studied over the last decades as preventive drugs in some oncological diseases. Metamizole is an NSAID belonging to the non-narcotic analgesics group and is highly recommended in oncology either alone or in combinations with opioid analgesics. There is a dearth of information regarding the cytotoxicity profile of metamizole and hence the present study evaluated the potential anticancer activity of metamizole in some permanent human tumour cell lines: HeLa, human cervical cancer cells; HT-29, a human colorectal adenocarcinoma cell line; and МСF-7, human breast adenocarcinoma cells. The studied tumour cells were sensitive to metamizole at doses higher than 25 μg/mL. Metamizole induced a statistically significant decrease in the viability of HeLa, HT-29 and MCF-7 cells in in vitro tests as measured by the MTT assay; the highest effect was observed at the 48th hour of the treatment. Metamizole could induce cell death by apoptosis. Metamizole also suppressed the migration of the three tumour cell lines. This was most clearly pronounced in HeLa cells. The results obtained indicate that metamizole is a suitable choice for the treatment of cancer pain and has prospects for further in-depth studies.
Introduction
Cancer pain is one of the most frightful factors for patients and a big challenge to healthcare providers. More often than not, patients with malignant diseases will experience recurrent episodes of pain. Nonsteroidal anti-inflammatory drugs (NSAIDs) are a first-line approach for mild cancer pain. NSAIDs (including non-opioid analgesics) in combination with opioid therapy are the choice in moderate cases, and opioids in severe ones. On the other hand, numerous non-clinical and clinical studies have demonstrated that NSAIDs have potential as anticancer drugs [Citation1–3]. Epidemiological studies have demonstrated that NSAIDs use is associated with a reduced risk of incidence and mortality from colon cancer [Citation4]. In addition, experimental studies have shown that NSAIDs induce apoptosis [Citation5]. Piazza et al. [Citation6] found that sulindac did not induce differentiation of HT-29 cells, but strongly induced apoptosis. Although the precise mechanism of this action is unknown, Chan et al. [Citation7] proposed that inhibition of cyclooxygenase (COX) stimulates the conversion of sphingomyelin to ceramide, a known mediator of apoptosis. Shiff et al. [Citation8] found that indomethacin, naproxen and piroxicam reduced the proliferation and induced apoptosis of HT-29 colon adenocarcinoma cells.
Metamizole is a popular non-opioid analgesic in many countries, but unknown in many others [Citation9, Citation10]. Due to its prohibition in the USA in 1977 and many other countries that followed suit, metamizole remains less studied than other NSAIDs. A PubMed search with the key words ‘metamizole’ and ‘apoptosis’ revealed only a few studies. Malsy et al. [Citation11] found that metamizole significantly inhibited cell proliferation and induced apoptosis in pancreatic cancer cells. Metamizole alone or in combination with paracetamol increased apoptosis in colon carcinoma cell lines (SW 480 and HT 29) in concentrations of 1 μmol/L, 250 μmol/L and 1 mmol/L [Citation12]. Shao and Feng [Citation13] reported that metamizole inhibited the proliferation of A549 (human lung carcinoma) cell line in a dose-depending manner, but not of HeLa. The antiproliferative effect was explained by the arrest in G1-phase of the cell cycle. Metamizole may inhibit JNK activation as well as TNF-α production [Citation14]. The treatment of osteosarcoma MG-63 osteoblast-like cells with metamizole showed an increase in apoptotic osteoblasts that later developed into necrotic cells [Citation15]. On the contrary, Zhang et al. [Citation16] demonstrated that metamizole has remarkable neuroprotective effect in cerebral ischemia, identifying metamizole as an anti-apoptotic agent.
In the context of the scarce and partly conflicting available data, the main purpose of this study was to investigate the cytotoxic potential of metamizole in HeLa, HT-29 and MCF-7 human tumour cell lines.
Materials and methods
Metamizole sodium (Analgin 500 mg/mL amp.; 2 mL, Sopharma; Batch No. 61116) was supplied by a local pharmacy. The solutions were diluted ex tempore. Acridine orange (Cat. N. A 6014), ethidium bromide (Cat. N. 46065) and penicillin–streptomycin (P 4333-100 mL) were purchased from Sigma-Aldrich Chemie GmbH (Darmstadt, Germany). Fetal bovine serum (FBS) was supplied from Gibco (Austria). Dulbecco’s Modified Eagle’s Medium with 4.5 g/L glucose, without L-glutamine was purchased from LONZA, Belgium, and DAPI (4’,6-diamidino-2-phenylindole) was supplied from AppliChem (Darmstadt, Germany).
Cell lines, maintenance and treatment
HeLa human cervical cancer cells, HT-29 human colorectal adenocarcinoma cells and МСF-7 human breast adenocarcinoma cells were obtained from the American Type Cultures Collection (ATCC). The cells were maintained in DMEM containing penicillin (100 units/mL), streptomycin 100 µg/mL, 2 mmol/L L-glutamine and 10% FBS in T-75 cm2 culture flasks at 37°C in an incubator with 5% CO2 supply and 95% humidity (CO2–incubator, model 3111; Thermo Fisher Scientific USA).
