Abstract
Strawberry (Fragaria ananassa Duch.) is an economically important crop. Its yield is highly dependent on photosynthesis, and carbon dioxide (CO2) is one of the most important constituents in photosynthesis. In this study, we investigated the physiological and molecular mechanisms of CO2 enrichment in increasing strawberry leaf growth, and ultimately fruit yield. Strawberry plants were grown under ambient CO2 (350–500 μmol/mol) and elevated CO2 (750–850 μmol/mol) in a controlled environment. The results showed that CO2 enrichment significantly increased the size, net photosynthetic rate and light saturation point of the leaves, but decreased the light compensation point of leaves. Using RNA sequencing (RNA-Seq), transcriptome profiling of leaf tissues under two CO2 concentrations was conducted. The analysis identified 150 differentially expressed genes in response to increased CO2 concentration; 14 of them were shown to be involved in photosynthesis. Gene Ontology (GO) enrichment and pathways analyses revealed that CO2 enrichment enhanced the biosysthesis of metabolism-promoting growth hormones; sped up the reduction step of CO2 assimilation, and increased the transport of photosynthetic products, which help explain the increased net photosynthetic rate and accelerated leaf growth under elevated CO2 level. These results provide a valuable reference for exploring the mechanism of CO2 application in strawberry production in greenhouses especially during offseason.
Introduction
Strawberry (Fragaria ananassa Duch.) is an economically important crop. The sweet taste, bright colour and rich nutrition make strawberry a popular fruit for human consumption [Citation1,Citation2]. At present, strawberry production is carried out largely in controlled environments especially greenhouses. During winter and spring seasons, lack of gas exchange in greenhouses may result in critical carbon dioxide (CO2) shortage, which greatly limits the photosynthetic rate and the yield, and could reduce the fruit quality. One solution to address this problem is the application of CO2 to increase plant growth and fruit yield [Citation3–5]. CO2 is the raw material of photosynthesis, by which plants convert solar energy into chemical energy, and simultaneously use inorganic carbon dioxide and water to synthesize organic matter and release oxygen, which are essential for most life in the biosphere [Citation6]. Many studies have investigated the physiological mechanisms by which CO2 enrichment promotes plant growth. Elevated CO2 increases the growth and leaf area in both C3 and C4 plants due to decreases in evapotranspiration, and stomatal conductance [Citation7]. In Arabidopsis thaliana, elevated CO2 can enhance photosynthesis in leaves, reduce stomatal density and electrical conductivity, improve plant quality and increase seed number [Citation8]. In soybean, elevated CO2 increases photosynthesis, growth rate, grain yield, and C/N rate [Citation9]. In rice and wheat, elevated CO2 can increase the thickness of leaves, accelerate the formation of the vascular system, and thereby enhance the transport of photosynthetic raw materials and promote photosynthesis [Citation10,Citation11]. Moreover, water use efficiency (WUE) and apparent quantum efficiency (AQE) of CO2 assimilation in rice are increased significantly in a high CO2 concentration environment. In leaves, the content of soluble sugars such as sucrose, fructose and glucose was increased, as well as the content of phosphorus (Pi) and light harvesting complex 2 (LHCII) in leaves, along with chlorophyll [Citation12].
Several studies examined the molecular mechanisms of elevated CO2 on plant growth and yield. In the triploid Populus tomentosa, the pyruvate kinase gene (PK) and the pyruvate decarboxylase gene (PDC) are up-regulated with increasing CO2 concentration [Citation13]. PK and PDC are enzymes that convert adenosine diphosphate (ADP) to adenosine triphosphate (ATP) during glycolysis, and provide energy for photosynthesis. The glutamate dehydrogenase gene (GDH) is also up-regulated. GDH provides carbon skeletons for the tricarboxylic acid (TCA) circle, thus increases the supply of energy and metabolites to support and promote photosynthesis [Citation13]. Under high CO2 concentrations, the photosynthetic rate of leaves in Arabidopsis thaliana was increased, and glucose-6-phosphate transmembrane transporter (GPT2) and sucrose synthase 3 genes (SUS3) were up-regulated. These two genes regulate the storage and transportation of source-sink metabolites, ensuring storage and conversion of photosynthetic products to maintain the photosynthetic efficiency [Citation14]. Elevated CO2 can inhibit light respiration, promote phenol antioxidation and enhance antioxidant enzyme activity, and increase production of secondary metabolites such as phenylpropanoids and tannins [Citation15].
RNA sequencing (RNA-Seq) has been used for analysis of transcriptome reprogramming under different CO2 conditions. For example, by comparing the transcript profiles in elevated CO2, Vicente et al. [Citation16] found that genes encoding the putative PSI P700 Chl A apoprotein A1 (PsaA), apoprotein A2 (PsaB) and PSII proteins D1 and D2 are up-regulated in durum wheat. In grape (Vitis vinifera L. cv. ‘Pinot Noir’), Zhao et al. [Citation17] conducted transcriptome profiling and found that the ribulose bisphosphate carboxylase small chain (RbcS) and rubisco activase (Rca) genes are up-regulated upon increased CO2 concentration, which promotes photosynthesis and accelerates transition of grape plantlets from heterotrophic to autotrophic.
