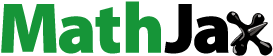
Abstract
Actinidia arguta (Sieb. et Zucc.) is abundant of vitamin C and bioactive compounds with high antioxidant activities. In this study, eight wild A. arguta accessions from different areas in Northeast China were collected. Some bioactive compounds were extracted from leaves, petioles and fruits of different germplasms for constituent analysis and non-enzymatic antioxidant assay. Liquid chromatography with tandem mass spectrometry (LC–MS/MS) was used to detect the flavonoid compounds. The results showed that the highest vitamin C content was found in young apical leaves of ‘CBS-6’ (7.47 mg/g fresh weight), and the highest soluble sugar content was in fruits of ‘CJ-1’ (196.52 mg/g fresh weight) and the highest total phenolic content and total flavonoids content were in young apical leaves of ‘CBS-11’ (3.48 mg/g fresh weight) and of ‘CBS-3’ (2.00 mg/g fresh weight), respectively. Ten flavonoid compounds including kaempferol, isorhamnetin and quercetin were detected in leaves, petioles and fruits. The total content of flavonoids was highest in young apical leaves (10219.84 µg/g) and the lowest in fruits (78.75 µg/g). Based on the comparison of the contents of several bioactive compounds, the two accessions ‘CJ-1’ and ‘CBS-8’ stood out; the young leaves had the strongest antioxidant activity among different tissues based on the non-enzymatic antioxidant assay. These results highlighted the radical-scavenging potential in vitro of A. arguta leaves as a major source of phenolics and vitamin C as well as flavonoids. It provided a theoretical basis for the utilization of leaves of A. arguta.
Supplemental data for this article is available online at https://doi.org/10.1080/13102818.2021.1908166 .
Supplemental data for this article can be accessed online at https://doi.org/10.1080/13102818.2021.1908166.
Introduction
Actinidia arguta (Sieb. et Zucc.) Planch. ex Miq. is well known as hardy kiwifruit with good cold resistance, which is native to East Asia and different from other commercial kiwifruits [Citation1]. A. arguta is widely distributed from 22°N to 47°N in latitude throughout China [Citation2]. It has a smooth and edible skin, good taste and flavour [Citation3]. A. arguta is known to have a very high vitamin C content and to be rich in bioactive and volatile compounds with high antioxidant activities [Citation4], such as flavonoids, phenolic compounds, etc. [Citation5, Citation6]. At present, it is grown commercially in China and some other countries, such as New Zealand, Canada, Japan and the United States [Citation7–9].
Kiwifruit has been recorded in China for more than 2000 years. It has rich nutritional value, strong protective activity and good medicinal value [Citation10–12]. In recent years, many of the previous studies have focused on the fruits of A. arguta [Citation13–15] and kiwifruit [Citation16]. The fruits have a high antioxidant capacity [Citation17, Citation18] and are also an important source of bioactive substances [Citation19], such as vitamins, phenolics, flavonoids, etc. Some researchers have reported flavonoid metabolites and other polyphenol content and evaluated the nutritional values of A. arguta fruits [Citation20–22]. Yu et al. [Citation23] investigated the flavonoid compounds in Actinidia chinensis and A. arguta fruits and identified a total of 125 flavonoids and 39 metabolites in two kiwi berries, which indicated that flavonoid components were abundant in kiwifruit and kiwi berry. Although the bioactivity and antioxidant activity of the A. arguta fruit has been well characterized [Citation4, Citation24], there have been few studies investigating the bioactivity of A. arguta leaves.
A. arguta leaves are accumulated during the pruning each year. They are an affordable and abundant raw material as a byproduct of farming. As early as 1977, A. arguta was proposed as a medicinal food homologous plant. In traditional Chinese medicine, its roots and leaves have various effects, including clearing heat and promoting diuresis and strengthening the stomach [Citation25]. Cyboran et al. [Citation26] reported that A. arguta leaf extract could be used as an effective natural antioxidant protecting the body against external oxidizing agents and also as food additives or supplements against oxidation-induced food aging. Webby [Citation27] identified a new flavonol triglycoside and isolated the kaempferol analogues in leaves of A. arguta. Almeida et al. [Citation19] evaluated the leaves of A. arguta regarding antioxidant and antimicrobial activity, as well as radical-scavenging activity for the first time, identified and quantified the phenolic compounds.
In this study, eight wild A. arguta accessions from different areas of northeastern China were collected. They showed vigorous growth; six of them were female plants, the fruits were all green, and the quality was good; and there were two male accessions, with a large number of blooms and concentrated flowering period. A series of physiological and biochemical substances, such as flavonoids, vitamin C and antioxidant capacity, in the leaves and fruits of eight wild accessions were determined and evaluated in this study. The results were expected to offer a scientific basis for selecting new germplasm with high functional nutrients, which will lay a good foundation for broadening the use of A. arguta.
Materials and methods
Plant materials
Eight A. arguta accessions from northeast China were grown in the open field in the Germplasm Resource Repository of Wild Kiwifruit in Shenyang Agricultural University, involving A. arguta cv. Changjiang No.1 (‘CJ-1’, female plant), A. arguta cv. Huanyou No.1 (‘HY-1’, female plant), BYS No. 13 (‘BYS-13’, male plant), CBS No. 3 (‘CBS-3’, female plant), CBS No. 6 (‘CBS-6’, female plant), CBS No. 11 (‘CBS-11’, male plant), CBS No. 8 (‘CBS-8’, female plant) and BYS No. 5 (‘BYS-5’, female plant) (, ). Six kinds of materials from plants of the accessions were used: young apical leaves (YAL), leaves with 1/4–1/3 size of the fully expanded (QL), leaves with 1/2–2/3 size of the fully expanded (HL), fully expanded mature leaves (ML), petioles of young apical leaves (P) and mature fruits (F) (). The four types of leaves and petioles were gathered at the summer pruning period in mid-June, F were picked from mid-August to mid-September, 2018. All samples were wrapped with tinfoil and frozen in liquid nitrogen right after being collected, then kept in a −80 °C refrigerator till the following experiments.
Figure 1. Four types of leaves, petioles and fruits of A. arguta accessions. (A) Mature leaves (ML); (B) Leaves expanded to 1/2–2/3 of the full leaf size (HL); (C) Leaves expanded to 1/4–1/3 of the full leaf size (QL); (D) Young apical leaves (YAL); (E) Petioles of young apical leaves (P); (F) Fruits (F).