The cells (HeLa, HT-29 and MCF-7) were plated at a density of 1 × 105 cells per well in polystyrene, flat bottom 96-well microtiter plates (Corning Costar, Rochester, NY, USA) in DMEM containing 10% FBS and allowed to adhere overnight in a CO2 incubator at 37 °C. At the end of the incubation, the cells were treated with different concentrations of metamizole (0, 15, 90, 150, 300 and 400 µg/mL) in a final volume of 100 μL/well in triplicate wells for each treatment for 24 h or 48 h at 37 °C in a 5% CO2 incubator. All studies were repeated at least twice. Control cells were grown in DMEM containing 10% FBS and allowed to stabilize overnight in a CO2 incubator at 37 °C and left without treatment.
MTT test
The MTT (3-(4,5-dimethylthiazol-2-yl)-2,5-diphenyl tetrazolium bromide) test is colorimetric assay first described by Mosmann [Citation17]. It is based on the reduction of yellow tetrazolium salt MTT to purple formazan by the mitochondrial enzymes of viable cells. The amount of the formed formazan was measured spectrophotometrically at 540 nm and 620 nm (as a referent) wavelength by an ELISA spectrophotometer (TECAN, SunriseTM, Grödig/Salzburg, Austria) and the cell vitality was calculated using the following formula: CV (%) = [OD540 (test)/OD540 (control)] × 100.
Wound-healing assay (scratch assay)
The wound-healing assay (scratch assay) was used for evaluation of the effect of metamizole on the tumour cell migration [Citation18]. The wound-healing test analyses the filling of a wound (created mechanically by making a scratch) in the cell monolayer. The filling of the cut with proliferating cells migrating from the periphery to the centre was observed at equal time intervals and was captured by a camera. The area was quantified. For the evaluation of cell migration, we used concentrations of metamizole (HeLa - 90 µg/mL, HT-29 - 150 µg/mL and MCF-7 - 15 µg/mL) that are approximately equal to half of the IC50 value (half maximal inhibitory concentration) measured at the 48th hour by the MTT test.
Twenty-four hours before the experiment, the three cell lines were grown in 24-well cell culture plates at a concentration of 2.5 × 105 cell/mL (1 mL/well) in DMEM medium supplemented with 10% FBS, at 5% СО2, 37°С and 95% humidity to form a monolayer. In the day of the experiment, a vertical wound down through the cell monolayer (volume 100 µL) was made and the cultures were treated with metamizole (1 mL of DMEM plus 10% FBS with the desired concentration of metamizole for each cell line). The cell cultures were then incubated under standard conditions as described above. At several time points (0 h, 24 h, 48 h and 72 h), snapshots were taken with an inverted microscope Olympus with a digital camera to evaluate the degree of cell migration (based on the measured area). Control cells from the corresponding cell line were grown without treatment.
Fluorescence microscopy detection of apoptosis
Acridine orange/ethidium bromide staining
The cytomorphology changes in the tumour cells cultured in the presences of metamizole were studied after double intravital staining with fluorescence acridine orange (AO) and ethidium bromide (EtBr) according to the standard procedure [Citation19].
The tumour cells in a concentration of 1 × 105 cells/mL were seeded on sterile glass lamellas placed on the bottom of 24-well plates in DMEM suplemented with 10% FBS and were grown for 24 h in CO2 to form a monolayer. On the next day, the cells were treated with metamizole in concentrations equal to ІС50 measured at the 24th hour in the MTT test (400 µg/mL in HeLa, 300 µg/mL in HT-29 and 400 µg/mL in MCF-7) and were grown in CO2 in standard conditions for another 24 h. Control cells from the corresponding cell line were grown without treatment. After the incubation period, the cell lines were washed twice with phosphate buffered physiological solution (PBS) to remove the non-adherent cells. Equal volumes of fluorescence dyes, prepared ex tempore containing acridine orange (10 μg/mL) and ethidium bromide (10 μg/mL) were used. The freshly stained tumour cells were evaluated and photographed for a period of 10 minutes under a fluorescence microscope (Leica DM 5000B, Wetzlar, Germany) before the fluorescence staining started to fade.
Acridine orange stains both live and dead cells, emitting strong yellow-green fluorescence, as a result of the intercalation in the double-strained DNA, as well as red-orange fluorescence resulting from the binding with single-strained RNA. EtBr is a fluorochrome that passes through the damaged membranes and after intercalation in DNA emits orange fluorescence. EtBr stains dead cells and late apoptotic cells, which have altered cell permeability.
DAPI staining
The cell lines were stained with the fluorescent dye DAPI to assess the nuclear morphology. DAPI staining is a reliable method to observe apoptotic changes in the cell nucleus. DAPI is highly selective for binding with the clusters of DNA rich in adenine and thymine but has lower binding affinity to RNA. The molecule of DAPI can pass through intact cytoplasmic membrane, which makes it a useful agent for studying the nucleus morphology of live as well as fixed cells. Typical signs of apoptosis of the nucleus are condensation and margination of nuclear chromatin, fragmentation of the nucleus and the formation of apoptotic bodies.
Cell lines in a concentration of 1 × 105 cells/mL in DMEM supplemented with 10% FBS, seeded in sterile glass lamellas placed on the bottom of 24-well plates were grown for 24 h to form a cell monolayer. On the next day, the cells were treated with metamizole in concentrations of 400 µg/mL in HeLa, 300 µg/mL in HT-29 and 400 µg/mL in MCF-7 cells, and were grown for another 24 h in standard conditions. Control cells from the corresponding cell line were grown without treatment. After 24 h, any non-adherent cells were removed by washing with PBS. The staining with DAPI was done after fixing the cells with methanol according to the manufacturer’s protocol. The glass lamellas with the stained cells were mounted with Mowiol medium on microscope slides and were stored in darkness until they were observed under a fluorescence microscope (Leica DM 5000B, Wetzlar, Germany).
