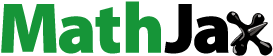
Abstract
Streptomyces spp. produce a range of secondary metabolites that can inhibit phytopathogen growth. This study aimed to optimise the environmental and nutritional parameters for improving the production of antifungal components by Streptomyces sp. HU2014 through statistical approaches. Spectrophotometry and bioassay revealed that two fractions, F6 and F8, from the cell-free filtrates of HU2014 culture broth were rich in antifungal compounds. Subsequently, single-factor experiments were conducted using glucose-peptone-yeast extract medium as basal medium, and the effects of different parameters on the production of bioactive metabolites by Streptomyces sp. HU2014 were evaluated and optimised using shake-flask experiments as follows: incubation time, 10 days; temperature, 25 °C; agitation speed, 150 rpm; inoculum size, 5% (v/v); initial pH, 7.0; and medium composition, dextrin, yeast extract and KNO3. The central composite face-centred design from response surface methodology predicted 39.6 g/L dextrin, 8.5 g/L yeast extract and 2.5 g/L KNO3 as the optimum medium composition. The validation experiment confirmed that a 50-fold dilution of the optimised filtered fermentation broth of the strain culture showed an increase of 14.9% in the inhibition of Rhizoctonia solani YL-3 when compared with the basal medium. A good correlation between measured and predicted values of the model validated both the responses. Thus, the present study used statistical optimisation to improve the bioactive metabolites productivity in Streptomyces sp. HU2014.
Introduction
Biological control agents (BCAs) are a group of micro-organisms that produce a wide variety of bioactive substances that have the potential to inhibit plant pathogens [Citation1]. BCAs have the advantages of safety, environment-friendliness and sustainable development. Some widely used commercial BCAs, such as Bacillus spp., Pseudomonas spp., and Streptomyces spp., have been applied to control phytopathogenic fungal diseases [Citation2–5]. Currently, the most widely used method to control phytopathogenic fungal diseases is the routine application of chemical pesticides and fertilisers; however, these chemicals have been proven to be harmful for agro-ecosystems. Thus, BCAs are increasingly becoming a preferred alternative to traditional control measures.
Actinomycetes, as an important micro-organism, can produce different kinds of bioactive secondary metabolites, which have significant application potential [Citation6–9]. Many reports have shown that Streptomyces, a genus belonging to Actinomycetes, could suppress many phytopathogens, including Magnaporthe oryzae, Fusarium spp., Rhizoctonia spp., Gaumannomyces graminis, and Pythium spp., both in vitro and in vivo [Citation8,Citation10–16]. The yield of bioactive metabolites from Streptomyces spp. is crucial for related research and application. Ahsan et al. [Citation17] optimised the fermentation conditions of antibacterial metabolites produced by Streptomyces TA1123 by measuring the antibiotic activity against Rhizoctonia solani AG-3. The activity of the Actinomycetes YJ1 strain was assessed though detecting the mycelial growth inhibition rate against Sclerotinia sclerotiorum [Citation6]. The maximum yield of crude pigmented secondary metabolites from Streptomyces hygroscopicus AVS7 was determined on the basis of its antimicrobial activity [Citation18].
It is also necessary to determine the various factors that regulate the production of secondary metabolites with antifungal properties. Strain fermentation, also known as shake-flask fermentation, is significantly influenced by medium components and culture conditions [Citation19–21]. Factorial design and response surface methodology (RSM) are powerful statistical tools, which can overcome the shortcomings of single-factor and orthogonal experimental design methods. While factorial design explores the role of each factor in the production of metabolites, RSM comprising mathematical and statistical approaches is used to assemble empirical models based on selected factors [Citation22].
A Streptomyces species, named Streptomyces sp. HU2014, was isolated and identified to exhibit strong antifungal activities against Rhizoctonia solani, and its complete genome sequence has been deposited in GenBank under the accession number CP097123 [Citation23]. In the present study, the fermentation conditions, carbon and nitrogen sources, and inorganic salts were optimised to achieve high metabolites production in HU2014 by using single-factor experiments, and the optimal medium composition and culture conditions were determined and assessed using RSM. The study aimed to improve the contents of active components and enhance the yield of bioactive metabolites from HU2014 by screening the culture conditions and medium composition.
Materials and methods
Organism
Rhizoctonia solani YL-3 and Streptomyces sp. HU2014 (maintained at the China Centre for Type Culture Collection; Preservation No. M2015207) were provided by Henan Institute of Science and Technology, China, and stored on potato dextrose agar (PDA) at 4 °C until further use.
