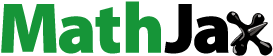
Abstract
In the present study, conditions for succinic acid (SA) production using xylose mother liquor (XML) as culture medium by Actinobacillus succinogenes GXAS137 were optimized. Firstly, single–factor experiments were performed to evaluate the basal culture medium for SA fermentation. Thereafter, the Plackett–Burman design was used to screen out three significant factors of XML, corn steep liquor powder (CSLP) and MgCO3 affecting the SA yields from the original nine factors. Subsequent use of steepest ascent experiment determined the center area of the three factors. Finally, the response surface methodology was used to further optimize the interactions between the three main factors and predict the maximum SA concentration through Box-Behnken design. The optimal conditions of SA fermentation were maximally documented in the XML (110 g/L), CSLP (18.86 g/L) and MgCO3 (69.12 g/L). The maximum production of SA was 58.06 ± 0.57 g/L after 60 h with a yield of 0.72 ± 0.06 g/g total sugar, approaching the predicted value (57.99 g/L). It was 1.63-fold of the SA production obtained with the basic medium (35.54 g/L). In addition, batch fermentations were carried out in a 1.3-L stirred bioreactor and SA reached 58.47 g/L. These results indicate that XML could be an alternative substrate for the economical production of SA by A. succinogenes GXAS137.
Introduction
Succinic acid (SA), a 4-C dicarboxylic acid also known as amber acid, is one of the most promising platform chemicals serving as a precursor for industrially important chemicals, agricultural and food additives and pharmaceutical ingredients [Citation1,Citation2]. The market demand for SA has seen an upward trend in recent years in view of its application in green solvents and biodegradable polymers, such as polybutylene succinate [Citation3]. The traditional production of SA is primarily via chemical synthesis using petroleum-derived maleic anhydride as a raw material, which requires costly catalysts, high temperature, high pressure and causes serious environmental problems, thereby rendering this process unsustainable [Citation4,Citation5]. Thus, SA production with a novel and green strategy through microbial fermentation from renewable and cheap materials has attracted great interest. Various microbial strains have been investigated for SA production: natural producers such as Actinobacillus succinogenes, Anaerobiospirillum succiniciproducens and Mannheimia succiniciproducens, and recombinant strains of Escherichia coli and Saccharomyces cerevisiae. Despite the competitive performance achieved by all these organisms, A. succinogenes has received substantial interest because of its ability to form SA at high productivities, yields and titres from a variety of carbohydrates [Citation1,Citation6]. However, the major issues with bio-based SA production are the higher costs ($2.86–3.00/kg) than conventional petrochemical routes ($2.40–2.60/kg) [Citation7]. The production cost could be reduced with utilization of cost-effective renewable feedstocks. Renewable biomass such as corn, rice, straw, corn fibre, etc. to replace pure sugars (glucose, sucrose, xylose etc.) as raw materials for SA production have been investigated [Citation8]. However, use of food crops as feedstock may raise societal concerns about sustainability, especially reducing the availability of food supply and increasing food prices [Citation9]. The lignocellulose-rich materials are inexpensive, abundant and renewable. However, many technical aspects are complex and expensive [Citation10]. Therefore, developing non-food raw materials is evidently important in view of a cost-effective process, food safety and sustainability.
Xylose mother liquor (XML) is a major byproduct generated during xylitol manufacturing. It is known as rather abundant waste, but it is also a resource enriched with multiple carbohydrates, such as xylose (35%–40%), L-arabinose (10%–15%), glucose (8%–10%) and galactose (8%–10%) [Citation11]. More than 50,000 metric tonnes of XML are produced per year in China [Citation12]. However, it is very challenging and not cost-effective to directly separate XML components owing to complex composition [Citation13]. Therefore, most XML is discarded directly except only some amount that finds application in low value-added products such as some toothpaste ingredients [Citation14]. Thereby, it causes serious environmental pollution and decreases the economic benefits of the xylitol industry [Citation15].
Recently, biotransformation has emerged as a more attractive means of producing high-value compounds from XML as renewable feedstock for microbial fermentation, such as 2,3-butanediol [Citation16], xylitol [Citation11], butanol [Citation15] and welan gum [Citation17]. However, the furfural and 5-hydroxymethyl furfural (HMF) of XML can inhibit microbial cell growth during the fermentation process [Citation18]. In SA production from XML by recombinant Escherichia coli strain, the conversion rate of xylose reached 63% and 52.09 g/L SA was obtained from 82.62 g/L XML [Citation19]. However, to the best of our knowledge, utilizing XML to produce SA by A. succinogenes strain is not reported yet.