Statistical analysis
The statistical significance of the results was assessed by analysis of variance (ANOVA), followed by post-hoc comparison test (Bonferroni) with the use of GraphPAD PRISM software, version 5 (GraphPad Software Inc., San Diego, USA). The data are presented as mean values with standard deviation (±SD). Probability values of p < .05 were considered statistically significant.
Results and discussion
Evaluation of the cell viability by MTT test
Several studies have shown that NSAIDs and COX-2 inhibitors suppress the proliferation and induce cell death in various cultured cell lines, as well as potentiate the cytotoxic effects of some antineoplastic drugs through COX-dependent and COX-independent mechanisms [Citation20–31]. In the present study, the results from the MTT test showed a concentration- and time-dependent antiproliferative effect of metamizole against HeLa, HТ-29 and MCF-7 tumour cells (). There was a statistically significant decrease (p < .01) in the cell viability of the treated tumour cells compared to the untreated group at the 24th hour in concentrations higher than 25 μg/mL. On the 48th hour, however, a decrease in the cell viability of the HeLa cell line was noticeable in metamizole concentrations higher than 12.5 μg/mL. In HТ-29 and MCF-7 cells, the effect of metamizole at the 48th hour was evident in all the tested concentrations. The IC50 values of metamizole on the 24th and 48th hour for the three cell lines were as follows: HeLa – 472.475 µg/mL and 183.401µg/mL; НТ-29 – 314.750 µg/mL and 316.765 µg/mL; MCF-7 – 561.792 µg/mL and 29.297 µg/mL, respectively.
Wound-healing assay (scratch assay)
The wound-healing assay studies the migration of a cell population through the speed of proliferation and gap closure. McDonald et al. [Citation32] measured celecoxib motility with the scratch assay on HT-29 cells, showing no significant percent of wound closure, whereas Li et al. [Citation33] indicated that celecoxib can cause a dose-dependent inhibition of the migration of the human pancreatic carcinoma, epithelial-like cell line PANC-1. Wynne and Djakiew [Citation34] demonstrated that decreased cell migration in a prostate cancer cell line (PC-3) treated with enantiomer R-flurbiprofen and ibuprofen is mediated by NSAID-activated gene-1 (Nag-1) protein and neurotrophin receptor p75NTR. Aspirin treatment also delayed the healing after scratching in a concentration-dependent manner in murine breast cancer cells (4T1) [Citation35]. Similar results were obtained with ibuprofen about the migration of human coronary artery smooth muscle cells (HCASMCs) [Citation36].
Based on the migration activity measured with the scratch wound-healing assay, the lowest migration potential was observed in the HT-29 cell line compared to HeLa and MCF-7 tumour cells. This study showed for the first time that metamizole affected the migration of HeLa, HT-29 and MCF-7 human tumour cells. Metamizole demonstrated statistically significant inhibition of the migration compared to the corresponding control at the 24th hour of treatment in HeLa cells (60.79 ± 1.21% for metamizole-treated cells and 88.92 ± 2.69% for the control); at the 48th hour in HT-29 cells (17.87 ± 1.07% for metamizole-treated cells and 25.81 ± 1.79% for the control) and at the 48th and the 72nd hour in MCF-7 cells (82.86 ± 1.29% and 95.96 ± 1.81% for metamizole-treated cells and 86.49 ± 1.29% and 100% for the control). The migration of HeLa cells at the 48th and 72nd hour, that of HT-29 cells at the 24th and the 72nd hour, and of MCF-7 cells at the 24th hour was not significantly reduced compared to the controls (). Overall, metamizole inhibited the migration of the three tumour cell lines, but the effect was statistically significant compared to the control in the HeLa cells at the 24th hour, in the HT-29 cells at the 48th hour and MCF-7 at the 48th and 72nd hour of treatment ().
Figure 2. Effect of metamizole (½ IC50) on the migration of human tumour cells 0, 24, 48 and 72 hours after treatment. Wound-healing assay (left panel). Quantification of the migration (right panel). Metamizole concentrations were 90 µg/mL in HeLa, 150 µg/mL in HT-29 and 15 µg/mL in MCF-7 cells. Pictures were taken with inverted microscope Olympus; magnification 10х. The data are mean values ± SD from three independent experiments. * p < .05; **р < .01; *** p <.001 compared to the control.
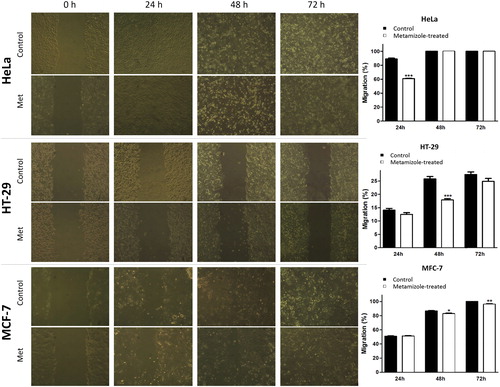
Fluorescence detection of apoptosis
Apoptosis of mammalian cells is accompanied by various morphological changes including cell shrinkage, cytoplasm and chromatin condensation, degradation of nuclear DNA into oligonucleosomal fragments and release of signalling molecules that attract phagocytes [Citation37–39]. Apoptosis can be visualized using different dyes. Acridine orange is an acidophilic dye that accumulates in areas of high lysosomal and phagocytotic activity, whereas ethidium bromide is an intercalating agent used to visualize DNA [Citation39].