Basal culture media
The PDA contained the following (g/L): potato, 200; dextrose, 12; and agar, 12; pH 7.0–7.2. Potato dextrose (PD) medium comprised the following (g/L): potato, 200 and dextrose, 12; pH 7.0–7.2. Glucose peptone yeast-extract medium (GPY) contained the following (g/L): glucose, 20; soybean peptone, 5; and yeast extract, 5; pH 7.0–7.2. Gause’s No. 1 medium consisted of the following (g/L): soluble starch, 20; NaCl, 0.5; KNO3, 1; K2HPO4⋅3H2O, 0.5; MgSO4⋅7H2O, 0.5; and FeSO4⋅7H2O, 0.01; pH 7.4–7.6. Czapek’s medium contained the following (g/L): NaNO3, 3; K2HPO4⋅3H2O, 1; KCl, 0.5; FeSO4⋅7H2O, 0.01; sucrose, 30; and MgSO4⋅7H2O, 0.5; pH 7.0–7.2.
Screening of culture media
To increase the production of active metabolites in HU2014, it is essential to optimise the culture conditions. The 11 basal culture media, PD, GPY, Gause’s No. 1, Czapek’s, and ISP (International Streptomyces project) 1, 2, 3, 4, 5, 6 and 7 [Citation24], were chosen. The mother solution of the heat sensitive components was sterilised with a bacteriological filter before use. HU2014 was cultured in PDA medium at 25 °C for 12 days. The spores were scraped off the surface of a HU2014 colony using an inoculation needle and suspended in sterile water at a density of 1.5 × 105-spores/mL as determined using a haemocytometer. The suspension was inoculated into 100-mL aliquots of the sterilised medium contained in 250-mL conical flasks and incubated at 150 rpm for 10 days at 25 °C. Subsequently, the broth was centrifuged using a high speed refrigerated centrifuge (Beckman Avanti J-25, California, USA) at 12,000 rpm for 10 min to separate the mycelia and supernatant. Then, the supernatant was filtered through a 0.22-µm candle filter. The resulting strain pellets were lyophilised (Christ ALPHA 1-4 LSC, Osterode, Germany) [Citation25] and then individually weighted (Sartorius BSA223, Shanghai, China). The cell-free filtrate of the HU2014 culture broth was mixed with melted PDA medium at a ratio of 1:9 (v/v) and poured into a Petri dish. The control was PDA medium without the cell-free filtrate. Then, R. solani YL-3 discs were placed at the centre of the petri dish and incubated at 25 °C for 3 days. Subsequently, the fungal colony diameter was measured using the growth rate method, and the mycelial growth inhibition rate was calculated as follows: Mycelial growth inhibition rate = (1 − Colony diameter of treatment/Colony diameter of control) × 100% [Citation26,Citation27]. The experiment was performed in triplicate. The basal medium was predominantly selected based on the antifungal activity of the fermentation metabolites from Streptomyces spp. [Citation6,Citation28].
Spectrophotometric analysis of cell-free filtrates collected at different growth periods
HU2014 spores were inoculated into GPY medium and incubated as described earlier, and the fermentation broth was collected at 2-day intervals from days 2 to 20. Subsequently, the broth was centrifuged to separate the mycelia and supernatant. Then, the supernatant was filtered through a 0.22-µm candle filter. The collected supernatants were diluted 10-fold with sterile distilled water, and their absorbance values were determined at 200-500 nm using an ultraviolet-visible spectrophotometer (Varioskan® Flash, Thermo Scientific, Massachusetts, USA), multiplied by the dilution factor, and denoted as AbsHU2014.
Spectrophotometric analysis and bioassay of four fractions
In the previous studies, four fractions (hereafter denoted as F2, F4, F6 and F8) were obtained from the cell-free filtrates of HU2014 culture broth [Citation23]. They were diluted with 70% methanol to a concentration of 0.1 mg/mL and their absorbances were determined as described above. The control was 70% methanol.
To trace the distribution of antifungal components in the fractions, antifungal activity assay against R. solani YL-3 was performed. Briefly, each fraction was dissolved in 2% dimethylsulphoxide to a final concentration of 0.5 mg/mL, mixed with melted PDA medium at a ratio of 1:9 (v/v) and poured into a Petri dish. The control was PDA medium supplemented with an equal volume of 2% dimethylsulphoxide. Then, the bioassay was conducted as indicated above.
Single-factor experiments for optimisation of fermentation conditions
The fermentation conditions were optimised using the one-factor at a time (OFT) approach. Based on the above experiment of screening of culture media, HU2014 was cultured in GPY medium under the following conditions: fermentation time (at 3-day intervals over 28 days), temperature (10, 20, 25, 30, 35 and 40 °C), agitation rate (60, 90, 120, 150 and 180 rpm), inoculum size (0.5%, 1%, 5%, 10% and 15%), and initial pH values (5.0, 6.0, 7.0, 8.0, 9.0 and 10.0). The bioassay and biomass measurements were conducted as described above.
