ABSTRACT
Bacterial diseases are a major cause of yield loss in tomato plants worldwide, and there is a need to identify strategies for control of these diseases. Bacterial wilt caused by Ralstonia solanacearum (RS) and bacterial spot caused by Xanthomonas axonopodis pv vesicatoria (Xav) are amongst the bacterial diseases affecting tomato crops. The current study investigates Euphorbia hirta (E. hirta) methanol extract (EHM) for its antibacterial activity against RS, and Xav induced diseases in tomato plants, along with its biostimulant potential in increasing fruit yield. EHM at 1280 mg/L exhibited 90% inhibition in the growth of both RS and Xav bacteria. Further, evaluation of EHM on Arka Vikas, a susceptible tomato variety, showed that pre-treatment of 15 days and 45 days tomato plants with 1280 mg/L EHM exhibited moderate resistance to bacterial wilt and spot disease, respectively, with increased tomato yield and improved chlorophyll b levels compared to control plants. The effectiveness of EHM in increasing fruit yield was further confirmed using field evaluation on ‘Namdhari NS 538’ variety of tomato plants. A 3.5-fold increase in numbers and weight of tomato was observed with foliar application of EHM (1280 mg/L). A significant reduction in fruit spoilage along with an increase in mRNA level of ethylene receptor factor (ERF1) was also observed. The study is the first to demonstrate the cost-effective and eco-friendly antibacterial and biostimulant potential of EHM (1280 mg/L) for tomato plants.
GRAPHICAL ABSTRACT
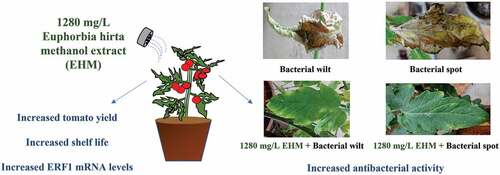
Introduction
Tomato (Solanum lycopersicum) is a widely consumed crop throughout the world. Bacterial wilt and bacterial spot are the common diseases affecting tomato fruit quality, leading to major loss in global tomato production (Nion & Toyota, Citation2015). The bacterial wilt caused by Ralstonia solanacearum (RS) acts by infecting tomato plants through roots, and invades the stem systemically. High bacterial populations clog the stem, causing complete wilt of mature plants (Zheng et al., Citation2017). Bacterial spot caused by Xanthomonas axonopodis pv vesicatoria (Xav) acts by entering through stomata and wound openings, causing defoliation (Robinson, Citation2014), and affects the quality of tomato crop due to spots on leaves and fruits (Potnis et al., Citation2015).
These diseases are currently controlled using antibiotics such as streptomycin, tetracycline, ampicillin and penicillin or by application of copper-based products. Recent reports indicate that bacteria acquire resistance due to excessive usage of antibiotics (Atif et al., Citation2020; Vu et al., Citation2017). Atif et al. (Citation2020) have stressed on the importance of effective alternative methods in the field of agriculture to reduce the usage of highly toxic chemicals. Our study focuses on identifying cost-effective, environmentally friendly, and biodegradable strategies for disease control and improving crop yield.
Plant biostimulants, defined as ‘any substance or compound other than primary, secondary and microelement plant nutrients, that can be demonstrated by scientific research to be beneficial to one or more plant species when applied exogenously’ (AAPFCO, Citation2012), are being used in agriculture (Du Jardin, Citation2015). Colla et al. (Citation2017) have reported the antibacterial and yield-enhancing biostimulant properties of plant extracts (Colla et al., Citation2017). The present study investigates the antibacterial and biostimulant potential of Euphorbia hirta (E. hirta).
E. hirta, commonly known as Asthma weed, is a pantropical and perennial herb belonging to the Euphorbiaceae family, frequently found in open grasslands and fields in India. It is a source of several phytochemical constituents, including reducing sugars, terpenoids, alkaloids, steroids, tannins, cardiac glycosides, anthraquinones, flavonoids, proteins, fats, oils, gums, mucilages, glycoside, saponin, coumarin, and phenolic compounds and is used in traditional herbal medicine for its antibacterial activity (Al-Snafi, Citation2017; Kumar et al., Citation2010). The methanol extract of E. hirta plant has been observed to exhibit antibacterial activity against RS, Xav, Escherichia coli and Staphylococcus aureus (Al-Snafi, Citation2017; Angelika & Suprihadi, Citation2014). Aqueous extract of E. hirta has been reported to show activity against Agrobacterium rhizogenes and Xanthomonas campestris (Sheikh et al., Citation2012). In addition, chloroform and hexane extracts have also been observed to exhibit antibacterial activity against plant pathogens Streptococcus mutans and Clostridium absonum (Ahmad et al., Citation2017).
In the present study, hexane, dichloromethane (DCM), ethyl acetate and methanol extracts of E. hirta leaf were tested for their antibacterial activity in vitro against RS and Xav. The bioactive methanol extract of E. hirta (EHM) was evaluated in vivo for its role in disease control in Arka Vikas variety of tomatoes for the first time. The EHM extract induced increase in fruit yield in Arka Vikas variety led to further evaluation of various doses of E. hirta bioactive extract on fruit yield in ‘Namdhari NS 538’ variety of tomatoes in field study.
Materials and methods
Bacterial strain identification and culture conditions
Ralstonia solanacearum (RS) and Xanthomonas axonopodis pv vesicatoria (Xav) were a kind gift from Dr. S. Umesha, Department of Studies in Biotechnology, University of Mysore, India. RS was identified as rod-shaped, gram-negative and motile bacteria when viewed under the microscope after gram staining. Further confirmation was obtained by observation of white with pink center colonies when RS was grown in media containing 2,3,5 – triphenyl tetrazolium chloride in agar medium similar to Kelman (Citation1954). Xav was identified by gram staining and appeared as a gram-negative rod-shaped bacterium under the microscope. Further confirmation was the observation of the formation of large, smooth-domed colonies with yellow mucoid-fluidal edges when grown on yeast dextrose chalk agar, while small yellow colonies were formed on nutrient agar similar to Mitrev and Kovačević (Citation2006).
RS was cultured and maintained in casein acid hydrolysate-peptone-dextrose-agar, also known as casamino acids-peptone-glucose (CPG) (pH 6.5–7) (HiMedia Pvt Ltd, India) and Xav was cultured and maintained in Nutrient agar (pH 7.3) (HiMedia Pvt Ltd, India). The bacteria were incubated at 28°C for 48 h at 150 rpm.
Sequential extraction of euphorbia hirta
Authenticated dry leaf powder of E. hirta was procured from Indian Medical Practitioner’s Co-operative Pharmacy and Stores (IMPCOPS), Chennai, India. The leaf powder (100 g) was soaked overnight in 200 mL of hexane, filtered using Whatman filter paper No. 1, and hexane extract (150 mL) was collected. The process was repeated five times and the collected 750 mL of hexane extract was concentrated using a rotary evaporator (Heidolph, Germany), and stored at room temperature after complete drying (EHH). Subsequently, 200 mL DCM was added to the same leaf powder residue, and a similar procedure repeated five times. The 750 mL DCM extract was concentrated and stored at room temperature after complete drying (EHD). The leaf powder residue was subsequently subjected to similar extraction procedure using ethyl acetate and the 750 mL ethyl acetate extract was concentrated and stored at room temperature after complete drying (EHE). Finally, the leaf powder residue was subjected to a similar extraction procedure using methanol and the 750 mL methanol extract was concentrated and stored at room temperature after complete drying (EHM).
The dry weights of the hexane, DCM, ethyl acetate, and methanol extracts were 2 g, 0.9 g, 1.2 g, and 3 g, respectively. The extracts were reconstituted to the required concentrations in 10 mM phosphate buffer saline (PBS) for in vitro and in vivo evaluation.
Broth microdilution test
The antibacterial test was performed as described by Klančnik et al. (Citation2010). The various extracts of the E. hirta leaf were serially diluted in an appropriate growth medium and added to individual wells of a sterile 96 well microtiter plate. The untreated bacteria served as experimental control and bacteria treated with streptomycin sulphate (50 mg/L) was used as a positive control (Im et al., Citation2020).