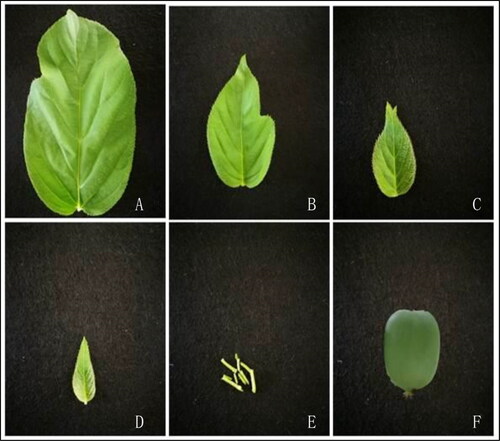
Table 1. Geographical description of the eight Actinidia arguta accessions examined in this study.
Determination of bioactive compounds in leaves and fruits of A. arguta
Vitamin C content
The vitamin C contents in leaves and fruits were determined according to the method of Yoon et al. [Citation28] with some improvement. Each sample (0.2 g) was ground into homogenate with 5 mL of oxalic acid–ethylenediaminetetraacetic acid (EDTA), then distilled water was used to bring the samples to a total volume of 10 mL. The extract (5 mL) was mixed with 0.5 mL of metaphosphoric acid–acetic acid solution, 1 mL of 1:19 sulfuric acid, and 2 mL of 5% ammonium molybdate solution. After 15 min, the mixture was centrifuged for 15 min at 6050.5g (Centrifuge 5804R, Eppendorf, Germany). The absorbance at 760 nm was recorded using a spectrophotometer (UV-2600, Shimadzu Instruments (Suzhou) Co., Ltd.). The total content was calculated using vitamin C as a standard.
Soluble sugar content
The soluble sugar content of the leaves and fruits was determined according to the method of Liu et al. [Citation29] with some modifications. Each sample (0.2 g) was finely powdered and added 15 mL of distilled water and placed in a boiling water bath for 15 min. After that the final volume was brought to 25 mL with distilled water. The extract (1 mL) was mixed with 5 mL of anthrone reagent. After 10 min of boiling in a water bath, the absorbance at 620 nm was recorded using a spectrophotometer (UV-2600, Shimadzu Instruments (Suzhou) Co., Ltd.). Glucose was used as a standard. The result was represented as mg/g fresh weight (mg/g FW).
Free amino acid content
The free amino acid content of the leaves and fruits were determined with some modification of the method proposed by Avino et al. [Citation30]. Each 0.1 g sample was ground into homogenate with acetic acid (10%), and the ammonia-free distilled water was used to bring the total volume of 10 mL. The extract (2 mL) was mixed with 3 mL of ninhydrin reagent and 0.1 mL of ascorbic acid solution and placed in a boiling water bath for 15 min. The absorbance was recorded at 570 nm using a spectrophotometer (UV-2600, Shimadzu Instruments (Suzhou) Co., Ltd.). Leucine was used as a standard.
Total phenolic content
The total phenolic content in A. arguta extracts was determined according to the Folin–Ciocalteu method as described by Navajas-Porras et al. [Citation31] with slight improvement. Each sample (0.2 g) was chopped and added 10 mL of distilled water and placed in a boiling water bath for 10 min. After that, the solution was centrifuged for 10 min at 4537.9g (Centrifuge 5804R, Eppendorf, Germany). The extract of A. arguta (250 µL) was added to 1 mL distilled water, followed by Folin–Ciocalteu reagent (250 µL) and then 7% sodium carbonate (2.5 mL) was added to the mixture. The distilled water was used to make total volume to 10 mL and placed at room temperature for 1.5 h. The absorbance at 760 nm was recorded using a spectrophotometer (UV-2600, Shimadzu Instruments (Suzhou) Co., Ltd.). Gallic acid was used as a standard. The results are presented in gallic acid equivalents as mg/g fresh weight (mg/g FW).
Total flavonoid content
The total flavonoids content was determined by the method of Zainudin et al. with minor modifications [Citation32]. The sample (0.5 g) was finely powdered and extracted with 10 mL formic-methanol (0.2%), centrifuged at 7563.2g (Centrifuge 5804R, Eppendorf, Germany) for 10 min. The extract (5 mL) was added to 0.3 mL of sodium nitrite (NaNO2, 5% w/v), and then 0.3 mL of aluminium chloride (AlCl3, 10% w/v) and 4% sodium hydroxide solution (2 mL) were added every 6 min, respectively. Distilled water was used to bring the total volume to 10 mL, then the samples were allowed to stand for 10 min at room temperature. The absorbance at 510 nm was recorded using spectrophotometer (UV-2600, Shimadzu Instruments (Suzhou) Co., Ltd.). The rutin was used as standard. The result was represented as mg/g fresh weight (mg/g FW).
Antioxidant activity assay
ABTS radical-scavenging activity
The ABTS radical-scavenging activity was assayed with minor modifications according to the spectrophotometric method [Citation33]. The reaction mixture containing a 1:2 ratio of 2.45 mmol/L potassium persulfate solution and 7 mmol/L aqueous solution of ABTS (ABTS+). The mixture was kept in darkness for 14 h at 29 °C, and then it was diluted with ethanol in order to obtain an absorbance value at 734 nm of 0.7 ± 0.02 units. The extracts or reference substances (Trol ox) (200 μL) were added to 3 mL of ABTS radical solution in a dark container and the absorbance was measured at 734 nm after 6 min. Calculations were done as follows:
where A0 is the absorbance of ABTS radical absolute ethanol solution and A1 is the absorbance of the mixture of ABTS radical extract.
DPPH radical-scavenging activity
The DPPH method was used to determine the free radical-scavenging activity of the extract [Citation34]. In order to assay the DPPH radical-scavenging activity, 0.2 g sample was extracted with 10 mL of absolute ethanol and filtered. Then 2 mL solution was taken and mixed with 2 mL of 0.2 mmol/L DPPH absolute ethanol solution. The mixture was kept at room temperature and protected from light for 30 min, and the absorbance was recorded at 517 nm. The following formula was used:
where A0 is the absorbance of DPPH ethanol solution and A1 is the absorbance of the mixture of DPPH and extract.