SA fermentation is a complex, non-linear, unstructured process. It is difficult to determine the optimal fermentation conditions by applying single-factor experiments. Response surface methodology (RSM) is a useful tool to optimize the parameters of fermentation processes by applying mathematics and statistics [Citation20]. It can be used to recognize and optimize the significant factors, identify the optimum conditions and finally optimize the fermentation process [Citation21].
In this study, a method combining single-factor experiment, Plackett–Burman design (PBD), steepest ascent method and Box–Behnken design (BBD) was adopted to optimize SA production from XML by A. succinogenes GXAS137 (XML loading, inexpensive nitrogen sources and minerals supplementation). This work may be helpful for SA fermentation from low-cost feedstocks and reuse of xylitol industrial by-products.
Materials and methods
Chemicals and materials
The XML with a total sugar content of 732.9 g/L (418.3 g/L xylose, 149.6 g/L arabinose, 82.5 g/L glucose and 80.3 g/L D-mannose) was supplied by Futian Pharmaceutical Co., Ltd. (Dezhou, Shandong Province, China). For SA fermentation, the XML was directly used as a sugar source without any pretreatment. Yeast extract (YE) was purchased from OXOID (England). Corn steep liquor powder (CSLP), peanut meal (PM), soybean meal (SM) and cotton meal (CM) were purchased from Comwin Pharm-culture Co., Ltd. (Beijing, China). The total nitrogen concentration (w/w) in YE, CSLP, SM, PM, ammonium sulphate and urea was 10.9, 7.4, 8.1, 7.6, 22.7 and 46%, respectively.
Microorganism and growth conditions
A. succinogenes GXAS137 and cultural method were obtained from a previous study [Citation10].
Single-factor experiments
Single-factor experiments were conducted to investigate the influence of fermentation parameters on SA production. The fermentation for control was conducted in 100-mL sealed anaerobic bottles with 50 mL of the medium with a salt mixture, which contained the following: 2.0 g/L of NaH2PO4·H2O, 3.0 g/L of K2HPO4, 0.2 g/L of CaCl2·2H2O, 0.2 g/L of MgCl2·6H2O and 1.0 g/L of NaCl. To investigate the influence of the nutritional conditions in SA production, anaerobic bottle experiments were conducted with various XML concentrations (60–110 g/L), different nitrogen sources (YE, CSLP, SM, PM and urea) and different MgCO3 concentrations (30–80 g/L) sequentially at 37 °C with agitation rate of 100 rpm for 60 h.
PBD experiment
To identify the key factors that influenced SA production, the effective technique for medium optimization of PBD was selected. The 12 trials with two concentration levels were undertaken to evaluate the significance of nine medium components [Citation19]. The experimental design and results of PBD are listed in .
Table 1. Factor levels of PBD for screening of significant variables affecting succinic acid production and corresponding results.
Steepest ascent design
Steepest ascent experiments were adopted to determine a suitable direction, which was used as the centre point for optimization using the RSM according to the results of PBD [Citation22].
RSM design
A three-factor three-level design with 13 trials BBD was applied to optimize the factors, and a second-order polynomial equation was obtained from the BBD.
Fermentation in stirred bioreactors
Batch fermentation was carried out in a 1.3-L fermenter (Eppendorf BioFlo/CelliGen 115), containing 0.7 L of fermentation medium. The fermentation medium was the same as that in the anaerobic bottles. The cells were used to inoculate 1.3-L fermenter at an inoculum size of 5% (v/v) until a cell count of about 2–4 × 108 cell/mL was reached. The fermentation was performed at a CO2 flow rate of 0.3 L/min, temperature of 37 °C with agitation rate of 100 rpm for 60 h.
Analytical methods
The concentrations of organic acids and sugars were determined by high-performance liquid chromatography (HPLC) [Citation10]. Analysis of furfural, HMF and their alcohols was done using a UV detector (Shimadzu SPD-10A) at 280 nm [Citation16].