Protein analysis
The RS and Xav were grown in their respective media, and treated with EHM for 48 h. The cultures were then centrifuged at 4000 g for 20 min and the supernatant discarded. The bacterial pellet was washed twice and suspended in 1 mL PBS and sonicated for 20 min (sonication cycle: 1 min on 1 min off (Saranya et al., Citation2014). After sonication, the samples were centrifuged at 10,000 g for 30 min. Proteins were precipitated from the supernatant through the addition of 1/10 the volume of 10% trichloroacetic acid (Awad & Brueck, Citation2020) and incubated at -20 ºC for a minimum period of 1 h. After incubation, the samples were centrifuged at 10,000 rpm for 30 min at 4 ºC, and the obtained pellet was washed twice with PBS and stored at -20 ºC. The protein concentration of these samples was determined by Bradford assay with bovine serum albumin (BSA) as the protein standard. Equal amounts of proteins (60 µg) were separated on 12% sodium dodecyl sulfate – polyacrylamide gel electrophoresis (SDS-PAGE) alongside protein markers.
In vivo studies for antibacterial activity
The tomato cultivar Arka Vikas (a susceptible variety of tomato plant grown in India) was procured from the Indian Institute of Horticulture Research, Bangalore, India. The study was conducted with slight modifications to Balestra et al. (Citation2009) protocol. The plant was grown in a soil mixture containing sand and manure in a ratio of 1:1. RS and Xav were grown in their respective medium for 48 h and subsequently diluted to 0.3 OD at 600 nm wavelength. Subsequently, a set containing 15 days old plants and another set containing 45 days old tomato plants were used for the study.
Treatments involved five groups, each group containing 3 plants:
Group 1: 10 mM PBS + no infection (experimental control for the study)
Group 2: 10 mM PBS + bacterial infection (infection control for the study)
Group 3: Streptomycin sulphate (50 mg/L) + bacterial infection (positive control for the study)
Group 4: EHM IC90 + bacterial infection (test for the study)
Group 5: EHM IC90 + no infection (to evaluate the effect of EHM IC90 on tomato production)
The RS and Xav infections were initiated by spraying bacterial suspension on the soil (soil drenching) and leaves (foliar application) of tomato plants, respectively (Henry et al., Citation2017). One day prior to infection, the plants were sprayed with streptomycin sulphate and EHM IC90. The experiments were carried out between October to March and the plants maintained in a naturally ventilated polyhouse were watered in the early mornings and growth parameters were recorded after 30 days of infection. The study was repeated three times.
Measurement of disease severity
The Horsfall and Barratt score gives a measurement of the severity of the disease and classifies plants to be either susceptible or resistant to disease based on the score. In the study, the disease severity of infected plants was calculated as per Horsfall and Barratt scoring pattern by measuring the ratio of infected leaves to total leaves of the plant and multiplying the ratio by a hundred, providing the percentage of infection (Horsfall, Citation1945).
Leaf pigment content
Leaf pigment content was measured with a slight modification to Kabir et al. (Citation2018) methodology. Leaf samples (50 mg) collected from plants after 30 days of infection with RS and Xav were macerated in 2 mL acetone (100%) and centrifuged for 30 min. The absorbance of the collected supernatant was measured at 662 nm (A662), 646 nm (A646), and 470 nm (A470). The amount (μg) of chlorophyll a (chl a), chlorophyll b (chl b) present in 1 mL of the extract were calculated using the following formula.
Chl a (μg/mL of leaf extract) = (11.75 × A662) – (2.35 × A646)
Chl b (μg/mL of leaf extract) = (18.61 × A646) – (3.96 × A662)
Determination of hydrogen peroxide (H2O2) content
Leaf samples collected after 30 days of infection (RS and Xav) were centrifuged for 15 min at 12,000 g after homogenization with 0.1% (w/v) trichloroacetic acid (5 mL) at 4 ºC. 0.5 mL of potassium phosphate buffer (10 mM, pH 7.0) and 1 mL potassium iodide (1 M) were added to 0.5 mL of the supernatant and incubated for 10 min. Then absorbance was measured at 390 nm. H2O2 was used as a standard for the study (Ahanger et al., Citation2020).
Nitric oxide (NO) release assay
Leaf samples collected after 30 days of infection (RS and Xav) were homogenized using 5 mL of extraction buffer (50 mM Tris-HCl, pH 8.5, 14.4 mM 2-mercaptoethanol, and 5% w/v insoluble polyvinylpolypyrrolidone) and centrifuged for 10 min at 10,000 g (4 ºC). Equal volumes of Griess reagent (0.5% sulfanilamide, 2.5% phosphoric acid, and 0.05% N-1-naphthyl ethylenediamine dihydrochloride), and supernatant were mixed to determine the nitrite levels in the supernatant. The reaction was incubated for 10 min at room temperature in the dark, and OD was measured at 540 nm. Sodium nitrite was used as a positive control for the study (Shilpa & Lakshmi, Citation2019).
Phenylalanine ammonia lyase (PAL) assay
Leaves of tomato plants collected after 30 days of infection (RS and Xav) were homogenized with 5 mL extraction buffer and centrifuged for 10 min at 10,000 g (4 ºC). The supernatant was used to analyze protein concentration using Bradford’s method and determination of PAL enzyme activity. The supernatant (0.5 mL) was incubated with 2.5 mL of L-phenylalanine (0.2% w/v in 50 mM Tris-HCl, pH 8.5) at 37 ºC for 2 h, and the formation of the trans-cinnamic acid was measured at 290 nm (Koike & Shimada, Citation1992). PAL activity was represented as the amount of trans-cinnamic acid formed per mg of protein using trans-cinnamic acid as standard.
In vivo studies for yield
The tomato cultivar ‘Namdhari NS 538’ was procured from Namdhari seeds. The plants were grown in a soil mixture containing sand and manure in a ratio of 1:1. The experimental sites were located at Alakapuram (15.9453° N, 80.6251° E) and Manthenavaripalem (15.9865° N, 80.6521° E) villages of Guntur district, Andhra Pradesh, India. Dried EHM (10.24 g) was dissolved in one-litre water. This extract served as the stock to prepare further dilutions (5120 mg/L - 160 mg/L), while dhanzyme (2 mL/L) served as a positive control for the study. EHM at various concentrations (5120, 2560, 1280, 640, 320 and 160 mg/mL) and dhanzyme (2 mL/L) (positive control) were screened for their effects on tomato yield (in terms of number and weight). Treatments were performed through seed soaking, soil drenching, foliar application and exposure of tomato plant to E. hirta powder mixed in water. This study was conducted with modifications to Parađiković et al. (Citation2018) protocol. Seeds soaked for 24 h in the varied concentration of EHM and dhanzyme were allowed to grow for 10 days. After 10 days, seedlings were transplanted into the field, maintaining a gap of 2 m by 2 m between the consecutive plants and groups. The 45-day-old plants were subjected to soil drenching and foliar application with dhanzyme and different concentrations of EHM, every 15 days. In the last group, E. hirta plant powder was suspended in water in the required concentrations and was applied by soil drenching. In all groups control plants were treated with water. Plants were watered in the early mornings for the entire duration of the experiment. The experiments were conducted between October and March. After 60 days of treatment, the average number of fruits per plant, average fresh weight of tomato per plant, and the number of fruits damaged 10 days after harvest is recorded. The study was repeated three times in three different years.
Lycopene content in tomato
The lycopene content in tomato samples was measured as per Adejo et al. (Citation2015) methodology, with slight modifications. Tomato fruits at the same stage of maturation (Red) were collected from all plants after 30 days of infection (RS and Xav) and homogenized to tomato puree, and 8 mL of a solvent mixture (hexane: ethanol: acetone (2:1:1) (v/v) was added to 0.1 g of the tomato juice. The mixture was vortexed and incubated overnight in the dark. Post-incubation, 1 mL of distilled water was added to the samples and vortexed. The samples were allowed to stand until the two phases separated and bubbles disappeared completely. Absorbance at 503 nm was measured for an aliquot of the upper phase. Water (100 μL) was used as a blank for the study. The amount of lycopene present in extracts was calculated using the following formula.
Lycopene (mg/Kg fresh weight) = (A503 × MWL x Vs x Vr)/(WT x EL)
Where A503 is absorbance recorded at 503 nm, MWL is the molecular weight of lycopene (g/mol), Vs is the volume of the solvent mixture (hexane, ethanol, acetone) (mL), Vr is the volume ratio of the upper layer to the mixed solvents (mL), WT is the weight of tomato added (g), and EL is the extinction coefficient of lycopene in hexane per mM.