Analysis of flavonoids compounds using liquid chromatography with tandem mass spectrometry (LC–MS/MS)
The flavonoid compounds were determined using LC–MS/MS analysis (). The content of 10 flavonoid compounds in different tissues of ‘CJ-1’ was quantitatively analyzed. Chromatographic analysis was conducted on an Agilent 6410 Triple Quad LC–MS/MS (Agilent Technologies, USA) with 1260 liquid chromatography system and electrospray ionization source (electrospray ionization, ESI). The chromatographic separation column was Agilent Poroshell 120 SB-C18 column (2.1 × 100 mm, 2.7 μm, Agilent Technologies, USA). A volume of 10 μL sample solution was injected for LC–MS/MS analysis with a flow rate of 0.3 mL/min. Flavones and flavonols were detected at 350 nm. The mobile phases A and B were composed of 2.5% (v/v) formic acid in water and 2.5% (v/v) formic acid in acetonitrile, respectively. The column was eluted using linear gradients at 30 °C: 0 min, 100% A, 0% B; 3 min, 90% A, 10% B; 5 min, 86.5% A, 13.5% B; 7 min, 86.5% A, 13.5% B; 8 min, 86% A, 14% B; 9 min, 85% A, 15% B; 10 min, 85% A, 15% B; 11 min, 84.5% A, 15.5% B; 12 min, 83.5% A, 16.5% B; 12.5 min, 82% A, 18% B; 13 min, 70% A, 30% B; 13.5 min, 69.5% A, 30.5% B; 14 min, 68.5% A, 31.5% B; 17 min, 60% A, 40% B; 20 min, 0% A, 100% B; 23 min, 95% A, 5% B; 25 min, 95% A and 5% B. The MS conditions were: electrospray ionization, positive ion mode; gas (N2) temperature 300 °C; nebulizer pressure, 15 psi; capillary voltage, 4000 V; capillary exit, 200 V. Mass spectra from m/z 100 to 1000 nm were recorded. The analysis of data was conducted using LC/MSD Trap Software 5.3. Naringenin, quercetin 3-O-rutinoside, myricetin, quercetin, dihydroquercetin, kaempferol, dihydromyricetin, dihydrokaempferol, (+)-catechin, (−)-epicatechin obtained from the National Institutes for Food and Drug Control, Beijing, China, and the standard for quantification was quercetin 3-O-rutinoside. The contents of total flavonoids were calculated at 350 nm. Each sample was analyzed in triplicates.
Statistical analysis
Analysis of variance was performed using SPSS 19.0 statistical analysis software (Chicago, USA). All experimental data are presented as mean values from triplicates with standard deviations (±SD). The data significance was checked at p ≤ .05. Pearson’s correlation analysis was conducted between the antioxidant capacity and the content of bioactive compounds.
Results
Determination of bioactive compounds in leaves and fruits of A. arguta
Vitamin C content
Overall, the vitamin C content in the leaves of the eight A. arguta accessions at different stages of maturity showed significant differences. The vitamin C content in young tissues (e.g. YAL, P and QL) was slightly higher than that of old tissues (e.g. HL and ML), and declined with leaf maturity. There was a trend for a decrease in the vitamin C content of the leaves towards leaf maturity (from YAL to ML). For example, in ‘CJ-1’ it ranged from 7.25 to 3.44 mg/g fresh weight from young apical leaves to mature leaves, as well as ‘HY-1’, ‘BYS-13’, ‘CBS-8’, etc. Interestingly, the vitamin C content in the petioles of young leaves was similar to that in young leaves, and in three accessions, ‘BYS-13’, ‘BYS-5’ and ‘CBS-11’, it was even higher than that in young leaves. On the other hand, the vitamin C content in the fruits was lower than that in immature leaves and petioles and higher than that in mature leaves. By comparing the vitamin C content of leaves, petioles and fruits of the eight accessions (), it was found that the vitamin C contents in YAL leaves and fruits of ‘CBS-6’ were the highest among the eight accessions, being 7.47 and 4.47 mg/g fresh weight, respectively, followed by ‘CBS-3’ and ‘CJ-1’, while the vitamin C content in ‘BYS-13’ was the lowest.
Soluble sugar content
The analysis of the soluble sugar content in six tissues of the eight accessions confirmed that the content was the highest in the fruits, followed by the leaves, and the petioles had the lowest content (). The soluble sugar content in the leaves of each accession mostly increased with the gradual maturity of the leaves, and the highest content was in mature leaves (ML). In ‘CJ-1’, the soluble sugar content in mature leaves (ML,156.85 mg/g) was lower than that in fruits, higher than that in other tissues, and 61.88% higher than that in petioles. The highest soluble sugar content (196.52 mg/g fresh weight) was obtained from the mature fruits of ‘CJ-1’. The other five female accessions were similar to ‘CJ-1’, the highest soluble sugar content was measured in the fruits and the lowest content in petioles. In the male accessions, the highest soluble sugar content was measured in mature leaves. On the other hand, the comparison of the soluble sugar content of the eight accessions in showed that the content of ‘CJ-1’, ‘HY-1’ and ‘CBS-6’ in different tissues was relatively higher than that in the other five accessions, and the content in ‘CBS-11’ was the lowest.
Free amino acid content
The free amino acid content in young tissues (e.g. YAL, P and QL) was significantly higher than that in old tissues (e.g. HL and ML), and declined with leaf maturity, the lowest content being measured in fruits. In ‘CJ-1’, the content of free amino acids in petioles was the highest, which was 1.55 mg/g fresh weight, higher than that in other tissues. The lowest content was observed in mature leaves and fruit (ML and F of ‘CJ-1’, 0.14 mg/g fresh weight), which was also the lowest among the eight accessions. Furthermore, the highest content was in QL of ‘BYS-13’ with 1.69 mg/g fresh weight, which was also the highest among the eight accessions. Among the eight accessions, the free amino acid content () in P of ‘CJ-1’, and in YAL and QL of ‘BYS-13’ were higher than those in the other six accessions, while there was no significant difference among the other six accessions.
Total phenolic content
A continuous decrease was observed in the total phenolic content of the eight accessions in the leaves with leaf maturity (from YAL to ML). While the content was highest in the fruits of six female accessions, and the values in the petioles of each accession were the lowest. In ‘CJ-1’, the total phenolic content in the fruits was higher than that in the leaves and petioles with significant differences; that in petioles was the lowest (0.37 mg/g fresh weight), and the highest content in fruits was 2.96 mg/g fresh weight; the difference was eight times. Overall, the total phenolic content () in ‘BYS-5’ was the highest in fruits (3.25 mg/g fresh weight), whereas in ‘BYS-13’ the values were lower than those in the other accessions. The highest value (3.48 mg/g) among the eight accessions was observed in the YAL of ‘CBS-11’.