Statistical design
All experiments were replicated three times and the data are presented as mean values with standard deviation (± SD). RSM was conducted by Design Expert Software Version 8.0. Analysis of variance (ANOVA) was performed using IBM-SPSS software (version 19.0, Chicago, USA). The differences were considered statistically significant at a 95% confidence level (p < 0.05).
Results
Single-factor experiment
Key parameters for SA production were identified using the single-factor experiment method.
The SA production by A. succinogenes GXAS137 was examined using different concentrations of XML in the range of 60–110 g/L (). The SA production increased significantly (p < 0.05) from 28.59 to 42.54 g/L when the initial XML content was below 100 g/L. Contrarily, when the initial XML content of 100 g/L was increased to 110 g/L, the SA content and yield dropped significantly from 42.54 to 38.45 g/L and 58.04 to 47.69%. Therefore, the optimal XML concentration was 100 g/L, which corresponded to an initial total sugar concentration of 73.29 g/L. As shown in , SA fermentation was altered with different nitrogen sources. In general, SA content was higher with nitrogen sources addition than without, and organic nitrogen sources were favourable for SA production as compared to inorganic nitrogen sources. XML probably contains a certain amount of nitrogenous substances for A. succinogenes growth, as production of 5.64 g/L SA could take place without adding the extra nitrogen source. Urea appeared to be a less favourable nitrogen source for increasing SA production, yielding a final concentration of 10.65 g/L. Compared to other nitrogen sources, CSLP was a relatively good nitrogen source for SA production (44.20 g/L) by the A. succinogenes GXAS137. This finding indicated that CSLP can replace expensive YE as a nitrogen source for SA production. The maximal final SA concentration (44.65 g/L) and yield (60.92%) were achieved by adding 16 g/L of CSLP (). As shown in , the optimal MgCO3 addition amount was 60 g/L, with SA concentration and yield of 41.65 g/L and 64.08%, respectively. The other key parameters were 1.00 g/L of K2HPO4, 1.50 g/L of KH2PO4, 1.00 g/L of NaCl, 0.60 g/L of MnCl2 and 0.30 g/L of CaCl2.
PBD experiment
On the basis of single-factor experiment results, PBD was selected for analyzing the significance of nine variables. The maximum and minimum effects of each variable on the concentration of SA were determined by Student’s t-test.
The model F-value was 20.26, and values of ‘Prob>F = 0.0479’ less than 0.0500, indicated that the model terms are significant. In this case, X1 (total sugars of XML), X2 (CLSP) and X5 (MgCO3) were significant model terms (>95% confidence levels) for SA production. The remaining variables had confidence levels <95% and were considered insignificant. The effects of the nine variables ranked as follows: X1>X2>X5>X7>X6>X3>X9>X4>X8. A first-order model equation was derived representing SA production as a function of the independent variables, as follows:
(1)
(1)
From the data analysis, X1, X2, X5, X6, X9 positively affected SA production, whereas X3, X4, X7, X8 showed a negative effect on SA production. These insignificant factors were excluded in the next optimization experiment, but were used in all trials at their (−1) level for negative contribution and (+1) level for the positive contribution [Citation23].
Steepest ascent design (SAD)
The coefficients of X1, X2 and X5 had positive effects, indicating that the path of the SAD should increase the concentration respectively to enhance the SA concentration. The experimental design and responses of the steepest ascent path experiments in six runs were as follows (g/L): Run 1 (XML 95, CSLP 12, MgCO3 50) 35.61 ± 0.32, Run 2 (XML 100, CSLP 14, MgCO3 55) 41.46 ± 0.46, Run 3 (XML 105, CSLP 16, MgCO3 60) 44.66 ± 0.38, Run 4 (XML 110, CSLP 18, MgCO3 65) 55.36 ± 0.51, Run 5 (XML 115, CSLP 20, MgCO3 70) 50.15 ± 0.44, Run 6 (XML 120, CSLP 22, MgCO3 75) 40.24 ± 0.35.
The results show that the SA yield was maximum at Run 4. The highest production response was 55.36 g/L when the concentrations of XML (X1), CSLP (X2) and MgCO3 (X5) were 110.00, 18.00 and 65.00 g/L, respectively.
Optimization of the screening culture conditions by response surface methodology (RSM)
Based on the results of steepest ascent design, the point with 110.0 g/L of XML, 18.0 g/L of CSLP and 65.0 g/L of MgCO3 considered the central level of BBD of three variables in coded and real levels is shown in . shows the ANOVA for the BBD.