Single-factor experiments for optimisation of medium components
Medium components play a crucial role in microbial fermentation. To screen the best medium for achieving higher bioactive metabolites production in HU2014, various carbon sources [including glucose (GLU), mannose (MAN), arabinose (ARA), galactose (GAL), starch (STA), maltose (MAL), glycerol (GLY) and dextrin (DEX)], nitrogen sources [including potato extract (PE), beef extract (BE), yeast extract (YE), soybean peptone (SP), malt extract (ME) and oat extract (OE)] and inorganic salt content (KH2PO4, MgSO4·7H2O, NaCl, CaCl2, FeSO4, and KNO3) were examined using the OFT approach [Citation29]. First, by employing GLU as the sole carbon source and a 10-g/L nitrogen source (PE, BE, YE, SP, ME and OE, independently), the optimal nitrogen source was selected based on the R. solani YL-3 mycelial growth inhibition rate and the guidance of strain biomass. Subsequently, the effect of optimal nitrogen source concentration (1.0, 2.5, 5.0, 10.0, 15.0, 20.0, 25.0 and 30.0 g/L) on mycelial growth inhibition rate was studied. Similarly, using SP and YE as the nitrogen sources and a 20-g/L carbon source (GLU, MAN, ARA, GAL, STA, MAL, GLY and DEX, independently), the strain biomass and R. solani YL-3 mycelial growth inhibition rate were evaluated on day 3. Thereby, the optimal carbon source was selected. Then, the effect of an optimal carbon source concentration (15.0, 20.0, 25.0, 30.0, 35.0, 40.0 and 45.0 g/L) on the mycelial growth inhibition rate was assayed. Finally, by adding 1 g/L inorganic salt to the GPY medium, the optimal inorganic salt was selected based on the R. solani YL-3 mycelial growth inhibition rate and the guidance of strain biomass. Subsequently, the effect of inorganic salt concentration (0.5, 1.0, 1.5, 2.0, 2.5 and 3.0 g/L KNO3) on the mycelial growth inhibition rate was evaluated. As no significant differences in R. solani YL-3 mycelial growth inhibition rates were observed among the 10-fold diluted cell-free filtrates of HU2014 culture broth incubated with the above-mentioned combination of medium components, a 50-fold diluted cell-free filtrate was employed in this experiment.
Optimisation of the main factors by Placket-Burman (PB) design
The PB design can quickly and effectively screen out the most important factors with a minimum number of tests. A total of eight parameters (number of tests, N = 12), DEX, YE, KNO3, pH, inoculum size, temperature, agitation speed and incubation time, were screened at two levels, maximum (+) and minimum (–) (). The experimental design and variable levels are shown in . The flask culture experiments were performed in accordance with the PB design, and the R. solani YL-3 mycelial growth inhibition rates were calculated, as described above.
Table 1. Experimental variables at two levels used for the antifungal effect of the cell-free filtrates from HU2014 culture using PB design.
Table 2. PB design for the optimisation of parameters influencing antifungal activity of the cell-free filtrates from HU2014 culture.
Optimisation of the selected factors using face-centred central composite design
Combined with the selected main factors and factor levels (), a total of 20 experiments were conducted using the RSM-based face-centred central composite design (FCCD) matrix (). The flask culture experiments were performed in accordance with the PB design, and the R. solani YL-3 mycelial growth inhibition rates were calculated, as described above. Each experimental point was tested according to the principle of randomness, and the data of inhibition rate were obtained and statistically analysed. Each of the three input factors was varied at three levels, as shown in . The partial regression coefficient was determined to obtain the regression equation and 3D diagram of the response surface. Based on the optimisation results, a validation experiment was conducted using the optimised medium and GPY medium.
Table 3. Input factors with their ranges and levels.
Table 4. RSM-based FCCD experimental design matrix for three factors.
Statistical analysis
In the bioassay of four fractions and optimised culture medium validation experiments, statistically significant differences (p < 0.05) were evaluated by analysis of variance (ANOVA), followed by Duncan’s test using SPSS version 16.0 (SPSS Inc. Chicago, IL, USA). Minitab software (version: 20.3.0, Minitab LLC, PA, USA) was used for the PB design experiments. Expert design (version:12.0.3.0 Stat-Ease, Inc., MN, USA) was used to generate the experimental designs and construct 3D surface plots and 2D contour plots to illustrate the relationship between response and effect of the investigated parameters and their experimental levels used in the present study.
Results
Spectrophotometric analysis of cell-free filtrates at different growth periods
The AbsHU2014 values of the cell-free filtrates of HU2014 culture broth were evaluated at 2-day intervals from day 2 to day 20. The absorbance increased from day 2 (IA1) to day 12 (IA6) and then followed a decreasing trend from day 14 (IA7) to day 20 (IA10) (). The peak value of AbsHU2014 in IA6 (on day 12) was 20.7. The characteristic ultraviolet-visible absorption peaks of the supernatants were at 291 nm, 305 nm, 320 nm, 333 nm and 352 nm. The curve trend of different fermentation times revealed that the contents of bioactive metabolites changed from pre-fermentation to post-fermentation. Consistently, metabolic shifts are related to the fermentation period [Citation30].
