Abstract
The present study investigated the expression signature of the MINA53 gene in 50 lung adenocarcinoma (LUAD) and 50 squamous cell lung carcinoma (LUSC) tumors and their adjacent non-tumor tissues via RT-qPCR. Association analysis was performed to explore the correlation between the levels of the MINA53 and the clinicopathological characteristics of the patients. The MINA53 gene expression was upregulated in early-stage tumors with poor tumor differentiation, without lymph nodes and distant metastases. To explore the potential role of MINA53 in carcinogenesis, we evaluated the expression pattern of five known lung cancer-associated genes: EGFR, mTOR, PTEN, STAT3 and PD-L1. The regression analysis showed a correlation between MINA53 expression and EGFR in LUAD and PD-L1 and STAT3 in LUSC. This suggests a distant oncogenic role of MINA53 in both subtypes of NSCLC and opens up the potential for development of new therapeutic strategies and the potential role of MINA53 as a prognostic and predictive biomarker.
Keywords:
Introduction
In the last decades, lung cancer (LC) is the second most common malignancy in both women and men and is the leading cause of death from cancer worldwide [Citation1]. Histologically LC is divided into two major types: non-small cell lung cancer (NSCLC) and small cell lung cancer. Further, they could be subdivided based on their driver mutations and expression profiles [Citation2]. In recent years, it has been shown that epigenetic modifications of DNA and histone proteins, especially on the lysine residues in the N-terminal tails of histones H4 and H3, alter the gene expression and contribute to tumor biology. They affect the accessibility of the chromatin by chromatin-binding proteins, various transcription factors and proteins involved in DNA replication and repair complexes [Citation3,Citation4]. Ionizing radiation, chemical agents, heavy metals, arsenic, mineral dust and tobacco smoke have been referred to as group I IARC human carcinogens [Citation5]. These environmental factors modify normal lung cells genetic and epigenetic profiles and lead to inflammation, fibrosis and cancers [Citation6].
The mineral dust-induced gene, or MYC Induced Nuclear Antigen 53 (also named mdig, MINA53/MINA, NO52, JMJD10, RIOX2), was first identified in alveolar macrophages from miners working in the coal mining industry [Citation7]. MINA53 gene is located on chromosome 3q12.1 and encodes a 53-kDa protein, found in the nucleus and nucleolus [Citation8]. The MINA53 protein contains a JmjC domain, a signature motif for JmjC family histone H3K9me3 demethylases and can promote activation of proto-oncogenes [Citation9]. Also, MINA53 can act as ribosomal oxygenase by hydroxylating the His39 residue of Rpl27a and may be involved in ribosome biogenesis, likely during the assembly process of pre-ribosomal particles [Citation10]. Recent studies have reported increased expression of MINA53 in various cancer types, including lung and breast cancer [Citation11], glioblastoma [Citation12], colon cancer [Citation8], esophageal squamous cell carcinoma [Citation13], lymphoma [Citation14], renal cell carcinoma [Citation15], hepatocellular carcinoma [Citation16], pancreatic cancer [Citation17], multiple myeloma [Citation18] and gastric cancer [Citation19]. However, the exact mechanism of action of MINA53 is not yet well understood. It has been shown that MINA53 can act as an oncogene by promoting cancer cell proliferation, tumorigenicity [Citation20], pulmonary inflammation [Citation21] and immune response [Citation20,Citation22]. Interestingly, a paradoxical role of MINA53 was observed on cell growth and invasion, depending on the stage and type of cancer. A detailed analysis by Thakur et al. [Citation23] for breast cancer showed increased expression of MINA53 in early stages of breast cancers and some subtypes of noninvasive breast cancers, such as medullary carcinoma, ductal carcinoma in situ and mucinous carcinoma. However, the mdig expression was lost in the invasive TNBC carcinoma and in cancer cells that metastasized to lymph nodes [Citation23]. In lung cancer, the prognostic value of MINA53 levels was different depending on the histological subtype and stages as well, as MINA53 predicted poorer first progression survival of lung adenocarcinomas, but not squamous cell carcinoma [Citation6,Citation24]. Further functional analysis in enlarged samples and validation is necessary to ascertain better the biological function and prognostic potential of MINA53 in NSCLC.
