Abstract
Chinese yam (‘yam’) was grown at different carbon dioxide concentrations ([CO2]), namely, ambient and elevated (ambient + 200 μmol mol−1), under low- and high-temperature regimes in summer and autumn, separately. For comparison, rice was also grown under these conditions. Mean air temperatures in the low- and high-temperatures were respectively 24.1 and 29.1 °C in summer experiment and 20.2 and 24.9 °C in autumn experiment. In summer experiment, yam vine length, leaf area, leaf dry weight (DW), and total DW were significantly higher under elevated [CO2] than ambient [CO2] in both temperature regimes. Additionally, number of leaves, vine DW, and root DW were significantly higher under elevated [CO2] than under ambient [CO2] in the low-temperature regime. In autumn experiment, tuber DW was significantly higher under elevated [CO2] than under ambient [CO2] in the high-temperature regime. These results demonstrate that yam shows positive growth responses to elevated [CO2]. Analysis of variance revealed that significant effect of [CO2] × air temperature interaction on yam total DW was not detected. Elevated-to-ambient [CO2] ratios of all growth parameters in summer experiment were higher in yam than in rice. The results suggest that the contribution of elevated [CO2] is higher in yam than in rice under summer. Yam net photosynthetic rate was significantly higher under elevated [CO2] than under ambient [CO2] in both temperature regimes in summer. Elevated [CO2] significantly affected on the rate in yam but not in rice in both experiments. These findings indicate that photosynthesis responds more readily to elevated [CO2] in yam than in rice.
Classification:
1. Introduction
Yams belonging to the Dioscorea genus are C3 plant species that are cultivated in tropical and sub-tropical regions of the world (Coursey & Haynes, Citation1970). Yam is the third most important tropical root and tuber crop after cassava and sweet potato in west Africa, central America, the Pacific islands, and southeast Asia (Srivastava et al., Citation2012). Among the various yam species, Chinese yam (Dioscorea opposita Thunb.), known as ‘nagaimo’ in Japanese, is cultivated in Japan, China, Korea, and Taiwan etc., and is one of the most important root and tuber crops in northern Japan. To feed the growing world population that is expected to reach 9.7 billion by 2050 (United nation, Department of Economic & Social Affairs, Citation2015), yam will have an increasingly important role.
The global atmospheric carbon dioxide concentration ([CO2]) has increased from 300 μmol mol−1 to the present 398 μmol mol−1 (National Oceanic & Atmospheric Administration- Earth System Research Laboratory, Citation2014). According to recent report (Inter-governmental Panel on Climate Change [IPCC], Citation2013), these levels will continue to increase in the foreseeable future. Associated with the [CO2] increase, the air temperature is predicted to rise 0.3–1.7 °C by 2081–2100 and 2.6–4.8 °C under high [CO2] emission scenarios (IPCC, Citation2013) as a result of global warming. Changes in both [CO2] and temperature are expected to affect yam production via their effects on many metabolic processes that influence crop growth and yield, both in terms of quantity and quality.
Many studies have evaluated the effects of elevated [CO2] and high temperature, separately and in combination, at experimental levels in growth chambers, greenhouses, or field studies. Such studies have mainly focused on cereal crops, especially the main grain cereals such as maize (Holden & Brereton, Citation2003; Oseni & Masarirambi, Citation2011), rice (Oryza sativa L.) (Cheng et al., Citation2009; Roy et al., Citation2012; Shimono et al., Citation2009), wheat (Nonhebel, Citation1993; Valizadeh et al., Citation2014), and sorghum (Prasad et al., Citation2006; Wall et al., Citation2001), or grain legumes such as soybean (Kumagai & Sameshima, Citation2014; Tacarindua et al., Citation2013) and peanut (Newman et al., Citation2005). Fewer studies have been conducted on the potential effects of climate change on root and tuber crops such as potato (Chen & Setter, Citation2012; Craigon et al., Citation2002; Katny et al., Citation2005) and cassava (Fernandez et al., Citation2002; Gleadow et al., Citation2009). In the case of yam, a small number of statistical analyses on the relationship between climatic data and yam yield in Africa (Ike, Citation2012; Zakari et al., Citation2014) as well as simulation studies based on models such as CROPSYSTVB-Yam and EPIC-Yam (Marcos et al., Citation2011; Srivastava & Gaiser, Citation2010; Srivastava et al., Citation2012) have been conducted but no experiments related to the effects of elevated [CO2] have been performed in yam (Raymundo et al., Citation2014), including Chinese yam. Therefore, understanding the responses of Chinese yam to climate change factors such as increasing [CO2] and air temperature is important to determine effective strategies for cultivation in the future.
In studies on plant responses to elevated [CO2], the source–sink hypothesis is an important consideration. According to this hypothesis, a plant with a low sink capacity will down-regulate acclimation of photosynthesis after long-term exposure to elevated [CO2] (Ainsworth et al., Citation2004). Chinese yam is a tuber crop with a large sink organ – the tuber – which is initiated from the early vegetative stage. In contrast, rice is considered as a limited sink plant during the vegetative stage. In this study, Chinese yam and rice were grown under two [CO2] conditions (ambient and elevated) with different air temperature regimes in summer and autumn, separately. We aimed to determine the effect of elevated [CO2] at different air temperature regimes under different seasonal conditions on the growth and photosynthesis of Chinese yam. In addition, our purpose was to clarify the differences in growth and photosynthetic responses to elevated [CO2] between Chinese yam (a large sink capacity plant) and rice (a limited sink capacity plant).