Table 2. The Box-Behnken design and the experimental responses of dependent variable Y (succinic acid concentration, g/L).
Table 3. Analysis of variance (ANOVA) and coefficient estimated for succinic acid.
The experimental results obtained from BBD were fitted to a second-order polynomial equation, as follows (in terms of coded factors):
(2)
(2)
The efficiency of the fit of the model was checked by the determination coefficient (R2). As shown in , the value of R2=0.999 indicates that only 0.1% of the total variations is not explained by the model. Values of Probability>F = 0.0002 indicated that the model terms were significant and only a 0.02% chance could occur due to noise. The interactions between the two varying factors of SA production are clearly interpretable. In this case, the linear coefficients (X1, X2, X3), cross-product coefficients (X1X2, X1X3, X2X3) and quadratic term coefficients (X22, X32) were significant model terms (p < 0.05). The F values confirmed that the order of factors influencing the SA production was as follows: X3 (MgCO3 content) > X2 (CSLP content) > X1 (XML content); the order of the interaction effects was as follows: X2X3 >X1X3 > X1X2.
The three dimensional (3 D) response surface curve of the three variables was depicted by statistically significant model to understand the interaction between two variables and determine the optimum concentration of each factor for the maximum SA production ().
Figure 2. Response surface curves of succinic acid production by A. succinogenes GXAS137 showing interaction between XML and CSLP (a), CSLP and MgCO3 (b), XML and MgCO3 (c). XML: xylose mother liquor; CSLP: corn steep liquor powder.
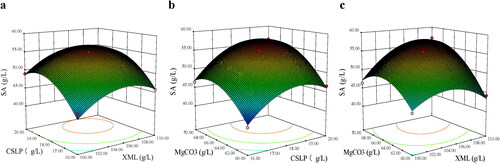
The steepness of the response surface indicated the degree of interaction influences of two experimental variables. Subsequently, the maximum SA production (57.99 g/L) was estimated from EquationEquation (2)(2)
(2) at the concentration of XML, CSLP and MgCO3 of 110.59, 18.86 and 69.12 g/L, respectively. Verification experiments were conducted to confirm the model adequacy in predicting the maximum SA yield using the optimum medium compositions. The good agreement between the predicted and the experimental result (58.06 ± 0.57 g/L) verifies the validity of the model and the existence of an optimal point.
Fermentation in 1.3-L stirred bioreactors
Batch fermentations in 1.3-L stirred bioreactors were carried out under the same conditions as in the anaerobic bottles, as follows: XML, CSLP and MgCO3 of 110.59, 18.86 and 69.12 g/L, respectively. Samples were collected from these reactors every 4 h for 60 h of fermentation.
The results in show that the fermentation process was completed within 60 h because the sugar was almost exhausted and SA production reached its peak within 60 h. Glucose was consumed faster than other sugars. The reason may be that glucose is the optimal carbon source for A. succinogenes (Guettler et al., 1999). Xylose, arabinose and galactose were simultaneously consumed, without showing any sign of diauxic growth. Acetic acid was the major by-product formed in the fermentation process, the final titre was about 10.71 g/L in 60 h. The final content of SA reached 58.47 g/L, which was 2.05-fold higher than the production obtained with the basic medium (28.59 g/L). The result indicated that the combined method of single–factor experiments, PBD and RSM is powerful for selection of optimized conditions for SA production from XML. The final content and productivity (0.97 g/L/h) of SA were better than in previous reports (52.09 g/L and 0.62 g/L/h) by Wang et al. using recombinant Escherichia coli strain SD121 [Citation19]. These results demonstrated that XML could be an alternative substrate for the economical production of SA by A. succinogenes GXAS137.
Figure 3. Time course of production of organic acids in batch fermentations with xylose mother liquor (XML). Cells were grown in a 1.3 L stirred bioreactor with a XML content of 110 g/L, corn steep liquor power (CSLP) of 18.86 g/L, and MgCO3 of 69.12 g/L. Symbols are arabinose (■), glucose (▲), mannose (▼), acetic acid (◆) xylose (△) and succinic acid (○).