Acridine orange/ethidium bromide staining
The control cells developed normal morphology and monolayer growth typical of the corresponding cell type (). Treatment with metamizole (400 µg/mL for HeLa, 300 µg/mL for HT-29 and 400 µg/mL for MCF-7 cells) resulted in the appearance of early apoptotic cells with bright green nuclei with chromatin condensation in the form of intense green areas and late apoptotic cells with orange nuclei with chromatin condensation. Typical signs of apoptosis were observed, such as cell shrinking, cell wrinkling, nucleus fragmentation and apoptotic bodies ().
Figure 3. Fluorescence microscopy of human tumour cells after 24 h treatment with metamizole. HeLa control (a); HeLa metamizole 400 µg/mL (b); HT-29 control (c); HT-29 metamizole 300 µg/mL (d); MCF-7 control (e); MCF-7 metamizole 400 µg/mL (f). Magnification 40Х; AO/EtBr staining.
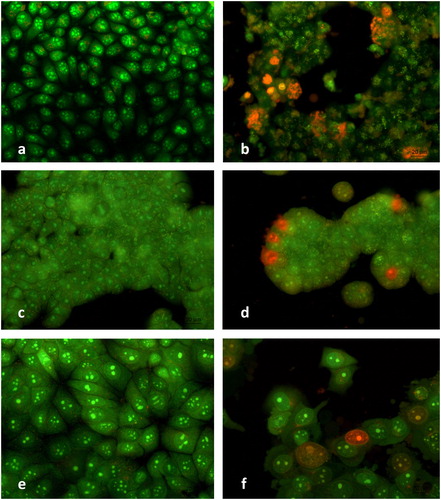
Metamizole induced marked alterations in the growth and morphology of the HeLa cells. The cell monolayer was damaged; the cells were oval to polygonal and early apoptotic cells (shrunk oval cells with condensation of the chromatin) were observed. Those at late apoptosis were predominant, characterized with cell blеbbing (blistering of the cell membrane), chromatin condensation and margination, nuclear fragmentation and apoptotic bodies. The presence of dead and destructed cells with pycnotic nuclei was indicative of late apoptosis and significant metamizole-induced cytotoxic effect (). The antineoplastic effect of metamizole was less pronounced in HT-29 compared to HeLa cells. There were morphologically altered cells with signs of early apoptosis (bright green nuclei with chromatin condensation in the form of dense green areas) and single cells with signs of late apoptosis (a bright orange coloured nucleus with condensed, aggregated and fragmented chromatin) (). The metamizole treatment of the MCF-7 cells resulted in the appearance of cells in the early stages of apoptosis (green nuclei with chromatin condensation) as well as single cells with signs of late apoptosis (bright orange stained nuclei with margination and chromatin condensation) and cells with typical signs of apoptosis - cell blеbbing, fragmentation of the nuclei and apoptotic bodies ().
The results demonstrated that the tested HeLa, HT-29 and MCF-7 tumour cells were sensitive to metamizole. They showed different degrees of morphological and growth changes, indicative of an early or late apoptosis, after metamizole treatment. Some of the signs of metamizole-induced apoptotic cell death included bright green nuclei with condensation of the chromatin appearing as intense green areas (early apoptotic cells) and orange nuclei with condensation and chromatin margination (late apoptotic cells). These findings are in accordance with the MTT test results and indicate that the induction of apoptosis could be one of the antineoplastic mechanisms of metamizole.
DAPI staining
We used DAPI staining to analyse the morphological alterations in the nucleus of metamizole treated cells (concentrations of 400 µg/mL, 300 µg/mL and 400 µg/mL, corresponding to HeLa, HT-29 and MCF-7 cells) observed at the 24th hour (). The non-treated controls of HeLa, HT-29 and MCF-7 cells had intact nuclei, equal in shape and size, with smooth edges and homogenously spread chromatin (). In the control MCF-7 cultures, cells in the phase of mitosis were also observed (). On the contrary, the treatment of HeLa, HT-29 and MCF-7 cells with metamizole led to significant alterations in the nucleus morphology typical of induction of cell death by the pathway of apoptosis: blebbing, pyknosis, nucleus fragmentation and granulated apoptotic bodies.
Figure 4. Fluorescence microscopy of human tumour cells after 24 h treatment with metamizole. HeLa control (a); HeLa metamizole 400 µg/mL (b); HT-29 control (c); HT-29 metamizole 300 µg/mL (d); MCF-7 control (e); MCF-7 metamizole 400 µg/mL (f). Magnification 40Х, DAPI staining.
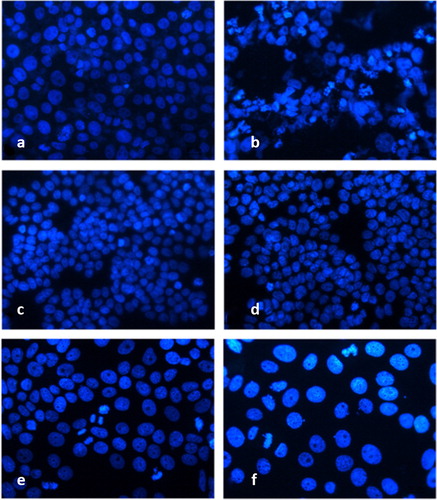
The observed morphological changes in HeLa cells after the treatment with metamizole had clear signs of apoptosis: different shape and size, with clear fragmentation of the nucleus, condensation of the chromatin and multiple apoptotic bodies (). In the HT-29 cells treated with metamizole, there were nuclei with uneven edges, condensed chromatin and single fragmented nuclei (). The nuclei of metamizole treated MCF-7 cells were single and fragmented, increased in size with condensed chromatin, and multiple apoptotic bodies ().