2. Materials and Methods
2.1. Plant materials and seedling preparation
We used two different plant materials: Chinese yam line Enshikei 6, and rice cv. Hitomebore. Seedlings were prepared as follows: yam bulbils were sterilized in 0.5% (v/v) sodium hypochlorite solution for 5 min and then thoroughly washed with water before sowing in small plastic nursery pots filled with commercial soil (containing 320 mg L−1 nitrogen (N), 210 mg L−1 phosphorus (P2O5), and 300 mg L−1 potassium (K2O)). The bulbils were sown on 9 June 2015 for use in summer and autumn experiments. Rice seeds were soaked in 20% (w/v) NaCl solution to eliminate unfilled seeds and then sterilized in 1% (v/v) SPORTAK® solution (AGRO-NET; Nissan Chemicals Ltd., Saitama, Japan) for 10 min. The seeds were washed with water and then incubated at 30 °C to germinate. The germinated seeds were sown in a seedling tray on 22 June 2015 for summer experiment and on 8 August 2015 for autumn experiment. Chinese yam and rice seedlings were grown under natural temperature, light, and humidity conditions in a greenhouse at Hirosaki University (40°59′N, 140°47′E), Hirosaki, Japan.
2.2. Temperature-gradient chamber and treatments
The two experiments were conducted in temperature-gradient chambers at Tohoku Agricultural Research Center, NARO (39°74′N, 141°13′E) in Morioka, Japan. Summer experiment was carried out from 11 July to 3 September 2015, and autumn experiment was carried out from 23 August to 3 October 2015. Both experiments were performed in the same manner, as described below.
In the temperature-gradient chamber, [CO2] and temperature were controlled independently. Two temperature-gradient chambers were used under two [CO2] conditions: ambient and elevated (ambient [CO2] + 200 μmol mol−1). Each chamber was a naturally sunlit greenhouse (6 m wide, 30 m long, and 3 m high). Summer and autumn experiments included a low- and a high-temperature regime. Thus, we were able to test the [CO2] responsiveness of Chinese yam and rice at ambient and elevated [CO2] under two temperature conditions (low and high). The daytime [CO2] averaged over the treatment period was 403 ± 10 μmol mol−1 in the ambient [CO2] plot and 598 ± 10 μmol mol−1 in the elevated [CO2] plot for summer experiment and 400 ± 9 μmol mol−1 in the ambient [CO2] plot and 591 ± 26 μmol mol−1 in the elevated [CO2] plot for autumn experiment. The temperatures averaged over the growing season for the low- and high-temperature regimes were, respectively, 24.1 ± 2.2 °C and 29.1 ± 2.4 °C in summer experiment, and 20.2 ± 1.5 °C and 24.9 ± 1.5 °C in autumn experiment. Temperatures in the low- and high-temperature plots were 0.3 and 5.3 °C higher, respectively, than the outside temperature. Both air temperature and [CO2] were measured at 5-s intervals and averaged every 1 min, 30 min and 24 h by a datalogger (CR 1000; Campbell Sci. Inc., Logan, UT, USA).
At the start of the experiments, the average Chinese yam seedling height was 22 cm (with five fully expanded leaves) in summer experiment and 49 cm (with 13 expanded leaves) in autumn experiment. Uniform plants were transferred into plastic pipes (50.5 cm long, 10.5 cm inner diameter; one plant/pipe) filled with the same type of soil as that used at the seedling stage. The plants were watered one to three times per week as required. For the rice plants, 2-week-old seedlings at the four- to five-leaf stage were transplanted into Wagner pots (two plants/pot) filled with commercial soil (containing 1.7 g L−1 N, 4.4 g L−1 P2O5, and 1.8 g L−1 K2O). The four treatments were started soon after transplanting Chinese yam and rice seedlings. Rice was flooded during all of these experiments. Hereafter, the treatments are abbreviated as follows:
(1) | Ambient [CO2] and low-temperature treatment (AL); | ||||
(2) | elevated [CO2] and low-temperature treatment (EL); | ||||
(3) | ambient [CO2] and high-temperature treatment (AH); | ||||
(4) | elevated [CO2] and high-temperature treatment (EH). |
2.3. Plant growth measurement and sampling
Chinese yam and rice plants in summer experiment and autumn experiment were respectively sampled at 55 d and 42 d after the beginning of treatment. For Chinese yam, leaves on each plant were counted and then the vine length was measured. After carefully washing the soil from the roots with running water, we separately sampled leaves, vines, roots, and tubers of individual plants in each treatment. The area of Chinese yam leaves was immediately measured using an automatic leaf area meter (AAM-9; Hayashi Denko Co. Ltd., Tokyo, Japan). Finally, all Chinese yam samples were dried at 80 °C for 4 d to constant weight. Dry weight (DW) was measured using an electronic scale (GX 3000; A&D Co., Ltd., Tokyo, Japan). Rice plants were sampled at the same times and in the same manner as that described above for Chinese yam. After counting numbers of leaves and tillers and measuring plant height, leaf blade area was immediately measured. Leaf blades, shoots without leaf blades, and roots were dried and weighed as described above. For Chinese yam and rice, six individual plants per treatment were used to measure each parameter.