RNA extraction, reverse transcription (RT) and polymerase chain reaction (PCR)
The collected leaf samples were frozen in liquid nitrogen before grinding with a pestle and mortar. The powdered sample was added to 1 mL of TRIzol reagent and stored at -20°C until further use. The RNA isolation was performed with a slight modification to Chomczynski and Sacchi (Citation1987) protocol. The obtained RNA pellet was dissolved in nuclease-free water and checked for its concentration and purity by determining the 260/280 and 260/230 ratios using a Nanodrop 2000/2000c spectrophotometer (Thermo Fisher Scientific Ltd). Total RNA (100 ng) was reverse transcribed using PrimeScript RT Reagent Kit (Takara Bio. Inc). The mRNA levels were determined by semi quantitative RT-PCR using Taq DNA polymerase (G Biosciences) and 20 ng cDNA under reaction conditions of 35 cycles of 95°C for 5 min, 95°C for 45 s, X °C (X = 48°C for ERF1 gene; 60°C for SlGA2ox1; 60°C for LeAGP1 and 57°C for GAPDH) for 45 s and 72°C for 45 s and final extension for 7 min at 72°C with a Veriti 96-well thermal cycler (Applied biosystems). The genes analyzed were ERF1 (F-AGCAGCTGGATAATCCATCT, R- TAGGGATTCAACGCGTAATGGA), PCR product size 197 bp), SlGA2ox1 (F-GGGCCATGGTGAATTCATGCA, R-GTACTCACCCCAAGTGAATGG, PCR product size 152 bp) and LeAGP-1 (F-CACTAAGCACGAGGCAACACA, R-AGCTACCAAACTTCCCAGCAT, PCR product size 197 bp). Glyceraldehyde 3-phosphate dehydrogenase (Gapdh) (F-GACAACGAATGGGGTTACAGT, R- CGTTTGTGGTCCGAGAGAACA, PCR product size 166 bp) served as the housekeeping gene for the study. The primers were purchased from integrated DNA technology. Inc. cDNA bands were quantified (ImageLab, Bio-Rad) and normalized to GAPDH levels and control was set arbitrarily as 1.
Statistical analysis
The experiments were performed three times independently, and the data are shown as mean ± standard error. In addition, statistical significance between the two groups was analyzed by Student's t-test, and between multiple groups were analyzed by one-way analysis of variance (ANOVA) using Tukey’s multiple-comparison test. The statistical analysis was performed using GraphPad Prism 8 (GraphPad Software Inc). Data with p <0.05 in comparison to the untreated control and infected group are indicated (*) and (#), respectively.
Results
E. hirta plant powder was subjected to sequential extraction using organic solvents with increasing polarity (hexane, DCM, ethyl acetate, and methanol). The four extracts were then dried and reconstituted using 10 mM PBS, and a dose–response analysis was performed for antibacterial activity using broth microdilution assay. Results showed the methanol extract of E. hirta (EHM) to exhibit maximum activity against RS compared to other extracts (Suplementary figure S1). For Xav, among the four extracts evaluated for their antibacterial activity, all the extracts except DCM exhibited increased bioactivities at different concentrations (Supplementary figure S1). Since EHM showed antibacterial activity against both RS and Xav among the four extracts screened, it was selected for further antibacterial tests.
EHM showed a 90% inhibition (IC90) of RS and Xav growth at 1280 mg/L concentration and a 50% (IC50) inhibition at 320 mg/L and 640 mg/L, respectively (). Further, treatment of RS and Xav with 1280 mg/L EHM exhibited an overall decrease in protein integrity, confirming the antibacterial potential of EHM IC90 (or 1280 mg/L EHM) (). However, treatment of RS and Xav with EHM IC50 showed no significant change in the total protein profile of the bacteria ().
Figure 1. Effect of 24 h and 48 h treatment of E. hirta methanol extract on Ralstonia solanacearum (RS) and Xanthomonas axonopodis pv vesicatoria (Xav) sequential extraction of E. hirta yielded, hexane, dichloromethane (DCM), ethyl acetate, and methanol extracts. Among them the methanol extract was tested for its effect on the growth of (a) RS and (b) Xav.
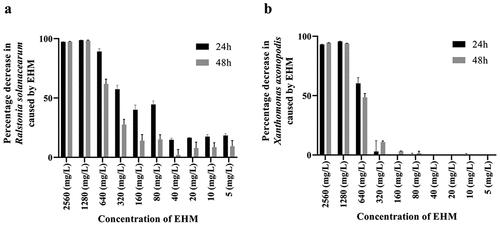
Figure 2. Effect of various treatments on protein profile of a) Ralstonia solanacearum (RS) and b) Xanthomonas axonopodis pv vesicatoria (Xav) lanes represent the following M: marker, 1: control (untreated bacteria), 2: streptomycin sulphate (50 mg/L), 3: E. hirta methanol extract (320 mg/L) for RS whereas E. hirta methanol extract (640 mg/L) for Xav and 4: E. hirta methanol extract (1280 mg/L) for both the bacteria. The treatments were performed for 24 h.
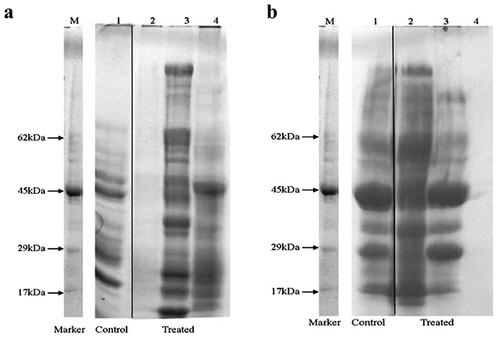
In vivo studies were performed using the tomato cultivar ‘Arka Vikas’ to measure disease severity in plants infected with RS and Xav using the Horsfall and Barratt scoring pattern. The 15-day and 45-day-old tomato plants were subjected to EHM IC90 and streptomycin sulphate treatments 1 day before infection with RS or Xav. The studies conducted were divided into groups based on treatments, as described earlier in materials and methods. The plants pre-treated with EHM IC90 showed moderate resistance to RS and Xav infection after 30 days of disease (). The study also showed 15-day-old plants to be more susceptible to RS infection than 45-day-old plants (), whereas 45-day-old mature plants were more prone to Xav infection than the young 15-day-old plants (). Therefore, for further studies, 15-day-old plants were infected with RS and 45-day-old plants with Xav to analyze the effect of EHM IC90 in vivo. Data were recorded after 30 days of infection with RS and Xav. Plants pre-treated with EHM IC90 displayed resistance to infection similar to those pre-treated with the positive control, streptomycin sulphate (50 mg/L).
Figure 3. Effect of various treatments on bacterial wilt infection and spot infection 15 days old plants infected with Ralstonia solanacearum (RS) and 45 days old plants infected with Xanthomonas axonopodis pv vesicatoria (Xav) were pre-treated with EHM (1280 mg/L) and streptomycin sulphate (50 mg/L) and photographed after 30 days of infection. Representative photographs are shown. 15 and 45 days old arka vikas plants infected with (a) RS and (b) Xav and pre-treated with EHM (1280 mg/L) and streptomycin sulphate (50 mg/L) were considered for percentage infection. Data shown reflect the mean ± standard error mean. (*) and (#) represent p < 0.05 in comparison to control group and infected group respectively.
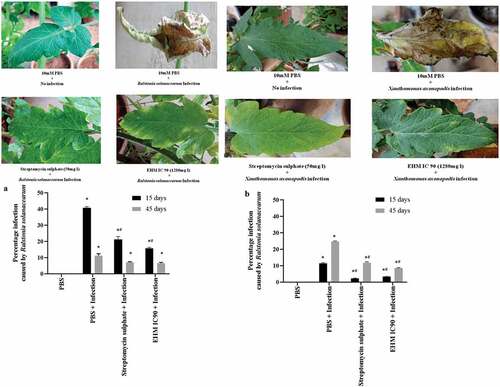
After 30 days infection period, leaves from plants of various treatments were evaluated for Chl a, Chl b, and carotenoids. Plants infected with RS showed a significant increase in the concentration of Chl b with no change in Chl a and carotenoid levels (). EHM IC90 pre-treatment in plants infected with RS showed a significant increase in Chl a and Chl b concentration, with no significant change in carotenoid content compared to control and infected plants (). Streptomycin sulfate pre-treatment was observed to significantly reduce Chl a, Chl b, and carotenoid concentration compared to uninfected and RS infected plants ().