There is scarce information in the literature on any anticancer properties of metamizole. NSAIDs are best studied as chemopreventive agents in colorectal cancer [Citation40–42]; however, the concentrations of NSAIDs used there are much higher than those required for COX inhibition. Several molecular COX-dependent and COX-independent mechanisms of antineoplastic effects of NSAIDs have been proposed, suggesting that complex cellular and molecular pathways are involved in induction of apoptosis, autophagy, inhibition of proliferation and prevention of cancers [Citation43–45]. The exact mechanism depends on the cancer type and particular NSAID. NSAIDs are a structurally diverse group of chemical compounds. The amphiphilic nature of NSAIDs allows them to interact with lipid membranes, modulate the membrane biomechanical properties and cell signalling pathways [Citation46].
There are literature data suggesting that NSAIDs could reduce the risk of several common cancers [Citation47–51]. However, there is no definite evidence that the pre-diagnostic use of NSAIDs is associated with higher survival of cancer patients [Citation52–55].
To date, several pyrazolone derivatives have been shown to possess promising antiproliferative activity in human cancer cell lines [Citation56–61]. Metamizole is pyrazolone derivative with pronounced analgesic, antipyretic, spasmolytic properties and favourable gastro-intestinal safety profile [Citation62,Citation63]. The drug also possesses weak anti-inflammatory and mild antiaggregant activities [Citation64]. However, metamizole is banned in many countries due to bone marrow toxicity [Citation65].
Herein, the antitumour effects of metamizole on three types of human tumour cell lines (HeLa, HT-29 and MCF-7) were investigated. Metamizole has central as well as peripheral mechanisms of action that do not solely rely on cyclooxygenase (COX) inhibition. In the literature there are diverse data considering COX expression in different cell lines [Citation66–69]. HeLa, HT-29 and MCF-7 cell lines have high expression of COX-1, but relatively low of COX-2 [Citation70–72]. Metamizole and its metabolites have been shown to inhibit COX-1 and COX-2 activity by a different pathway than the classical aspirin-like NSAIDs mechanism [Citation73]. The complex mechanism of action of metamizole is still obscure, as it is not a typical NSAID, but is rather classified as a non-opioid analgesic.
Conclusions
In the present study, HeLa, HT-29 and MCF-7 human tumour cells were sensitive to metamizole as observed after the 24th and the 48th hour of the study. Concentrations higher than 25 μg/mL significantly decreased the viability/proliferation of HeLa, HT-29 and MCF-7 human tumour cells in in vitro tests as measured by the MTT assay, with a better effect observed at the 48th hour of treatment. Based on the IC50 values at the 48th h, the MCF-7 cells (human breast adenocarcinoma cells) were most sensitive, followed by HeLa (human cervical cancer cells) and HT-29 (human colorectal adenocarcinoma cell line). The antiproliferative effect of metamizole was achieved at least partly by induction of apoptosis as observed by fluorescence. Dual AO/EtBr staining enabled the observation of cells in early and late apoptosis (cell membrane blebbing with condensation and margination of chromatin, nuclear fragmentation and apoptotic bodies) after metamizole treatment. DAPI staining also indicated morphological changes typical of apoptotic cell death (aggregated and fused chromatin, fragmented nuclei). Metamizole induced significant suppression of cell migration in HeLa, HT-29 and MCF-7 tumour lines. According to the migration activity in the scratch wound-healing assay, HeLa cells had the highest migration potential, it was medium for MCF-7 and low for HT-29. Our study demonstrated a high anti-proliferative effect of metamizole achieved by induction of cell death via the apoptotic pathway. There was decreased migrability in all three types of studied human tumour cell lines. The obtained results warrant further research on the anticancer properties of metamizole to unravel the biological targets of carcinogenesis and mechanisms for the tumour suppressing activity of NSAIDs.
Abbreviations | ||
AO | = | acridine orange |
ATCC | = | American Type Cultures Collection |
COX | = | cyclooxygenase |
DAPI | = | 4′,6-diamidino-2-phenylindole |
DMEM | = | Dulbecco's Modified Eagle's Medium |
DNA | = | deoxyribonucleic acid |
EtBr | = | ethidium bromide |
FBS | = | fetal bovine serum |
HCASMCs | = | human coronary artery smooth muscle cells |
Nag-1 | = | NSAID-activated gene-1 |
NSAIDs | = | non-steroidal anti-inflammatory drugs |
PBS | = | phosphate buffered physiological solution |
RNA | = | ribonucleic acid |
Disclosure statement
No potential conflict of interest was reported by the authors.
Additional information
Funding
References
- Thun MJ. Aspirin, NSAIDs, and digestive tract cancers. Cancer Metastasis Rev. 1994;13(3):269–277.
- Harris RE, Kasbari S, Farrar WB. Prospective study of nonsteroidal anti-inflammatory drugs and breast cancer. Oncol Rep. 1999;6(1):71–74.
- Marx J. Anti-inflammatories inhibit cancer growth–but how? Science. 2001;291(5504):581–582.