2.4. Photosynthesis measurements
Photosynthetic gas exchange measurements were conducted on fully expanded leaves at 2–3 d before sampling. For Chinese yam, the measurements were conducted on fully expanded leaves in the middle of the vine because old leaves in the basal part of the vine and young leaves near the tip were not suitable for measuring normal photosynthesis. In rice, 14th and ninth expanded leaves of rice plants were used for measuring photosynthesis in summer experiment and autumn experiment, respectively. The light-saturated photosynthetic rate was measured between 0700 and 1400 using a portable photosynthesis measurement system (Li-6400; Li-Cor, Lincoln, NE, USA). The [CO2] in the air entering the leaf chamber was adjusted to 400 and 600 μmol mol−1 in ambient and elevated [CO2] plots, respectively. The air temperature in the chamber matched that of the respective regime. The relative humidity of air entering the chamber was adjusted to approximately 60%. The photosynthetic photon flux density inside the chamber was set to 1500 μmol m−2 s−1 using an internal light source and the leaf temperature was set to 27 °C. The measurements were conducted in the center part of a single leaf of three or four individual plants per treatment for Chinese yam and two or three individual plants for rice.
2.5. Statistical analysis
To test the significance of differences related to [CO2] levels, temperature regimes, and their interaction, we applied two-way analysis of variance (ANOVA) to the following data: for Chinese yam, the number of leaves, vine length, leaf area, leaf DW, vine DW, tuber DW, root DW, shoot DW, total DW, and leaf photosynthesis; for rice, the number of leaves and tillers, shoot length, leaf blade area, leaf blade DW, shoot DW, root DW, total DW, and leaf photosynthesis. When ANOVA produced a significant result, Tukey–Kramer’s test for significant differences between means was performed. All statistical analyses were performed with SPSS statistical software (SPSS ver. 24.0; IBM Corp, New York, NY, USA).
3. Results
3.1. Growth responses of Chinese yam and rice to elevated [CO2]
ANOVA revealed significant effects of both [CO2] and air temperature in summer experiment and of only temperature in autumn experiment on number of leaves, vine length, and leaf area in Chinese yam (Table ). A [CO2] × air temperature interaction for leaf area was found in summer experiment. According to Tukey–Kramer’s test, vine length and leaf area in Chinese yam in summer experiment were significantly (p < 0.05) higher under elevated [CO2] than under ambient [CO2] with both temperature regimes (Table ). The number of leaves was also significantly higher in EL than in AL. There was a trend towards more leaves in EH than in AH in summer experiment. In autumn experiment, no significant difference was observed in yam growth between elevated and ambient [CO2] treatments in terms of number of leaves, vine length, and leaf area under either temperature regime.
Table 1. Effects of elevated CO2 concentration on the plant growth of Chinese yam and rice.
For rice, significant effects of both [CO2] and temperature on the number of leaves in summer experiment and on the number of leaves and tillers and leaf blade area in autumn experiment were found on the basis of the ANOVA results (Table ). Air temperature also affected shoot length in both experiments and the number of tillers in summer experiment. The number of leaves was significantly (p < 0.05) higher in EL than in AL in summer experiment (Table ). There were no significant differences between elevated and ambient [CO2] in shoot length, number of tillers, and leaf blade area in both temperature regimes in summer experiment. In autumn experiment, the number of leaves, the number of tillers, and leaf blade area were significantly (p < 0.05) higher in EH than in AH. There were no significant differences in the number of leaves, number of tillers, and leaf blade area between AL and EL in autumn experiment. Rice shoot length was not significantly different between ambient [CO2] and elevated [CO2] in both temperature regimes in autumn experiment.
To understand differences in the contribution of elevated [CO2] between Chinese yam and rice, we calculated the mean ratio of each growth parameter under elevated vs. ambient [CO2]. Ratios of the number of leaves under elevated vs. ambient [CO2] under low- and high-temperature regimes, respectively, were 1.29 and 1.54 in summer experiment and 1.38 and 1.06 in autumn experiment in Chinese yam whereas the corresponding values in rice were 1.04 and 1.01 in summer experiment and 1.00 and 1.02 in autumn experiment (Table ). The ratios for vine length under low- and high-temperature regimes were, respectively, 1.49 and 1.86 in summer experiment and 1.11 and 1.05 in autumn experiment in Chinese yam; the corresponding ratios for shoot length in rice were 1.01 and 1.01 in summer experiment and 1.00 and 1.03 in autumn experiment. Leaf area ratios in Chinese yam under low- and high-temperature regimes were, respectively, 1.59 and 1.71 in summer experiment and 1.16 and 0.88 in autumn experiment; in rice, they were 1.01 and 1.03 in summer experiment and 1.15 and 1.29 in autumn experiment (Table ). The ratios of the number of tillers in rice ranged from 1.05 to 1.18 in both experiments.