In our previous study [Citation5], we found that CO2 enrichment can promote fruit growth and yield and improve fruit quality in strawberries, but the physiological and molecular mechanisms remain unknown. To understand the physiological and molecular mechanisms by which elevated CO2 affects strawberry growth, we performed comparative analyses of strawberry plants under normal and elevated CO2 concentrations in the greenhouses. The growth rate and photosynthetic indices in strawberry leaves under two different CO2 concentrations were measured. We also conducted transcriptome profiling of strawberry leaves under carbon-rich and ambient conditions by RNA-Seq. Differentially expressed genes (DEGs) were identified, enriched Gene Ontology (GO) functions and Kyoto Encyclopedia of Genes and Genomes (KEGG) metabolic pathways were analysed. Genes related to growth promotion under elevated CO2 were identified. The results from this comprehensive study laid a solid foundation for systematic elucidation of gene regulation in photosynthesis, provided an effective way to promote strawberry growth in a controlled environment, and could ultimately revolutionize the worldwide strawberry production as CO2 enrichment has done to many vegetable crops.
Materials and methods
Plant materials and cultivation
In this experiment, the strawberry variety 'Hongyan' was selected because it is one of the leading strawberry cultivars in China. Seedlings were provided by Shanxi 'Juxin Weiye' Agricultural Technology Development Co., Ltd., Taigu, Shanxi. Cultivation was carried out from 25 Oct 2016 to 05 Jan 2017 in the Chinese Solar Greenhouse of Shanxi Agricultural University gardening station (N37°, E112°). Plants were cultivated using the raised bed production method (bottom width 60 cm, top width 40 cm, height 35 cm). Row spacing was 1 m while the plant spacing was 30 cm × 30 cm arranged in a matted row system [Citation18]. Two drip tapes were laid on the raised beds and were 10 cm from each edge. Honeybees were used to pollinate the flowers during bloom.
Application of CO2
For most plants, the ideal CO2 concentration is 800 μmol/mol according to previous research results. Higher CO2 concentrations beyond the optimum hinder plant growth and thus reduce crop yield [Citation19]. Therefore, we selected 750–850 μmol/mol as carbon rich rather than higher concentration. The Chinese Solar Greenhouse used in this study was divided into carbon-rich (750–850 μmol/mol) and ambient CO2 (350–450 μmol/mol) areas separated by a wall of thin plastic film. Sixty plants in two rows were grown in each area. When plants began to bloom, CO2 was applied to the carbon-rich area from 7:30 am to 10:00 am every morning, and the concentration was controlled by a 'Greenhouse CO2 Automatic Control System' developed by Shengyan Electronic Scientific Technology Co., LTD., Handan, Hebei, China. CO2 was supplied through a series of polyvinyl chloride (PVC) pipes that are connected to a compressed CO2 cylinder. Three 8 m long CO2 releasing pipes with an inside diameter of 2 cm were mounted on the top of the Chinese Solar Greenhouse. Tiny openings of 0.5 cm in diameter were drilled on the underside of those pipes and were spaced 2 cm apart. The CO2 releasing pipes were connected to electric motors and were rotated continuously when CO2 was being supplied, thus ensuring the CO2 concentration in the elevated CO2 area remains elevated and uniform. Environmental data such as CO2 concentration, temperature and humidity in carbon-rich and ambient (control) areas of the greenhouse were automatically recorded at 10 min intervals.
Determination of leaf growth indices
Ten days after CO2-enrichment treatments, 10 pieces of young leaves with a similar vigour at the same position were tagged on carbon-rich and control plants. The petiole length, leaf width, and leaf length of tagged leaves were measured every 7 days until leaf growth stopped. Growth curves of petiole length, leaf length and leaf width were then plotted. There were three replicates in each dataset.
Determination of leaf photosynthesis indices
After carbon-rich treatment, the light response curves of normal and treated leaves were determined. Measurements were carried out between 9:30 am to 11:00 am on a sunny and calm day in January, 2017 when plants were actively growing. Three fully expanded leaves of comparable size were selected for each treatment. At the time when the measurements took place, the environmental conditions were as follows: air temperature: 25 °C; relative humidity: ∼60%; CO2 concentration was 800 μmol/mol under carbon-rich conditions, and 400 μmol/mol under ambient conditions. Photosynthetic indices were measured with the Li-6400XT Portable Photosynthesis System; A 2 × 3 light-emitting diode (LED) light source and a Li-6400 LI-COR liquefied CO2 cylinder (P/N 9964-037) were used for measurements. The light intensity was set as 20, 50, 100, 150, 200, 400, 600, 700, 800, 1000, 1200, 1400, 1600, 1800 and 9999 μmol/m2s, respectively for determining light response curves. Fifteen indices including net photosynthetic rate (NPR) were obtained after 3–5 min of adaptation to each light intensity. The light saturation point (LSP), light compensation point (LCP), maximum net photosynthesis rate (Amax) and apparent quantum efficiency (AQE) were obtained by fitting using LI-Cor software.
Transcriptome profiling of strawberry leaves under different CO2 concentrations
Sample preparation
Samples for RNA-Seq were collected 10 d after carbon-rich treatment. The environment conditions at 10 am (1.5 h after CO2 application) on the day of sample collection were: sunny day; air temperature: 29 °C, relative humidity: 53%. Three plants of comparable vigour were selected from control and carbon-rich environments, and the third leaf (inner to outer) of these were harvested, combined and sampled (sample weight 0.15 g with two samples per treatment, two biological replicates). Samples from carbon-rich conditions were coded as HYF-1 and HYF-2, while samples from ambient CO2 condition were coded as HYD-1 and HYD-2. Samples were immediately frozen in liquid nitrogen and stored at −80 °C for RNA extraction and transcriptome sequencing.