Figure 4. Effect of various treatments on levels of chlorophyll pigment the (a) 15 day old plants were infected with Ralstonia solanacearum (RS) and (b) 45 day old plants were infected with Xanthomonas axonopodis pv vesicatoria (Xav). After 30 days of infection, leaves from every group were analyzed for chlorophyll a (Chl a), chlorophyll b (Chl b) and carotenoids concentration (μg) per ml of the leaf extract. Acetone was used to extract leaf pigments, and the absorbance of collected supernatant was measured at 662 nm, 646 nm, and 470 nm. Data shown reflect the mean ± standard error mean. (*) and (#) represent p < 0.05 in comparison to control group and infected group respectively.
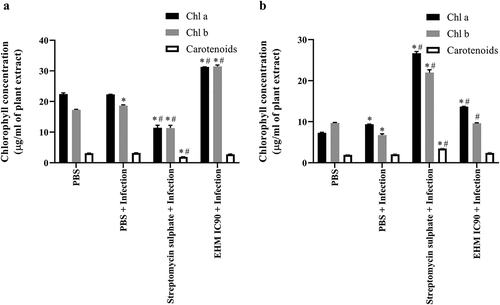
In the study involving plants infected with Xav, a significant increase in concentration of Chl a and decrease in Chl b concentration was observed with no significant change in carotenoid levels compared to control plants (). However, plants treated with EHM IC90 before Xav infection showed a significant increase in chlorophyll content compared to both control and infected plants. In contrast, a significant rise in Chl b content was observed only in comparison to infected plants, with no significant change in carotenoid content compared to control plants or infected plants (). Streptomycin sulfate treatment showed a significant increase in Chl a, Chl b, and carotenoid concentrations compared to control and Xav-infected plants ().
H2O2 and NO are generated in response to infection, whereas the PAL enzyme has been associated with disease resistance. No significant change in H2O2 and a significant decrease in NO and an increase in PAL activity was observed upon infection with RS (). RS-infected plants pre-treated with EHM IC90 showed a significant decrease in NO levels compared to uninfected plants, and a reduction in H2O2 and increased PAL activity in comparison to control plants (). The streptomycin sulfate (positive control) treated plants showed no significant change in H2O2 levels, a decrease in NO levels and an increase in PAL activity in comparison to RS infected and uninfected plants ().
Figure 5. Effect of various treatments on defence molecules in plants infected with (a) Ralstonia solanacearum (RS) and (b) Xanthomonas axonopodis pv vesicatoria (Xav) hydrogen peroxide, nitric oxide and PAL activity were expressed in terms of fold difference. The study was conducted using leaf tissue homogenate. All the experiments mentioned above were performed independently three times in triplicates. Data shown reflect the mean ± standard error mean. (*) and (#) represent p < 0.05 in comparison to control group and infected group respectively.
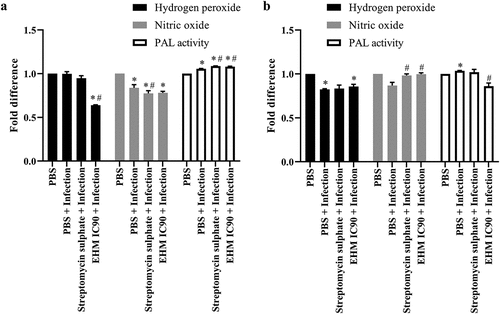
A significant reduction in H2O2 levels and an increased PAL activity with no significant change in NO levels were observed with Xav-infected plants in comparison to control (). Xav-infected plants pre-treated with EHM IC90 showed a significant reduction in H2O2 levels compared to control plants and a significant increase in NO levels and decreased PAL activity in comparison to the infected plants (). Pre-treatment with streptomycin sulfate showed no significant change in H2O2 levels and PAL activity. However, an increase in NO levels was observed in comparison to infected plants ().
Analysis of tomato fruit yield showed that the plants treated with EHM IC90 prior to RS and Xav infection exhibited a significant increase in fruit number in comparison to uninfected and infected plants (). EHM IC90 pre-treatment to plants infected with RS and Xav showed a significant increase in total tomato weight per plant compared to control and infected plants (). The studies also showed an increase in fruit yield in terms of number and weight upon pre-treatment with positive control. To confirm that the increase in tomato yield was not in response to the infections, uninfected plants were treated with EHM IC90. The results showed a significant increase in the number of tomatoes and the total weight of tomatoes per plant compared to the control group. These observations indicate the yield increasing potential of EHM IC90 in tomato plants.
Figure 6. Effect of various treatments on tomato production (number and weight) (a) the values represented as the average of the number of tomatoes and (b) total weight of tomatoes per plant produced after 30 days from pre-treated 15 days old plants infected with Ralstonia solanacearum (RS) and 45 days old plants infected with Xanthomonas axonopodis pv vesicatoria (Xav). The experiments mentioned above were repeated three times in triplicates. Data shown reflect the mean ± standard error mean. (*) and (#) represent p < 0.05 in comparison to control group and infected group respectively.
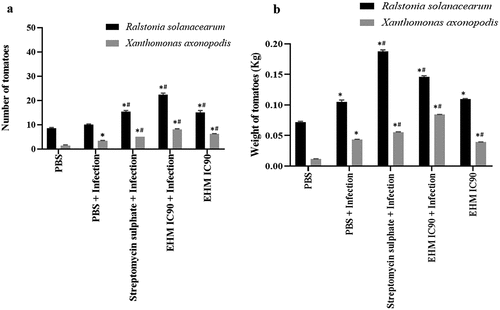
Analysis of the lycopene content in the tomato fruits showed a significant difference between the control and infected plants. EHM IC90 pre-treated RS and Xav-infected plants showed a significant increase in lycopene content compared to their respective uninfected, infected, and streptomycin sulphate groups (). Streptomycin sulfate pre-treated plants showed a significant increase in lycopene content in RS-infected plants and exhibited no significant change in Xav-infected plants (). Uninfected plants treated with EHM IC90 showed a significant increase in lycopene content compared to control, indicating improved fruit quality by EHM IC90 in tomato plants.
Figure 7. Effect of various treatments on lycopene levels of tomatoes lycopene values are represented as the average of lycopene levels (mg/Kg fresh weight) from tomatoes collected after (a) 30 days of infection 15 days old plants infected with Ralstonia solanacearum (RS) and (b) 45 days old plants infected with Xanthomonas axonopodis pv vesicatoria (Xav). The experiments mentioned above were repeated three times in triplicates. Data shown reflect the mean ± standard error mean. (*) and (#) represent p < 0.05 in comparison to control group and infected group respectively.
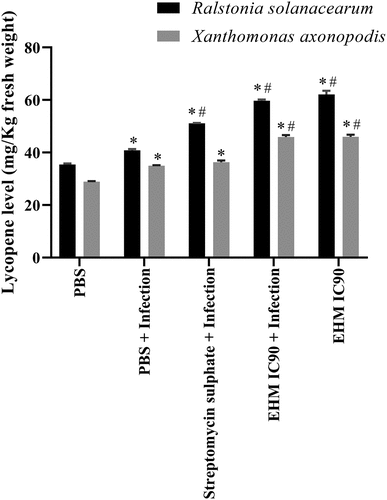
Further, the effect of various concentrations of EHM (5120, 2560, 1280, 640, 320 and 160 mg/mL) on Namdhari NS 538 tomato yield was analyzed. The effect of EHM extract on tomato yield with different modes of treatment such as seed soaking (SS), soil drenching (RT), foliar spray (SP) and soil drenching with EHM plant powder mixed with water (w/v) (PW) were analyzed. It was observed from the study that tomato plants subjected to 1280 mg/L EHM (EHM IC90) foliar spray application showed a significant increase in tomato weight () and number () compared to control plants. Furthermore, plants subjected to dhanzyme (2 mL/L) foliar application showed a significant increase in fruit yield. As the foliar application of tomato plants with 1280 mg/L EHM showed an increase in tomato yield, fruits from these plants were further analyzed for the differences in weight of 10 tomatoes per plant, the number of fruits damaged in 10 days and lycopene content in comparison to control plants and dhanzyme treated plants.
Figure 8. Effect of various concentrations of E. hirta methanol (EHM) extracts on tomato (a) number and (b) weight by soaking tomato seeds (SS), or 45 days old tomato plants soil drenched (RT), or foliar sprayed (SP) with 5120, 2560, 1280, 640, 320 and 160 mg/mL EHM or soil drenched using E. hirta powder mixed in water (PW) at 5120, 2560, 1280, 640, 320 and 160 mg/mL concentrations. Control plants were treated with water while dhanzyme (2 mL/L) served as positive control. The experiments mentioned above were repeated three times in triplicates. Data shown reflect the mean ± standard error mean. (*) represent p < 0.05 in comparison to control group.