Komiya et al. [Citation25] analyzed cell lines transfected with MINA53 using gene expression microarray and discovered 125 upregulated and 129 downregulated genes. Among them, 59 were involved in cell invasion and metastasis, 34 were associated with transcriptional regulation, 20 genes were associated with cancer metabolism, 17 were associated with growth factors, 12 were associated with cell cycle and proliferation [Citation25].
Aziz et al. used a different approach and performed functional enrichment analysis of gene sets co-expressed with MINA53 in gastric cancer by data acquisition and computation analysis of publicly available datasets. The enriched GO-term and KEGG gather in 12 clusters such as tRNA regulation of protein translation; cell division regulation; spliceosome; mRNA processing; intracellular transport of virus and protein transporter activity; ER and Golgi vesicle-mediated transport; DNA repair and regulation of signal transduction by p53; T-cell/C-type lectin/Fc-epsilon receptor signaling pathway; protein kinase activity, cell cycle, and regulation of apoptotic processes; structural constituent of ribosomes and mitochondrial translational elongation/termination; anaphase-promoting complex-dependent catabolic process and cell-cell adhesion [Citation26].
The present study aimed at analyzing the expression patterns of MINA53 and a set of five selected mRNAs, members of major signaling pathways in the two main subtypes of NSCLC: adenocarcinoma (LUAD) and squamous cell lung cancer (LUSC). Association and regression analysis were performed to explore the correlations further between the MINA53 and several known biomarkers in NSCLC such as EGFR, mTOR, PTEN, STAT3 and PD-L1 and their role in LUAD and LUSC.
Materials and methods
Ethics statement
The study was approved by the Ethics Committee of the Medical University of Sofia and all participants gave written informed consent.
Patients and tissue specimens
Fresh-frozen tumor and normal tissues were obtained from a total of 100 patients diagnosed with NSCLC. Patients were recruited at the University Hospital for Pulmonary Diseases ‘St. Sofia’ during the period 2009–2017. Tissues were quickly frozen and stored at −80 °C until RNA extraction at the Molecular Medicine Center biobank at the Department of Medical Chemistry and Biochemistry, Medical University of Sofia. The samples were evaluated by an experienced pathologist and diagnosed as adenocarcinoma or squamous cell lung carcinomas (n = 100; 50 LUAD, 50 LUSC). The clinical characteristics of NSCLC patients are presented in and .
Table 1. Association between mean RQ levels of PD-L1, STAT3, PTEN, EGFR, MINA53, mTOR and clinicopathological characteristics in patients with LUAD.
Table 2. Association between mean RQ levels of PD-L1, STAT3, PTEN, EGFR, MINA53, mTOR and clinicopathological characteristics in patients with LUSC.
RNA isolation, cDNA synthesis and real-time polymerase chain reaction (PCR)
Total RNA from fresh frozen tissues was extracted using an RNeasy mini kit (Qiagen, Germany) in accordance with the manufacturer’s instructions. The quality and quantity of the isolated RNA samples were quantified using NanoDrop (Thermo Fisher Scientific, United States) and Qubit (Thermo Fisher Scientific, United States). For reverse transcription reaction with the QuantiTect Reverse Transcription Kit (Qiagen, Germany), 250 ng of each sample were used. We performed quantitative real-time reverse transcription PCR (RT-qPCR) using SYBR technology (Qiagen, Germany), QuantiTect SYBR Green PCR Kit and QuantiTect Primer Assay (Qiagen, Germany) on a 7900 HT Fast Real-Time PCR (Applied Biosystems). ACTB gene was used as a reference control for normalization. Each reaction was performed in triplicate, according to the manufacturer’s protocol. Relative quantification (RQ) of the gene expression levels of PD-L1, STAT3, PTEN, EGFR, MINA53 and mTOR was done using the 2-ΔΔCt method. The RQ value reveals how the expression in tumour tissues has changed compared to the adjacent non-tumor tissues. RQ value ≤0.5 was interpreted as underexpression, RQ ≥2 as overexpression, and RQ between 1.99 and 0.5 as no change in expression of the studied mRNAs.