3.2. Effects of elevated [CO2] and temperature on dry matter accumulation
ANOVA revealed significant effects of both [CO2] and temperature in summer experiment and of temperature in autumn experiment on Chinese yam leaf DW, vine DW, and root DW, except for tuber DW, which was affected by only [CO2] in summer experiment and by both factors in autumn experiment (Table ). According to Tukey–Kramer’s test, leaf DW, vine DW, and root DW of Chinese yam in summer experiment were significantly (p < 0.05) higher in EL than in AL (Table ). Leaf DW was also higher in EH than in AH. Chinese yam vine DW, root DW, and tuber DW tended to be higher in EH than in AH in summer experiment. In autumn experiment, Chinese yam tuber DW was significantly (p < 0.05) higher in EH than in AH, but there was no difference in tuber DW between AL and EL. There were no significant differences in Chinese yam leaf DW, vine DW, and root DW between ambient [CO2] and elevated [CO2] under low- and high-temperatures. The results of the ANOVA demonstrated the existence of significant effects of [CO2] and temperature on total plant DW of Chinese yam (p < 0.001 for all combinations, except for p < 0.01 for [CO2] in autumn experiment) in both experiments. No interaction was uncovered between [CO2] and temperature on Chinese yam total DW (Figure ). Total DW was significantly (p < 0.05) higher in elevated [CO2] than in ambient [CO2] under both temperature regimes in summer experiment and not significantly higher in autumn experiment (Figure ). In general, most Chinese yam DW values were greater in summer experiment than in autumn experiment; the exception was tuber DW (Table and Figure ).
Table 2. Effects of elevated CO2 concentration on the dry weight of Chinese yam and rice.
Figure 1. Effects of elevated CO2 concentration on total plant dry weight in Chinese yam and rice.
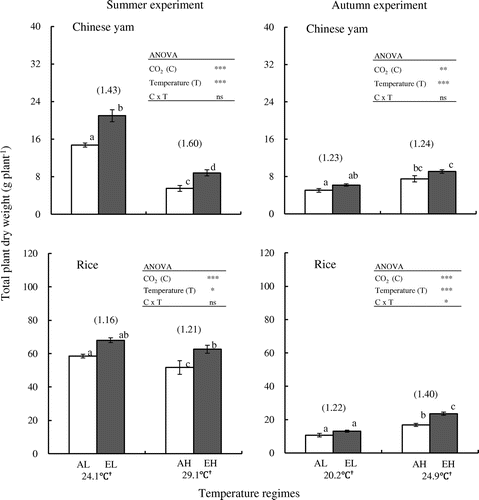
In the case of rice, leaf blade DW and shoot DW were significantly affected, according to the ANOVA, by both [CO2] and temperature in autumn experiment but not in summer experiment (Table ). Significant effects of [CO2] and temperature on total plant DW in rice (p < 0.001 for all combinations, except for p < 0.05 for temperature in summer experiment) were also found in both experiments. An interaction between [CO2] and temperature was uncovered in rice in autumn experiment (Figure ). According to Tukey–Kramer’s test, rice root DW and total DW in summer experiment were significantly (p < 0.05) higher in EH than in AH (Table and Figure ). Rice leaf blade DW and shoot DW did not differ between ambient and elevated [CO2] under either growth temperature regimes in summer experiment. Rice root DW and total DW did not differ significantly between AL and EL in summer experiment. In autumn experiment, rice leaf blade DW, shoot DW, and total DW were significantly (p < 0.05) higher in EH than in AH. None of the measured parameters differed significantly between AL and EL in autumn experiment (Table and Figure ).
For aboveground parts, the ratios of leaf DW in elevated vs. ambient [CO2] under low- and high-temperature regimes in Chinese yam were, respectively, 1.61 and 1.76 in summer experiment and 1.31 and 1.07 in autumn experiment (Table ). The corresponding data in rice were 1.07 and 1.07 in summer experiment and 1.16 and 1.36 in autumn experiment. Vine DW ratios in Chinese yam were 1.59 and 1.67 in summer experiment and 1.25 and 1.08 in autumn experiment under low- and high-temperature regimes, respectively, while the relative ratios of shoot DW in rice were 1.10 and 1.07 in summer experiment and 1.25 and 1.33 in autumn experiment. For underground parts, the ratios of root DW under low- and high-temperature regimes, respectively, were 1.38 and 1.83 in summer experiment and 1.30 and 1.06 in autumn experiment in Chinese yam; the corresponding ratios in rice were 1.03 and 1.31 in summer experiment and 1.17 and 1.23 in autumn experiment (Table ). In addition, tuber DW ratios in Chinese yam were 1.44 and 1.40 in summer experiment and 1.22 and 1.36 in autumn experiment. For total plant DW, the ratios under low- and high-temperature regimes in Chinese yam were respectively 1.43 and 1.60 in summer experiment and 1.23 and 1.24 in autumn experiment, while the corresponding values in rice were 1.16 and 1.21 in summer experiment and 1.22 and 1.40 in autumn experiment (Figure ).