Spectrophotometric analysis and bioassay of four fractions
The spectrophotometric analysis () revealed that fractions F6 and F8 had the same strong characteristic absorption peaks at 319, 333 and 351 nm, whereas F2 and F4 had peaks at 291, 305 and 319 nm. The characteristic peaks of the active components in the four fractions were in agreement with those of the cell-free filtrates (). The bioassay revealed that the F6 and F8 fractions could inhibit the growth of R. solani YL-3 with the maximum inhibition rates of 100%, followed by F4 (60.4%) and F2 (34.6%) (, Supplemental Figure S1). Therefore, the fractions rich in the main active components were determined to be F6 and F8.
Figure 2. Scanning broad spectrum of four fractions extracted from the cell-free filtrates of HU2014 culture broth by spectrophotometry (A) and the antifungal activity of four fractions against R. solani YL-3 on day 3 (B). Values are means ± SD of three independent experiments. Means with the same letter for inhibition rate are not significantly different (p<0.05). F2, F4, F6 and F8 represent one of fractions, respectively.
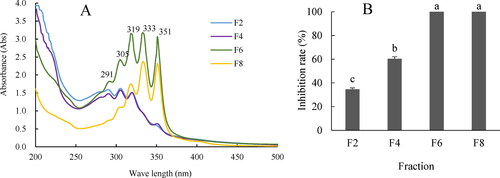
Screening of culture media and fermentation conditions
HU2014 was inoculated in 11 different media to screen the best culture medium for achieving higher production of antifungal metabolites. GPY medium presented the highest R. solani YL-3 mycelial growth inhibition rate (100%) and the biomass of the strain was approximately 5.6 g/L, followed by the ISP2 medium (at 96.4% and 5.4 g/L, respectively). Hence, GPY medium was used as the basal medium for further studies ().
Figure 3. Antifungal activities of cell-free filtrates of HU2014 culture broth against R. solani YL-3 and biomass growth of HU2014 in different conditions: Different basal media (A) and different culture conditions (B-F). Each treatment was repeated three times with three biological replicates, and the data show Means ± SD. G1 represents Gause’s No.1 medium. Cza represents Czapek’s medium.
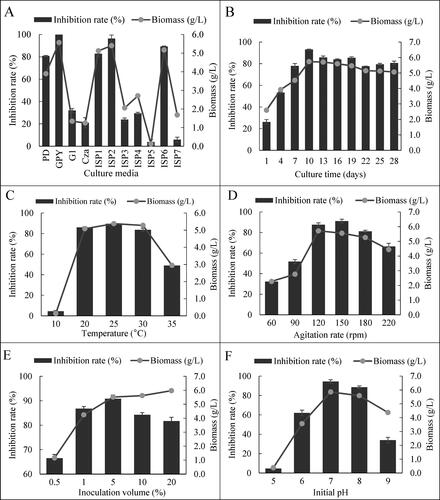
The biomass growth of HU2014 reached a maximum on day 10. This coincided with the highest R. solani YL-3 mycelial growth inhibition rate (). Temperature was another important factor that affected the metabolic activities of HU2014, and the R. solani YL-3 mycelial growth inhibition rate increased from 10 °C to 25 °C and then decreased, with the highest R. solani YL-3 inhibition rate (89.6%) and the maximum HU2014 biomass (5.4 g/L) being observed at 25 °C (). Similarly, the maximum R. solani YL-3 mycelial growth inhibition rate and corresponding HU2014 biomass were achieved at an agitation speed of 150 rpm, inoculum size of 5%, and pH of 7.0 (). Hence, based on the curve of the strain growth and AbsHU2014, cultivation for 10 days was taken as the optimal incubation period required for maximum biomass production.
Optimisation of medium components
As the antifungal effects of HU2014 significantly varied in different culture media, the types and concentrations of carbon sources, nitrogen sources and inorganic salts were presumed to have crucial influence on the R. solani YL-3 mycelial growth inhibition rate. When DEX was the sole carbon source in the medium, the growth of HU2014 reached a maximum biomass of 6.4 g/L and the highest R. solani YL-3 mycelial growth inhibition rate of 86.7%, followed by ARA (at 5.6 g/L and 83.0%, respectively) (). Therefore, DEX was selected as the optimal carbon source for HU2014 to produce antifungal substances. As shown in , the concentration of DEX was optimised, and 40.0 g/L DEX was the concentration that supported HU2014 to induce the highest R. solani YL-3 mycelial growth inhibition rate.
Figure 4. The antifungal activities of cell-free filtrates of HU2014 culture broth with different medium components against R. solani YL-3 and the biomass of the strain (ACE), and variation of the R. solani YL-3 (BDF) inhibition rate with different concentration of medium components.