RNA extraction and analysis of transcriptome sequencing data
Strawberry leaf transcriptome sequencing was performed using an Illumina Hi-Seq 2500 platform by a commercial service at Biomarker Technologies Co., Ltd. (Beijing, China). In brief, total RNA was extracted from each sample, purified and mRNA was isolated and fragmented. Synthesis of the first-strand cDNA was performed using mRNA as template, followed by second-strand cDNA synthesis, end repair, addition of poly-A to the 3′ end, and attachment to the sequencing linker. Enrichment was carried out and the sequencing sample library was constructed for sequencing.
Raw data were obtained by sequencing, and the quality was evaluated. High quality reads were then aligned with the reference genome, and expression levels of matched genes were analysed statistically. DEGs were identified by comparing gene expression under different CO2 conditions, and subjected to GO function and KEGG metabolic pathway analyses to identify genes related to CO2 responses, and explore their functions and mechanisms.
Real-time quantitative polymerase chain reaction (RT-qPCR) analysis
The expression patterns of four selected strawberry genes (gene00630-v1.0-hybrid, gene19575-v1.0- hybrid, gene21583-v1.0-hybrid and gene30952-v1.0-hybrid) from RNA-Seq were verified by real-time quantitative polymerase chain reaction (RT-qPCR). Primers for RT-qPCR of each gene were designed using the Primer 3 online tool, and RT-qPCR were performed using a SYBR Premix Ex Taq II (Tli RNaseH Plus) kit (RR820A; TaKaRa); the strawberry ACTIN gene was used as an internal reference. Primer sequences of all five genes are presented in . Each 25-μL RT-qPCR reaction contained 20 ng of cDNA, and thermal cycling was performed with an initial denaturation at 94 °C for 1 min, followed by 40 cycles at 94 °C for 30 s, 55 °C for 30 s, and 72 °C for 30 s using an ABI 7500 real-time PCR instrument. Two biological replicates and two technical replicates were performed for each sample, and the relative expression levels were calculated by the 2−ΔΔCt method [Citation20].
Table 1. Primers used for real-time fluorescence quantitative PCR.
Results and discussion
Comparison of leaf growth under different CO2 concentrations
Dynamic curves of strawberry petiole length (), leaf width () and leaf length () under two CO2 concentrations are shown. Leaves grew faster during the early stages, then slower thereafter and eventually stopped growing. Petioles were significantly longer while the leaves were longer and wider under elevated CO2 conditions, indicating that CO2 enrichment significantly promoted the leaf growth of strawberry plants. These results are consistent to those from other previous studies by Bao and Zhang [Citation21] and Xin et al. [Citation22]. Elevated CO2 increased strawberry fruit yield at low temperature, but deceased it at high temperature, when compared to the corresponding treatments in ambient CO2 [Citation23]. For wheat, exposure to elevated CO2 significantly increased aboveground biomass and grain yield components [Citation24]. In addition, elevated CO2 significantly increased plant height, stem diameter and leaf area under drought stress in cucumber [Citation25]. Our results in strawberry were similar to these results.
Figure 1. Comparison of leaf growth in strawberry under elevated and ambient CO2 concentrations. Petiole length (a), leaf width (b) and leaf length (c) under two different CO2 levels. Note: Data are mean values with standard error (±SEM) of three independent experiments (n = 3) with at least three measurements for each replicate. *P < 0.05.
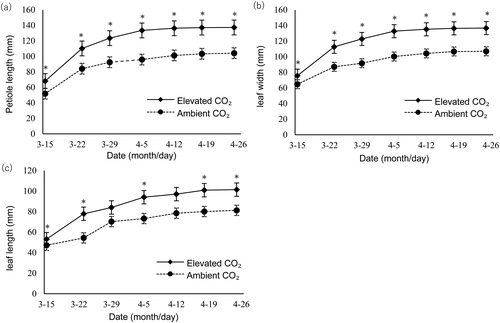
Comparison of photosynthesis indices in leaves under two CO2 concentrations
Light response curves were plotted under carbon-rich and control conditions using LI-Cor software (). The net photosynthetic rate gradually increased with increasing photosynthetic active radiation (PAR), but when it exceeded 500 μmol/m2s, the rate of increase became smaller, the curve gradually became less steep, and the light saturation point (LSP) was gradually reached. The LSP under carbon-rich condition was 1419.86 μmol/m2s, which was 1378.86 μmol/m2s at ambient CO2 condition, indicating that elevated CO2 concentration increased the LSP of strawberry leaves. The apparent quantum efficiency (AQE) reflects the light energy utilisation efficiency, which was higher under carbon-rich conditions (). This suggested that elevated CO2 improved the light energy utilisation rate of strawberry leaves. Comparison of photosynthetic response curves under carbon-rich and control conditions at the same PAR revealed that the maximum NPR of strawberry leaves under carbon-rich condition (28.21 μmol/m2s) was significantly higher than that under normal physiological condition (11.11 μmol/m2s). In mung bean, Gao et al. [Citation26] reported similar results: Pn (net photosynthetic rate) of upper most fully-expended leaves in mung bean was stimulated by 18.7 and 7.4% at elevated CO2 respectively at full bloom stage (R2) and R4 growth stages [Citation26]. Photosynthetic indices of strawberry leaves, such as NPR and LSP, were greatly improved under carbon-rich conditions. This explains why carbon-rich conditions can promote leaf growth from another point of view, indicating that photosynthetic rate and related metabolic processes are markedly enhanced by elevated CO2.
Figure 2. Comparison of light response curves of strawberry leaves under different CO2 concentrations.
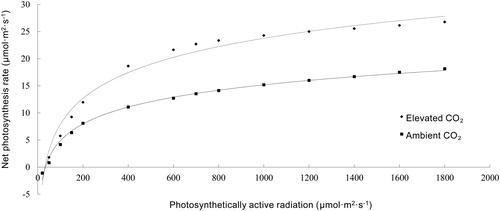
Table 2. Comparison of photosynthetic parameters of strawberry leaves under elevated and ambient CO2 concentrationsTable Footnote*.