3.3. Photosynthetic responses of Chinese yam and rice to elevated [CO2]
ANOVA uncovered significant effects of [CO2] and temperature on net leaf photosynthesis in Chinese yam (p < 0.001 in summer experiment and p < 0.05 in autumn experiment) but no significant effects on rice in either experiments (Figure ). However, there is no significant [CO2] × air temperature interaction on Chinese yam photosynthesis in both experiments. According to Tukey–Kramer’s test, the single-leaf net photosynthetic rate of Chinese yam was significantly higher under elevated [CO2] than ambient [CO2] under both low- and high-temperature regimes in summer experiment, but not in autumn experiment. Nevertheless, an increasing trend in the single-leaf net photosynthetic rate of Chinese yam was observed going from ambient to elevated [CO2] in autumn experiment. For rice, no significant differences in net photosynthetic rate were detected between ambient and elevated [CO2] in either experiments (Figure ).
Figure 2. Effects of elevated CO2 concentration on net photosynthetic rates of single leaf in Chinese yam and rice.
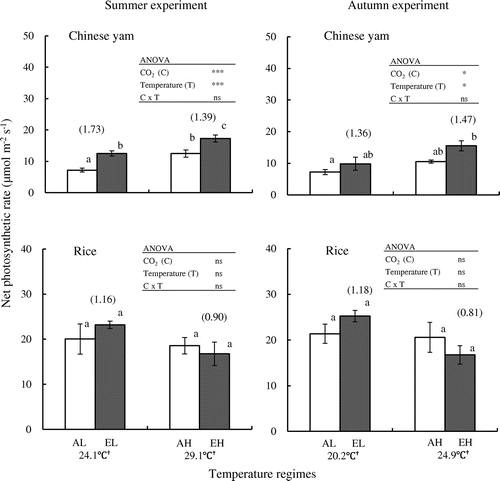
Ratios of photosynthesis in elevated vs. ambient [CO2] under low- and high-temperature regimes in Chinese yam were, respectively, 1.73 and 1.39 in summer experiment and 1.36 and 1.47 in autumn experiment; the corresponding values in rice were 1.16 and 0.90 in summer experiment and 1.18 and 0.81 in autumn experiment (Figure ).
4. Discussion
Many previous studies of the effects of elevated [CO2] on the growth of C3 plants have been published. In these studies, increased [CO2] has been reported to lead to increased dry matter production in many major food crops, including rice (Baker et al., Citation1992; Cheng et al., Citation2009; Roy et al., Citation2012; Shimono et al., Citation2008; Ziska et al., Citation1997), potato (Aien et al., Citation2014; Chen & Setter, Citation2012; Conn & Cochran, Citation2006; Miglietta et al., Citation1998), and cassava (Cruz et al., Citation2014; Imai et al., Citation1984; Rosenthal et al., Citation2012). To the best of our knowledge, however, no reports have appeared on yam response to elevated [CO2]. In the present study, the vine length, leaf area, leaf DW and total plant DW of Chinese yam were significantly higher in elevated [CO2] than in ambient [CO2] under both low- and high-temperature regimes in summer experiment (Tables and , Figure ). In addition, the number of leaves, vine DW, and root DW of Chinese yam were significantly higher in EL than in AL (Tables and ). In autumn experiment, tuber DW was significantly higher in EH than in AH and all other parameters of Chinese yam tended to exhibit higher values under elevated [CO2] than under ambient [CO2] (Tables and , Figure ). ANOVA results demonstrated the existence of significant effects of [CO2] and air temperature on total plant DW of Chinese yam in both experiments meanwhile there is no significant effect of [CO2] × air temperature interaction on Chinese yam total DW in both experiments (Figure ). These results demonstrate that Chinese yam shows positive growth responses to elevated [CO2]. However, no interaction between [CO2] and air temperature on the total DW in Chinese yam was identified in both experiments.
Not only [CO2] but also air temperature significantly affected total plant DW in rice in both summer and autumn experiments (Figure ). In both experiments, elevated [CO2] clearly increased rice total DW under the high-temperature regime. These results indicate that elevated [CO2] and air temperature affect total plant DW in rice as with Chinese yam. In summer experiment, ratios of all growth parameters related to size and weight (Tables and , Figure ) in elevated vs. ambient [CO2] were higher in Chinese yam than in rice. ANOVA revealed that elevated [CO2] more strongly affected most growth parameters except for total DW in Chinese yam than in rice in summer experiment. In this study, solar radiation was higher in summer experiment (180 ± 80 w m−2) than in autumn experiment (150 ± 61 w m−2). The results consequently suggest that the contribution of elevated [CO2] is higher in Chinese yam than that in rice under summer conditions. Additional study is needed to confirm in the future.