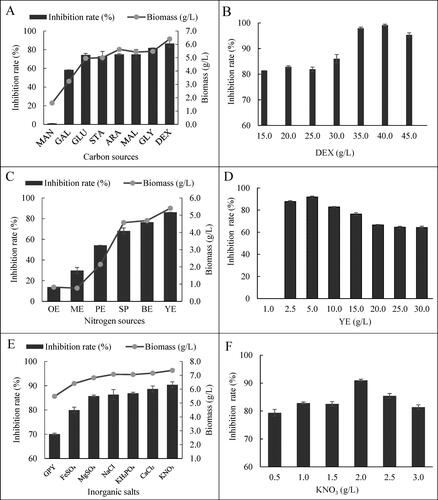
Similarly, screening of nitrogen sources revealed YE as the optimal nitrogen source, which supported the growth of HU2014 with a maximum biomass of 5.4 g/L and the highest R. solani YL-3 mycelial growth inhibition rate of 86.2% (). Furthermore, the optimal concentration of YE was 5.0 g/L, at which HU2014 produced the highest mycelial growth inhibition rate (). Likewise, screening of inorganic salts revealed KNO3 as the optimal inorganic salt, which supported the growth of HU2014 with a maximum biomass of 7.4 g/L and the highest R. solani YL-3 mycelial growth inhibition rate of 90.4% (). The inhibition rate was the highest at 2.0 g/L KNO3 ().
Optimisation of main factors by PB design
The influence of eight factors, namely DEX, YE, KNO3, pH, inoculum size, temperature, agitation speed and incubation time, on the antifungal activities of HU2014 against R. solani YL-3 was studied using PB design. The results of the standardised effects and ANOVA showed that DEX, YE and KNO3 were the most significant factors ( and ). The other factors that had less significant effects on the R. solani YL-3 mycelial growth inhibition rate were omitted in the subsequent optimisation experiments using FCCD. For model term values, differences were considered significant at a level of p < 0.05.
Figure 5. Pareto chart of the standardised effects for eight medium factors on the antifungal activities of the cell-free filtrates of HU2014 culture broth.
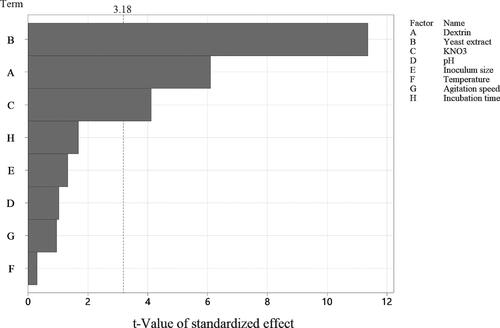
Table 5. ANOVA for the experimental result of PB design.
Optimisation of the selected factors using FCCD
For the optimisation of medium components, ANOVA for quadratic model was employed (). The regression equation can be given as follows:
where Y represents the inhibition rate, and A, B and C denote DEX, YE and KNO3, respectively. The significance of the model for the inhibition rate response was validated by p < 0.0001. The ANOVA and regression showed that the lack of fit was insignificant for this model.
Table 6. ANOVA for Quadratic RSM model details.
The effects of different factors on the antifungal activity of bioactive metabolites from HU2014 were analysed. The contour plot of the R. solani YL-3 mycelial growth inhibition rate in the presence of DEX and YE, with a significant interaction, denoted that the carbon and nitrogen sources had the highest effect on the mycelial growth inhibition rates (). KNO3 had a smaller effect on the R. solani YL-3 mycelial growth inhibition rate, when compared with DEX and YE, with an increase in concentration from 1.5 to 2.5 g/L (). Similarly, the combined effects of DEX with KNO3 and YE with KNO3 on the R. solani YL-3 mycelial growth inhibition rate were assessed using 3D plots (). Plotting the actual vs. predicted values for R. solani YL-3 mycelial growth inhibition rate () showed close adherence between these values along the line, thus validating the RSM model. The RSM model () suggested 97 optimal solutions, of which No. 20 was the best optimal solution. The maximum R. solani YL-3 mycelial growth inhibition rate was 97.7% in the presence of 39.6 g/L DEX, 8.5 g/L YE and 2.5 g/L KNO3 (). The validation experiments showed that the mycelial growth inhibition rate induced by the cell-free filtrates in the optimised medium against R. solani YL-3 increased by 14.9%, when compared with that in basal medium (GPY) ().
Discussion
As important members of Actinomycetes, Streptomyces spp. are well known for the production of bioactive metabolites [Citation31]. In the present study, the fractions F6 and F8 from the cell-free filtrates of HU2014 culture broth were found to exhibit strong antifungal activity against the phytopathogenic fungus R. solani YL-3, thereby showing good potential for application in agriculture. Thus, these metabolites are worthy of further research.