Effects of elevated CO2 on the strawberry leaf transcriptome
Evaluation of sequencing data quality
Four libraries (HYD-1, HYD-2, HYF-1 and HYF-2) were subjected to transcriptome sequencing, and the total number of reads from HYD-1, HYD-2, HYF-1 and HYF-2 were 60,118,096, 57,630,836, 57,697,614 and 68,370,330, respectively (). Among the raw reads, 42.23%, 42.15%, 42.35% and 43.00% could be mapped to the wild, diploid strawberry (F. vesca) draft genome, of which 41.66%, 41.64%, 41.80% and 42.43%, were uniquely mapped reads, respectively (i.e. reads mapped to unique locations of the reference genome). Multiple mapped reads (the number of reads aligned to multiple locations in the reference genome) accounted for 0.57%, 0.51%, 0.55% and 0.57%, respectively (). Despite of the polyploidy nature of the strawberry variety we used, the RNA-Seq statistics shown in suggested that the dataset was reasonably good for subsequent analysis.
Table 3. RNA-Seq data statistics.
Identification of DEGs in strawberry leaves under different CO2 conditions
DEGs were identified between reads mapped to the wild strawberry genome under natural conditions (HYD-1, HYD-2) and carbon-rich conditions (HYF-1, HYF-2). Fragments per kilobase of transcript per million mapped reads (FPKM) were used to represent relative gene expression of transcripts. DEGs between the two CO2 conditions were defined as those with fold change (FC) ≥1.5 at a false discovery rate (FDR) <0.05. The numbers of up- or down-regulated DEGs in different FC ranges are presented in . Among the total 150 DEGs identified, 98 and 52 were up- and down-regulated, respectively. Of the 150, 132 exhibited 1.5–10-fold changes (86 up- and 46 down-regulated), and 13 exhibited 10–50-fold changes (8 up- and 5 down-regulated) ().
Table 4. Differentially expressed genes under different CO2 concentrations.
GO enrichment analysis of DEGs
Based on a corrected p-value <0.05, DEGs between carbon-rich and natural conditions were functionally classified using GO enrichment analysis. Of the 150 DEGs, 125 (81.7%) had GO annotations. Among the seven enriched GO functions (), four belong to biological processes, three molecular functions, but none belonged to cellular components. In terms of biological processes, genes involved in geranyl diphosphate metabolic process (GO:0033383) were the most enriched (six DEGs), among which four genes were related to biosynthesis of gibberellin (GA) that exhibited differential expression levels of >10-fold. The remaining enriched biological processes were farnesyl diphosphate metabolic process (GO:0045338), cadinene biosynthetic process (GO:1901928) and killing of cells of other organisms (GO:0031640). Regarding molecular function, three categories were enriched: terpene synthase activity (GO:0010333), haem binding (GO:0020037) and (+)-delta-cadinene synthase activity (GO:0047461). Genes related to haem binding were the most abundant (10 DEGs), which is important because Fe is an essential element for chlorophyll biosynthesis, and plays an important role in photosynthesis [Citation27]. There was no enrichment of cellular component categories.
Table 5. GO enrichment analysis of DEGs under different CO2 concentrations.
The direct products of photosynthesis in plants are primary metabolites such as sugars and fats, while secondary metabolites such as terpenoids, phenols and alkaloids are derived from organic molecules such as sugars. According to GO functional annotation, the expression levels of genes involved in the secondary metabolism in leaves were increased following carbon-rich treatment, which was reflected in the enrichment of geranyl diphosphate metabolic process, farnesyl diphosphate metabolic process and cadinene biosynthetic process categories, as well as the enrichment of terpene synthase activity and (+)-delta-cadinene synthase activity in the molecular function category. These terpene-based secondary metabolites constitute phytohormones such as auxins or gibberellins (GAs), photosynthetic pigments (carotenoids), anthocyanidins (volatile terpenes that can attract insect pollinators), and plant protection substances. Carotenoids are essential components for the formation of thylakoid membranes, and can combine with pigment protein complexes and photosynthetic reaction centers to transfer absorbed light energy to chlorophyll molecules, and thereby play an auxiliary and protective role in photosynthetic processes. In addition, terpenes can also play an important role in plant growth and development, heat tolerance and resisting photooxidative stress [Citation28].
Many genes exhibited more than 10-fold expression changes under different CO2 conditions. Under elevated CO2 concentration, the expression of 13 DEGs was altered >10-fold (eight and five up- and down-regulated, respectively); details of 11 DEGs are shown in including their Arabidopsis homologs and predicated functions. Two genes not presented in , Fragaria_vesca_newGene_256 and gene04191-v1.0- hybrid, did not have GO annotations.
Table 6. Representative genes with >10-fold expression change under carbon-rich condition in strawberry.
Gene gene32260-v1.0-hybrid was significantly up-regulated after elevated CO2 treatment. This gene was predicted to encode a type II NADPH dehydrogenase. NADPH is the energy currency for CO2 assimilation that converts CO2 into carbohydrates via dehydrogenation. Up-regulation of this gene under carbon-rich conditions indicated that a high CO2 concentration can increase the CO2 assimilation ability, and thereby enhance production of carbohydrates. The gene02096-v1.0-hybrid gene may be involved in the synthesis of aldehyde dehydrogenase, similar to its homolog in Arabidopsis. The most common aldehyde dehydrogenase is NADP-glyceraldehyde-3-phosphate dehydrogenase, which catalyses the conversion of 3-phosphoglycerate to glyceraldehydes-3-phosphate, and this is the key enzyme in CO2 assimilation during the photosynthetic process. Up-regulation of this gene suggested that the synthesis of carbohydrates was promoted under elevated CO2 conditions, too.