Total flavonoids content
Overall, the total flavonoids content in the leaves of the eight A. arguta accessions showed a decreasing trend with the leaf maturity. The total flavonoids content in the young leaves was higher than that in the old leaves, whereas that in petioles was higher than in fruits, but lower than in leaves. Among the eight accessions, the lowest content was measured in fruits. The total flavonoids content values of YAL in the eight accessions were highest, followed by QL, HL and ML. When compared to ML among the eight accessions, the total flavonoids content of YAL was higher from 1.83-fold to 3.41-fold. In ‘CJ-1’, the total flavonoids content in different tissues ranked in the order of leaf > petiole > fruit, and the content in YAL (1.83 mg/g) was 6.3-fold higher than that in the fruits (F, 0.29 mg/g). There was no significant difference among the total flavonoids content in the eight accessions (); the content in ‘CJ-1’, ‘CBS-8’ and ‘CBS-3’ was slightly higher than in the other six accessions.
Figure 7. Comparison of total flavonoids content of different tissues in eight A. arguta accessions. YAL, young apical leaves; QL, leaves expanded to 1/4–1/3 of the full leaf size; HL, leaves expanded to 1/2–2/3 of the full leaf size; ML, mature leaves; P, petioles of young apical leaves; F, fruits.
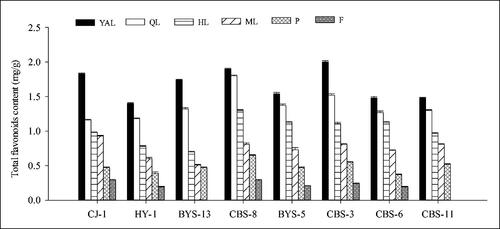
Antioxidant activity assay
ABTS free radical-scavenging assay
It was observed that the ABTS radical-scavenging activity in the leaf samples from the eight A. arguta accessions decreased with the leaf maturity. The ABTS radical-scavenging activity in the young leaves was higher than that in the old leaves, and higher than that in the petioles and fruits; the lowest values were in the fruits. Interestingly, the ABTS radical-scavenging activity in the petioles of young leaves was almost the lowest, unlike that in young leaves. The ABTS radical-scavenging activity values of YAL in the eight accessions were highest, followed by QL, HL and ML, such as ‘CJ-1’ where it ranged from 41.51 to 14.90 mmol/g fresh weight from young apical leaves to mature leaves, as well as ‘HY-1’, ‘BYS-13’, ‘BYS-5’ and ‘CBS-6’, which also showed a similar trend. On the other hand, in ‘CJ-1’, except for ML, the ABTS radical-scavenging activity of leaves was greater than that of petioles and fruits, and the ABTS radical-scavenging activity of YAL (41.51 mmol/g) was 2.5-fold higher than that in the fruit (16.65 mmol/g). In the eight accessions (), the ABTS radical-scavenging activity ‘CJ-1’ was slightly higher than that in the other accessions and the lowest activity was measured in ‘BYS-13’. The highest value in the fruits was in ‘CBS-3’, which was 18.51 mmol/g.
Figure 8. Comparison of ABTS+ antioxidant activity of different tissues in eight A. arguta accessions. YAL, young apical leaves; QL, leaves expanded to 1/4–1/3 of the full leaf size; HL, leaves expanded to 1/2–2/3 of the full leaf size; ML, mature leaves; P, petioles of young apical leaves; F, fruits.
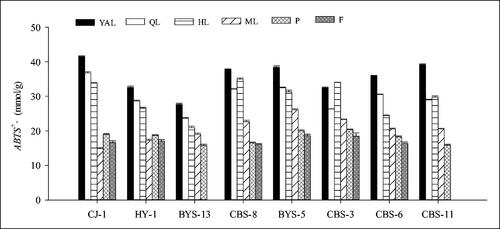
DPPH free radical-scavenging assay
The DPPH radical-scavenging activity in the eight A. arguta accessions leaves decreased with the leaf maturity similar to the ABTS radical-scavenging activity. The DPPH radical-scavenging activity of the young leaves and petioles was higher than that of the old leaves. While the values in fruits were only higher than mature leaves. The DPPH radical-scavenging activity of YAL in eight accessions was highest except ‘BYS-13’, ‘CBS-3’ and ‘CBS-8’, followed by QL, HL and ML, such as ‘CJ-1’ where it ranged from 91.22 to 71.29 µmol TE/L from young apical leaves to mature leaves. In ‘CJ-1’, the DPPH radical-scavenging activity of YAL (91.22 µmol TE/L) was 1.3-fold higher than that in the fruit, and the value of fruits (71.29 µmol TE/L) was only higher than ML (65.60 µmol TE/L). When we compared the DPPH radical-scavenging activity of leaves and petioles of different maturity in the eight accessions (), it was found that ‘CJ-1’ showed slightly higher activity than the other accessions, whereas there was no significant difference among these accessions.
Figure 9. Comparison of DPPH antioxidant activity of different tissues in eight A. arguta accessions. YAL, young apical leaves; QL, leaves expanded to 1/4–1/3 of the full leaf size; HL, leaves expanded to 1/2–2/3 of the full leaf size; ML, mature leaves; P, petioles of young apical leaves; F, fruits.
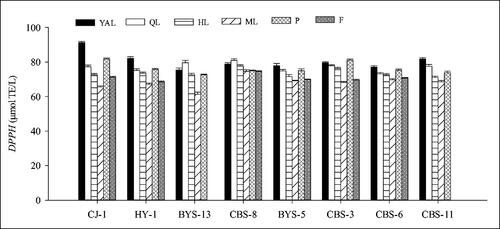
Correlation between bioactive compounds and antioxidant activity
To identify the relationship between the main bioactive substances in the leaves of A. arguta and their relationship with the ability to scavenge free radicals, a correlation analysis was done (). The vitamin C content and the soluble sugar content had significantly negative correlation (r2 = −0.936; p < .05), and positive correlation with the free amino acid content (r2 = 0.957; p < .05), total phenolic content (r2 = 0.997; p < .01) and total flavonoids content (r2 = 0.999; p < .05). The soluble sugar content and free amino acid content (r2 = −0.969; p < .05) exhibited significantly negative correlation. An extremely positive correlation was found between the free amino acid content and total flavonoids content (r2 = 0.968; p < .05). There was positive correlation between the total phenolic content and total flavonoids content (r2 = 0.993 at p < .01).
Table 2. Correlation analysis between main bioactive substances and free radical-scavenging ability of Actinidia arguta.
The results showed that the vitamin C content, free amino acid content as well as total phenolic content and total flavonoids content were significantly positively correlated with the DPPH free radical-scavenging rate (r2 = 0.989, 0.952, 0.986, 0.987, respectively; p < .05), while significant negative correlation was found between the soluble sugar content and DPPH free radical-scavenging rate (r2 = −0.967; p < .05). A strong positive correlation was found between the total phenolic content and ABTS free radical-scavenging rate (r2 = 0.956; p < .05). The ABTS and DPPH radical-scavenging activities were positively correlated (r2 = 0.970; p < .05).
