Abstract
Fatty acids (FAs) in oilseeds are important for human health. Gas chromatography-mass spectrometry (GC-MS) is a popular analytical technology for FAs measurement. FAs need to be derivatized into fatty acid methyl esters (FAMEs), which is essential for GC-MS analysis. The previous methods for methylation of FAs usually include reaction at high temperature and need specific vessels, for which samples with a large scale cannot be analyzed in a short time, leading to low efficiency. Here we report a high-throughput method for profiling fatty acids in plant seeds based on one-step acid-catalyzed methylation followed by GC-MS. This method for FA methylation via derivatization by sulfuric acid with methanol (5%, v/v) and incubation at 64 °C for 4 h in a more convenient 2 mL tube, is efficient. Satisfactory methodological performance was achieved by optimization of the parameters. Only 5 mg seeds were needed as a microscale sample usage by this method. The method is a successful approach to increase the efficiency of sample preparation and analysis for large-scale experiments.
Introduction
Edible vegetable oil is an essential part of human daily diet by providing necessary energy and nutritional components (e.g. fatty acid and vitamins). Oilseed crops are important sources of vegetable oils for food and industry. Soybean (Glycine max), rapeseed (Brassica napus), peanut (Arachis hypogaea) and sunflower (Helianthus annuus) are the top four oil crops in the world. The main storage form of vegetable oil is the triglycerides (about 95-98%), which are composed of fatty acids (FAs) and glycerol [Citation1]. FAs from plants include saturated FAs (C16:0, C18:0, C22:0, etc.), monounsaturated FAs (C18:1, C22:1, etc.) and polyunsaturated FAs (C18:2, C18:3, C20:4, etc.) [Citation2], in which polyunsaturated FAs is essential for human life. Some FAs are beneficial to human health, such as C18:1 and C18:2, which can effectively prevent coronary heart disease. On the contrary, long-chain saturated fatty acids (LCFA) containing 12:0-16:0 chain length would increase serum total cholesterol. High intake of these FAs will increase the level of cholesterol in the blood, and excessive intake of saturated fats is thought to contribute to the development of heart disease, weight gain and obesity [Citation3]. The oil quality and functionality mostly depend on the FAs composition. Therefore, a reliable quantification method to determine FAs in oil crops is extremely demanded.
Total FAs in edible oil could be traditionally detected by titrimetry as recommended by the American Oil Chemists’ Society (AOCS) [Citation4]. Liquid chromatography-mass spectrometry (LC-MS) has been also used for lipids analysis, but intensive sample purification for the enrichment of FAs is usually required before analysis in the complex fatty matrices. Gas chromatography (GC) [Citation5], gas chromatography-flame ionization detection (GC-FID) [Citation6, Citation7] and gas chromatography-mass spectrometry (GC-MS) [Citation8–10] have been previously applied for FAs determination. In GC and GC-FID analysis, FAs composition is identified only by retention time without any information on molecular mass or other structural characteristics that can be used to discriminate between various FAs, which lead to some misinformation. The combination of mass spectrometry (MS) with GC could produce precise identification results for FAs composition by use of electron ionization. So GC-MS is frequently used for the identification and quantification of FAs in plants and animals in recent years, owing to its effectiveness and high sensitivity [Citation11, Citation12]. The main challenges for FAs assay include the unstable retention time and difficult separation, since these long-chain FAs with high polarity [Citation13] and high boiling points (BP) are not directly volatilizable under general conditions. Generally, derivatization steps are required in GC-MS analysis. FAs needed to be methylated and converted into fatty-acid methyl esters (FAMEs), being turned into volatiles at low BP, thus providing higher resolution for fatty-acid peaks under reduced vaporization temperature [Citation11, Citation14]. As a result, the methylation derivatization