- Thun MJ. NSAID use and decreased risk of gastrointestinal cancers. Gastroenterol Clin North Am. 1996;25(2):333–348.
- Gu M, Nishihara R, Chen Y, et al. Aspirin exerts high anti-cancer activity in PIK3CA-mutant colon cancer cells. Oncotarget. 2017;8(50):87379–87389.
- Piazza GA, Alberts DS, Hlzson LJ, et al. Sulindac sulfone inhibits azoxymethane-induced colon carcinogenesis in rats without reducing prostaglandin levels. Cancer Res. 1997;57:2909–2915.
- Chan TA, Morin PJ, Vogelstein B, et al. Mechanisms underlying nonsteroidal antiinflammatory drug-mediated apoptosis. P Natl Acad Sci USA. 1998;95(2):681–686.
- Shiff SJ, Koutsos MI, Qiao L, et al. Nonsteroidal antiinflammatory drugs inhibit the proliferation of colon adenocarcinoma cells: effects on cell cycle and apoptosis. Exp Cell Res. 1996;222(1):179–188.
- Nikolova I, Tencheva J, Voinikov J, et al. Metamizole: a review profile of a well-known “forgotten” drug. Part I: pharmaceutical and nonclinical profile. Biotechnol Biotechnol Equip. 2012;26(6):3329–3337.
- Nikolova I, Petkova V, Tencheva J, et al. Metamizole: a review profile of a well-known “forgotten” drug. Part II: clinical Profile. Biotechnol Biotechnol Equip. 2013;27(2):3605–3619.
- Malsy M, Graf B, Bundscherer A. Effects of metamizole, MAA, and paracetamol on proliferation, apoptosis, and necrosis in the pancreatic cancer cell lines PaTu 8988 t and Panc-1. BMC Pharmacol Toxico. 2017 [cited 2018 Feb 01];18(1):77. DOI: 10.1186/s40360-017-0185-y
- Bundscherer AC, Malsy M, Gruber MA, et al. Acetaminophen and metamizole induce apoptosis in HT 29 and SW 480 colon carcinoma cell lines in vitro. Anticancer Res. 2018;38(2):745–751.
- Shao J, Feng G. Selective killing effect of oxytetracycline, propafenone and metamizole on A549 or Hela cells. Chinese J Cancer Res. 2013;25(6):662–670.
- Shiang J-C, Jan R-L, Tsai M-K, et al. Dipyrone & 2, 5-dimethylcelecoxib suppress Th2-related chemokine production in monocyte. Indian J Med Res. 2014;140(1):109–115.
- De Luna-Bertos E, Ramos-Torrecillas J, García-Martínez O, et al. Therapeutic doses of nonsteroidal anti-inflammatory drugs inhibit osteosarcoma MG-63 osteoblast-like cells maturation, viability, and biomineralization potential. Sci World J. 2013;2013:809891.
- Zhang Y, Wang X, Baranov SV, et al. Dipyrone inhibits neuronal cell death and diminishes hypoxic/ischemic brain injury. Neurosurgery. 2011;69(4):942–956.
- Mosmann T. Rapid colorimetric assay for cellular growth and survival: application to proliferation and cytotoxicity assays. J Immunol Methods. 1983;65(1-2):55–63.
- Hulkower KI, Herber RL. Cell migration and invasion assays as tools for drug discovery. Pharmaceutics. 2011;3(1):107–124.
- Abdel-Wahab SI, Abdul AB, Mohan S, et al. Oncolysis of breast, liver and leukemia cancer cells using ethyl acetate and methanol extracts of Goniothalamus umbrosus. Res J Biol Sci. 2009;4(2):209–215.
- Wang G, Li J, Zhang L, et al. Celecoxib induced apoptosis against different breast cancer cell lines by down-regulated NF-κB pathway. Biochem Bioph Res Co. 2017;490(3):969–976.
- Li Q, Peng J, Liu T, et al. Effects of celecoxib on cell apoptosis and Fas, FasL and Bcl‐2 expression in a BGC‐823 human gastric cancer cell line. Exp Ther Med. 2017;14(3):1935–1940.
- Piazuelo E, Esquivias P, De Martino A, et al. Acetylsalicylic acid exhibits antitumor effects in esophageal adenocarcinoma cells in vitro and in vivo. Digest Dis Sci. 2016;61(10):2896–2907.
- Pang LY, Argyle SA, Kamida A, et al. The long-acting COX-2 inhibitor mavacoxib (Trocoxil™) has anti-proliferative and pro-apoptotic effects on canine cancer cell lines and cancer stem cells in vitro. BMC Vet Res. 2014 [cited 2018 Feb 01];10(1):184. DOI: 10.1186/s12917-014-0184-9
- Leidgens V, Seliger C, Jachnik B, et al. Ibuprofen and diclofenac restrict migration and proliferation of human glioma cells by distinct molecular mechanisms. PloS one. 2015;10(10):e0140613.
- Roller A, Bähr OR, Streffer J, et al. Selective potentiation of drug cytotoxicity by NSAID in human glioma cells: the role of COX-1 and MRP. Biochem Bioph Res Co. 1999;259(3):600–605.
- Roh J-L, Kim EH, Jang H, et al. Aspirin plus sorafenib potentiates cisplatin cytotoxicity in resistant head and neck cancer cells through xCT inhibition. Free Radical Bio Med. 2017;104:1–9.