The gene15456-v1.0-hybrid homolog in Arabidopsis encodes a peroxidase superfamily protein, which is an important protective enzyme in plants [Citation29]. Peroxidases are involved in photorespiration in plants, and can oxidise glycolic acid, a by-product of photosynthesis, into acetaldehyde acid and hydrogen peroxide. Up-regulation of this enzyme under carbon-rich conditions can improve plant immunity, making plants more resistant to diseases and other abiotic stresses.
The four strawberry genes, gene12601-v1.0-hy-brid, gene19489-v1.0-hybrid, gene12094-v1.0 -hybrid and gene30965-v1.0-hybrid are all homologs of Arabidopsis terpene synthase 21 (TPS21) that encodes the terpene synthase. The function of AtTPS21 is still unclear, but studies have shown that AtTPS1 is mainly localised to chloroplasts, and it can improve the ability of plants to resist stress by stimulating photosynthesis [Citation30]. AtTPS1 also plays an important role in central metabolism and cell division.
The gene31308-v1.0-hybrid gene was expressed in chloroplasts in strawberry, which is predicted to encode pyridoxal phosphate (PLP)-dependent transferases. PLP is a coenzyme of transaminase and decarboxylase enzymes involved in amino acid metabolism.
Analysis of KEGG pathways of DEGs
KEGG annotation resulted in 56 DEGs annotated to 31 pathways (). The most enriched annotation pathway was plant hormone signal transduction (seven DEGs). Among them, GID1 (gene27756-v1.0-hybrid) participates in diterpenoid biosynthesis and is related to GA metabolism, and this gene was up-regulated. BZR1/2 (gene15105-v1.0-hybrid) participating in the brassinolide (BR) biosynthesis pathway was also up-regulated. The gene27842-v1.0-hybrid gene is related to zeatin biosynthesis, and its expression was 8-fold higher after carbon-rich treatment. Previous studies have shown that the concentrations of growth-promoting hormones, such as GAs, Zeatin riboside (ZR) and indole-3-acetic acid (IAA), increased with elevated CO2 concentration, whereas the concentration of hormones inhibiting plant growth such as abscisic acid (ABA) is reduced significantly [Citation13]. Results from the present study also support the notion that the metabolism of growth-promoting hormones was enhanced under carbon-rich condition. These observations indicated that metabolism of growth promoting hormones was enhanced under carbon-rich conditions, which would improve plant growth and development, and increase the yield and quality of crops. This further explained how carbon-rich conditions can improve plant growth.
Three or four DEGs were annotated to amino sugar and nucleotide sugar metabolism, starch and sucrose metabolism, phenylalanine metabolism, phenylpropanoid biosynthesis, alpha-linolenic acid metabolism, and sesquiterpenoid and triterpenoid biosynthesis pathways. Four DEGs were annotated to protein processing in the endoplasmic reticulum, one DEG was related to protein export and the spliceosome, indicating that increasing the environmental CO2 concentration can accelerate net photosynthetic rate and increase photosynthetic production. The corresponding transport rate is also accelerated, and source and sink transportation are reduced, resulting in improved plant growth.
Analysis of DEGs related to photosynthesis
Based on comparison of the transcriptomes of strawberry leaves under ambient and carbon-rich conditions, and GO annotation of DEGs, 14 DEGs were related to photosynthesis, of which 10 were up-regulated and four down-regulated under high CO2 concentration. Details of the 14 strawberry genes are presented in .
Table 7. Comparison of strawberry genes and Arabidopsis homologs under ambient and elevated CO2 concentrations.
Both gene32260-v1.0-hybrid and gene02096-v1.0-hybrid participate in the reduction phase of CO2 assimilation, and their functions have been described previously. In our experiment, gene32260-v1.0-hybrid and gene02096-v1.0-hybrid, respectively encoding NAD(P)H dehydrogenase C1 and aldehyde dehydrogenase, were strongly up-regulated under carbon-rich conditions. This suggested that the process of converting active chemical energy into stabilized chemical energy can be accelerated in a carbon-rich environment, and the abilities of plants to store energy can be enhanced, therefore much more photosynthates can be produced.
Gene20355-v1.0-hybrid was predicted to participate in the transfer and transportation of monosaccharides such as D-ribose, D-xylose and glucose. Its homolog protein O-mannosyltransferase 5 (PMT5) belongs to the alcohol/monosaccharide transporter protein family that catalyses the formation of endoplasmic reticulum (ER) membrane glycoproteins with transmembrane-spanning domains [Citation31]. It participates in the transportation of monosaccharides such as D-xylose, galactose and glucose. Another gene Gene19575-v1.0-hybrid has been predicted to participate in regulating photosynthesis and transportation of glycerol phosphate. Its homolog in Arabidopsis encodes a glucose-6-phosphate/phosphate transporter that participates in the transportation of glucose-6-phosphate in carbon metabolism pathways such as the pentose phosphate pathway and glycolysis. These genes were all up-regulated under elevated CO2, which implied that the ability to transport photoassimilates is improved under high CO2 concentration. It explained the reason why elevated CO2 not only speeds up fruit growth and improves fruit quality, but also increases crop yield, consistent with previous results [Citation5].
