ABSTRACT
A limited amount of visual information is retained between saccades, which is subsequently stored into a memory system, such as transsaccadic memory. Since the capacity of transsaccadic memory is limited, selection of information is crucial. Selection of relevant information is modulated by attentional processes such as the presaccadic shift of attention. This involuntary shift of attention occurs prior to execution of the saccade and leads to information acquisition at an intended saccade target. The aim of the present study was to investigate the influence that another attentional effect, inhibition of return (IOR), has on the information that gets stored into transsaccadic memory. IOR is the phenomenon where participants are slower to respond to a cue at a previously attended location. To this end, we used a transsaccadic memory paradigm in which stimuli, oriented on a horizontal axis relative to saccade direction, are only visible to the participant before executing a saccade. Previous research showed that items in close proximity to a saccade target are likely to be reported more accurately. In our current study, participants were cued to fixate one of the stimulus locations and subsequently refixated the centre fixation point before executing the transsaccadic memory task. Results indicate that information at a location near a saccade landing point is less likely to be acquired into transsaccadic memory when this location was previously associated with IOR. Furthermore, we found evidence which implicates a reduction of the overall amount of elements retained in transsaccadic memory when a location near a saccade target is associated with IOR. These results suggest that the presaccadic shift of attention may be modulated by IOR and thereby reduces information acquisition by transsaccadic memory.
The way our visual system deals with the challenge of processing visual information across eye movements (known as saccades) is both complex and fascinating. While viewing a scene, the eyes saccade about three times per second to foveate specific parts of the scene for high acuity processing (Hollingworth & Henderson, Citation1998). One of the challenges of integrating visual information is the inability of the visual system to process all information simultaneously (Wolfe, Citation1994). Selection of visual information is therefore crucial as we are only able to attend to a small amount of information at any given time and unattended information is lost (Wolfe, Reinecke, & Brawn, Citation2006). Loss of information can occur during different stages of visual processing and is especially true whilst executing a saccade. Between saccades a limited amount of objects are retained in a memory trace, known as transsaccadic memory (Bays & Husain, Citation2008; Irwin, Citation1991; Irwin & Gordon, Citation1998; Prime, Tsotsos, Keith, & Crawford, Citation2007).
The crucial factor to select relevant information for storage and subsequently store this information is visual attention (Irwin & Gordon, Citation1998; Prime et al., Citation2007). Johnson et al. (Citation2008) concluded that visual attention plays a general role in both maintaining feature memory and binding features into representations of objects. After being selected, visual information is acquired in a memory storage, such as transsaccadic memory. Transsaccadic memory can be loaded as a result from selection by endogenously orientated attention and by automatic, exogenous attention. For instance, before executing an eye movement attention is shifted to the intended location of the eye movement (Deubel & Schneider, Citation1996; Hoffman & Subramaniam, Citation1995). This presaccadic shift of attention is thought to be involuntary and mandatory. Information at the intended target location of an eye movement is attended before the movement is executed, due to the presaccadic shift of attention (Deubel & Schneider, Citation1996; Irwin & Gordon, Citation1998). How the presaccadic shift of attention affects transsaccadic memory has been explored in the paradigm used by Irwin and Andrews (Citation1996). In this paradigm a stimulus array is presented before executing an eye movement. As the eye movement is cued, and subsequently executed, the stimulus is removed. Results showed that the information in near proximity to the intended saccade target is better acquired than information away from a saccade target, due to the presaccadic shift of attention.
Besides the presaccadic shift of attention, other attentional processes may modulate information acquisition by transsaccadic memory, yet these factors still remain unknown. One particular candidate is inhibition of return (IOR; see Posner & Cohen, Citation1984). IOR has been described as an attentional effect where a slowed reaction time is observed to information at a previously attended location (Pratt, Hillis, & Gold, Citation2001). In the IOR paradigm by Posner and Cohen (Citation1984) the participants are presented with a cue, followed by a target to which the participant responds. The presented cue is uninformative and draws attention by its onset (exogenous cue). When the interval between cue presentation and target is short, a facilitation of reaction time is observed. However, when this interval is relatively long a slowed reaction time is observed, resulting from attentional disengagement (Castel, Pratt, & Craik, Citation2003; Posner & Cohen, Citation1984).
Other than reaction time, IOR may also affect sensory-perceptual processes and thereby influence performance on certain discrimination tasks (Reuter-Lorenz, Jha, & Rosenquist, Citation1996). Research has indicated a reduced ability for human observers to discriminate contrast differences at a location associated with IOR (Sapir, Jackson, Butler, Paul, & Abrams, Citation2013). Yet, it is currently unclear how IOR modulates information acquisition across saccades by transsaccadic memory. Converging evidence suggests that IOR is closely related to the oculomotor system and that IOR and oculomotor activation are integrally linked together (Hilchey, Klein, & Satel, Citation2014). As acquisition of information into transsaccadic memory is dependent on attentional processes such as the presaccadic shift of attention, we inferred that transsaccadic memory and IOR may also interact at a target discrimination level.