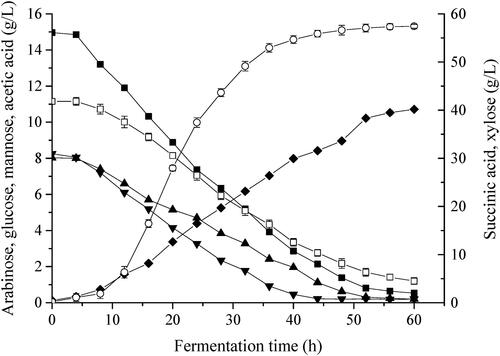
Discussion
XML contains two major inhibitors: furfural and 5-hydroxymethylfurfural (HMF), which are usually toxic to microorganisms and inhibit their growth and metabolism by affecting their DNA mutation, disrupting their membranes and decreasing their intracellular pH and other cellular targets [Citation13]. Therefore, XML is usually detoxified to remove/or reduce these inhibitors before it can be used as fermentation feedstock. Unfortunately, these processes bring new problems such as an increase in the cost, loss of sugars, and formation of undesired products [Citation12,Citation14]. Gunnarsson et al. (2014) showed that A. succinogenes was not inhibited by common inhibitors, including total soluble phenolic compounds, HMF and furfural when SA was produced from Jerusalem artichoke tuber hydrolysate [Citation24]. A. succinogenes also can tolerate up to 1 g/L HMF, 0.28 g/L furfural and 10 g/L acetic acid concentrations [Citation4]. Therefore, in this study, XML were successfully fermented without any need for detoxification. The results indicate that the toxic furan compounds of furfural and 5-hydroxymethyl furfural) in XML at 760 mg/L (of which furfural was 420 mg/L) were produced in hemicellulose pre-treatments, probably not resulting in decreased carbon metabolism and SA synthesis by A. succinogenes GXAS137. Therefore, XML might be a potential and competitive renewable feedstock for SA production.
A. succinogenes is a fastidious organism with high demand for nitrogen, and yeast extract is the best for both microbial growth and SA production. However, the high cost of yeast extract is hindering its greater application in large-scale industrial production of SA [Citation25]. To reduce the production costs, considerable effort has been devoted to reducing the cost by substituting YE with cheap nitrogen source such as spent brewer’s yeast hydrolysate, CSLP, peanut, soybean and cotton meals [Citation26]. Our findings showed that CSLP satisfied the nutritional requirement for A. succinogenes growth and fermentation utilizing XML as a carbon source. However, the amount of added CSLP is higher than that of duckweed as a substrate for SA production [Citation27]. The reason may be that duckweed is richer in nitrogen sources than XML.
MgCO3 is an important CO2 donor in A. succinogenes fermentation. It reacts with organic acids (SA, acetic acid, formic acid) in the fermentation broth and increases the dissolved concentrations of HCO3−, CO32− and CO2 [Citation28,Citation29]. It has also reported that Mg2+ play an important role in maintaining cellular metabolism in recent studies. Mg2+ is a cofactor for key enzyme, which is phosphoenolpyruvate carboxykinase (PEPCK) for SA production [Citation30]. However, excessive addition of MgCO3 would reduce the SA production and increase the cost of fermentation.
Optimization of the fermentation media has proved to be an effective way to enhance microbial fermentation products [Citation20,Citation21]. There are many factors affecting SA fermentation of A. succinogenes. Therefore, it is necessary to design an efficient experimental methodology to identify the significant variables and to optimize factors using a minimum number of experiments for SA production. PBD is an authentic method to evaluate the relative importance of various variables or medium components for cellular metabolite production, which greatly decreases the total number of experiments. However, there are some drawbacks associated with its efficiency. PBD considers only some main effects and ignores the interactions among the variables [Citation31]. RSM is a useful tool that can be used to evaluate and analyze the interactions between different factors [Citation32]. The combinations of PBD and CCD overcome the shortcomings of traditional methods and have been widely used for optimizing reaction conditions and processing parameters [Citation33]. In this study, a method combining single-factor experiments, PBD and RSM was developed to optimize SA production. First, single-factor experiments were applied to investigate the influence of fermentation parameters in SA production. Then, PBD identified the main factors affecting SA production, which were determined to be XML, CSLP and MgCO3 concentration. Then, the path of steepest ascent design was used to determine the central point of the response surface (XML 110.00 g/L; CSLP, 18.00 g/L; MgCO3 65.00 g/L). Next, BBD was used to model the fermentation process and to analyze the optimal conditions of the SA production. The SA production of verification experiments reached 58.06 ± 0.57 g/L, which was approximately 1.7 times higher than that of the original medium (34.15 ± 0.43 g/L). The results showed that a method combining single-factor experiments, PBD and RSM to optimize the fermentation medium had a significant effect in optimizing the production of SA by A. succinogenes GXAS137.