of FAs plays a vital role for FAs analysis. The methylation methods for FAs include acid catalysis (i.e. the method of converting FAs into FAMEs by use of reaction mixture buffer of sulfuric acid or hydrochloric acid and methanol) [Citation5, Citation6], base catalysis [Citation7] and other methods. The advantages and disadvantages of FA methylation methods are summarized in . Currently, the acid-catalyzed methylation method has been widely used to analyze FAs in plant samples, among which the methylation using sulfuric acid with methanol (5%, v/v) has been widely used for Arabidopsis thaliana seeds and oilseeds. The acid catalysis operations reported in previous literature rources were complicated with high reaction temperature and complex instruments, by which the experimental efficiency was limited. The temperature and time of the methylation was 90-95 °C and 1.5 h, respectively [Citation8, Citation20, Citation21]. In order to improve the efficiency of acid-catalysis, the reaction temperature was usually set to 70-100 °C [Citation8–10, Citation22–24]. It is noteworthy that the conjugated linoleic acids profile would alter via geometric isomerization at high temperature by acid-catalyzed methylation, which led to a decrease in cis/trans C18:2c9t11 and an increase in trans/trans C18:2t9t11 [Citation15, Citation25]. Concurrently, a tightly sealed reaction container is required (e.g. Teflon-lined screw cap glass tube and vacuum rotary evaporator) in the process of methylation at high temperature [Citation8–10, Citation20, Citation21, Citation23, Citation24], which leads to low efficiency for experiment operation, and it is impossible to carry out mass sample methylation at the same time. Therefore, a simple, efficient and gentle FAs methylation method needs to be explored.
Table 1. Comparison of different methods of fatty acid methylation.
The objective of the present work was to develop a one-step acid-catalyzed methylation method based on sulfuric acid catalysis [Citation20] for the determination of oil content and FAs composition in Arabidopsis thaliana seeds. With less seed sample in a small sized 2-mL Eppendorf (EP) tube as an alternative reaction container, we demonstrated that FAs can be well methylated at a lower temperature (64 °C). The present method was validated by using different oilseeds (e.g. Brassica juncea seeds, Arachis hypogaea, Sesamum indicum, Helianthus annuus, and Glycine max).
Materials and methods
Reagents
Analytical grade sulfuric acid was purchased from Huafu Chemical (Jiangsu, China), Chromatographic grade methanol and n-hexane were purchased from Merck (USA) and TEDIA (USA), respectively. The carrier gas was high purity helium (99.9999%, Saizhong Ltd. Changsha, China) for GC-MS. The volatile fatty acid standards of palmitic acid (≥99.0%), hexadecenoic acid (cis-9) (≥99.0%), stearic acid (≥99.5%), octadecenoic acid (cis-9) (≥99.0%), oleic acid (≥99.0%), linolenic acid (≥99.0%), arachidic acid (≥99.0%) and erucic acid (≥99.0%) were purchased from Aladdin (Shanghai, China). The mixed FAs standard solution (6.7 μg/μL C16:0, 0.7 μg/μL C16:1, 2.7 μg/μL C18:0, 10.9 μg/μL C18:1n9c, 24.7 μg/μL C18:2n6c, 1.35 μg/μL C20:0, 16.75 μg/μL C18:3n6, 1.0 μg/μL C22:1n9) was prepared in n-hexane and kept at −20 °C in the dark.
Sampling
Seeds of Arabidopsis thaliana ecotype Columbia (Col-0) were surface-sterilized in 70% alcohol for 15 min, followed by washing in sterile distilled water 3-5 times. Sterilized seeds were dispersed in 0.1% agar and plated on 1/2 MS medium solidified by 0.8% agar. Seeds were placed at 4 °C for 3 days of vernalization, and then plants were grown at 22°C under 16 h light/8 h dark [Citation26, Citation27]. Then, 7-day old seedlings were transplanted into soil and kept under the same conditions. Eight weeks later, mature seeds were harvested, cleaned and dried. Brassica juncea, Sesame (Sesamum indicum), peanut (Arachis hypogaea), edamame (Glycine max) and sunflower (Helianthus annuus) seeds were purchased from the local market. The seeds were well ground in liquid nitrogen because of their thick testa. Five milligrams of homogenized seeds were weighed and transferred to a 2 -mL EP tube (no mixed standards added) for further operations.