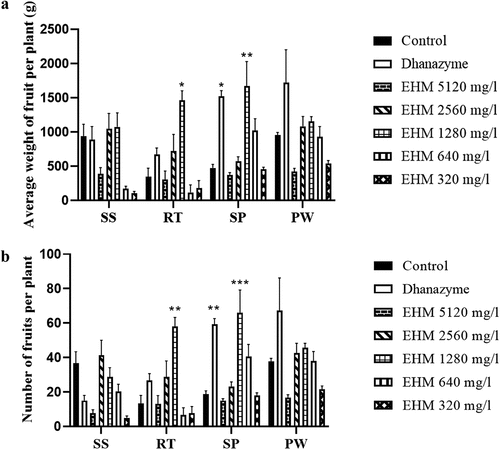
Results show that foliar application of 1280 mg/L EHM significantly increased the average weight of tomatoes compared to control plants (). Weight distribution also showed an overall increase in fruit weight upon treatment with 1280 mg/L EHM (). EHM (1280 mg/L) foliar application in tomato plants was found to increase the shelf life of tomatoes by significantly decreasing the number of tomatoes damaged in 10 days compared to tomatoes from control plants (). A significant fold change in lycopene content in the tomatoes was observed in the 1280 mg/L EHM foliar sprayed plants compared to control plant tomatoes ().
Figure 9. Effect of E. hirta methanol (EHM) extract on marketability of tomato the effect of foliar application of tomato plants with 1280 mg/L EHM on (a) average weight of ten tomatoes, (b) weight distribution of ten tomatoes from each group, (c) number of fruits spoiled in 10 days and (d) lycopene content. Control plants were treated with water while dhanzyme (2 mL/L) served as positive control. The data is obtained from experiments performed three times in triplicates. Data shown reflect the mean ± standard error mean. (*) represent p < 0.05 in comparison to control group.
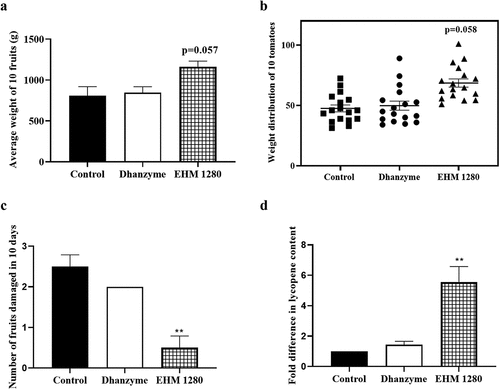
Genes such as ERF1, SlGA2ox1 and LeAGP-1 regulate growth and yield in tomato plants. RNA was isolated from leaves of tomato plants from the control, dhanzyme and 1280 mg/L EHM foliar spray group to study the effect of treatments on mRNA levels of these genes by semi-quantitative RT-PCR method. The ERF1 gene was found to increase upon treatment with 1280 mg/L EHM compared to control plants (). However, 1280 mg/L EHM treatment showed an insignificant increase in SlGA2ox1 gene expression at the mRNA level (), while no changes were observed in LeAGP-1 mRNA levels compared to control plants (). Compared to control plants, plants treated with dhanzyme showed no significant difference in ERF1, SlGA2ox1 and LeAGP-1 mRNA levels.
Figure 10. Effect of E. hirta methanol (EHM) extract on growth and development related genes at mRNA level the effect of foliar application of tomato plants with 1280 mg/L EHM on (a) ERF1 (b) SlGa2ox1 and (c) LeAGP-1 mRNA levels. mRNA was isolated from leaves at the end of the experiment. Control plants were treated with water while dhanzyme (2 mL/L) served as positive control. The data is obtained from three independent experiments. The genes were normalized using housekeeping gene, gapdh. Data shown reflect the mean ± standard error mean. (*) represent p < 0.05 in comparison to control group.
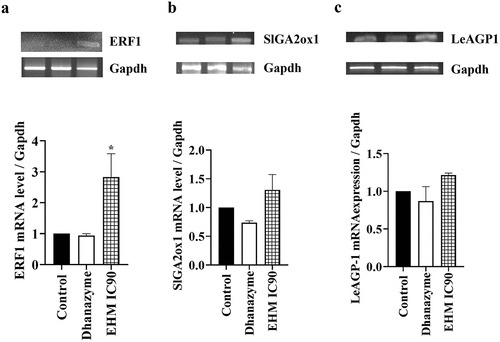
Discussion
Plant extracts exhibit activity either by inhibiting bacterial growth or improving the plant immune system (Yakhin et al., Citation2017). The methanol extract of E. hirta (EHM) was selected for further studies due to its antibacterial activity against both RS and Xav. The methanol extract of E. hirta has been reported to exhibit a wide range of antibacterial activity against Proteus mirabilis, Shigella dysenteriae (Abubakar, Citation2009), Staphylococcus aureus, Bacillus thuringiensis, Bacillus subtilis, Micrococcus sp, Salmonella typhi (Rajeh et al., Citation2010), Escherichia coli, Klebsiella pneumoniae (Abubakar, Citation2009; Rajeh et al., Citation2010), and Pseudomonas aeruginosa (Perumal & Mahmud, Citation2013). Further, methanol extract has the advantage of polarity, enabling the plants to absorb the secondary metabolites more efficiently and exhibit activity (Parekh et al., Citation2005).
EHM at 1280 mg/L concentration exhibited 90% inhibition of RS and Xav growth and therefore referred to as EHM IC90. The antibacterial effect of EHM IC90 was further confirmed by testing its effect on protein degradation. Subsequently, in vivo analysis using Arka Vikas showed that RS infects young plants while Xav infects older plants. RS survives in soil and enters through roots and up the plant’s stem; hence, young seedlings get infected sooner due to quick access to the xylem than the matured plants (Caldwell et al., Citation2017; Singh et al., Citation2018; Xue et al., Citation2020). Xav entry is through stomata and abrasions of young fruits, and hence matured plants having large leaf surface areas are more susceptible to Xav infection (Thieme et al., Citation2005). The plants pre-treated with EHM IC90 indicated moderate resistance to RS and Xav infection. RS and Xav-infected plants pre-treated with EHM IC90 showed a significant increase in the fruit number and weight per plant compared to the control and infected plants in Arka Vikas variety.
Plant growth, functioning and development are characterized by measuring chlorophyll, NO, H2O2 and PAL levels. Chlorophyll is essential for photosynthesis and energy production (Johnson, Citation2016). NO is a ubiquitous gaseous transmitter involved in redox homeostasis that regulates specific checkpoints to control the switch between development and stress of a plant (Sánchez-Vicente et al., Citation2019). H2O2 is a reactive oxygen species (ROS) generated in plants during metabolism and is also an important signalling molecule in plants, which mediates different physiological and biochemical processes. Both NO and H2O2 have been reported to play an essential role in plant development (Niu & Liao, Citation2016). PAL is an enzyme that catalyzes the conversion of L-phenylalanine into trans-cinnamate. It is involved in both plant development and secondary metabolite production to respond to biotic stress (Chang et al., Citation2008). The role of these metabolites in EHM IC90 mediated alleviation of diseases was examined.
Chlorophyll metabolism plays an essential role in regulating defence signalling under various stress conditions and reduces upon infection with a pathogen (Kangasjärvi et al., Citation2012). Rajendran et al. (Citation2016) have reported that the photosynthetic processes depend upon the infection severity. Tomato plants infected with RS increased Chl b content significantly, whereas infection of tomato plants with Xav increased Chl a content and reduced Chl b content. The difference in response to infection by plants could be due to the variance in the mode of infection exhibited by both the bacteria, wherein RS infects systemically, and Xav infects locally. In the current study, RS and Xav-infected plants pre-treated with EHM IC90 increased both Chl b and Chl a levels in tomato plants.
Plants respond to infections by activating several defence mechanisms. Oxidative burst is detected early in response to infection leading to an accumulation of ROS (H2O2 and superoxide) and NO, which induces a hypersensitive response (HR) causing programmed cell death (Mishra et al., Citation2012). ROS production during oxidative burst limits the growth of the invading pathogens through HR responses and triggers the defence process in plants (Kumar et al., Citation2016). PAL enzyme induces defence mechanisms that initiate a series of reactions to form bioactive molecules, which play a vital role in plant resistance toward a pathogen (Mishra et al., Citation2012). However, the pathogens infect the plants by circumventing recognition and causing ambiguity to host pattern recognition receptors by the pathogen-associated molecular patterns (PAMPs) or produce virulent factors to suppress PAMP triggered immunity (PTI) (Kumar et al., Citation2016; Mishra et al., Citation2012).