The results of some studies have suggested that increasing [CO2] will result in increased photosynthesis (Kimball et al., Citation2002; Vu et al., Citation1997; Yang et al., Citation2006). Several recent studies have shown that photosynthesis of C3 plants increases by 30–50% with doubled [CO2] (Ainsworth & Rogers, Citation2007; Baker et al., Citation1992). De Costa et al. (Citation2003) observed that leaf net photosynthetic rates of two rice crops were significantly higher in CO2-enriched chambers than in ambient-air chambers, namely, 51–75% higher in the maha season (October–March) and 22–23% higher in the yala season (April–September). Katny et al. (Citation2005) have reported that photosynthesis in potato is increased by 10–40% under elevated [CO2]. In radish, net photosynthetic rate has been observed to increase by 46% on average under elevated [CO2] (Usuda, Citation2004). In our study, [CO2] and air temperature were found by ANOVA to have significant effects on net photosynthetic rate in both experiments for Chinese yam, but these effects were not significant for rice (Figure ). However, there is no significant effect of [CO2] × air temperature interaction on yam photosynthesis in both experiments as with it on the total DW. The net photosynthetic rate of Chinese yam was higher under elevated [CO2] than under ambient [CO2] in summer experiment and showed an increasing trend from ambient [CO2] to elevated [CO2] in autumn experiment. The rates in summer experiment were increased by 73 and 39% in elevated [CO2] under low- and high-temperature regimes, respectively. In rice under the same experimental conditions, however, no significant differences in net photosynthetic rate were detected between ambient and elevated [CO2] in summer and autumn experiments (Figure ). These findings indicate that photosynthesis responds more readily to elevated [CO2] in Chinese yam than in rice under the both seasons.
Some studies have shown that plant photosynthetic responses to elevated [CO2] may vary depending on the sink–source balance. Ainsworth et al. (Citation2004) tested the source–sink hypothesis of down-regulated photosynthesis under elevated [CO2] in field trials with two lines of soybean. They found that plants with a smaller sink capacity showed a more severe photosynthetic down-regulation than those with a larger sink capacity under elevated [CO2]. Shimono et al. (Citation2010) examined the role of sink size on photosynthetic acclimation under elevated [CO2] by removing the panicle in rice, and confirmed that sink-limitation in rice did not prevent photosynthetic down-regulation under long-term elevated [CO2]. Usuda and Shimogawara (Citation1998) have reported that elevated [CO2] increases DW accumulation by 105% in storage roots of radish and enhances sink capacity. Chen and Setter (Citation2012) have shown that elevated [CO2] during the potato tuber initiation stage mainly increases DW accumulation in existing sinks (leaves and stems), with cell proliferation in tubers strongly up-regulated. Chinese yam is a root and tuber crop whose tubers have a large sink capacity, whereas the sink capacity of rice is small during the vegetative phase. The results of our research indicate that the effect of elevated [CO2] on photosynthetic rate is greater in Chinese yam than in rice. Hence, our results are consistent with the source–sink hypothesis.
In the future, climatic conditions will be changed with increasing [CO2] and air temperature. The information on the effects of elevated [CO2] at different conditions in Chinese yam that were uncovered in this study will be useful for developing strategies to grow and breed yams suitable for cultivation under different future climatic conditions.
Disclosure Statement
The authors have no conflict of interest to declare.
Acknowledgment
We would like to thank Hiroki Suto, Teaching and Research Center, for Bio-coexistence, Hirosaki University, Japan, for his technical support in the plant cultivation.
References
- Aien, A., Pal, M., Khetarpal, S., & Kumar, P. S. (2014). Impact of elevated atmospheric CO2 concentration on the growth and yield in two potato cultivars. Journal of Agriculture Science and Technology, 16, 1661–1670.
- Ainsworth, E. A., & Rogers, A. (2007). The response of photosynthesis and stomatal conductance to rising [CO2]: Mechanisms and environmental interactions. Plant, Cell and Environment, 30, 258–270. doi:10.1111/j.1365-3040.2007.01641.x
- Ainsworth, E. A., Rogers, A., Nelson, R., & Long, S. P. (2004). Testing the ‘source–sink’ hypothesis of down-regulation of photosynthesis in elevated [CO2] in the field with single gene substitutions in Glycine max. Agricultural and Forest Meteorology, 112, 85–94. doi:10.1016/j.agrformet.2003.09.002.
- Baker, J. T., Allen, J. L. H., & Boote, K. J. (1992). Temperature effects on rice at elevated CO2 concentration. Journal of Experimental Botany, 43, 959–964. doi: 10.1093/jxb/43.7.959
- Chen, T. C., & Setter, T. L. (2012). Response of potato dry matter assimilation and partitioning to elevated CO2 at various stages of tuber initiation and growth. Environmental and Experimental Botany, 80, 27–34. doi.10.1016/j.envexpbot.2012.02.003
- Cheng, W., Sakai, H., Yagi, K., & Hasegawa, T. (2009). Interactions of elevated [CO2] and night temperature on rice growth and yield. Agricultural and Forest Meteorology, 149, 51–58. doi:10.1016/j.agrformet.2008.07.006
- Conn, J. S., & Cochran, V. L. (2006). Responses of potato (Solanum tuberosum L.) to elevated atmospheric CO2 in north American Subartic. Agriculture, Ecosystems and Environment, 112, 49–57. doi:10.1016/j.agee.2005.07.010
- Coursey, D. G., & Haynes, P. H. (1970). Root crops and their potential as food in the tropics. World Crops, 22, 261–265.