Analysis of flavonoids compounds using LC–MS/MS
By LC–UV–MS analysis, the composition of flavonoids in A. arguta could be identified by the UV–vis spectrum, elution order, retention time and MS fragmentation pattern by reference with published data [Citation35]. A total of 10 peaks were detected in the flavonoid extract of A. arguta, which were identified by the retention time in LC–MS/MS system, elution order, λmax in the visible region, main MS2 fragments and molecular ion (). Some components had similar absorption UV spectra. The maximum absorption peaks were at 240–280 nm and 330- 380 nm. Therefore, it was inferred that these components belonged to the flavonol glycoside compound (). As shown in , for example, a series of strong [M + H]+ ions were observed in the (+) ESI–MS spectrum of the flavonoid extract of A. arguta leaves, including m/z 579, m/z 595, m/z 625, m/z 653, m/z 757, etc., as well as stronger Y0+ ions, [Y0 + H]− ions and other fragment ions, such as m/z 285, m/z 300, m/z 301, m/z 314, m/z 315, m/z 316 plasma and m/z 271, m/z 255, m/z 243, m/z 227 and other fragment ions (). It was preliminarily determined to indicate aglycone components belonging to isorhamnetin, quercetin and kaempferol. From the above information, it could be inferred that the compound contained one glycoside or two glycosides. On the basis of the UV–vis spectrum, retention time, main MS2 fragments and molecular ion, F1, F2 and F3 were tentatively identified as kaempferol-3-O-rutinoside (cis), kaempferol-3-O-rutinoside (trans) and kaempferol-3-O- neohesperidoside (P), respectively. The possible structure of F4 or F5 was isorhamnetin-3-O-α-L-rhamnosyl-(1 → 3)-α-L-rhamnosyl-(1 → 6)-β-D-galactoside. F6 was identified as isorhamnetin-3-O-neohesperidoside (P) (cis) at that stage; F7 might be isorhamnetin-3-O-neohesperidoside (P) (trans) and F8 might be isorhamnetin-3-O-rutinoside. The possible structure of F9 was isorhamnetin-3-O-neohespeidoside or isorhamnetin-7-O-(4″-O-acetyl-glucosyl)-3-O-xyloside. F10 was tentatively determined as quercetin-3-O-rhamnoglycoside ().
Table 3. Chromatographic and spectraldata of flavonoid glycosides from Actinidia arguta leaves.
The content of 10 flavonoid compounds in different tissues of ‘CJ-1’ was quantitatively analyzed (). Quercetin-3-O-rhamnoglycoside was among the highest content of total flavonoids in the five tissues, accounting for 32.62% in YAL, 29.71% in QL, 31.58% in ML, 58.95% in P and 56.55% in F, respectively. Kaempferol, isorhamnetin and quercetin compounds were found in the leaves (YAL, QL, HL and ML), petioles (P) and fruits (F). The highest level of flavonoid accumulation was detected in the leaves, and flavonoids in P and F were 151.63 µg/g and 78.75 µg/g, respectively. It varied significantly in different types of leaves, which was 10219.84 µg/g, 4977.83 µg/g, 3986.94 µg/g and 350.55 µg/g in YAL, QL, HL and ML, respectively. The highest total flavonoid content was found in YAL, and the content in YAL was higher than that in ML, P and F, respectively, by 29.2-fold, 67.4-fold and 129.8-fold. On the other hand, it was interesting to find that the concentration of some flavonoids from different tissues, such as isorhamnetin-3-O-glucoside, kaempferol-3-O-rutinoside and quercetin-3-O-glucoside, showed significant differences in different maturity. Quercetin compounds were the most widely distributed in A. arguta (). However, the levels of isorhamnetin and kaempferol compounds were higher than those of quercetins in HL. The content of isorhamnetin compounds in YAL was the highest followed by quercetin and kaempferol compounds. Among the flavonoids in QL, kaempferol compounds were present at the highest level, followed by isorhamnetin and quercetin compounds (relatively low). The content of isorhamnetin compounds was the highest in HL, followed by kaempferol and quercetin compounds. The content of isorhamnetin compounds was highest in ML, followed by quercetin and kaempferol compounds. However, the levels of quercetin compounds were the highest in P and F, followed by isorhamnetin and kaempferol compounds.
Table 4. Contents of flavonoids in different parts of leaves in CJ-1.
Discussion
Evaluation on bioactive compounds and antioxidant activity in different tissues of A. arguta
In a previous report, the maturity was important for the nutritional quality of fruits and vegetables [Citation36]. The content of bioactive compounds and antioxidant capacity in leaves were impacted by the maturation degree; for example, vitamin C [Citation37], total carotenoids, total polyphenol [Citation32], etc. Lisiewska et al. [Citation38] reported diminishing vitamin C concentrations with dill growth. In our study, the vitamin C content decreased as the leaves became mature, and vitamin C concentrations of fruits were lower than those of immature leaves and petioles, while higher than mature leaves [Citation38]. However, Yamada et al. [Citation39] and Omary et al. [Citation40] found an opposite trend in leaf maturity development of spinach and broccoli, respectively.
In some plants, the polyphenols concentrations also decrease with the leaf maturity, such as that of young aronia leaves being higher than that in old leaves [Citation41]. The polyphenols in the leaves of Cosmos caudatus decreased with maturity [Citation42], and the highest levels of phenolic compounds were observed at the young leaf stages of Rosmarinus officinalis [Citation43]. In blackberry, raspberry and strawberry leaves, Wang and Lin [Citation44] also found the same trend. Zhang et al. [Citation45] reported that the levels of total phenolics and flavonoids declined remarkably with pomegranate leaf growth, and demonstrated that the high content in the young leaves was due to these metabolites being synthesized accompanying bud germination, which were provided the substrates by the stem and/or root. At present, there are few reports on the evaluation of A. arguta leaves. According to Thi and Hwang [Citation41], the total polyphenol content of aronia leaves was lower than that of the aronia fruit. In our study, the total flavonoids content and total phenolic content decreased with the increase of maturity, whereas in A. arguta fruits, the total phenolic content was higher than that in leaves and petioles, but the total flavonoids content was lower than that in leaves and petioles. The free amino acid content in different parts of the leaves and different tissues showed a declining trend with the maturity. The free amino acid content in the petiole of young leaves was not much different from that in young leaves, but they were higher than that of in fruits. This was consistent with the result of Song et al. [Citation46], who reported that the total free amino acid content showed a decreasing trend with e maturity.