- Barbarić M, Kralj M, Marjanović M, et al. Synthesis and in vitro antitumor effect of diclofenac and fenoprofen thiolated and nonthiolated polyaspartamide-drug conjugates. Eur J Med Chem. 2007;42(1):20–29.
- Yamazaki R, Kusunoki N, Matsuzaki T, et al. Selective cyclooxygenase‐2 inhibitors show a differential ability to inhibit proliferation and induce apoptosis of colon adenocarcinoma cells. FEBS letters. 2002;531(2):278–284.
- Bock JM, Menon SG, Sinclair LL, et al. Celecoxib toxicity is cell cycle phase specific. Cancer Res. 2007;67(8):3801–3808.
- Sánchez-Alcázar JA, Bradbury DA, Pang L, et al. Cyclooxygenase (COX) inhibitors induce apoptosis in non-small cell lung cancer through cyclooxygenase independent pathways. Lung Cancer. 2003;40(1):33–44.
- Dyakova L, Culita DC, Zhivkova T, et al. 3d metal complexes with meloxicam as therapeutic agents in the fight against human glioblastoma multiforme and cervical carcinoma. Biotechnol Biotechnol Equip. 2015;29(6):1190–1200.
- McDonald BF, Quinn AM, Devers T, et al. In‐vitro characterisation of a novel celecoxib microbead formulation for the treatment and prevention of colorectal cancer. J Pharm Pharmacol. 2015;67(5):685–695.
- Li S, Gu Z, Xiao Z, et al. Anti-tumor effect and mechanism of cyclooxygenase-2 inhibitor through matrix metalloproteinase 14 pathway in PANC-1 cells. Int J Cli Exp Patho. 2015;8(2):1737–1742.
- Wynne S, Djakiew D. NSAID inhibition of prostate cancer cell migration is mediated by Nag-1 induction via the p38 MAPK-p75NTR pathway. Molec Cancer Res. 2010;8(12):1656–1664.
- Hsieh C-C, Huang Y-S. Aspirin breaks the crosstalk between 3T3-L1 adipocytes and 4T1 breast cancer cells by regulating cytokine production. PloS one. 2016 [cited 2018 Feb 01];11(1):e0147161. DOI: 10.1371/journal.pone.0147161
- Dannoura A, Giraldo A, Pereira I, et al. Ibuprofen inhibits migration and proliferation of human coronary artery smooth muscle cells by inducing a differentiated phenotype: role of peroxisome proliferator‐activated receptor γ. J Pharma Pharmacol. 2014;66(6):779–792.
- He B, Lu N, Zheng Zhou Z. Cellular and Nuclear Degradation during Apoptosis. Curr Opin Cell Biol. 2009;21(6):900–912.
- Bonanno E, Tagliafierro G, Carlà EC, et al. Synchronized onset of nuclear and cell surface modifications in U937 cells during apoptosis. Eur J Histochem. 2002;46(1):61–74.
- Elmore S. Apoptosis: a review of programmed cell death. Toxicol Pathol. 2007;35(4):495–516.
- Wang D, DuBois RN. The role of anti-inflammatory drugs in colorectal cancer. Annu Rev Med. 2013;64:131–144.
- Veettil SK, Lim KG, Ching SM, et al. Effects of aspirin and non-aspirin nonsteroidal anti-inflammatory drugs on the incidence of recurrent colorectal adenomas: a systematic review with meta-analysis and trial sequential analysis of randomized clinical trials. BMC Cancer. 2017;17(1):763. DOI: 10.1186/s12885-017-3757-8
- Chan AT, Arber N, Burn J, et al. Aspirin in the chemoprevention of colorectal neoplasia: an overview. Cancer Prev Res. 2012;5(2):164–178.
- Umar A, Steele VE, Menter DG, et al. Mechanisms of nonsteroidal anti-inflammatory drugs in cancer prevention. Semin Oncol. 2016;43(1):65–77.
- Gurpinar E, Grizzle WE, Piazza GA. NSAIDs inhibit tumorigenesis, but how? Clin Cancer Res. 2014;20(5):1104–1113.
- Gurpinar E, Grizzle WE, Piazza GA. COX-independent mechanisms of cancer chemoprevention by anti-inflammatory drugs. Front Oncol. 2013;3:181. DOI: 10.3389/fonc.2013.00181
- Zhou Y, Hancock JF, Lichtenberger LM. The nonsteroidal anti-inflammatory drug indomethacin induces heterogeneity in lipid membranes: potential implication for its diverse biological action. PLoS One. 2010 [cited 2018 Feb 01];5(1):e8811. DOI: 10.1371/journal.pone.0008811
- Yiannakopoulou EC. Aspirin and NSAIDs for breast cancer chemoprevention. Eur J Cancer Prev. 2015;24(5):416–421.
- Sostin N, He S, Sostin O, et al. NSAIDs Use after Breast Cancer Diagnosis Improves Relapse-Free and Overall Survival: Retrospective Pilot Study. Am J Cli Patho. 2016;146(suppl_1):S139–S140.
- Vidal AC, Howard LE, Moreira DM, et al. Aspirin, NSAIDs, and risk of prostate cancer: results from the REDUCE study. Cli Cancer Res. 2015;21(4):756–762.
- Muranushi C, Olsen CM, Green AC, et al. Can oral nonsteroidal antiinflammatory drugs play a role in the prevention of basal cell carcinoma? A systematic review and metaanalysis. J Am Acad Dermatol. 2016;74(1):108–119.