Preparation of calibration curve
Ten FAs (C16:0, C16:1, C18:0, C18:1, C18:2, C18:3, C20:2, C20:1, C20:2 and C22:1) were quantitatively analyzed using mixed FAs standard solution containing 37 methylated FAs (Sigma-Aldrich, 18919-1AMP). A calibration curve was constructed using a standard solution of 37 mixed methylated FAs [Citation28, Citation29] at 7 different concentrations ranging from 0.8 to 25 μg/μL(0.8, 1, 2, 4, 8, 10, and 25 μg/μL).
Preparation of FAME
High temperature acid-catalyzed method: 10 mg intact A. thaliana seeds were weighed on an analytical balance and were placed into a 10 mL round bottom capped glass tube, then 1 mL sulfuric acid with methanol (5%, v/v) was added, and 20 μL of mixed FAs standards for quantification was also added into the mixture. Afterwards, the mixture was subjected to vortexing. The methylation reaction was performed at 85 °C for 2 h [Citation5, Citation6]. After cooling to room temperature, FAMEs were extracted with 800 μL of hexane. The mixture was vortexed for 30 s and then left to stand for 2 min. Finally, the supernatant of organic phase was collected and filtered for GC-MS analysis.
Lower temperature acid-catalyzed method: 10 mg of A. thaliana seeds or indicated weight (0.5, 1, 2, 5, and 10 mg) were transferred to a 2-mL EP tube, the seeds were mixed with 1 mL of sulfuric acid with methanol (5%, v/v) and 20 μL of mixed standards. Then the tube was vortexed for 5 s and heated at the indicated temperature (25 °C, 37 °C, 42 °C and 64 °C) or 64 °C for the indicated time (2, 4, 8, 12 and 16 h). After cooling to room temperature, FAMEs were extracted with 800 μL of hexane. The extraction mixture was again vortexed for 30 s and then was centrifuged at 12,000 g for 2 min. The upper organic layer containing the FAME was transferred to a vial and stored at −20 °C for the subsequent GC-MS analysis.
GC-MS analysis
The concentration of FAMEs was determined by GC-MS. GC-MS analyses were carried out with a GC MS-TQ8050 (Shimadzu Corporation, Japan) equipped with a SH-Rt-2560 column sized as 100 m × 0.25 mm × 0.25 μm (Shimadzu Corporation, Japan). GC inlet temperature was 240 °C. The total flow rate, column flow rate, linear velocity and purge flow rate were 100.0 mL/min, 1.50 mL/min, 25.2 cm/s, and 3.0 mL/min, respectively. The oven temperature program employed for separation of FAMEs was as follows: the initial temperature of 125 °C was increased to 180 °C at an increment of 10 °C/min, held for 2 min, and then programmed at 200 °C at a rate of 3 °C/min, held for 20 min and finally increased to 230 °C at 5 °C/min for 10 min. The injection volume was 1.0 μL and splitless. The ion source (electron ionization, EI) detector temperature was maintained at 240 °C, and the solvent delay was set at 12 min. The MS system was run in a scan mode of acquisition for selection of appropriate EI mass fragments for each analysis [Citation11, Citation23].
Results
Establishment of calibration curve
In order to study the efficiency of our pretreatment method, firstly the calibration curve was established and the GC-MS analysis conditions of each component were determined. Ten kinds of common FAs in vegetable oil (C16:0, C16:1, C18:0, C18:1, C18:2, C18:3, C20:2, C20:1, C20:2 and C22:1) were analyzed. As depicted in , the calibration curves of all the analytes exhibited good linear determination coefficients R2 (0.9985-0.9999), which demonstrated good linear relationship between the concentration of FA (y) and peak area (x) in GC-MS detection among linear ranges. In this work, the linear range for C16:0 was 0.048 − 1.5 μg/μL, for C18:0, C18:1 and C20:1 it was 0.032 − 1 μg/μL, and for C16:1, C18:2, C18:3, C20:0, C20:2 and C22:1 it was 0.016 − 0.5 μg/μL, respectively). The electron ionization mass spectrum of the 10 FAs is shown in .