ABTS and DPPH are important and effective methods being widely used to study and evaluate the free radical-scavenging capacity and antioxidant activity of plants [Citation47]. In previous studies, many researchers used these two methods to evaluate the antioxidant activity, such as grape [Citation48], Tropaeolum majus [Citation47], etc. Furthermore, the antioxidant activity of the leaves could be attributed to the conjunction of phytochemicals (phenolic acids and flavonoids), as they are considered natural antioxidants in fruits and vegetables [Citation45]. In our study, the vitamin C content, free amino acid content as well as total phenolic content and total flavonoids content were significantly positively correlated with the DPPH free radical-scavenging capacity, and a strong positive correlation was found between the total phenolic content and ABTS free radical-scavenging capacity. We inferred that the antioxidant activity of natural extracts was due not only to the presence of individual components in them, it was a combination of the overall action of all ingredients, just like Mileva et al. [Citation49] reported that the biological features of plant extracts cannot be attributed to the activities to single constituents. It could be explained that their scavenging activity was due to the combination of effects with one another [Citation49]. Our data indicated that YAL exhibited a very good activity against ABTS and DPPH radicals, maybe due to high total phenolic content and total flavonoids content in it.
The leaf flavonoids identification of A. arguta
The LC–MS/MS is a useful technique to conduct structural identification of flavonol aglycone type [Citation35, Citation50, Citation51]. The LC–MS/MS method has been developed for the rapid screening and determination of bioactive compounds in fruit efficiently and sensitively [Citation52]. Singh et al. [Citation51] identified quercetin-O-pentohexoside, quercetin-3-diglucoside, quercetin-O-xylo-pentoside and kaempferol-O-glucoside using LC–MS/MS and found some molecular ions in the full scan mass spectra, such as m/z 625, m/z 595, m/z 579, and some other ion fragments, including m/z 301, m/z 285 [Citation51]. The LC–MS/MS analysis was used to determine the overall composition of the leaf flavonoids in A. arguta in this study and in the light of the UV–vis spectrum. The characteristic absorption peak existed at 240–280 nm and 330–380 nm, which indicated that these compounds were all flavonol glycoside compounds [Citation53]. It was found that the concentration of some flavonoids from different tissues showed significant differences at different stages of maturity.
Bioactive potential of A. arguta leaves
In recent years, antioxidant phytochemicals have been reported to restrain the propagation of free radical reactions. Phytochemicals such as flavonoids and other polyphenols defend the human body against diseases and increase life expectancy [Citation54]. Simultaneously, considerable regard has been devoted to the development of natural antioxidants of plants origin, including Salvia [Citation55], and Prunus salicina [Citation56]. Our study indicated that the flavonoid components in young leaves of A. arguta contained quercetin, kaempferol and isorhamnetin compounds, and the content of total flavonoid was higher than that of mature leaves. A previous study showed that the high content of polyphenols can be used in functional foods, which can prevent certain diseases. Such bioactivity is attributed to anti-inflammatory, antiviral, antibacterial, anticarcinogenic, hepatoprotective, antiallergic, antithrombotic and vasodilatory action [Citation57]. According to the Dictionary of Traditional Chinese Medicine, A. arguta could be used as a medicine food homologous plant. Its roots and leaves have the effects of clearing heat and promoting diuresis and strengthening the stomach [Citation25]. It is expected that the young leaves can be processed into tea or nutrition capsules in the future, like Ginkgo biloba [Citation58] and pomegranate [Citation45]. Therefore, the results from this study could contribute to the development of functional foods for A. arguta leaves in the future.
Conclusions
The bioactive composition and antioxidant activity of eight different wild A. arguta accessions and different tissues were systematically examined and evaluated in this study. The results indicated that the contents of different bioactive compounds in the eight accessions varied greatly, and ‘CJ-1’ and ‘CBS-8’ had relatively outstanding performance. According to the comprehensive evaluation of the antioxidant activity among different tissues, the young leaves had the strongest antioxidant activity. This work not only lays the foundation for the better utilization of these resources, but also is of importance for the development of functional foods using the leaves of A. arguta.
Data availability statement
All data that support the findings reported in this study are available from the corresponding author upon reasonable request.
Disclosure statement
No potential conflict of interest was reported by the authors.
References
- Ferguson AR. Kiwifruit (Actinidia). Genetic Resources of Temperate Fruit & Nut Crops. 1991;290:603–656. Available from: https://doi.org/10.17660/actahortic.1991.290.14.
- Vorobiev DP. Review of far east species of genera Actinidia Lindley. Bulletin of Gornotaiegny Station of Far East Branch of the Academy of Sciences of USSR. Chabarovsk. 1939;3:5–38.
- Kabaluk JT, Kempler C, Toivonen PMA. Actinidia arguta – characteristics relevant to commercial production. Fruit Varieties J. 1997;51(2):117–122. Available from: https://doi.org/10.1007/s001220050500.
- Latocha P, Wołosiak R, Worobiej E, et al. Clonal differences in antioxidant activity and bioactive constituents of hardy kiwifruit (Actinidia arguta) and its year-to-year variability. J Sci Food Agric. 2013;93(6):1412–1419. Available from: https://doi.org/10.1002/jsfa.5909.
- Leontowicz H, Leontowicz M, Latocha P, et al. Bioactivity and nutritional properties of hardy kiwi fruit Actinidia arguta in comparison with Actinidia deliciosa ‘Hayward’ and Actinidia eriantha ‘Bidan’. Food Chem. 2016;196:281–291. Available from: https://doi.org/10.1016/j.foodchem.2015.08.127.
- Matich AJ, Young H, Allen JM, et al. Actinidia arguta: volatile compounds in fruit and flowers. Phytochemistry. 2003;63(3):285–301. Available from: https://doi.org/10.1016/S0031-9422(03)00142-0.
- Liang CF. On the distribution of Actinidia. Guihaia. 1983;3:229–248.
- Ferguson R, Huang H. Genetic resources of kiwifruit: domestication and breeding. Hortic Rev. 2007;33:1–121. Available from: https://doi.org/10.1002/9780470168011.ch1.
- Latocha P, Krupa T. Morphological, chemical and sensory analyses of promising genotypes of hardy kiwifruit (Actinidia Lindl.) obtained in the breeding programme at WULS. Ann Warsaw Univ Life Sci –SGGW, Hortic Landsc Architect. 2007;28:111–119.
- Xu X, Zhang Q. Researches and utilizations of germplasm resource of kiwifruit in China. Chinese Bull Bot. 2003;020(006):648–655. (in Chinese)
- Jung KA, Song TC, Han D, et al. Cardiovascular protective properties of kiwifruit extracts in vitro. Biol Pharm Bull. 2005;28(9):1782–1785. Available from: https://doi.org/10.1248/bpb.28.1782.