Statistical analysis
Data analysis was carried out with SPSS Statistics v. 23 for Windows (IBM SPSS, NY, USA). The distribution of the data was tested with the Kolmogorov-Smirnov test for normality. Mann Whitney U test and Kruskal-Wallis were used as appropriate, and differences were considered statistically significant at a level of p ≤ 0.05. The relationship among the expression levels of PD-L1, STAT3, PTEN, EGFR, MINA53 and mTOR was examined using linear regression.
Results
In this study, 50 patients with LUAD (33 males and 17 females) and 50 patients with LUSC (42 males and 8 females) were included. The clinicopathological characteristics of the patients are shown in and , along with the expression levels of the analyzed mRNAs.
The expression levels of all investigated mRNAs in LUAD samples were significantly altered in tumor tissue compared to the adjacent non-tumor tissues. PD-L1 (p = 0.002), STAT3 (p = 0.032), PTEN (p = 0.019), EGFR (p = 0.038) levels were significantly downregulated, and MINA53 (p = 0.001) and mTOR (p = 0.001) were upregulated in LUAD (). In LUSC, the expression levels of PTEN were not significantly different compared to normal tissue. PD-L1 (p = 0.033), EGFR (p = 0.026), MINA53 (p = 0.013) and mTOR (p = 0.029) were significantly upregulated, and STAT3 (p = 0.003) was downregulated in LUSC tissues (). Log2RQ values for box plot preparation and Mann-Whitney U test for evaluating the level of significance were used.
Figure 1. mRNA expression levels. (A) Comparison between the levels of expression in LUAD (brick) and adjacent non-tumor tissues (turquoise) by RT-qPCR; (B) Comparison between the levels of expression in LUSC (brick) and adjacent non-tumor tissues (turquoise) by RT-qPCR; (C) Comparison between the levels of expression in LUAD (brick) and LUSC (turquoise).
Note: Criteria: Mann–Whitney U test was performed to evaluate the significance of expression level differences, p ≥ 0.05 consider as non-significant, *: p ≤ 0.05, **: p ≤ 0.01, ***: p ≤ 0.001, LUAD – lung adenocarcinoma, LUSC – lung squamous cell lung cancer
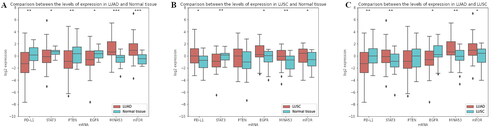
and summarize the associations between RQ data of the studied mRNAs and the clinicopathological characteristics of the LUAD and LUSC patients. In LUAD tumors, we found a statistically significant association between nodal stage and the expression levels of PD-L1 (p = 0.014), mTOR (p = 0.041); between the presence of distant metastases and the expression levels of PD-L1 (p = 0.007), STAT3 (p = 0.045), mTOR (p = 0.038); between tumor differentiation and the expression levels of PD-L1 (p = 0.050), PTEN (p = 0.001) and EGFR (p = 0.006). In LUSC tumors, the expression of PD-L1 (p = 0.029) and EGFR (p = 0.044) displayed a statistically significant association with nodal stage and tumor differentiation. The expression of PTEN correlated with the presence of distant metastases, as well as the expression of STAT3 (p = 0.0001) with tumor differentiation.
We found a significant association between MINA53 and nodal stage, as well as the presence of distant metastases and tumor differentiation in both subtypes of NSCLC. MINA53 expression was significantly decreased in a patients with lymph node (p = 0.007, p = 0.003) and distant metastasis (p = 0.019, p = 0.001) in LUAD and LUSC, respectively. In well-differentiated tumors, the expression of MINA53 was significantly decreased compared to poorly differentiated tumours in both subtypes, although the magnitude of expression was much higher in LUAD compared to LUSC ( and ).
We also performed a linear regression analysis to see the accordance of MINA53 expression with PD-L1, STAT3, PTEN, EGFR and mTOR expression in both subtypes of NSCLC. In LUAD tumors we found a correlation between MINA53 and EGFR (R2 = 0.115, p = 0.018) (). The mRNA levels of PD-L1 (R2 = 0.048, p = 0.134), STAT3 (R2 = 0.019, p = 0.349), PTEN (R2= 0.053, p = 0.116) and mTOR (R2 = 0.0001, p = 0.939) have no significant correlation with the expression level of MINA53. In LUSC tumors the expression levels of MINA53 correlated with PD-L1 (R2 = 0.131, p = 0.032) and STAT3 (R2 = 0.134, p = 0.028) (). The mRNA levels of PTEN (R2 = 0.004, p = 0.773), mTOR (R2 = 0.013, p = 0.523) and EGFR (R2 = 0.108, p = 0.071) had no correlation with the expression level of MINA53.