Figure 1. GC/MS chromatogram of derivatized methanol for the ten FAs. C16:0, C16:1, C18:0, C18:1, C18:2, C20:0, C20:1, C18:3, C20:2, C22:1.
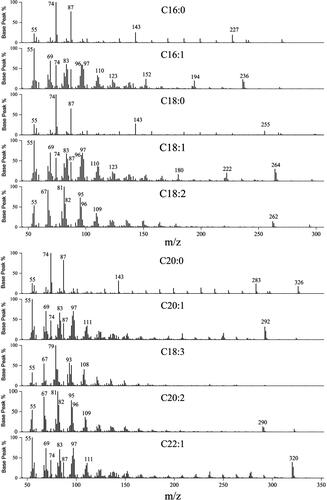
Table 2. Performance of the proposed method for ten FAs.
Optimization of derivatization conditions
Comparison of the recoveries between the two methods
In order to establish an efficient method for FAs methylation, A. thaliana seeds were used as the experimental material to optimize the experimental conditions including reaction temperature, time and reaction container. Considering the influence of methanol volatilization on methylation efficiency, we firstly chose 64 °C, which is below the methanol volatilization point (64.7 °C), as the test temperature for our experiment. The recovery rates of FAs were compared in the case of 85 °C and 64 °C methylation temperatures and the reaction took 2 h (85 °C) in a 10-mL round bottom capped glass tube and 4 h (64 °C) in a 2-mL EP tube, respectively, as described in the Materials and Methods section () [Citation30]. In previous studies, the temperature of FAs methylation ranged from 70 °C to 95 °C, at which the experimental vessels with good sealing (e.g. Teflon-lined screw cap glass tube, vacuum rotary evaporator) were required (). Therefore, a large number of samples cannot be placed at once. As shown in , the recoveries of most FA components (C16:1, C18:1, C18:2, C18:3 and C22:1) at 64 °C were a little higher than or close to the recoveries at 85 °C. Although the recoveries of some FA components (C16:0, C18:0 and C20:0) at 64 °C were a little lower than those at 85 °C, the recoveries of the three FAs were in accordance with the standards of 80-120%, so it is feasible to choose 64 °C as the methylation temperature. Moreover, 2-mL EP tube can be applied as the optimum selection for a reaction container with low cost and high experimental efficiency in the laboratory.
Figure 2. Scheme of the FAME extraction at different temperatures. Previous fatty acid methylation program (reaction at 85 °C for 2 h in Teflon-lined screw cap glass tube by use of Thermostatic water bath) (A), Optimized fatty acid methylation program (reaction at 64 °C for 4 h in a 2-mL EP tube by use of a constant temperature drying oven) (B). The red box illustrates the difference in sample throughput between the two methods.
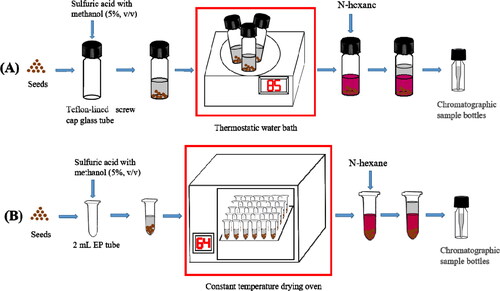
Table 3. Recoveries of FAs composition at different methylation temperatures.