- Park Y-S, Leontowicz H, Leontowicz M, et al. Comparison of the contents of bioactive compounds and the level of antioxidant activity in different kiwifruit cultivars. J Food Comp Anal. 2011;24(7):963–970. Available from: https://doi.org/10.1016/j.jfca.2010.08.010.
- Li W, Ding Z, Ruan M, et al. Kiwifruit R2R3-MYB transcription factors and contribution of the novel AcMYB75 to red kiwifruit anthocyanin biosynthesis. Sci Rep. 2017;7(1):16861. Available from: https://doi.org/10.1038/s41598-017-16905-1.
- Pérez-Burillo S, Oliveras MJ, Quesada J, et al. Relationship between composition and bioactivity of persimmon and kiwifruit. Food Res Int. 2018;105:461–472. Available from: https://doi.org/10.1016/j.foodres.2017.11.022.
- Wang L, Tang W, Hu Y, et al. A MYB/bHLH complex regulates tissue-specific anthocyanin biosynthesis in the inner pericarp of red-centered kiwifruit Actinidia chinensis cv. Hongyang. Plant J. 2019;99:359–378. Available from: https://doi.org/10.1111/tpj.14330.
- Liang D, Xia H. Comparison of the contents of bioactive compounds and the level of antioxidant activity in different kiwifruit genotypes. In 2016 Joint International Conference on Social Science and Environmental Science (SSES 2016) and International Conference on Food Science and Engineering (ICFSE 2016). 2016. p. 426–429.
- Wang H, Cao G, Prior RL. Total antioxidant capacity of fruits. J Agric Food Chem. 1996;44(3):701–705. Available from: https://doi.org/10.1021/jf950579y.
- Latocha P, Krupa T, Wołosiak R, et al. Antioxidant activity and chemical difference in fruit of different Actinidia sp. Int J Food Sci Nutr. 2010;61(4):381–394. Available from: https://doi.org/10.3109/09637480903517788.
- Almeida D, Pinto D, Santos J, et al. Hardy kiwifruit leaves (Actinidia arguta): an extraordinary source of value-added compounds for food industry. Food Chem. 2018;259:113–121. Available from: https://doi.org/10.1016/j.foodchem.2018.03.113.
- Li Y, Fang J, Qi X, et al. Combined analysis of the fruit metabolome and transcriptome reveals candidate genes involved in flavonoid biosynthesis in Actinidia arguta. IJMS. 2018;19(5):1471. Available from: http://dx.doi.org/10.3390/ijms19051471.
- Li Y, Fang J, Qi X, et al. A key structural gene, AaLDOX, is involved in anthocyanin biosynthesis in all red-fleshed kiwifruit (Actinidia arguta) based on transcriptome analysis. Gene. 2018;648:31–41. Available from: https://doi.org/10.1016/j.gene.2018.01.022.
- Wojdyło A, Nowicka P. Anticholinergic effects of Actinidia arguta fruits and their polyphenol content determined by liquid chromatography-photodiode array detector-quadrupole/time off light-mass spectrometry (LC–MS–PDA-Q/TOF). Food Chem. 2019;271:216–223. Available from: https://doi.org/10.1016/j.foodchem.2018.07.084.
- Yu M, Man Y, Lei R, et al. Metabolomics study of flavonoids and anthocyanin-related gene analysis in kiwifruit (Actinidia chinensis) and kiwiberry (Actinidia arguta). Plant Mol Biol Rep. 2020;38(3):353–369. Available from: https://doi.org/10.1007/s11105-020-01200-7.
- Zuo LL, Wang ZY, Fan ZL, et al. Evaluation of antioxidant and antiproliferative properties of three Actinidia (Actinidia kolomikta, Actinidia arguta, Actinidia chinensis) extracts in vitro. IJMS. 2012;13(5):5506–5518. Available from: https://doi.org/10.3390/ijms13055506.
- Zhao G, Dai S, Chen R. Dictionary of Traditional Chinese Medicine. China: Shanghai Science and Technology Press; 2006. (in Chinese).
- Cyboran S, Oszmiański J, Kleszczyńska H. Modification of the properties of biological membrane and its protection against oxidation by Actinidia arguta leaf extract. Chem Biol Interact. 2014;222:50–59. Available from: https://doi.org/10.1016/j.cbi.2014.08.012.
- Webby RF. A flavonol triglycoside from Actinidia arguta var. giraldii. Phytochemistry. 1991;30(7):2443–2444. Available from: https://doi.org/10.1016/0031-9422(91)83680-J.
- Yoon YE, Kuppusamy S, Cho KM, et al. Influence of cold stress on contents of soluble sugars, vitamin c and free amino acids including gamma-aminobutyric acid (GABA) in spinach (Spinacia oleracea). Food Chem. 2017;215:185–192. Available from: https://doi.org/10.1016/j.foodchem.2016.07.167.
- Liu H, Fu Y, Hu D, et al. Effect of green, yellow and purple radiation on biomass, photosynthesis, morphology and soluble sugar content of leafy lettuce via spectral wavebands “knock out”. Sci Hortic-Amsterdam. 2018;236:10–17. Available from: https://doi.org/10.1016/j.scienta.2018.03.027.
- Avino P, Campanella L, Russo MV. Use of different anticoagulants for HPLC separation and quantification of the free amino acid content of plasma. J Sep Science. 2003;26(5):392–396. Available from: https://doi.org/10.1002/jssc.200390050.
- Navajas-Porras B, Pérez-Burillo S, Morales-Pérez J, et al. Relationship of quality parameters, antioxidant capacity and total phenolic content of EVOO with ripening state and olive variety. Food Chem. 2020;325:126926. Available from: https://doi.org/10.1016/j.foodchem.2020.126926.
- Zainudin MAM, Hamid AA, Anwar F, et al. Variation of bioactive compounds and antioxidant activity of carambola (Averrhoa carambola L.) fruit at different ripening stages. Sci Hortic-Amsterdam. 2014;172:325–331. Available from: https://doi.org/10.1016/j.scienta.2014.04.007.
- Chandel M, Sharma U, Kumar N, et al. Antioxidant activity and identification of bioactive compounds from leaves of Anthocephalus cadamba by ultra-performance liquid chromatography/electrospray ionization quadrupole time of flight mass spectrometry. Asian Pac J Trop Med. 2012;5(12):977–985. Available from: https://doi.org/10.1016/S1995-7645(12)60186-2.
- Chávez-Mendoza C, Sanchez E, Muñoz-Marquez E, et al. Bioactive compounds and antioxidant activity in different grafted varieties of bell pepper. Antioxidants (Basel). 2015;4(2):427–446. Available from: https://doi.org/10.3390/antiox4020427.