In the present study, RS infection showed a significant decrease in NO levels, probably because leaves were used for experiments, whereas bacteria are present in the stem. PAL is a primary enzyme in the phenylpropanoid pathway and is involved in synthesizing phenols and lignin. Increased PAL activity has been associated with disease resistance (Vanitha et al., Citation2009). Pre-treatment of RS infected plants with EHM IC90 significantly increased PAL levels compared to control and infected plants.
X. euvescatoria suppresses ROS signalling in tomatoes by T3SS effectors such as Xanthomonas outer protein (Xop) X (Stork et al., Citation2015) and Xop B (Priller et al., Citation2016). Similarly, plants infected with Xav showed a significant reduction in H2O2 levels. In addition, these plants also showed an increased PAL activity, with no significant change in NO levels. However, Xav-infected plants pre-treated with EHM IC90 displayed a significant decrease in PAL activity. They increased NO levels, with no significant change in H2O2 levels compared to infected plants. The increased PAL activity may be responsible for the moderate resistance exhibited by EHM IC90 treatments during RS infection in plants (Agarwal et al., Citation2016). However, Xav-infected plants pre-treated with EHM IC90 decreased PAL activity compared to uninfected plants. The differences in the mechanism of action could be due to variations in their site of infection.
Lycopene is a carotenoid pigment and a natural antioxidant responsible for the characteristic red color of ripe tomatoes (Vanitha et al., Citation2009). Hence, the effect of EHM IC90 on lycopene was evaluated to determine the quality of the fruit. EHM IC90 pre-treatment increased lycopene content in fruits obtained from plants infected with RS and Xav compared to control plants. Fruits obtained from uninfected tomato plants subjected to EHM IC90 foliar spray showed a significant increase in lycopene levels compared to control plant fruits. EHM IC90 treated plants showed significant alleviation of the bacterial diseases, and also showed an increase in tomato number, weight, and lycopene content, indicating its biostimulant potential towards tomato plants.
Biostimulant is a term used to describe any biological substance exhibiting at least one of the following functions in agriculture such as enhancement of nutrition efficiency, increasing abiotic stress tolerance and/or improving quality of the produce in terms of nutritional value, protein content, shelf life, etc. (Du Jardin, Citation2015). As 1280 mg/L reduced the bacterial wilt and spot diseases and increased tomato yield together with lycopene content in Arka Vikas variety of tomato plants, the biostimulant potential of EHM was further evaluated by treating a different variety of tomato plants, Namdhari ‘NS 538’ to confirm that the biostimulant potential of EHM is not specific to a single variety of tomato.
Plants contain several secondary metabolites known to improve the immunity of host plants, increase crop yield, and control diseases (Colla et al., Citation2017). A study by Rouphael et al. (Citation2017) tested the effect of 5.0 mL/L legume-derived protein hydrolysate by foliar applications every 10 days on Akyra and Sir Elyan tomato varieties in Naples, Italy. They observed an increase in the number of fruits in akyra tomato variety and mean fruit weight in Sir Eylan tomato varieties (Rouphael et al., Citation2017). Legume-derived protein hydrolysate (5.0 mL/L) also increased levels of lycopene, antioxidants, mineral content and ascorbic acid (Rouphael et al., Citation2017).
The commercial biostimulants are available as granules, soluble powder or liquid. To improve crop yield, they are either used for seed treatment or applied to plants through foliar spray or soil drench, as suggested by the manufacturer (Colla et al., Citation2015). Hence, Namdhari NS 538 plants were grown in fields and exposed to various concentrations of EHM in different ways to identify the best concentration and method of application beneficial to the farmers. Our study showed that plants exposed to 1280 mg/L EHM (same as EHM IC90) through foliar spray every 15 days until fruiting, increased the fruit number and weight. EHM IC90 foliar sprayed plants increased lycopene content in the fruits and increased the shelf life of the tomatoes by significantly reducing the fruit spoilage.
Further, studies were performed to examine the role of EHM IC90 in regulating genes involved in growth and development. Our study showed an increase in ERF1 gene expression in tomato plants treated with EHM 1280 mg/L. An increase in this gene implies induced ethylene signalling in the plant. This gene is a receptor for ethylene, a plant hormone known to regulate many diverse developmental and physiological processes in plants. Ethylene is involved in every plant life cycle phase, like seed germination, root hair development, root nodulation, flower senescence, abscission, and fruit ripening (Jiroutova et al., Citation2018). The ERF1 gene is influenced by EIN3, a sequence-specific DNA-binding protein that regulates gene expression by binding directly to ethylene response elements (Solano et al., Citation1998). In addition, ERF1 is essential for expressing other downstream genes in the ethylene-induced signalling pathway (Amist & Singh, Citation2022), thereby improving fruiting in the plant. Gibberellins are important hormones that control seed development, growth and fruit set in tomato. SlGA2ox1 gene is expressed to oxidize the bioactive gibberellic acid (GA) or their immediate precursors into their inactive form. SlGA2ox1 gene indirectly effects the growth and development of fruit by reducing the fruit quality of tomato due to lack of or reduction in bioactive GA which causes reduced fruit set and fruit weight leading to improper fruit growth (Chen et al., Citation2016). Reduced GA molecule also causes early ripening (Lin et al., Citation2019). EHM IC90 treated plants have showed no significant increase in SlGA2ox1, indicating that fruit quality remains unaffected. Further, the role of EHM IC90 in the LeAGP-1 gene was analyzed because arabinogalactan proteins (AGPs) are extensively glycosylated hydroxyproline-rich glycol proteins found among plants (Cruz-Valderrama et al., Citation2021). A study has shown that overexpression of the LeAGP-1 gene in tomato plants presented more inflorescence, causing problems in developing floral buds (Lin et al., Citation2019). Due to their role in plant growth and reproduction, the effect of EHM IC90 on LeAGP-1 levels was investigated. Plants treated with EHM 1280 mg/L showed no change in LeAGP-1 gene expression compared to control plants, indicating the observed increase in tomato fruit yield in plants sprayed with EHM 1280 mg/L.
To conclude, E. hirta methanol extract (1280 mg/L) has been found to potentially increase plant resistance to bacterial spot and wilt diseases, and improve tomato fruit yield, shelf life and lycopene content. 1280 mg/L EHM extract can be an easy to use, cost-effective and eco-friendly alternative to chemical stimulants for farmers to manage bacterial diseases and increase tomato yield.
PPS2022_049RP-File012.tif
Download TIFF Image (147.6 KB)Acknowledgment
We would like to thank Professor. S. Umesha, Department of Studies in Biotechnology, University of Mysore, India, for providing us RS and Xav strains for conducting our research work. We would also like to thank Dr. N. Mathivanan for his scientific advice and providing access to the greenhouse facility at Centre for Advanced Studies in Botany, University of Madras.
Disclosure statement
No potential conflict of interest was reported by the author(s).
Data availability statement
The produced datasets of the present study are accessible from the corresponding author on request.