- Craigon, J., Frangmeier, A., Jones, M., Donnelly, A., Bindi, M., De, T. L., … Ojanpera, K. (2002). Growth and marketable-yield responses of potato to increased CO2 and ozone. European Journal of Agronomy, 17, 273–289. doi:10.1016/S1161-0301(02)00066-7
- Cruz, J. L., Alves, A. A. C., LeCain, D. R., Ellis, D. D., & Morgan, J. A. (2014). Effect of elevated CO2 concentration and nitrate: ammonium ratios on gas exchange and growth of cassava (Manihot esculenta Crantz). Plant and Soil, 374, 33–43.10.1007/s11104-013-1869-8
- De Costa, W. A. J. M., Weerakoon, W. M. W., Herath, H. M. L. K., & Abeywardena, R. M. I. (2003). Response of growth and yield of rice (Oryza sativa) to elevated atmospheric carbon dioxide in the subhumid zone of Sri Lanka. Journal of Agronomy and Crop Science, 189, 83–95. doi:10.1046/j.1439-037X.2003.00013.x
- Fernandez, M. D., Tezara, W., Rengifo, E., & Herrera, A. (2002). Lack of downregulation of photosynthesis in a tropical root crop, cassava, grown under an elevated CO2 concentration. Functional Plant Biology, 29, 805–814. doi:10.1071/PP01165
- Gleadow, R. M., Evans, J. R., McCaffery, S., & Cavagnanro, T. R. (2009). Growth and nutritive value of cassava (Manihot esculenta Crantz) are reduced when grown in elevated CO2. Plant Biology, 1, 76–82. doi:10.1111/j.1438-8677.2009.00238.x
- Holden, N. M., & Brereton, A. J. (2003). Potential impact of climate change on maize production and the introduction of soybean in Ireland. Irish Journal of Agricultural and Food Research, 42, 1–15.
- Ike, P. C. (2012). Trend analysis of climate change factors and yield of yam in Bayelsa state, Nigeria. Journal of Agriculture and Food Sciences, 10, 18–26. Retrieved from http://dx.doi.org/10.4314/jafs.v10i1.3
- Imai, K., Coleman, D. F., & Yanagisawa, T. (1984). Elevated atmospheric partial pressure of carbon dioxide and dry matter production of cassava (Manihot esculenta Crantz). Japanese Journal of Crop Science, 53, 479–485. Retrieved from http://doi.org/10.1626/jcs.53.479
- Inter-governmental Panel on Climate Change. (2013). Fifth assessment report on climate change 2013. Working Group 1.. Geneva: IPCC secretariat.
- Katny, C. A. M., Thoma, H. G., Schrier, A. A., Fangmeier, A., Jager, J. H., & Bel, E. J. A. (2005). Increase of photosynthesis and starch in potato under elevated CO2 is dependent on leaf age. Journal of Plant Physiology, 162, 429–438. doi:10.1016/j.jplph.2004.07.005
- Kimball, B. A., Kobayashi, K., & Bindi, M. (2002). Responses of agricultural crops to free-air CO2 enrichment. Advances in Agronomy, 77, 293–386. doi:10.1016/S0065-2113(02)77017-X
- Kumagai, E., & Sameshima, R. (2014). Genotypic differences in soybean yield responses to increasing temperature in a cool climate are related to maturity group. Agricultural and Forest Meteorology, 198–199, 265–272. doi:10.1016/j.agrformet.2014.08.016
- Marcos, J., Cornet, D., Bussiere, F., & Sierra, J. (2011). Water yam (Dioscorea alata L.) growth and yield as affected by the planting date: Experiment and modeling. European Journal of Agronomy, 34, 247–256. doi:10.1016/j.eja.2011.02.002
- Miglietta, F., Magliulo, V., Bindi, M., Cerio, L., Vaccari, FP., Loduca, V., & Peressotti, A. (1998). Free Air CO2 enrichment of potato (Solanum tuberosum L.): development, growth and yield. Global Change Biology, 4, 163–172. doi:10.1046/j.1365-2486.1998.00120.x
- Newman, Y. C., Sollenberger, L. E., Boote, K. J., Allen, Jr., L. H., Vu, J. C. V., & Hall, M. B. (2005). Temperature and carbon dioxide effect on nutritive value of rhizome peanut herbage. Crop Science, 45, 316–321. doi:10.2135/cropsci2005.0316
- National Oceanic and Atmospheric Administration- Earth System Research Laboratory. (2014). Trends in atmospheric carbon dioxide. Retrieved from http://www.esrl.noaa.gov/gmd/ccgg/trends/
- Nonhebel, S. (1993). The effect of changes in temperature and CO2 concentration on simulated spring wheat yields in The Netherlands. Climatic Change, 24, 311–329. doi:10.1007/BF01091853
- Oseni, T. O., & Masarirambi, MT. (2011). Effect of climate change on maize (Zea mays) production and food security in Swaziland. American-Eurasian Journal of Agricultural and Environmental Sciences, 11, 385–391.