- Xue L, Wang Z, Zhang W, et al. Flower pigment inheritance and anthocyanin characterization of hybrids from pink-flowered and white-flowered strawberry. Sci Hortic-Amsterdam. 2016;200:143–150. Available from: https://doi.org/10.1016/j.scienta.2016.01.020.
- Lee SK, Kader AA. Preharvest and postharvest factors influencing vitamin C content of horticultural crops. Postharvest Biol Tec. 2000;20(3):207–220. Available from: https://doi.org/10.1016/s0925-5214(00)00133-2.
- Wawire M, Oey I, Mathooko F, et al. Thermal stability of ascorbic acid and ascorbic acid oxidase in African cowpea leaves (Vigna unguiculata) of different maturities. J Agric Food Chem. 2011;59(5):1774–1783. Available from: https://doi.org/10.1021/jf103469n.
- Lisiewska Z, Kmiecik W, Korus A. Content of vitamin C, carotenoids, chlorophylls and polyphenols in green parts of dill (Anethum graveolens L.) depending on plant height. J Food Compos Anal. 2006;19(2–3):134–140. Available from: https://doi.org/10.1016/j.jfca.2005.04.009.
- Yamada C, Iwasaki Y, Yoshida K. Effect of growth stage on contents of reducing sugar, ascorbic acid, oxalate and nitrate in spinach. JJSNFS. 2003;56(3):167–173. Available from: https://doi.org/10.4327/jsnfs.56.167.
- Omary MB, Brovelli EA, Pusateri DJ, et al. Sulforaphane potential and vitamin C concentration in developing heads and leaves of broccoli (Brassica oleracea var. italica). J Food Quality. 2003;26(6):523–530. Available from: https://doi.org/10.1111/j.1745-4557.2003.tb00266.x.
- Thi ND, Hwang ES. Bioactive compound contents and antioxidant activity in aronia (Aronia melanocarpa) leaves collected at different growth stages. JFN. 2014;19(3):204–212. Available from: https://doi.org/10.3746/pnf.2014.19.3.204.
- Dian-Nashiela F, Noriham A, Nooraain H, et al. Antioxidant activity of herbal tea prepared from Cosmos caudatus leaves at different maturity stages. Int Food Res J. 2015;22(3):1189–1194.
- Bãno M-d, Lorente J, Castillo J, et al. Phenolic diterpenes, flavones, and rosmarinic acid distribution during the development of leaves, flowers, stems, and roots of Rosmarinus officinalis. Antioxidant activity. J Agric Food Chem. 2003;51(15):4247–4253. Available from: https://doi.org/10.1021/jf0300745.
- Wang SY, Lin HS. Antioxidant activity in fruits and leaves of blackberry, raspberry, and strawberry varies with cultivar and developmental stage. J Agric Food Chem. 2000;48(2):140–146. Available from: https://doi.org/10.1021/jf9908345.
- Zhang L, Gao Y, Zhang Y, et al. Changes in bioactive compounds and antioxidant activities in pomegranate leaves. Sci Hortic-Amsterdam. 2010;123(4):543–546.
- Song J, Bi J, Chen Q, et al. Assessment of sugar content, fatty acids, free amino acids, and volatile profiles in jujube fruits at different ripening stages. Food Chem. 2019;270(1):344–352. Available from: https://doi.org/10.1016/j.foodchem.2018.07.102.
- Garzon GA, Wrolstad RE. Major anthocyanins and antioxidant activity of nasturtium flowers (Tropaeolum majus). Food Chem. 2009;114(1):44–49.
- Sridhar K, Charles AL. In vitro antioxidant activity of Kyoho grape extracts in DPPH and ABTS assays: estimation methods for EC50 using advanced statistical programs. Food Chem. 2019;275(1):41–49.
- Mileva M, Krumova E, Miteva-Staleva J, et al. Chemical compounds, in vitro antioxidant and antifungal activities of some plant essential oils belonging to Rosaceae family. C. R. Acad. Bulg. Sci. 2014;67(10):1363–1368.
- Dartora N, de Souza LM, Santana-Filho AP, et al. UPLC-PDA–MS evaluation of bioactive compounds from leaves of Ilex paraguariensis with different growth conditions, treatments and ageing. Food Chem. 2011;129(4):1453–1461. Available from: https://doi.org/10.1016/j.foodchem.2011.05.112.
- Singh AP, Wilson T, Luthria D, et al. LC–MS–MS characterisation of curry leaf flavonols and antioxidant activity. Food Chem. 2011;127(1):80–85. Available from: https://doi.org/10.1016/j.foodchem.2010.12.091.
- Pandey R, Mahar R, Hasanain M, et al. Rapid screening and quantitative determination of bioactive compounds from fruit extracts of Myristica species and their in vitro antiproliferative activity. Food Chem. 2016;211:483–493. Available from: https://doi.org/10.1016/j.foodchem.2016.05.065.
- Du QH, Zhang QY, Han T, et al. Dynamic changes of flavonoids in Actinidia valvata leaves at different growing stages measured by HPLC–MS/MS. Chin J Nat Med. 2016;14(1):66–72. Available from: https://doi.org/10.3724/SP.J.1009.2015.00066.
- Khoobi M, Emami S, Dehghan G, et al. Synthesis and free radical scavenging activity of coumarin derivatives containing a 2-methylbenzothiazoline motif. Arch Pharm (Weinheim). 2011;344(9):588–594. Available from: https://doi.org/10.1002/ardp.201000271.
- Zupkó I, Hohmann J, Rédei D, et al. Antioxidant activity of leaves of Salvia species in enzyme-dependent and enzyme-independent systems of lipid peroxidation and their phenolic constituents. Planta Med. 2001;67(4):366–368. Available from: https://doi.org/10.1055/s-2001-14327.
- Delgado-Adámez J, Fernández-León MF, Velardo-Micharet B, et al. In vitro assays of the antibacterial and antioxidant activity of aqueous leaf extracts from different Prunus salicina Lindl. cultivars. Food Chem Toxicol. 2012;50(7):2481–2486. Available from: https://doi.org/10.1016/j.fct.2012.02.024.
- Jeribi C, Karoui IJ, Hassine DB, et al. Comparative study of bioactive compounds and antioxidant activity of Schinus terebinthifolius RADDI fruits and leaves essential oils. Int J Sci Res (IJSR). 2014;3(12):453–458.
- Yuan D, Chen X, Hou L, et al. Study on production of Ginkgo biloba tea with pilefermentation. J Food Sci Biotech. 2016;36(7):84–89. (in Chinese).