Supplementary material
Supplemental data for this article can be accessed online at https://doi.org/10.1080/1343943X.2022.2156892
Additional information
Funding
References
- AAPFCO. (2012). Product label guide. Association of American Plant Food Control Officials. http://agr.mt.gov/agr/Programs/Pesticides/PDFs/AAPFCO_Labeling_Guide_2012.pdf
- Abubakar, E. M. M. (2009). Antibacterial activity of crude extracts of Euphorbia hirta against some bacteria associated with enteric infections. Journal of Medicinal Plants Research, 3(7), 498–505. https://academicjournals.org/journal/JMPR/article-full-text-pdf/C84129214592
- Adejo, G. O., Agbali, F. A., & Otokpa, O. S. (2015). Antioxidant, total lycopene, ascorbic acid and microbial load estimation in powdered tomato varieties sold in dutsin-ma market. Open Access Library Journal, 2(108), 1–7. https://www.scirp.org/html/68576_68576.htm
- Agarwal, P., Patel, K., Das, A. K., Ghosh, A., & Agarwal, P. K. (2016). Insights into the role of seaweed Kappaphycus alvarezii sap towards phytohormone signalling and regulating defence responsive genes in Lycopersicon esculentum. Journal of Applied Phycology, 28(4), 2529–2537. https://link.springer.com/article/10.1007/s10811-015-0784-1
- Ahanger, M. A., Mir, R. A., Alyemeni, M. N., & Ahmad, P. (2020). Combined effects of brassinosteroid and kinetin mitigates salinity stress in tomato through the modulation of antioxidant and osmolyte metabolism. Plant Physiology and Biochemistry, 147, 31–42. https://doi.org/10.1016/j.plaphy.2019.12.007
- Ahmad, W., Singh, S., & Kumar, S. (2017). Phytochemical screening and antimicrobial study of Euphorbia hirta extracts. Journal of Medicinal Plants Studies, 5, 183–186. https://doi.org/10.4103/2229-516X.117082
- Al-Snafi, A. E. (2017). Pharmacology and therapeutic potential of Euphorbia hirta (syn: Euphorbia pilulifera)-A review. IOSR Journal of Pharmacy (IOSRPHR), 7(3), 7–20. http://www.iosrphr.org/papers/v7i3V1/B0703010720.pdf
- Amist, N., & Singh, N. B. (2022). Ethylene and phytohormone crosstalk in plant defense against abiotic stress. Ethylene in Plant Biology, 277–290. https://doi.org/10.1002/9781119744719.ch12
- Angelika, G. P., & Suprihadi, A. (2014). Uji aktivitas antibakteri ekstrak tumbuhan Euphorbia hirta L. terhadap ralstonia solanacearum, Escherichia coli, dan staphylococcus aureus secara in vitro. Jurnal Akademika Biologi, 3(2), 49–58. https://ejournal3.undip.ac.id/index.php/biologi/article/view/19444
- Atif, M., Ilavenil, S., Devanesan, S., AlSalhi, M. S., Choi, K. C., Vijayaraghavan, P., Alfuraydi, A. A., & Alanazi, N. F. (2020). Essential oils of two medicinal plants and protective properties of jack fruits against the spoilage bacteria and fungi. Industrial Crops and Products, 147, 112239. https://doi.org/10.1016/j.indcrop.2020.112239
- Awad, D., & Brueck, T. (2020). Optimization of protein isolation by proteomic qualification from Cutaneotrichosporon oleaginosus. Analytical and Bioanalytical Chemistry, 412(2), 449–462. https://doi.org/10.1007/s00216-019-02254-7
- Balestra, G. M., Heydari, A., Ceccarelli, D., Ovidi, E., & Quattrucci, A. (2009). Antibacterial effect of allium sativum and ficus carica extracts on tomato bacterial pathogens. Crop Protection, 28(10), 807–811. https://doi.org/10.1016/j.cropro.2009.06.004
- Caldwell, D., Kim, B. S., & Iyer-Pascuzzi, A. S. (2017). Ralstonia solanacearum differentially colonizes roots of resistant and susceptible tomato plants. Phytopathology, 107(5), 528–536. https://doi.org/10.1094/PHYTO-09-16-0353-R
- Chang, A., Lim, M. H., Lee, S. W., Robb, E., & Nazar, R. N. (2008). Tomato phenylalanine ammonia-lyase gene family, highly redundant but strongly underutilized. The Journal of Biological Chemistry, 283(48), 33591–33601. https://doi.org/10.1074/jbc.M804428200
- Chen, S., Wang, X., Zhang, L., Lin, S., Liu, D., Wang, Q., Cai, S., El-Tanbouly, R., Gan, L., Wu, H., & Li, Y. (2016). Identification and characterization of tomato gibberellin 2-oxidases (Ga2oxs) and effects of fruit-specific SlGa2ox1 overexpression on fruit and seed growth and development. Horticulture research, 3(1), 3. https://doi.org/10.1038/hortres.2016.59
- Chomczynski, P., & Sacchi, N. (1987). Single-step method of RNA isolation by acid guanidinium thiocyanate-phenol-chloroform extraction. Analytical Biochemistry, 162(1), 156–159. https://doi.org/10.1016/0003-2697(87)90021-2
- Colla, G., Cardarelli, M., Bonini, P., & Rouphael, Y. (2017). Foliar applications of protein hydrolysate, plant and seaweed extracts increase yield but differentially modulate fruit quality of greenhouse tomato. HortScience, 52(9), 1214–1220. https://doi.org/10.21273/HORTSCI12200-17
- Colla, G., Nardi, S., Cardarelli, M., Ertani, A., Lucini, L., Canaguier, R., & Rouphael, Y. (2015). Protein hydrolysates as biostimulants in horticulture. Scientia horticulturae, 196, 28–38. https://doi.org/10.1016/j.scienta.2015.08.037
- Cruz-Valderrama, J. E., Bernal-Gallardo, J. J., Herrera-Ubaldo, H., & de Folter, S. (2021). Building a flower: The influence of cell wall composition on flower development and reproduction. Genes, 12(7), 978. https://doi.org/10.3390/genes12070978
- Du Jardin, P. (2015). Plant biostimulants: Definition, concept, main categories and regulation. Scientia horticulturae, 196, 3–14. https://doi.org/10.1016/j.scienta.2015.09.021
- Henry, E., Toruño, T. Y., Jauneau, A., Deslandes, L., & Coaker, G. (2017). Direct and indirect visualization of bacterial effector delivery into diverse plant cell types during infection. The Plant Cell, 29(7), 1555–1570. https://doi.org/10.1105/tpc.17.00027
- Horsfall, J. G. (1945). An improved grading system for measuring plant diseases. Phytopathology, 35, 655.
- Im, S. M., Yu, N. H., Joen, H. W., Kim, S. O., Park, H. W., Park, A. R., & Kim, J. C. (2020). Biological control of tomato bacterial wilt by oxydifficidin and difficidin-producing bacillus methylotrophicus DR-08. Pesticide Biochemistry and Physiology, 163, 130–137. https://doi.org/10.1016/j.pestbp.2019.11.007
- Jiroutova, P., Oklestkova, J., & Strnad, M. (2018). Crosstalk between brassinosteroids and ethylene during plant growth and under abiotic stress conditions. International Journal of Molecular Sciences, 19(10), 3283. https://doi.org/10.3390/ijms19103283
- Johnson, M. P. (2016). An overview of photosynthesis. Essays in biochemistry, 60(3), 255–273. https://doi.org/10.1042/EBC20160016
- Kabir, F., Gulfraz, M., Raja, G. K., Inam-Ul-Haq, M., Ahmad, M. S., Nasir, M. F., Awais, M., & Batool, I. (2018). Nutrients utilization and biomass production by microalgae culture development in wastewater. International Journal of Biosciences, 12, 460–469. http://dx.doi.org/10.12692/ijb/12.6.460-469
- Kangasjärvi, S., Neukermans, J., Li, S., Aro, E. M., & Noctor, G. (2012). Photosynthesis, photorespiration, and light signalling in defence responses. Journal of Experimental Botany, 63(4), 1619–1636. https://doi.org/10.1093/jxb/err402
- Kelman, A. (1954). The relationship of pathogenicity in pseudomonas solanacearum to colony appearance on a tetrazolium medium. Phytopathology, 44(12), 693–695. https://www.cabdirect.org/cabdirect/abstract/19551101405
- Klančnik, A., Piskernik, S., Jeršek, B., & Možina, S. S. (2010). Evaluation of diffusion and dilution methods to determine the antibacterial activity of plant extracts. Journal of Microbiological Methods, 81(2), 121–126. https://doi.org/10.1016/j.mimet.2010.02.004
- Koike, M., & Shimada, T. (1992). Alfalfa-verticilliumalbo-atrum interactions II. In vitro peroxidase and phenylalanine ammonia-lyase activities enhanced by treatment with fungal elicitors. Plant Tissue Culture Letters, 9(2), 81–85. https://doi.org/10.5511/plantbiotechnology1984.9.81