Gene27842v1.0- hybrid is predicted to regulate the activity of ATP dimethylallyl transferase. Its homolog isopentenyltransferase 3 (IPT3) in Arabidopsis encodes an isopentene transferase, a rate-limiting enzyme that synthesises zeatin using ATP. Therefore, up-regulation of this gene could promote the synthesis of cytokinin (CTK), thereby improving plant growth.
Gene12771-v1.0-hybrid probably encodes an iron-nicotinamide transmembrane transport protein. Its homolog YELLOW STRIPE like 5 (YSL5) in Arabidopsis encodes yellow strip-like protein, members of which are widely distributed in plants. It takes part in the uptake of metal ions such as Fe2+, Zn2+, Cu2+ and Mn2+. In A. thaliana, it is mainly involved in the transport of Fe and nicotinamide chelates [Citation32], and helps the transportation of Fe to aid photosynthesis and chlorophyll production.
Gene21583-v1.0-hybrid was predicted to be involved in cell communication, and its homolog sigma factor binding protein 2 (SIB2) encodes σ-factor binding protein, which is a binding protein of Sig1. AthSig1 is associated with PsaA expression, which is one of the important genes encoding photosystem I [Citation33].
Gene00801-v1.0-hybrid was predicted to participate in regulating the activity of nicotinamidase and nicotinamide adenine dinucleotide (NAD) metabolism. The homolog nicotinamidase 2 (NIC2) encodes nicotinamidase, a key enzyme that interconverts nicotinamide and nicotinic acid, and nicotinamide is essential for the production of NAD/NADH [Citation34]. Gene26936-v1.0-hybrid was predicted to possess electron carrier activity, and to participate in respiratory and electron transfer chains. Its homolog cytochrome c-2 (CYTC-2) encodes cytochrome C, a protein located between the inner and outer mitochondrial membranes related to energy production [Citation35]. Gene00630-v1.0-hybrid was predicted to participate in regulating the activity of electron carriers. Its homolog thioredoxin H-type (ATTRX) encodes thioredoxin, which regulates photosynthesis via the plant REDOX system, and controls the activity of enzymes such as RCA involved in carbon metabolism [Citation36]. Our results indicated that these genes were all up-regulated. This suggested that plant metabolism is promoted under carbon-rich conditions, resulting in more metabolites and energy being produced, which is of great benefit to plant growth and development.
Gene05306-v1.0-hybrid was predicted to be involved in the response to high light intensity and regulation of the electron transfer chain. Its homolog lipoxygenases (LOX) encodes lipoxygenase, a key enzyme of fatty acid oxidation in plants. Lipoxygenase plays an important role in regulating plant growth and development, ripening, senescence and stress responses. As fruits ripen, lipoxygenase activity gradually increases [Citation37].
Gene05590-v1.0-hybrid was predicted to participate in red light and far-red light signalling. Its homolog encodes a homeodomain-leucine zipper (HD-Zip) protein that is rapidly induced in proportion to a change from red light to far-red light. HD-Zip takes part in plant-specific biological processes such as photosynthesis and morphogenesis [Citation38].
Gene26839-v1.0-hybrid was predicted to participate in gluconeogenesis and the production of phosphoenolpyruvate carboxykinase. Its homologs phosphoenolpyruvate carboxykinase 1 (PCK1) and PEPCK encode phosphoenolpyruvate carboxykinase.
Gene17250-v1.0-hybrid is involved in the synthesis of targeted proteins in chloroplasts. Its homologs are predicted to encode transcription factors that up-regulate translocon at the outer envelope membrane of chloroplasts 33 (Toc33) and Toc75. Toc33 and Toc75 are structural components of outer membrane protein transporters found in chloroplasts in A. thaliana [Citation39], and they play an important role in protein transport.
The above analysis revealed five strawberry genes (gene32260-v1.0-hybrid, gene02096-v1.0-hybrid, gene27842-v1.0-hybrid, gene19575-v1.0-hybrid and gene20355-v1.0-hybrid) that may be involved in photosynthetic carbon metabolism and the regulation of modules in photosynthetic processes, since all were markedly differentially expressed. Additionally, gene04191-v1.0-hybrid and Fragaria_vesca_newGene_256 did not have GO annotations, but were significantly differentially expressed between ambient and carbon-rich conditions. Their functions in carbon-rich responses may merit further investigation.
Fourteen genes involved in photosynthesis of strawberry plants were successfully identified in this study through RNA-Seq. Further analysis and functional verification of these genes will need to be carried out in future studies. Better and deeper understanding of the specific functions of these genes could help us develop molecular tools to drastically increase strawberry yields while maintaining and possibly improving fruit quality. The new knowledge could benefit fruit growers and all consumers.
RT-qPCR verification of RNA-Seq data
To verify the accuracy of the acquired RNA-Seq data, four genes (gene00630-v1.0-hybrid, gene19575 -v1.0-hybrid, gene30952-v1.0-hybrid and gene21583-v1.0-hybrid) were selected for RT-qPCR verification using samples HYD-1/HYD-2 and HYF-1/HYF-2 (all in duplicate) under normal and elevated CO2 conditions, which were collected from RNA-Seq. The gene expression levels obtained by RT-qPCR were compared with FPKM values from RNA-Seq (). The results showed that the relative expression levels of all four genes were consistent with the FPKM values, confirming the reliability of the RNA-Seq data.
Figure 4. Quantitative real-time PCR validation of RNA-Seq data based on gene expression levels. FPKM, reads per kb per million reads. Note: The strawberry ACTIN gene was used as an internal reference. Columns with error bars indicate relative quantification (RQ). RQmax and RQmin were calculated using an ABI 7500 instrument.