Conclusions
In the present study, a method combining single-factor experiment, PBD, steepest ascent method and BBD was developed to optimize SA production from XML by A. succinogenes GXAS137. The most appropriate combination was 110.59 g/L XML, 18.86 g/L CSLP and 69.12 g/L MgCO3 in the fermentation medium, the maximal SA production reaching 58.06 ± 0.57 g/L in anaerobic bottle. Scale-up to a 1.3-L fermenter resulted in 58.47 g/L production of SA. The results indicated that XML can be a very promising feedstock for SA production.
Disclosure statement
No potential conflict of interest was reported by the authors.
Data availability statement
The data that support the findings of this study are available from the corresponding author, [HYZ], upon reasonable request.
Additional information
Funding
References
- Ahn JH, Seo H, Park W, et al. Enhanced succinic acid production by mannheimia employing optimal malate dehydrogenase. Nat Commun. 2020;11(1):1–12.
- Yang Q, Wu M, Dai Z, et al. Comprehensive investigation of succinic acid production by Actinobacillus succinogenes: a promising native succinic acid producer. Biofuels Bioprod Bioref. 2020;14(5):950–964.
- Louasté B, Eloutassi N. Succinic acid production from whey and lactose by Actinobacillus succinogenes 130Z in batch fermentation. Biotechnol Rep (Amst). 2020;27:e00481.
- Ferone M, Raganati F, Ercole A, et al. Continuous succinic acid fermentation by Actinobacillus succinogenes in a packed-bed biofilm reactor. Biotechnol Biofuels. 2018;11(1):1–11.
- Xiao Y, Zhang Z, Wang Y, et al. Two-stage crystallization combining direct succinimide synthesis for the recovery of succinic acid from fermentation broth. Front Bioeng Biotech. 2020;7:471.
- Ferone M, Ercole A, Raganati F, et al. Efficient succinic acid production from high-sugar-content beverages by Actinobacillus succinogenes. Biotechnol Progr. 2019;35(5):e2863.
- Jokodola EO, Narisetty V, Castro E, et al. Process optimisation for production and recovery of succinic acid using xylose-rich hydrolysates by Actinobacillus succinogenes. Bioresour Technol. 2022;344(Pt B):126224.
- Chen J, Yang S, Alam MA, et al. Novel biorefining method for succinic acid processed from sugarcane bagasse. Bioresour Technol. 2021;324:124615.
- Lo E, Brabo-Catala L, Dogaris I, et al. Biochemical conversion of sweet sorghum bagasse to succinic acid. J Biosci Bioeng. 2020;129(1):104–109.
- Shen NK, Zhang HY, Qin Y, et al. Efficient production of succinic acid from duckweed (landoltia punctata) hydrolysate by Actinobacillus succinogenes GXAS137. Bioresour Technol. 2018;250:35–42.
- Jin LQ, Yang B, Xu W, et al. Immobilization of recombinant Escherichia coli whole cells harboring xylose reductase and glucose dehydrogenase for xylitol production from xylose mother liquor. Bioresour Technol. 2019;285:121344.
- Sha F, Zheng Y, Chen J, et al. D-Tagatose manufacture through bio-oxidation of galactitol derived from waste xylose mother liquor. Green Chem. 2018;20(10):2382–2391.
- Zhang L, Chen Z, Wang J, et al. Stepwise metabolic engineering of Candida tropicalis for efficient xylitol production from xylose mother liquor. Microb Cell Fact. 2021;20(1):1–12.
- Cheng HR, Wang HG, Lv JY, et al. A novel method to prepare L-Arabinose from xylose mother liquor by yeast-mediated biopurification. Microb Cell Fact. 2011;10(1):1–11.
- Li Z, Xiao H, Jiang W, et al. Improvement of solvent production from xylose mother liquor by engineering the xylose metabolic pathway in Clostridium acetobutylicum EA 2018. Appl Biochem Biotechnol. 2013;171(3):555–568.