Optimization of methylation temperature
The recoveries at 64 °C and 85 °C were compared in the previous part. The results showed that the better recoveries can be obtained by lowering the temperature to 64 °C. We compared the FAs methylation results at 25 °C (close to room temperature), 37 °C (the general optimal temperature for enzymes), 42 °C (often used in molecular experiments) and 64 °C. As shown in , two to four of the ten target FAs could not be detected when the methylation temperature was 25 °C, 37 °C or 42 °C. However, the ten target FAs could be detected at 64 °C, and the relative contents of FAs were similar with earlier reports [Citation5, Citation20]. Therefore, the subsequent optimization experiment is based on the methylation temperature of 64 °C.
Optimization of methylation time
At the optimized methylation temperature of 64 °C, we further explored the effect of methylation time (2 h, 4 h, 8 h, 12 h and 16 h) on FAs analysis. After methylation for 2 h, FAs with lower abundance such as C20:2 and C22:1 could not be detected. However, 10 FAs were detected after methylation for 4 h, 8 h, 12 h, 16 h, and the relative contents of FAs () were similar to previous reports [Citation20, Citation31]. Considering the reliability and experimental efficiency, 4 h was chosen to complete the FAs methylation.
Figure 4. Relative content of FAs composition in Arabidopsis thaliana seeds with different methylation time. NA: not available. The error bars represent the standard deviation of the measurements (n = 3).
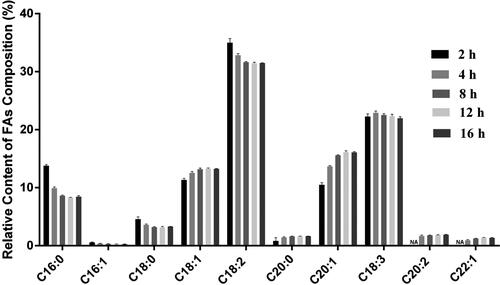
Determination of sample usage on the quantification of FAs in Arabidopsis thaliana seeds. The relative contents of FAs composition respectively in 0.5, 1, 2, 5 and 10 mg of A. thaliana seeds were analyzed. The result in demonstrated that C16:1, C20:2 and C22:2 could not be detected in 0.5 mg and 1 mg seeds, neither C22:1 in 2 mg seeds. However, all 10 target FAs could be detected in 5 mg and 10 mg seeds (), and the relative contents of FAs were similar with earlier reports [Citation5, Citation20, Citation31]. In conclusion, both 5 mg and 10 mg seeds were used as sample amount for GC-MS analysis, and 5 mg could be suitable for FAs analysis of precious seeds.
Application of the method in different seeds
Now that we had constructed a new method for FAs methylation, we also studied whether it can be applied in other oil seeds. Soybean (Glycine max), rapeseed (Brassica napus), peanut (Arachis hypogaea) and sunflower (Helianthus annuus) are the top 4 oil crops in the world, and sesame(Sesamum indicum) is also rich in oil, so the above 5 kinds of seeds were chosen as material for the method application experiment. Because these seeds are larger than A. thaliana seeds, and the seed coat may have an inhibitory effect on FAs methylation, these oilseeds were first ground with liquid nitrogen and then 5 mg were weighed out for the methylation reaction. The differences in the oil content and FAs types in these oilseeds are respectively presented in and . Seven FAs were detected in Glycine max, 10 target FAs in Brassica juncea seeds, and 6 FAs in Arachis hypogaea, Helianthus annuus and Sesamum indicum seeds, respectively, and there were obvious differences in their relative contents. The FAs content of Sesamum indicum was the highest, followed by Arachis hypogaea, Helianthus annuus, Brassica juncea and Glycine max. The oil contents of these oilseeds were consistent with previous reports [Citation7, Citation13], and the majority of the 10 target FAs in the oilseeds could be detected [Citation7], such as C16:0, C18:0, C18:1, C18:2, C20:0 and C18:3. Moreover, Brassica juncea seeds are richer in erucic acid (C22:1) [Citation32] than other oilseeds analyzed in this study (). It was found that the present method is also suitable for the FAs analysis of different plant seeds (e.g. Brassica juncea seeds, Arachis hypogaea, Sesamum indicum, Helianthus annuus and Glycine max).