Some widely used commercial BCAs, such as Bacillus spp., Pseudomonas spp. and Streptomyces spp., have been applied in controlling phytopathogenic fungal diseases [Citation2–5]. Because the active metabolite production by micro-organisms is affected by fermentation conditions and medium composition [Citation32,Citation33], the addition of suitable nutrients can promote metabolite synthesis, cell growth, antibiotic fermentation units and antibiotic extraction processes [Citation34]. As microbial fermentation is a large-networked, nonuniform and complex process, optimisation of the culture medium is particularly important. RSM is an effective tool to improve the production of bioactive metabolites through optimisation of cultural characteristics and physicochemical conditions.
Many factors, such as incubation time, temperature, pH, carbon source, inorganic salt content and nitrogen source, can have a significant influence on the microbial metabolites production efficiency during fermentation [Citation35–38], and a Pareto chart can intuitively reflect the important factors of multiple vertical factors. Statistical optimisation of different parameters affecting the antifungal activity of the bioactive metabolites from HU2014 revealed that DEX as a carbon source, YE as a nitrogen source and KNO3 as an inorganic salt could significantly influence the productivity of antifungal metabolites. It must be noted that the nutritional demand of Streptomyces spp. plays a vital role in the synthesis of secondary metabolites [Citation39], and the analysis of optimal media components and cultural characteristics is correlated with the suitability of the number of carbon and nitrogen sources in the fermentation medium. Some Streptomyces spp. have been reported to produce high yield of secondary metabolites in the presence of DEX as the carbon source [Citation40–42]. Likewise, the nitrogen source in the culture medium has been found to be related to the yield of antibiotic metabolites in the course of fermentation. For instance, moisture, YE and CaCl2 have been reported to significantly influence the antibiotics production by Streptomyces sp. AS4 [Citation43]. Nevertheless, it is necessary to seek further cheaper alternative nitrogen sources for mass production of bioactive metabolites.
Inorganic salts play a role in the production of active metabolites during the process of microbial fermentation. It has been reported that the regulation of pigment biosynthesis pathway in Serratia sp. S2B is affected by inorganic micronutrients, particularly, sulphate ions [Citation44]. Furthermore, KNO3 has been found to exert a significant positive effect on the production of antimicrobial agents by Streptomyces sp. NEAE-1 [Citation45]. In the present study, addition of most of the tested inorganic salts to the basal medium (GPY) significantly increased the R. solani YL-3 mycelial growth inhibition rate of HU2014. In particular, KNO3 was needed to produce the highest mycelial growth inhibition rate and the maximum HU2014 biomass, which could be owing to its advantage of being an inorganic salt and a nitrogen source. Based on the PB design, DEX, YE and KNO3 were selected for further FCCD. The quadratic model with different concentrations of medium components was investigated by RSM, and the 3D response surface plots showed the interaction effects of individual factors. The medium components, especially the DEX and YE ratios, significantly affected the growth of HU2014 and its production of bioactive metabolites. However, although RSM has some advantages in many aspects, it still presents certain limitations [Citation46,Citation47], and other more powerful techniques are necessary to overcome these constraints.
Conclusions
In this study, two fractions, F6 and F8 from the cell-free filtrates of HU2014 culture broth exhibited strong antifungal activities against the phytopathogenic fungus R. solani YL-3, showing good agricultural application potential, which is worthy of further research. The optimised fermentation conditions and the media that enhanced the biomass and the antifungal effects of the antibiotic products from HU2014 against R. solani YL-3 were identified. To the best of our knowledge, this is the first report on HU2014 bioactive metabolites enrichment and fermentation.
Author contributions
LH and CL contributed to the conceptualisation, design and supervision of this study. HZ wrote the manuscript and conducted the main experiments. XW contributed to data analysis and graphical representation. TR contributed to the review of the manuscript. All authors have read and approved the final version of the paper.
Supplemental Material
Download PDF (686.4 KB)Data availability statement
The data supporting the findings of this study are available from the authors upon reasonable request.
Disclosure statement
No potential conflict of interest was reported by the author(s).
Additional information
Funding
References
- Wright MG, Bennett GM. Evolution of biological control agents following introduction to new environments. BioControl. 2018;63(1):105–116.
- Moharam MHA, Stephan D, Koch E. Evaluation of plant-derived preparations and microorganisms as seed treatments for control of covered kernel smut of Sorghum (Sporisorium sorghi). J Plant Dis Prot. 2018;125:159–166.
- Martinez-Diz MD, Diaz-Losada E, Andres-Sodupe M, et al. Field evaluation of biocontrol agents against black-foot and petri diseases of grapevine. Pest Manag Sci. 2021;77(2):697–708.
- Khan MR, Doohan FM. Comparison of the efficacy of chitosan with that of a fluorescent pseudomonad for the control of Fusarium head blight disease of cereals and associated mycotoxin contamination of grain. Biol Control. 2009;48(1):48–54.