- Rothwell PM, Fowkes FG, Belch JF, et al. Effect of daily aspirin on long-term risk of death due to cancer: analysis of individual patient data from randomised trials. Lancet. 2011;377(9759):31–41.
- Nagle CM, Ibiebele TI, DeFazio A, et al. Aspirin, nonaspirin nonsteroidal anti-inflammatory drugs, acetaminophen and ovarian cancer survival. Cancer Epidemiol. 2015;39(2):196–199.
- Brøns N, Baandrup L, Dehlendorff C, et al. Use of nonsteroidal anti-inflammatory drugs and risk of endometrial cancer: a nationwide case–control study. Cancer Causes Control. 2015;26(7):973–981.
- Verdoodt F, Friis S, Dehlendorff C, et al. Non-steroidal anti-inflammatory drug use and risk of endometrial cancer: a systematic review and meta-analysis of observational studies. Gynecol Oncol. 2016;140(2):352–358.
- Tang L, Hu H, Liu H, et al. Association of nonsteroidal anti-inflammatory drugs and aspirin use and the risk of head and neck cancers: a meta-analysis of observational studies. Oncotarget. 2016 [cited 2018 Feb 01];7(40):65196. DOI: 10.18632/oncotarget.11239
- Kakiuchi Y, Sasaki N, Satoh-Masuoka M, et al. A novel pyrazolone, 4, 4-dichloro-1-(2, 4-dichlorophenyl)-3-methyl-5-pyrazolone, as a potent catalytic inhibitor of human telomerase. Biochem Bioph Res Co. 2004;320(4):1351–1358.
- Brana MF, Gradillas A, Ovalles AG, et al. Synthesis and biological activity of N, N-dialkylaminoalkyl-substituted bisindolyl and diphenyl pyrazolone derivatives. Bioorg Med Chem. 2006;14(1):9–16.
- Ohki H, Hirotani K, Naito H, et al. Synthesis and mechanism of action of novel pyrimidinyl pyrazole derivatives possessing antiproliferative activity. Bioorg Med Chem Lett. 2002;12(21):3191–3193.
- Wang X, Wang X, Liang Y, et al. A cell-based screen for anticancer activity of 13 pyrazolone derivatives. Chin J Cancer. 2010;29(12):980–987.
- Zhao B, Shang X, Xu L, et al. Novel mixed ligand di-n-butyltin (IV) complexes derived from acylpyrazolones and fluorinated benzoic acids: Synthesis, characterization, cytotoxicity and the induction of apoptosis in Hela cancer cells. Eur J Med Chem. 2014;76:87–97.
- Salama SK, Mohamed MF, Darweesh AF, et al. Molecular docking simulation and anticancer assessment on human breast carcinoma cell line using novel bis (1, 4-dihydropyrano [2, 3-c] pyrazole-5-carbonitrile) and bis (1, 4-dihydropyrazolo [4′, 3′: 5, 6] pyrano [2, 3-b] pyridine-6-carbonitrile) derivatives. Bioorg Chem. 2017;71:19–29.
- Jasiecka A, Maślanka T, Jaroszewski J. Pharmacological characteristics of metamizole. Pol J Vet Sci. 2014;17(1):207–214.
- Konijnenbelt‐Peters J, Heijden C, Ekhart C, et al. Metamizole (Dipyrone) as an alternative agent in postoperative analgesia in patients with contraindications for nonsteroidal anti‐inflammatory drugs. Pain Practice. 2017;17(3):402–408.
- Lampl C, Likar R. Metamizole (dipyrone): mode of action, drug-drug interactions, and risk of agranulocytosis. Schmerz (Berlin, Germany). 2014;28(6):584–590.
- Stamer UM, Gundert-Remy U, Biermann E, et al. Dipyrone (metamizole): considerations on monitoring for early detection of agranulocytosis. Schmerz. 2017;31(1):5–13.
- Zelenay S, van der Veen AG, Böttcher JP, et al. Cyclooxygenase-dependent tumor growth through evasion of immunity. Cell. 2015;162(6):1257–1270.
- Liu XH, Rose DP. Differential expression and regulation of cyclooxygenase-1 and -2 in two human breast cancer cell lines. Cancer Res. 1996;56(22):5125–5127.
- Yu DS, Chen HI, Chang SY. The expression of cyclooxygenase in transitional cell carcinoma cell lines: its correlation with tumor differentiation, invasiveness and prostanoids production. Eur Urol. 2003;44(4):491–494.
- Sugimoto T, Koizumi T, Sudo T, et al. Correlative expression of cyclooxygenase-1 (Cox-1) and human epidermal growth factor receptor type-2 (Her-2) in endometrial cancer. Kobe J Med Sci. 2007;53(5):177–187.
- Jeon Y-T, Song Y-C, Kim S-H, et al. Influences of cyclooxygenase-1 and-2 expression on the radiosensitivities of human cervical cancer cell lines. Cancer Lett. 2007;256(1):33–38.
- Liu C, Yang C, Lu L, et al. Luminescent iridium (III) complexes as COX-2-specific imaging agents in cancer cells. Chem Com. 2017;53(19):2822–2825.
- Buecher B, Bouancheau D, Broquet A, et al. Growth inhibitory effect of celecoxib and rofecoxib on human colorectal carcinoma cell lines. Anticancer Res. 2005;25(1A):225–233.
- Pierre S, Schmidt R, Brenneis C, et al. Inhibition of cyclooxygenases by dipyrone. Bri J Pharmacol. 2007;151(4):494–503.