- Prasad, P. V. V., Boote, K. J., & Allen, L. H. (2006). Adverse high temperature effects on pollen viability, seed-set, seed yield and harvest index of grain-sorghum [Sorghum bicolor (L.) Moench] are more severe at elevated carbon dioxide due to higher tissue temperatures. Agricultural and Forest Meteorology, 139, 237–251. doi:10.1016/j.agrformet.2006.07.003
- Raymundo, R., Asseng, S., Cammarano, D., & Quiroz, R. (2014). Potato, sweet potato, and yam models for climate change: A review. Field Crops Research, 166, 173–185. doi:10.1016/j.fcr.2014.06.017
- Rosenthal, D. M., Slattery, R. A., Grennan, A. K., Cavagnaro, T. R., Fauquet, C. M., & Ort, D. R. (2012). Cassava about-FACE: Greater than expected yield stimulation of cassava (Manihot esculenta) by future CO2 levels. Global Change Biology, 18, 2661–2675. doi:10.1111/j.1365-2486.2012.02726.x
- Roy, K. S., Bhattacharyya, P., Neogi, S., Rao, K. S., & Adhya, T. K. (2012). Combined effect of elevated CO2 and temperature on dry matter production, net assimilation rate, C and N allocations in tropical rice (Oryza sativa L.). Field Crops Research, 139, 71–79. doi:10.1016/j.fcr.2012.10.011
- Shimono, H., Okada, M., Yamakawa, Y., Nakamura, H., Kobayashi, K., & Hasegawa, T. (2008). Rice yield enhancement by elevated CO2 is reduced in cool weather. Global Change Biology, 14, 276–284. doi:10.1111/j.1365-2486.2007.01498.x
- Shimono, H., Okada, M., Yamakawa, Y., Nakamura, H., Kobayashi, K., & Hasegawa, T. (2009). Genotype variation in rice yield enhancement by elevated CO2 relates to growth before heading, and not to maturity group. Journal of Experimental Botany, 60, 523–532. doi:10.1093/jxb/ern288
- Shimono, H., Suzuki, K., Aoki, K., Hasegawa, T., & Okada, M. (2010). Effect of panicle removal on photosynthetic acclimation under elevated CO2 in rice. Photosynthetica, 48, 530–536.10.1007/s11099-010-0070-z
- Srivastava, K. A., & Gaiser, T. (2010). Simulating biomass accumulation and yield of yam (Dioscorea alata) in the Upper Ouémé Basin (Benin Republic)- I. Compilation of physiological parameters and calibration at the field scale. Field Crops Research, 116, 23–29. doi:10.1016/j.fcr.2009.10.018
- Srivastava, KA., Gaiser, T., Heiko, P., & Ewert, F. (2012). The impact of climate change on Yam (Dioscorea alata) yield in the savanna zone of West Africa. Agriculture, Ecosystems and Environment, 153, 57–64. doi:10.1016/j.agee.2012.03.004
- Tacarindua, C. R. P., Shiraiwa, T., Homma, K., & Kumagai, E. (2013). The effects of increased temperature on crop growth and yield of soybean grown in a temperature gradient chamber. Field Crops Research, 154, 74–81. doi:10.1016/j.fcr.2013.07.021
- United nation, Department of Economic and Social Affairs. (2015). World population prospects: The 2015 revision. Retrieved from https://esa.un.org/unpd/wpp/Publications/Files/WPP2015_Methodology.pdf
- Usuda, H. (2004). Effect of elevated CO2 on the capacity of photosynthesis in two radish cultivars differing in capacity of storage roots. Plant Production Science, 7, 377–385. doi:10.1626/pps.7.377
- Usuda, H., & Shimogawara, K. (1998). The effects of increased atmospheric carbon dioxide on growth, carbohydrates, and photosynthesis in radish, Raphanus sativus. Plant and Cell Physiology, 39, 1–7.10.1093/oxfordjournals.pcp.a029280
- Valizadeh, J., Ziaei, S. M., & Mazloumzadeh, S. M. (2014). Assessing climate change impacts on wheat production (a case study). Journal of the Saudi Society of Agricultural Sciences, 13, 107–115. doi:10.1016/j.jssas.2013.02.002
- Vu, J. C. V., Allen, L. H. J. R., Boote, K. J., & Bowes, G. (1997). Effects of elevated CO2 and temperature on photosynthesis and Rubisco in rice and soybean. Plant, Cell and Environment, 20, 68–76. doi:10.1046/j.1365-3040.1997.d01-10.x
- Wall, G. W., Brooks, T. J., Adam, N. R., Cousins, A. B., Kimball, B. A., Pinter, Jr., P. J., … Webber, A. N. (2001). Elevated atmospheric CO2 improved Sorghum plant water status by ameliorating the adverse effects of drought. New Phytologist, 152, 231–248. doi:10.1046/j.0028-646X.2001.00260.x
- Yang, L., Huang, J., Yang, H., Dong, G., Liu, G., Zhu, J., & Wang, Y. (2006). Seasonal changes in the effects of free-air CO2 enrichment (FACE) on dry matter production and distribution of rice (Oryza sativa L.). Field Crops Research, 98, 12–19. doi:10.1016/j.fcr.2005.11.003
- Zakari, D. M., Mohammad, A. B., Medugu, N. I., & Sandra, I. (2014). Impact of climate change on yam production in Abuja, Nigeria. International Journal of Science, Environment and Technology, 3, 458–472.
- Ziska, L. H., Namuco, O., Moya, T., & Quilang, J. (1997). Growth and yield response of field-grown tropical rice to increasing carbon dioxide and air temperature. Agronomy Journal, 89, 45–53. doi:10.2134/agronj1997.000219620089000100