Figure 6. GC-MS spectrum for the FAMEs of five plant materials: Brassica juncea (a); Glycine max (b); Helianthus annuus (c); Arachis hypogaea (d); Sesamum indicum (e). FAMEs: (1) C16:0; (2) C16:1; (3) C18:0; (4) C18:1; (5) C18:2; (6) C20:0; (7) C20:1; (8) C18:3; (9) C20:2; (10) C22:1.
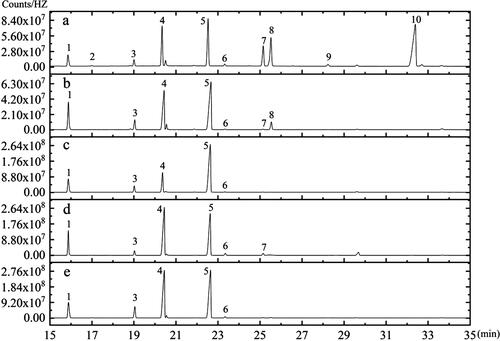
Table 4. FAs composition and total FAs contents in different materials.
Discussion
In this study, we optimized and validated a one-step acid-catalyzed methylation method in treatment conditions including reaction container, temperature, time and sample usage. Firstly, Arabidopsis thaliana were selected as experimental materials for method optimization, which provides an ideal system for the study of lipid biosynthetic and metabolism pathways in oilseeds, due to its popularity as a model plant and close relationship with Brassica napus, a major oilseed crop [Citation33, Citation34].
The fatty acid pretreatment methods in the previous literature require the use of well-sealed containers at high temperatures, such as rotary evaporators, Teflon-lined screw cap glass tubes, etc., which is the key point to limit the efficiency of the previous methylation methods. This prompted us to explore whether the efficiency could be improved by reducing the reaction temperature and prolonging the reaction time. Considering that while reducing the reaction temperature, the requirement for tight sealing of the reaction vessel is not as high as the previous method, we used common EP tubes. The comparison of the results of FA methylation by the use of the previous method (reaction at 85 °C for 2 h in 10-mL round bottom capped glass tubes) with that of our method (reaction at 64 °C for 4 h in the 2-mL EP tube), our new method with a lower reaction temperature (64 °C) and a more convenient container (2-mL EP tube) could work well (). Taking 2-mL EP tubes instead of rotary evaporators or Teflon-lined screw cap glass tubes as reaction containers in FA methylation pretreatment, the number of samples that can be processed is not subject to the conditions of equipment and containers, and specific sample quantity can be changed at any time according to experimental requirements, so the experimental efficiency is improved. In addition, lower reaction temperature means more safety for the operators and the environment ().
Although 10 mg A. thaliana seeds were usually recommended for seed oil detection in previous studies [Citation10, Citation24], a microscale sample preparation method would be advantageous for the small sized A. thaliana seeds. The data showed that both 5 mg and 10 mg seeds were used as a sample amount for GC-MS analysis, and 5 mg could be suitable for FAs analysis of seeds. In addition, the method in this study was applied to detect the FAs composition of Brassica juncea seeds, Arachis hypogaea, Sesamum indicum, Helianthus annuus and Glycine max successfully (), which suggests that this method also can be introduced to detect other plants.
In order to illustrate the difference and the advantage of our method, the reported methods based on acid catalysis are summarized in focusing on comparing the methylation temperature, time, reaction device and sample size. As shown in , the method in our work with a simpler vessel, lower temperature and smaller sample size would improve the efficiency of the experiment.
Table 5. Comparison of acid catalysis methylation methods for FAs in plant samples.
Conclusions
This study proposed a simplified and high throughput methylation method followed by GC-MS analysis for analysis of FAs in oilseeds based on one-step acid-catalyzed methylation. The proposed method is a successful approach to increase the efficiency of sample preparation and analysis for large-scale experiments.