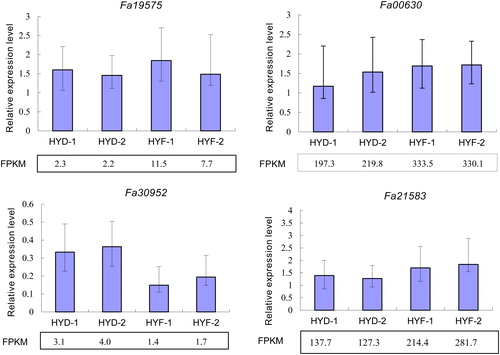
Conclusions
In this study, the leaf growth and photosynthetic indices were determined under two CO2 concentrations in strawberry. The results showed that petioles were significantly longer, and the leaves were longer and wider under elevated CO2 conditions. At the same PAR, the maximum NPR of strawberry leaves under carbon-rich condition was significantly higher than normal condition, and CO2 enrichment significantly increased the net photosynthetic rate and light saturation point of the leaves, but decreased the light compensation point of leaves. Using RNA-Seq, transcriptome profiling of leaf tissues under two CO2 concentrations identified 150 DEGs in response to increased CO2 concentration; 13 DEGs exhibiting 10–50-fold changes (8 up- and 5 down-regulated); 14 DEGs were shown to be involved in photosynthesis. Gene Ontology enrichment and pathways analyses revealed that CO2 enrichment enhanced the biosynthesis of metabolism-promoting growth hormones such as GA, BR and ZR, because gene27756-v1.0-hybrid involved in GA synthesis, gene15105-v1.0- hybrid (BZR1/2) involved in BR synthesis and gene27842-v1.0-hybrid related to zeatin biosynthesis all were up-regulated under carbon-rich conditions. In addition, gene32260-v1.0-hybrid and gene02096-v1.0-hybrid, respectively encoding NAD(P)H dehydrogenase C1 and aldehyde dehydrogenase, were strongly up-regulated under carbon-rich conditions, indicating that CO2 enrichment sped up the reduction step of CO2 assimilation. Genes gene20355-v1.0-hybrid involved in transportation of monosaccharides and gene19575-v1.0-hybrid involved in carbon metabolism and transportation of glyceraldehyde phosphate were both up-regulated under carbon-rich conditions, suggesting that elevated CO2 increased the transport of photosynthetic products. These results help explain the increased net photosynthetic rate and accelerated leaf growth under elevated CO2 level.
Acknowledgements
The authors would like to thank Prof. Yiqun Weng of the USDA-ASR, University of Wisconsin Madison, for his valuable comments and suggestions in the preparation and revision of this manuscript. The authors thank Prof. Yu Gao of the Ohio State University for his revision of this manuscript. The RNA sequencing was carried out by Biomarker Technologies Co., Ltd, Beijing, China.
Disclosure statement
The authors declare that they have no competing interests.
Additional information
Funding
References
- Luo Y, Chen ZL, Song WT, et al. Identification and analysis for anthocyanins compositions in strawberry. J China Agric Univ. 2014;19(05):86–94.
- Ariza MT, Reboredo-Rodríguez P, Mazzoni L, et al. Strawberry achenes are an important source of bioactive compounds for human health. IJMS. 2016;17(7):1103.
- Fitzgerald GJ, Tausz M, O'Leary G, et al. Elevated atmospheric [CO2] can dramatically increase wheat yields in semi-arid environments and buffer against heat waves. Glob Chang Biol. 2016;22(6):2269–2284.
- Huang GL, Wang HL. Effect of CO2 enrichment on growth and yield of cucumber in greenhouse. Agric Technol. 2017;37(02):14.
- Zhao J, Lan H, Shen YN, et al. Effects of carbon-rich on quality and growth characteristics of fruit in strawberry. J Shanxi Agric Sci. 2018;46(04):538–543. http://dx.doi.org/10.3969/j.issn.1002-2481.2018.04.13
- Winston B. Materials needed for photosynthesis. Sciencing Network. 2018. Available from: https://sciencing.com/materials-needed-photosynthesis-7402849.html
- Kimball BA. Crop responses to elevated CO2 and interactions with H2O, N, and temperature. Curr Opin Plant Biol. 2016;31:36–43.
- Teng N, Jin B, Wang Q, et al. No detectable maternal effects of elevated CO(2) on Arabidopsis thaliana over 15 generations. PLoS One. 2009;4(6):e6035.
- Bishop KA, Betzelberger AM, Long SP, et al. Is there potential to adapt soybean (Glycine max Merr.) to future [CO2]? An analysis of the yield response of 18 genotypes in free-air CO2 enrichment. Plant Cell Environ. 2015;38(9):1765–1774.
- Masle J. The effects of elevated CO(2) concentrations on cell division rates, growth patterns, and blade anatomy in young wheat plants are modulated by factors related to leaf position, vernalization, and genotype. Plant Physiol. 2000;122(4):1399–1415.
- Tsutsumi K, Konno M, Miyazawa S, et al. Sites of action of elevated CO2 on leaf development in rice: Discrimination between the effects of elevated CO2 and nitrogen deficiency. Plant Cell Physiol. 2014;55(2):258–268.
- Chen GY, Yong ZH, Liao Y, et al. Photosynthetic acclimation in rice leaves to free-air CO2 enrichment related to both ribulose-1,5-bisphosphate carboxylation limitation and ribulose-1,5-bisphosphate regeneration limitation. Plant Cell Physiol. 2005;46(7):1036–1045.
- Liu J, Zhang J, He C, et al. Genes responsive to elevated CO2 concentrations in triploid white poplar and integrated gene network analysis. PLoS One. 2014;9(5):e98300.