- Wang XX, Hu HY, Liu DH, et al. The implementation of high fermentative 2, 3-butanediol production from xylose by simultaneous additions of yeast extract, Na2EDTA, and acetic acid. N Biotechnol. 2016;33(1):16–22.
- Liu X, Lin L, Xu X, et al. Two-step economical welan gum production by sphingomonas sp. HT-1 from sugar industrial by-products. Carbohydr Polym. 2018;181:412–418.
- Dessie W, Xin F, Zhang W, et al. Inhibitory effects of lignocellulose pretreatment degradation products (hydroxymethylfurfural and furfural) on succinic acid producing Actinobacillus succinogenes. Biochem Eng J. 2019;150:107263.
- Wang H, Pan J, Wang J, et al. Succinic acid production from xylose mother liquor by recombinant Escherichia coli strain. Biotechnol Biotechnol Equip. 2014;28(6):1042–1049.
- Yun TY, Feng RJ, Zhou DB, et al. Optimization of fermentation conditions through response surface methodology for enhanced antibacterial metabolite production by streptomyces sp. 1-14 from cassava rhizosphere. PLoS One. 2018;13(11):e0206497.
- Yan L, Zhang Z, Zhang Y, et al. Improvement of tacrolimus production in streptomyces tsukubaensis by mutagenesis and optimization of fermentation medium using plackett–burman design combined with response surface methodology. Biotechnol Lett. 2021;43(9):1765–1778.
- Zhu LW, Wang CC, Liu RS, et al. Actinobacillus succinogenes ATCC 55618 fermentation medium optimization for the production of succinic acid by response surface methodology. Biomed Res Int. 2021;626137:1–9.
- Lu DM, Jiang LY, Chen LA, et al. Optimization of fermentation conditions of the engineered Corynebacterium glutamicum to enhance L-ornithine production by response surface methodology. J Biotechnol Biomater. 2011;1(7):1–7.
- Gunnarsson IB, Karakashev D, Angelidaki I. Succinic acid production by fermentation of Jerusalem artichoke tuber hydrolysate with Actinobacillus succinogenes 130Z. Ind Crop Prod. 2014;62:125–129.
- Shen NK, Qin Y, Wang Q, et al. Production of succinic acid from sugarcane molasses supplemented with a mixture of corn steep liquor powder and peanut meal as nitrogen sources by Actinobacillus succinogenes. Lett Appl Microbiol. 2015;60(6):544–551.
- Bukhar NA, Loh SK, Nasrin AB, et al. Compatibility of utilising nitrogen-rich oil palm trunk sap for succinic acid fermentation by Actinobacillus succinogenes 130Z. Bioresour Technol. 2019;293:122085.
- Shen NK, Wang QY, Zhu J, et al. Succinic acid production from duckweed (landoltia punctata) hydrolysate by batch fermentation of Actinobacillus succinogenes GXAS137. Bioresour Technol. 2016;211:307–312.
- Koendjbiharie JG, Post WB, Palmer MM, et al. Effects of CO2 limitation on the metabolism of pseudoclostridium thermosuccinogenes. BMC Microbiol. 2020;20(1):13.
- Guettler MV, Rumler D, Jain MK. Actinobacillus succinogenes sp. nov., a novel succinic acid producing strain from the bovine rumen. Int J Syst Evol Micr. 1999;49(1):207–216.
- Feng J, Li T, Zhang X, et al. Efficient production of polymalic acid from xylose mother liquor, an environmental waste from the xylitol industry, by a T-DNA-based mutant of aureobasidium pullulans. Appl Microbiol Biotechnol. 2019;103(16):6519–6527.
- Singh V, Haque S, Niwas R, et al. Strategies for fermentation medium optimization: an in-depth review. Front Microbiol. 2016;7:2087.
- Behera SK, Meena H, Chakraborty S, et al. Application of response surface methodology (RSM) for optimization of leaching parameters for ash reduction from low-grade coal. Int J Min Sci Technol. 2018;28(4):621–629.
- Raina N, Slathia PS, Sharma P. Response surface methodology (RSM) for optimization of thermochemical pretreatment method and enzymatic hydrolysis of deodar sawdust (DS) for bioethanol production using separate hydrolysis and co-fermentation (SHCF). Biomass Convers Bior. 2020:1–21. https://doi.org/10.1007/s13399-020-00970-0.