Figure 2. Linear regression and correlation analysis of the expression levels of (A) MINA53 and EGFR in LUAD samples; (B) MINA53 and PD-L1; (C) MINA53 and STAT3 in patients with LUSC.
Note: Criteria: Linear fit-line, 95% CI band and R2 are shown to illustrate the linear correlation between log2RQ values of both variables. The Pearson’s coefficient (r) and the p-value (p) are displayed.
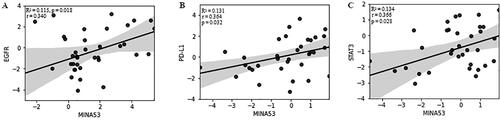
Discussion
The lack of early-detection methods, high frequency of drug resistance, metastasis and invasiveness are significant contributors to the highest mortality incidence of lung cancer. Several factors, including tobacco smoking, other chemicals and heavy metals, DNA damages, genetic alterations, and immune status, orchestrate the complex process of lung cancer development. One of the most significant components that maintain genomic stability is the epigenetic modification of the genomic DNA and histone proteins. Aberrations in histones H3 and H4 methylation patterns have been associated with the initiation and maintenance of lung cancer [Citation27]. Such aberrations could be induced by environmental genes activated due to exposure to health hazards or carcinogens [Citation28]. The current study investigated the expression signature of one such gene, MINA53, in the two subtypes of NSCLC and determined its association with clinicopathological characteristics and correlation with expression levels of known biomarkers in NSCLC.
In the LUAD subtype, MINA53 and mTOR mRNA levels were elevated, and PD-L1, STAT3, PTEN, EGFR expression was downregulated (). In LUSC samples, we discovered that MINA53, PD-L1, PTEN, EGFR and mTOR were with increased expression, and only STAT3 was decreased compared to the adjacent non-tumour tissues (). We found no correlation between the expression of PTEN, mTOR and MINA53.
EGFR is a target for the treatment of patients with NSCLC. Our results confirmed previous findings by Kosaka et al. [Citation29] and Brabender et al. [Citation30] that higher EGFR expression was observed in squamous cell carcinoma than in adenocarcinoma ( and ) [Citation29,Citation30]. After regression analysis in LUAD tumors, we observed a correlation between EGFR and MINA53 gene expression patterns (). The correlation between both biomarkers was also observed in the study of Dan Ma et al. [Citation31]. In cell lines, the overexpression of MINA53 upregulated EGFR levels and stimulated tumour proliferation via regulating the G1 to S phase transition [Citation31].
In our samples, there was no statistically significant difference in the expression of MINA53 between early-stage and advanced-stage LUAD and LUSC. However, the observed patterns showed elevated expression of MINA53 in early-stage LUAD and LUSC ( and ). These findings could be explained by the tumor heterogeneity and distinct morphological subtypes of LUAD and LUSC samples. Komiya et al. [Citation32] show that an elevated expression of MINA53 in stage І-ІІ lung cancer patients is associated with a favorable prognosis. Conversely, the downregulation of MINA53 in the advanced stage of NSCLC induced an invasive phenotype [Citation32]. These results indicated the dual function of MINA53 and its role as an early event in lung cancer carcinogenesis related to the cytokine network such as EGFR, IL-6, and HGF.
Some environmental factors, such as tobacco smoke, silica and arsenic, induce the expression of MINA53 through JNK-STAT3 signaling [Citation33]. STAT3 acted as a transcriptional factor of critical cell cycle regulators and took part in tumor-immune cell communication by reducing effective antitumor immunity through different mechanisms, including regulating PD-L1 gene expression [Citation34,Citation35]. Besides, it mediated the release of various cytokines and chemokines, influencing the tumor microenvironment and tumour-infiltrating lymphocytes [Citation36]. PD-L1 expression was significantly higher in LUSC than LUAD tumors (), and we observed a correlation between the expression levels of STAT3 and PD-L1 with MINA53 (). However, further functional analysis in larger cohorts and validation is necessary to ascertain better the biological function and prognostic potential of MINA53 and its relationship with STAT3 and PD-L1 in LUAD and LUSC.