- Jauregui I, Aparicio-Tejo PM, Avila C, et al. Root and shoot performance of Arabidopsis thaliana exposed to elevated CO2: A physiologic, metabolic and transcriptomic response. J Plant Physiol. 2015;189:65–76.
- Xu Z, Jiang Y, Zhou G. Response and adaptation of photosynthesis, respiration, and antioxidant systems to elevated CO2 with environmental stress in plants. Front Plant Sci. 2015;6:701.
- Vicente R, Pérez P, Martínez-Carrasco R, et al. Quantitative RT-PCR platform to measure transcript levels of C and N metabolism-related genes in durum wheat: Transcript profiles in elevated [CO2] and high temperature at different levels of N supply. Plant Cell Physiol. 2015;56(8):1556–1573.
- Zhao X, Li WF, Wang Y, et al. Elevated CO2 concentration promotes photosynthesis of grape (Vitis vinifera L. cv. 'Pinot noir') plantlet in vitro by regulating RbcS and Rca revealed by proteomic and transcriptomic profiles. BMC Plant Biol. 2019;19(1):42.
- Li ML. Strawberry cultivation technology summary. Jinzhong: Shanxi Agricultural University; 2016.
- Xu M. The optimal atmospheric CO2 concentration for the growth of winter wheat (Triticum aestivum). J Plant Physiol. 2015;184:89–97.
- Livak KJ, Schmittgen TD. Analysis of relative gene expression data using real-time quantitative PCR and the 2(-Delta Delta C(T)) method. Methods. 2001;25(4):402–408.
- Bao R, Zhang N. Effects of CO2 application on growth and photosynthetic characteristics of strawberry in greenhouse. Agric Technol Serv. 2013;30(10):1095–1096. http://dx.doi.org/10.3969/j.issn.1004-8421.2013.10.037
- Xin Y, Wu GL, Chen WY, et al. Effects of different carbon dioxide enrichment methods on strawberry in protected cultivation under low temperature and weak light. J Nucl Agric Sci. 2013;27(08):1234–1239.
- Sun P, Mantri N, Lou H, et al. Effects of elevated CO2 and temperature on yield and fruit quality of strawberry (Fragaria × ananassa Duch.) at two levels of nitrogen application. PLoS One. 2012;7(7):e41000.
- Soba D, Ben Mariem S, Fuertes-Mendizábal T, et al. Metabolic effects of elevated CO2 on wheat grain development and composition. J Agric Food Chem. 2019;67(31):8441–8451.
- Cui Q, Li Y, He X, et al. Physiological and iTRAQ based proteomics analyses reveal the mechanism of elevated CO2 concentration alleviating drought stress in cucumber (Cucumis sativus L.) seedlings. Plant Physiol Biochem. 2019;143:142–153.
- Gao J, Han X, Saman S, et al. Leaf photosynthesis and yield components of mung bean under fully open-air elevated [CO2]. J Integr Agric. 2015;14(5):977–983.
- Eberhard S, Finazzi G, Wollman FA. The dynamics of photosynthesis. Annu Rev Genet. 2008;42:463–515.
- Atamian HS, Harmer SL. Circadian regulation of hormone signaling and plant physiology. Plant Mol Biol. 2016;91(6):691–702.
- Zong H, Liu S, Xing R, et al. Protective effect of chitosan on photosynthesis and antioxidative defense system in edible rape (Brassica rapa L.) in the presence of cadmium. Ecotoxicol Environ Saf. 2017;138:271–278.
- Avonce N, Leyman B, Mascorro-Gallardo JO, et al. The Arabidopsis trehalose-6-P synthase AtTPS1 gene is a regulator of glucose, abscisic acid, and stress signaling. Plant Physiol. 2004;136(3):3649–3659.
- Girrbach V, Strahl S. Members of the evolutionarily conserved PMT family of protein O-mannosyltransferases form distinct protein complexes among themselves. J Biol Chem. 2003;278(14):12554–12562.
- Kumar RK, Chu HH, Abundis C, et al. Iron-nicotianamine transporters are required for proper long distance iron signaling. Plant Physiol. 2017;175(3):1254–1268.
- Santabarbara S, Heathcote P, Evans MC. Modelling of the electron transfer reactions in Photosystem I by electron tunnelling theory: The phylloquinones bound to the PsaA and the PsaB reaction centre subunits of PS I are almost isoenergetic to the iron-sulfur cluster F(X). Biochim Biophys Acta. 2005;1708(3):283–310.
- Kajikawa M, Sierro N, Hashimoto T, et al. A model for evolution and regulation of nicotine biosynthesis regulon in tobacco. Plant Signal Behav. 2017;12(6):e1338225.
- Ow YP, Green DR, Hao Z, et al. Cytochrome C: Functions beyond respiration. Nat Rev Mol Cell Biol. 2008;9(7):532–542.
- Bhat JY, Miličić G, Thieulin-Pardo G, et al. Mechanism of enzyme repair by the AAA+ chaperone rubisco activase. Mol Cell. 2017;67(5):744–756.
- Karakurt Y, Huber DJ. Ethylene-induced gene expression, enzyme activities, and water soaking in immature and ripe watermelon (Citrullus lanatus) fruit. J Plant Physiol. 2004;161(4):381–388.
- Turchi L, Baima S, Morelli G, et al. Interplay of HD-Zip II and III transcription factors in auxin-regulated plant development. J Exp Bot. 2015;66(16):5043–5053.
- O'Neil PK, Richardson LGL, Paila YD, et al. The POTRA domains of Toc75 exhibit chaperone-like function to facilitate import into chloroplasts. Proc Natl Acad Sci USA. 2017;114(24):E4868–E4876.