Disclosure statement
No potential conflict of interest was reported by the authors.
Data availability
All data that support the findings reported in this study are available from the corresponding author upon reasonable request.
Additional information
Funding
References
- Thomas A, Matthäus B, Fiebig HJ. Fats and fatty oils. In: Ullmann’s encyclopedia of industrial chemistry. Vol. 14. Weinheim: Wiley-VCH Verlag GmbH & Co. KGaA; 2015. p. 1–84.
- Karaman M, Atlagić K, Novaković A, et al. Fatty acids predominantly affect anti-hydroxyl radical activity and FRAP value: the case study of two edible mushrooms. Antioxidants. 2019;8(10):480–491.
- Haug A, Høstmark AT, Harstad OM. Bovine milk in human nutrition-a review. Lipids Health Dis. 2007; 6:25–41.
- Berner D. Aocs’ 4th edition of methods. J Am Oil Chem Soc. 1989;66(12):1749–1749.
- Chen MX, Wang Z, Zhu YN, et al. The effect of transparent TESTA2 on seed fatty acid biosynthesis and tolerance to environmental stresses during young seedling establishment in Arabidopsis thaliana. Plant Physiol. 2012;160(2):1023–1036.
- Chen MX, Du X, Zhu Y, et al. Seed fatty acid reducer acts downstream of gibberellin signalling pathway to lower seed fatty acid storage in Arabidopsis thaliana. Plant Cell Environ. 2012;35(12):2155–2169.
- Chernova A, Mazin P, Goryunova S, et al. Ultra-performance liquid chromatography-mass spectrometry for precise fatty acid profiling of oilseed crops. PeerJ. 2019; 7:e6547–6543.
- Lee EJ, Oh M, Hwang JU, et al. Seed-specific overexpression of the pyruvate transporter BASS2 increases oil content in Arabidopsis Seeds. Front Plant Sci. 2017; 8:194–204.
- Poirier Y, Ventre G, Caldelari D. Increased flow of fatty acids toward beta-oxidation in developing seeds of Arabidopsis deficient in diacylglycerol acyltransferase activity or synthesizing medium-chain-length fatty acids. Plant Physiol. 1999;121(4):1359–1366.
- Mu JY, Tan HL, Zheng Q, et al. LEAFY COTYLEDON1 is a key regulator of fatty acid biosynthesis in Arabidopsis thaliana. Plant Physiol. 2008;148(2):1042–1054.
- Quehenberger O, Armando AM, Dennis EA. High sensitivity quantitative lipidomics analysis of fatty acids in biological samples by gas chromatography-mass spectrometry. Biochim Biophys Acta. 2011;1811(11):648–656.
- Hoving LR, Heijink M, van Harmelen V, et al. GC-MS analysis of short-chain fatty acids in feces, cecum content, and blood samples. Methods Mol Biol. 2018; 1730:247–256.
- Qu S, Du Z, Zhang Y. Direct detection of free fatty acids in edible oils using supercritical fluid chromatography coupled with mass spectrometry. Food Chem. 2015; 170:463–469.
- Matsumoto Y, Ando Y, Hiraoka Y, et al. A simplified gas chromatographic fatty-acid analysis by the direct saponification/methylation procedure and its application on wild tuna larvae. Lipids. 2018;53(9):919–929.
- Liu Z, Ezernieks V, Rochfort S, et al. Comparison of methylation methods for fatty acid analysis of milk fat. Food Chem. 2018; 261:210–215.
- Christie WW. Lipid analysis. 2nd ed. Oxford: Pergamon Press; 1982.
- Kamga AW, Behar F. Hatcher P.G. Quantitative analysis of long chain fatty acids present in a Type I kerogen using electrospray ionization Fourier transform ion cyclotron resonance mass spectrometry: compared with BF3/MeOH methylation/GC-FID. J Am Soc Mass Spectrom. 2014; 5:880–890.