We found a significantly decreased expression of MINA53 in LUAD and LUSC patients with lymph node and distant metastasis. Our discoveries are in agreement with the previous studies illustrating the role of MINA53 as an early molecular event during lung carcinogenesis [Citation24,Citation32,Citation37]. Geng et al. [Citation37] demonstrated that in lung cancer cell lines, MINA53 expression could inhibit the activation of GSK-3β and benefit the phosphorylation and destabilization of β-catenin, inhibiting the invasion and metastasis through regulating significant molecular markers of EMT.
Another possible mechanism of MINA53 regulation on cell migration and metastasis was proposed by Zhang et al. [Citation10]. MINA53 demethylates H3K9me3 and H3K27me3 and promotes the expression of gene families of PCDH, ECM, PSG, and others involved in cell adhesion, G-protein signaling, or cytoskeleton organization proteins, which may endorse cell invasiveness and metastasis. At the same time, demethylation of both histone molecules induces the expression of IRX family genes that act as essential transcription repressors for the chemokines, antagonizing the cell migration or metastatic potentials [Citation10].
According to Wang et al. [Citation38], MINA53 could interact with XRCC5, XRCC6, RBBP4, CBX8, PRMT5 and TDRD and interfere with the NHEJ repair capacity of DNA double-strand breaks. It will enhance the genomic mutations and facilitate carcinogenesis [Citation38]. Xuan et al. [Citation39] found MINA53 could regulate the expression of essential DNA replication proteins such as CDC45, MCM2-7 and GINS1-4 complexes.
Reduced expression of MINA53 would scale down the expression of those genes and gives the possibility for the development of MINA53 as a molecular target for anti-cancer therapy [Citation39]. The suppression of MINA53 could promote cell apoptosis induced by various chemotherapy drugs, could lead to DNA replication stress due to keeping of the trimethylation of H3, and could suppress the expression of distinct DNA replication factors.
Our data indicated that elevated expression of MINA53 associated with NSCLC poor tumor differentiation, suggesting its vigorous oncogenic activity during the early stages of carcinogenesis ( and ). The methylation of H3 has a significant role in gene silencing, DNA repair, the pluripotency of the stem cells, and cell lineage differentiation through heterochromatin compaction [Citation40]. The elevated expression of MINA53 may lead to reprogramming the somatic cells into stem cells or cancer stem cells by acting as an activator of transcription of the stemness genes Oct3/4, Nanog and Sox2 [Citation41,Citation42]. Zhang et al. confirmed this finding through the CRISPR-Cas9 approach and RNA sequencing. In MINA53 knockout cells, they observed reduced expression of cell proliferative and stemness genes, elevated trimethylation markers on H3 and differentiation of the cancer stem cells [Citation4].
Conclusions
This study identified distinct mRNA expression profiles for LUAD and LUSC. We found MINA53 being upregulated in early-stage tumur stages, without lymph nodes and distant metastases. In addition, the expression of MINA53 correlates with the expression of EGFR in LUAD and with PD-L1 and STAT3 in LUSC. However, our results are based on the expression analysis of MINA53, EGFR, mTOR, PTEN, STAT3 and PD-L1 in bulk tumors, and further bioinformatic and functional analyses are needed to support the potential role of MINA53 as a prognostic and predictive biomarker. Also, this could contribute to understanding the complex mechanisms of lung cancer carcinogenesis and current treatments resistance.
Disclosure statement
No potential conflict of interest was reported by the authors.
Data availability statement
The data that support the findings of this study are available from the corresponding author, (VP), upon reasonable request.
Additional information
Funding
References
- Wild CP, Weiderpass E, Stewart BW. World cancer report: cancer research for cancer prevention. Cancer Control. 2020;199:512.
- Kashima J, Kitadai R, Okuma Y. Molecular and morphological profiling of lung cancer: a foundation for “next-generation” pathologists and oncologists. Cancers. 2019;11(5):599.