- Lagogianni CS, DI T. Effective biopesticides and biostimulants to reduce aflatoxins in maize fields. Front Microbiol. 2019;10:1–8.
- Song Q, Huang Y, Yang H. Optimization of fermentation conditions for antibiotic production by actinomycetes YJ1 strain against Sclerotinia sclerotiorum. J Agric Sci. 2012;4:95.
- Hei YY, Zhang HL, Tan NN, et al. Antimicrobial activity and biosynthetic potential of cultivable actinomycetes associated with Lichen symbiosis from Qinghai-Tibet Plateau. Microbiol Res. 2021;244:126652.
- Coombs JT, Franco C. Isolation and identification of actinobacteria from surface-sterilized wheat roots. Appl Environ Microbiol. 2003;69(9):5603–5608.
- Qin S, Li J, Chen HH, et al. Isolation, diversity, and antimicrobial activity of rare actinobacteria from medicinal plants of tropical rain forests in Xishuangbanna. Appl Environ Microbiol. 2009;75(19):6176–6186.
- Viaene T, Langendries S, Beirinckx S, et al. Streptomyces as a plant’s best friend? FEMS Microbiol Ecol. 2016;92:1–10.
- Wan MG, Li GQ, Zhang JB, et al. Effect of volatile substances of Streptomyces platensis F-1 on control of plant fungal diseases. Biol Control. 2008;46(3):552–559.
- Wu ZM, Yang Y, Kt L. Antagonistic activity of a novel antifungalmycin N2 from Streptomyces sp. N2 and its biocontrol efficacy against Rhizoctonia solani. FEMS Microbiol Lett. 2019;366:1–8.
- Tian XL, Cao LX, Tan HM, et al. Study on the communities of endophytic fungi and endophytic actinomycetes from rice and their antipathogenic activities in vitro. World J Microbiol Biotechnol. 2004;20(3):303–309.
- Jung B, Park SY, Lee YW, et al. Biological efficacy of Streptomyces sp. strain BN1 against the cereal head blight pathogen Fusarium graminearum. Plant Pathol J. 2013;29(1):52–58.
- Wang CL, Wang ZF, Qiao X, et al. Antifungal activity of volatile organic compounds from Streptomyces alboflavus TD-1. FEMS Microbiol Lett. 2013;341(1):45–51.
- Coombs JT, Michelsen PP, Franco CM. Evaluation of endophytic actinobacteria as antagonists of Gaeumannomyces graminis var. tritici in wheat. Biol Control. 2004;29(3):359–366.
- Ahsan T, Chen JG, Wu YH, et al. Screening, identification, optimization of fermentation conditions, and extraction of secondary metabolites for the biocontrol of Rhizoctonia solani AG-3. Biotechnol Equip. 2017;31(1):91–98.
- Vellingiri MM, Jeyasundar P, Venkatesan BP, et al. Statistical optimization of parameters for enhanced bioactive metabolites produced by Streptomyces hygroscopicus AVS7. Arab J Sci Eng. 2021;46(6):5345–5360.
- Zhao SL, Ren FE, Liu JL, et al. Screening and identification of actinomycetes for maize leaf spot and optimization of fermentation conditions. Acta Microbiol Sin. 2012;52:1228–1236.
- Sun HQ, Zhou SN, Tan ZJ, et al. Optimization of fermentation conditions of an endophytic antagonistic actinomycete from tobacco. Chin J Appl Environ Biol. 2011;17:914–917.
- Kamoun F, Weekers F, Ayed RB, et al. Multiple linear regression models to simulate spore yields of Bacillus amyloliquefaciens BS13 through optimization of medium composition. Biotech Appl Biochem. 2022;69(6):2686–2697.
- Thompson DR. Response surface experimentation. J Food Process Preserv. 1982;6(3):155–188.
- Zhu HX, Hu L, Hu HY, et al. Identification of a novel Streptomyces sp. strain HU2014 showing growth promotion and biocontrol effect against Rhizoctonia spp. in wheat. Plant Dis. 2022:1–15. DOI: 10.1094/PDIS-06-22-1493-RE.
- Shirling ET, Gottlieb D. Methods for characterization of Streptomyces species. Int J Syst Bacteriol. 1966;16(3):313–340.
- Grossmann L, Ebert S, Hinrichs J, et al. Effect of precipitation, lyophilization, and organic solvent extraction on preparation of protein-rich powders from the microalgae Chlorella protothecoides. Algal Res. 2018;29:266–276.
- Zhang JW, Li SK, Wu WJ. The main chemical composition and in vitro antifungal activity of the essential oils of Ocimum basilicum Linn. Var. pilosum (Willd.) Benth. Molecules. 2009;14(1):273–278.
- Fan L, Luo Z, Li Y, et al. Synthesis and antifungal activity of imidazo[1,2-b]pyridazine derivatives against phytopathogenic fungi. Bioorg Med Chem Lett. 2020;30:127–139.