- Antolín EM, Delange DM, Canavaciolo VG. Evaluation of five methods for derivatization and GC determination of a mixture of very long chain fatty acids (C24:0-C36:0). J Pharm Biomed Anal. 2008;46(1):194–199.
- Park Y, Albright KJ, Cai ZY, et al. Comparison of methylation procedures for conjugated linoleic acid and artifact formation by commercial (trimethylsilyl) diazomethane. J Agric Food Chem. 2001;49(3):1158–1164.
- Li Y, Beisson F, Pollard M, et al. Oil content of Arabidopsis seeds: the influence of seed anatomy, light and plant-to-plant variation. Phytochemistry. 2006;67(9):904–915.
- Ettaki H, Troncoso-Ponce MA, To A, et al. Overexpression of MYB115, AAD2, or AAD3 in Arabidopsis thaliana seeds yields contrasting omega-7 contents. Plos One. 2018;13(1):e0192156.
- Pleite R, Martínez-Force E, Garcés R. Increase of the stearic acid content in high-oleic sunflower (Helianthus annuus) seeds. J Agric Food Chem. 2006;54(25):9383–9388.
- Liu F, Xia Y, Wu L, et al. Enhanced seed oil content by overexpressing genes related to triacylglyceride synthesis. Gene. 2015;557(2):163–171.
- Epp MD, Pollard MR. Fatty acid content and composition of Oenothera hookeri seeds containing mutant plastids. Lipids. 1993;28(11):1005–1009.
- Kramer JK, Fellner V, Dugan ME, et al. Evaluating acid and base catalysts in the methylation of milk and rumen fatty acids with special emphasis on conjugated dienes and total trans fatty acids. Lipids. 1997;32(11):1219–1228.
- Chen D, Hao F, Mu H, et al. S-acylation of P2K1 mediates extracellular ATP-induced immune signaling in Arabidopsis. Nat Commun. 2021;12:2750.
- Jarsch IK, Konrad SS, Stratil TF, et al. Plasma membranes are subcompartmentalized into a plethora of coexisting and diverse microdomains in Arabidopsis and Nicotiana benthamiana. Plant Cell. 2014;26(4):1698–1711.
- Pejin B, Bianco A, Newmaster S, et al. FAs of Rhodobryum ontariense (Bryaceae). Nat Prod Res. 2012;26(8):696–702.
- Zhang L, Li P, Sun X, et al. Classification and adulteration detection of vegetable oils based on fatty acid profiles. J Agric Food Chem. 2014;62(34):8745–8751.
- Chen M, Xuan L, Wang Z, et al. TRANSPARENT TESTA8 inhibits seed fatty acid accumulation by targeting several seed development regulators in Arabidopsis thaliana. Plant Physiol. 2014;165(2):905–916.
- Lagercrantz U, Putterill J, Coupland G, et al. Comparative mapping in Arabidopsis and Brassica, fine scale genome collinearity and congruence of genes controlling flowering time. Plant J. 1996;9(1):13–20.
- Murphy DJ. Engineering oil production in rapeseed and other oil crops. Trends Biotechnol. 1996;14(6):206–213.
- Lee J-B, Jeong YA, Ahn DJ, et al. SPME-GC-MS analysis of methanol in biospecimen by derivatization with pyran compound. Molecules. 2019;25(1):41–52.
- Sharafi Y, Majidi MM, Goli SAH, et al. Oil content and fatty acids composition in Brassica species. Int. J. Food. Prop. 2015;18(10):2145–2154.
- Salimon J, Abdullah BM, Salih N. Hydrolysis optimization and characterization study of preparing fatty acids from Jatropha curcas seed oil. Chem Cent J. 2011; 5:67–75.
- Ahmadvand M, Sereshti H, Parastar H. Second-order calibration for the determination of fatty acids in pomegranate seeds by vortex-assisted extraction-dispersive liquid–liquid micro-extraction and gas chromatography-mass spectrometry. RSC Adv. 2015;5(15):11633–11643.