- Tian LJ, Wu YP, Wang D, et al. Upregulation of long noncoding RNA (lncRNA) X-inactive specific transcript (XIST) is associated with cisplatin resistance in non-small cell lung cancer (NSCLC) by downregulating microRNA-144-3p. Med Sci Monit. 2019;25:8095–8104.
- Zhang Q, Thakur C, Shi J, et al. New discoveries of mdig in the epigenetic regulation of cancers. Semin Cancer Biol. 2019;57:27–35.
- Field RW, Withers BL. Occupational and environmental causes of lung cancer. Clin Chest Med. 2012;33(4):681–703.
- Shi J, Thakur C, Zhao Y, et al. Pathological and prognostic indications of the mdig gene in human lung cancer. Cell physiol biochem. 2021;55(Suppl 2):13.
- Zhang Y, Lu Y, Yuan B-Z, et al. The human mineral dust-induced gene, mdig, is a cell growth regulating gene associated with lung cancer. Oncogene. 2005;24(31):4873–4882. https://www.nature.com/articles/1208668
- Teye K, Tsuneoka M, Arima N, et al. Increased expression of a myc target gene Mina53 in human Colon cancer. Am J Pathol. 2004;164(1):205–216. http://dx.doi.org/10.1016/S0002-9440(10)63111-2
- Zhou H, Geng F, Chen Y, et al. The mineral dust-induced gene, mdig, regulates angiogenesis and lymphangiogenesis in lung adenocarcinoma by modulating the expression of VEGF-A/C/D via EGFR and HIF-1α signaling. Oncol Rep. 2021;45(5):110001.
- Zhang Q, Thakur C, Fu Y, et al. Mdig promotes oncogenic gene expression through antagonizing repressive histone methylation markers. Theranostics. 2020;10(2):602–614. http://www.thno.org//creativecommons.org/licenses/by/4.0/
- Thakur C, Lu Y, Sun J, et al. Increased expression of mdig predicts poorer survival of the breast cancer patients. Gene. 2014;535(2):218–224.
- Huang MY, Xuan F, Liu W, et al. MINA controls proliferation and tumorigenesis of glioblastoma by epigenetically regulating cyclins and CDKs via H3K9me3 demethylation. Oncogene. 2017;36(3):387–396.
- Tsuneoka M, Fujita H, Arima N, et al. Mina53 as a potential prognostic factor for esophageal squamous cell carcinoma. Clin Cancer Res. 2004;10(21):7347–7356.
- Teye K, Arima N, Nakamura Y, et al. Expression of myc target gene mina53 in subtypes of human lymphoma. Oncol Rep. 2007;18(4):841–848.
- Ishizaki H, Yano H, Tsuneoka M, et al. Overexpression of the myc target gene Mina53 in advanced renal cell carcinoma. Pathol Int. 2007;57(10):672–680.
- Ogasawara S, Komuta M, Nakashima O, et al. Accelerated expression of a myc target gene Mina53 in aggressive hepatocellular carcinoma. Hepatol Res. 2010;40(3):330–336.
- Tan XP, Dong WG, Zhang Q, et al. Potential effects of Mina53 on tumor growth in human pancreatic cancer. Cell Biochem Biophys. 2014;69(3):619–625.
- Wu K, Li L, Thakur C, et al. Proteomic characterization of the world trade center dust-activated mdig and c-myc signaling circuit linked to multiple myeloma. Sci Rep. 2016;6(1):36305–36312.
- Liu H, Guo J, Liu J, et al. Expression of EphA2 and EphrinA1 and its significance in gastric carcinoma. Chinese J Clin Oncol. 2008;35(10):573–577.
- Thakur C, Chen F. Current understanding of mdig/MINA in human cancers. Genes and Cancer. 2015;6(7–8):1–15. www.genesandcancer.com/article/73/text/
- Lian SL, Mihi B, Koyanagi M, et al. A SNP uncoupling mina expression from the TGFβ signaling pathway. Immun Inflamm Dis. 2018;6(1):58–71.
- Hemmers S, Mowen KA. T(H)2 bias: mina tips the balance. Nat Immunol. 2009;10(8):806–808. https://www.nature.com/articles/ni0809-806
- Thakur C, Chen B, Li L, et al. Loss of mdig expression enhances DNA and histone methylation and metastasis of aggressive breast cancer. Signal Transduct Target Ther. 2018;3(1):25.