- Al-Dhabi NA, Esmail GA, Ghilan AKM, et al. Characterization and fermentation optimization of novel thermo stable alkaline protease from Streptomyces sp. Al-Dhabi-82 from the Saudi Arabian Environment for eco-friendly and industrial applications. J King Saud Univ Sci. 2020;32:1258–1264.
- Tortora GJ, Funke BR, Case CL. Microbiology: an introduction. 12th ed. New York (NY): Pearson; 2015. p. 150–172.
- Guan Y, Wang QX, Lv C, et al. Fermentation time-dependent pectinase activity is associated with metabolomics variation in Bacillus licheniformis DY2. Process Biochem. 2021;101:147–155.
- Latha S, Sivaranjani G, Dhanasekaran D. Response surface methodology: a non-conventional statistical tool to maximize the throughput of Streptomyces species biomass and their bioactive metabolites. Crit Rev Microbiol. 2017;43(5):567–582.
- Ratnam B, Rao MN, Rao M, et al. Optimization of fermentation conditions for the production of ethanol from sago starch using response surface methodology. World J Microbiol Biotechnol. 2003;19(5):523–526.
- Son HJ, Heo MS, Kim YG, et al. Optimization of fermentation conditions for the production of bacterial cellulose by a newly isolated Acetobacter sp. A9 in shaking cultures. Biotechnol Appl Biochem. 2001;33(1):1–5.
- Kiers JL, Laeken A, Rombouts FM, et al. In vitro digestibility of Bacillus fermented soya bean. Int J Food Microbiol. 2000;60(2-3):163–169.
- El-Naggar NEA, El-Bindary AA, Nour NS. Statistical optimization of process variables for antimicrobial metabolites production by Streptomyces anulatus NEAE-94 against some multidrug-resistant strains. Int J Pharmacol. 2013;9(6):322–334.
- Oskay M. Antifungal and antibacterial compounds from Streptomyces strains. Afr J Biotechnol. 2009;8:3007–3017.
- Ganesan G, Velayudhan S, Solomon R. Statistical optimization of medium constituents and conditions for improved antimicrobial compound production by marine Streptomyces sp. Arch Biol Sci (Beogr). 2017;69(4):723–731.
- Sabu S, Singh ISB, Joseph V. Optimisation of critical medium components and culture conditions for enhanced biomass and lipid production in the oleaginous diatom Navicula phyllepta: a statistical approach. Environ Sci Pollut Res. 2017;24(34):26763–26777.
- Romero-Rodríguez A, Maldonado-Carmona N, Ruiz-Villafán B, et al. Interplay between carbon, nitrogen and phosphate utilization in the control of secondary metabolite production in Streptomyces. Antonie Van Leeuwenhoek Int J Gen Mol Microbiol. 2018;111(5):761–781.
- Feng T, Zhao J, Chu J, et al. Statistical optimizing of medium for clavulanic acid production by Streptomyces clavuligerus using response surface methodology. Appl Biochem Biotechnol. 2021;193(12):3936–3948.
- Abdelwahed NAM, Gomaa EZ, Hassan AA. Statistical modelling and optimization of fermentation medium for lincomycin production by Streptomyces lincolnensis immobilized cells. Braz Arch Biol Techn. 2017;60:1–14.
- Ng IS, Ye CM, Zhang ZX, et al. Daptomycin antibiotic production processes in fed-batch fermentation by Streptomyces roseosporus NRRL11379 with precursor effect and medium optimization. Bioprocess Biosyst Eng. 2014;37(3):415–423.
- Farraj A, Varghese DA, Vagvolgyi R, et al. Antibiotics production in optimized culture condition using low cost substrates from Streptomyces sp. AS4 isolated from mangrove soil sediment. J King Saud Univ Sci. 2020;32:1528–1535.
- Rastegari B, Karbalaei-Heidari HR. Sulfate as a pivotal factor in regulation of Serratia sp. strain S2B pigment biosynthesis. Res Microbiol. 2016;167(8):638–646.
- El-Naggar NEA, El-Bindary AA, Nour NS. Production of antimicrobial agent inhibitory to some human pathogenic multidrug-resistant bacteria and Candida albicans by Streptomyces sp. NEAE-1. Int J Pharmacol. 2013;9:335–347.
- Selvakumar S, Ravikumar R. 2017. A novel approach for optimization to verify RSM model by using Multi-Objective genetic algorithm (MOGA). Proceedings of the International Conference on Materials Manufacturing and Modelling (ICMMM). Mar 09–11; Vellore, India.
- Chaudhary T, Yadav D, Chhabra D, et al. Low-cost media engineering for phosphate and IAA production by Kosakonia pseudosacchari TCPS-4 using multi-objective genetic algorithm (MOGA) statistical tool. 3 Biotech. 2021;11(4):1–11.