- Kumar, S., Malhotra, R., & Kumar, D. (2010). Euphorbia hirta: Its chemistry, traditional and medicinal uses, and pharmacological activities. Pharmacognosy Reviews, 4(7), 58. https://doi.org/10.4103/0973-7847.65327
- Kumar, R., Soni, M., & Mondal, K. K. (2016). XopN-T3SS effector of Xanthomonas axonopodis pv. punicae localizes to the plasma membrane and modulates ROS accumulation events during blight pathogenesis in pomegranate. Microbiological research, 193, 111–120. https://doi.org/10.1016/j.micres.2016.10.001
- Lin, S., Huang, L., Miao, Y., Yu, Y., Peng, R., & Cao, J. (2019). Constitutive overexpression of the classical arabinogalactan protein gene BcMF18 in arabidopsis causes defects in pollen intine morphogenesis. Plant Growth Regulation, 88(2), 159–171. https://doi.org/10.1007/s10725-019-00496-0
- Mishra, A. K., Sharma, K., & Misra, R. S. (2012). Elicitor recognition, signal transduction and induced resistance in plants. Journal of Plant Interaction, 7(2), 95–120. https://doi.org/10.1080/17429145.2011.597517
- Mitrev, S., & Kovačević, B. (2006). Characterization of Xanthomonas axonopodis pv. vesicatoria isolated from peppers in macedonia. Journal of Plant Pathology, 88(3), 321–324. https://www.jstor.org/stable/41998338
- Nion, Y. A., & Toyota, K. (2015). Recent trends in control methods for bacterial wilt diseases caused by Ralstonia solanacearum. Microbes and Environments, 30(1), ME14144. https://doi.org/10.1264/jsme2.ME14144
- Niu, L., & Liao, W. (2016). Hydrogen peroxide signaling in plant development and abiotic responses: Crosstalk with nitric oxide and calcium. Frontiers in plant science, 7, 230. https://doi.org/10.3389/fpls.2016.00230
- Parađiković, N., Teklić, T., Zeljković, S., Lisjak, M., & Špoljarević, M. (2018). Biostimulants research in some horticultural plant species—a review. Food and Energy Security, 8(2), e00162. https://doi.org/10.1002/fes3.162
- Parekh, J., Jadeja, D., & Chanda, S. (2005). Efficacy of aqueous and methanol extracts of some medicinal plants for potential antibacterial activity. Turkish Journal of Biology, 29(4), 203–210. https://journals.tubitak.gov.tr/biology/vol29/iss4/3/
- Perumal, S., & Mahmud, R. (2013). Chemical analysis, inhibition of biofilm formation and biofilm eradication potential of Euphorbia hirta L. against clinical isolates and standard strains. BMC Complementary and Alternative Medicine, 13(1), 1–8. https://doi.org/10.1186/1472-6882-13-346
- Potnis, N., Timilsina, S., Strayer, A., Shantharaj, D., Barak, J. D., Paret, M. L., Vallad, G. E., & Jones, J. B. (2015). Bacterial spot of tomato and pepper: Diverse X anthomonas species with a wide variety of virulence factors posing a worldwide challenge. Molecular Plant Pathology, 16(9), 907–920. https://doi.org/10.1111/mpp.12244
- Priller, J. P. R., Reid, S., Konein, P., Dietrich, P., Sonnewald, S., & Davis, K. R. (2016). The Xanthomonas campestris pv. vesicatoria type-3 effector XopB inhibits plant defence responses by interfering with ROS production. Plos One, 11(7), e0159107. https://doi.org/10.1371/journal.pone.0159107
- Rajeh, M. A. B., Zuraini, Z., Sasidharan, S., Latha, L. Y., & Amutha, S. (2010). Assessment of Euphorbia hirta L. leaf, flower, stem and root extracts for their antibacterial and antifungal activity and brine shrimp lethality. Molecules, 15(9), 6008–6018. https://doi.org/10.3390/molecules15096008
- Rajendran, D. K., Park, E., Nagendran, R., Hung, N. B., Cho, B. K., Kim, K. H., & Lee, Y. H. (2016). Visual analysis for detection and quantification of pseudomonas cichorii disease severity in tomato plants. The Plant Pathology Journal, 32(4), 300. https://doi.org/10.5423/2FPPJ.OA.01.2016.0032
- Robinson, R. K. (2014). Chapter: Xanthomonas vesicatoria. Encyclopedia of food microbiology. Academic press.
- Rouphael, Y., Cardarelli, M., Bonini, P., & Colla, G. (2017). Synergistic action of a microbial-based biostimulant and a plant derived-protein hydrolysate enhances lettuce tolerance to alkalinity and salinity. Frontiers in plant science, 8, 131. https://doi.org/10.3389/fpls.2017.00131
- Sánchez-Vicente, I., Fernández-Espinosa, M. G., Lorenzo, O., & Brouquisse, R. (2019). Nitric oxide molecular targets: Reprogramming plant development upon stress. Journal of Experimental Botany, 70(17), 4441–4460. https://doi.org/10.1093/jxb/erz339
- Saranya, N., Devi, P., Nithiyanantham, S., & Jeyalaxmi, R. (2014). Cells disruption by ultrasonication. BioNanoScience, 4(4), 335–337. https://doi.org/10.1007/s12668-014-0149-2
- Sheikh, M., Malik, A. R., Meghavanshi, M. K., & Mahmood, I. (2012). Studies on some plant extracts for their antimicrobial potential against certain pathogenic microorganisms. American Journal of Plant Sciences, 3(2), 209. https://doi.org/10.4236/ajps.2012.32025
- Shilpa, K., & Lakshmi, B. S. (2019). Influence of exogenous cinnamic acid on the production of chlorogenic acid in cichorium intybus L cell culture. South African Journal of Botany, 125, 527–532. https://doi.org/10.1016/j.sajb.2019.01.004
- Singh, N., Phukan, T., Sharma, P. L., Kabyashree, K., Barman, A., Kumar, R., Sonti, R. V., Genin, S., & Ray, S. K. (2018). An innovative root inoculation method to study ralstonia solanacearum pathogenicity in tomato seedlings. Phytopathology, 108(4), 436–442. https://doi.org/10.1094/PHYTO-08-17-0291-R
- Solano, R., Stepanova, A., Chao, Q., & Ecker, J. R. (1998). Nuclear events in ethylene signaling: A transcriptional cascade mediated by ETHYLENE-INSENSITIVE3 and ETHYLENE-RESPONSE-FACTOR1. Genes & Development, 12(23), 3703–3714. https://doi.org/10.1101/gad.12.23.3703
- Stork, W., Kim, J. G., & Mudgett, M. B. (2015). Functional analysis of plant defense suppression and activation by the xanthomonas core type III effector XopX. Molecular Plant-Microbe Interactions, 28(2), 180–194. https://doi.org/10.1094/MPMI-09-14-0263-R
- Thieme, F., Koebnik, R., Bekel, T., Berger, C., Boch, J., Büttner, D., Caldana, C., Gaigalat, L., Goesmann, A., Kay, S., Kirchner, O., Lanz, C., Linke, B., McHardy, A. C., Meyer, F., Mittenhuber, G., Nies, D. H., Niesbach-Klösgen, U., Patschkowski, T. , and Kaiser, O. (2005). Insights into genome plasticity and pathogenicity of the plant pathogenic bacterium Xanthomonas campestris pv. vesicatoria revealed by the complete genome sequence. Journal of Bacteriology, 187(21), 7254–7266. https://doi.org/10.1128/JB.187.21.7254-7266.2005
- Vanitha, S. C., Niranjana, S. R., & Umesha, S. (2009). Role of phenylalanine ammonia lyase and polyphenol oxidase in host resistance to bacterial wilt of tomato. Journal of Phytopathology, 157(9), 552–557. https://doi.org/10.1111/j.1439-0434.2008.01526.x
- Vu, T. T., Kim, H., Tran, V. K., Vu, H. D., Hoang, T. X., Han, J. W., Choi, Y. H., Jang, K. S., Choi, G. J., Kim, J. C., & Gupta, V. (2017). Antibacterial activity of tannins isolated from Sapium baccatum extract and use for control of tomato bacterial wilt. Plos One, 12(7), e0181499. https://doi.org/10.1371/journal.pone.0181499
- Xue, H., Lozano-Durán, R., & Macho, A. P. (2020). Insights into the root invasion by the plant pathogenic bacterium Ralstonia solanacearum. Plants (Basel, Switzerland), 9(4), 516. https://doi.org/10.3390/plants9040516
- Yakhin, O. I., Lubyanov, A. A., Yakhin, I. A., & Brown, P. H. (2017). Biostimulants in plant science: A global perspective. Frontiers in plant science, 7, 2049. https://doi.org/10.3389/fpls.2016.02049
- Zheng, X., Zhu, Y., Liu, B., Lin, N., & Zheng, D. (2017). Invasive properties of Ralstonia solanacearum virulent and avirulent strains in tomato roots. Microbial Pathogenesis, 113, 144–151. https://doi.org/10.1016/j.micpath.2017.10.046