- Yu M, Sun J, Thakur C, et al. Paradoxical roles of mineral dust induced gene on cell proliferation and migration/invasion. PLoS One. 2014;9(2):e87998.
- Komiya K, Sueoka-Aragane N, Sato A, et al. Mina53, a novel c-Myc target gene, is frequently expressed in lung cancers and exerts oncogenic property in NIH/3T3 cells. J Cancer Res Clin Oncol. 2010;136(3):465–473.
- Aziz N, Hong YH, Jo MK, et al. Molecular signatures of JMJD10/MINA53 in gastric cancer. Cancers. 2020;12(5):1141. https://www.mdpi.com/2072-6694/12/5/1141/htm
- Lu Y, Chang Q, Zhang Y, et al. Lung cancer-associated JmjC domain protein mdig suppresses formation of tri-methyl lysine 9 of histone H3. Cell Cycle. 2009;8(13):2101–2109.
- Thakur C, Wolfarth M, Sun J, et al. Oncoprotein mdig contributes to silica-induced pulmonary fibrosis by altering balance between Th17 and treg T cells. Oncotarget. 2015;6(6):3722–3736.
- Kosaka T, Yatabe Y, Endoh H, et al. Mutations of the epidermal growth factor receptor gene in lung cancer: biological and clinical implications. Cancer Res. 2004;64(24):8919–8923.
- Brabender J, Danenberg KD, Metzger R, et al. Epidermal growth factor receptor and HER2-neu mRNA expression in non-small cell lung cancer is correlated with survival. Clin Cancer Res. 2001;7(7):1850–1855.
- Ma D, Guo D, Li W, et al. Mdig, a lung cancer-associated gene, regulates cell cycle progression through p27(KIP1). Tumour Biol. 2015;36(9):6909–6917.
- Komiya K, Sueoka-Aragane N, Sato A, et al. Expression of Mina53, a novel c-Myc target gene, is a favorable prognostic marker in early stage lung cancer. Lung Cancer. 2010;69(2):232–238. http://dx.doi.org/10.1016/j.lungcan.2009.10.010
- Sun J, Yu M, Lu Y, et al. Carcinogenic metalloid arsenic induces expression of mdig oncogene through JNK and STAT3 activation. Cancer Lett. 2014;346(2):257–263.
- Yu H, Pardoll D, Jove R. STATs in cancer inflammation and immunity: a leading role for STAT3. Nat Rev Cancer. 2009;9(11):798–809.
- Johnson DE, O’Keefe RA, Grandis JR. Targeting the IL-6/JAK/STAT3 signalling axis in cancer. Nat Rev Clin Oncol. 2018;15(4):234–248.
- Huynh J, Chand A, Gough D, et al. Therapeutically exploiting STAT3 activity in cancer - using tissue repair as a road map. Nat Rev Cancer. 2019;19(2):82–96.
- Geng F, Jiang Z, Song X, et al. Mdig suppresses epithelial-mesenchymal transition and inhibits the invasion and metastasis of non‑small cell lung cancer via regulating GSK-3β/β-catenin signaling. Int J Oncol. 2017;51(6):1898–1908.
- Wang W, Lu Y, Stemmer PM, et al. The proteomic investigation reveals interaction of mdig protein with the machinery of DNA double-strand break repair. Oncotarget. 2015;6(29):28269–28281.
- Xuan F, Huang M, Zhao E, et al. MINA53 deficiency leads to glioblastoma cell apoptosis via inducing DNA replication stress and diminishing DNA damage response. Cell Death Dis. 2018;9(11):1062.
- Kuratomi K, Yano H, Tsuneoka M, et al. Immunohistochemical expression of Mina53 and Ki67 proteins in human primary gingival squamous cell carcinoma. Kurume Med J. 2006;53(3–4):71–78.
- M H, W D, Epigenetic WR. Dynamics of stem cells and cell lineage commitment: digging Waddington’s canal. Nat Rev Mol Cell Biol. 2009;10(8):526–537. https://pubmed.ncbi.nlm.nih.gov/19603040/
- Kappes F, Waldmann T, Mathew V, et al. The DEK oncoprotein is a Su(var) that is essential to heterochromatin integrity. Genes Dev. 2011;25(7):673–678.