The aim of the current study was to investigate how information acquisition across saccades is modulated by IOR. Under normal circumstances, information in the near proximity of a saccade target is typically acquired into transsaccadic memory. We hypothesize that when information is located near a saccade target at a location that is associated with IOR, that this information would be acquired into transsaccadic memory to a lesser extent. As a consequence, information at that particular location should be reported less accurately. To test this hypothesis we used an adapted version of the original transsaccadic memory paradigm by Irwin and Gordon (Citation1998) where one of the stimulus locations was previously fixated by the participants. This paradigm allowed us to investigate how IOR influences the overall amount of items acquired in transsaccadic memory. If only the information that appears at a saccade goal is acquired due to presaccadic shift of attention and IOR would reduce information acquisition we should observe a reduction in overall number of items acquired by transsaccadic memory. However, if observers have the ability to compensate for this proposed loss of information, by more accurately acquiring stimuli further away from the saccade target, the hypothesized effect of IOR would not decrease overall transsaccadic memory capacity.
Experiment 1
Method
Participants
Fourteen participants, 10 female, aged 19 to 35 (M = 23.3) from the Utrecht University community participated for monetary compensation of 6 Euros per hour. Participants completed 500 trials in a two and a half hour session. All participants reported normal or corrected-to-normal vision and were naïve to the purpose of the study. Written informed consent was obtained from all participants. The study was reviewed and approved by the Faculty Research Ethics Committee (FETC) of the University of Utrecht.
Stimuli and apparatus
Stimuli were white letters (font height 1° visual angle) on a black background. The letters used were drawn from a subset of 12 letters (A, S, D, F, G, H, J, K, L, V, B and N) with no repeating letters present in one trial. Fixation dots and probes were presented as white circles of 0.6° with a centred black circle of 0.1°. The fixation dot was placed in the centre of the screen. Stimuli and probes could appear at 10 locations, placed on two imaginary horizontal axes (3° upwards and downwards from fixation; ). The stimuli and probes were evenly spaced along these horizontal axes subtending 4° left and right from fixation. During the experiment two saccade targets appeared along the horizontal axis on the same height as the fixation target, also subtending 4° left and right.
Figure 1. The possible locations of the stimuli used in Experiment 1 and Experiment 2. The dashed circles are placeholders and were not presented during the experiment. The figure presented here is not drawn to scale and is shown in inverted contrast.
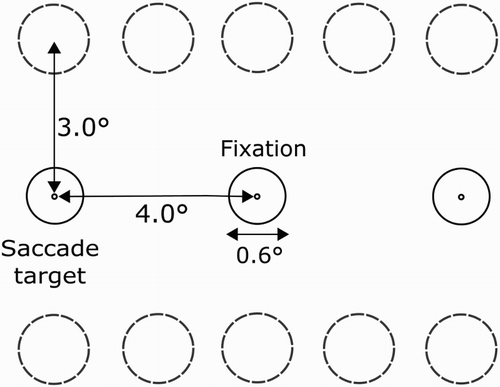
The experiment was performed in a darkened room. Stimuli were presented on a LG 24MB65PM LCD monitor with a spatial resolution of 1280 by 800 pixels and a refresh rate of 60 Hz. The screen size was 50.8 cm wide and 33.9 cm high. The participant was seated 70 cm from the monitor. Participants placed their heads on a desk-mounted chin rest to reduce head movement. Eye movements were tracked with an Eyelink 1000 (SR Research Ltd., Canada) sampling at 1000 Hz. All participants were calibrated using a 9-point calibration procedure. For offline saccade detection the SR research algorithm was used, where an eye movement was classified as a saccade when the movement velocity exceeded 35°/s or when the movement acceleration was greater than 9500°/s2. The left eye was tracked for all participants.
The paradigm was programmed in Python 2.7. The Pygaze library was used to connect to the eye tracker using the native Pygaze event detection to detect saccades online (Dalmaijer, Mathôt, & Van der Stigchel, Citation2013). Eyetracker data files were analyzed with Python 2.7 and R 3.1.3 was used for statistical procedures (Ihaka & Gentleman, Citation1996). The lme4 R package was used for statistical analyses (Kuznetsova, Brockhoff, & Christensen, Citation2013).
Procedure
The experiment was divided into 10 blocks of 50 trials. Prior to starting the experiment the eyetracker was calibrated using a standard 9-point calibration procedure. After that a practice block of 15 trials was initiated. If the participant did not perform correct saccades (within 2° of the saccade targets) on at least five trials the practice block was repeated (one participant). Practice trials were identical to regular trials. Before every trial a drift check was performed. If drift was greater than 1.5° visual angle, the eye tracker was recalibrated.
The procedure of the experiment is depicted in . Participants fixated on a central stimulus for 750, 1000 or 1250 ms. The variable interval was used to prevent pre-emptive saccades. A probe would then appear at one of 10 locations. This location will be referred to as the onset location. Participants were instructed to make a saccade to this stimulus as quickly as they could in response to its onset. When the stimulus disappeared, participants had 500 ms to make a saccade back to the fixation point. After this, the letter stimulus array and the saccade targets appeared. After a variable interval of 750 to 1250 ms a tone was played for 400 ms, alerting participants to initiate a saccade. In half of the trials this was a low tone of 200 Hz, instructing a leftward saccade. In the other half the tone was a high tone of 1000 Hz, instructing a rightward saccade. When the saccade was completed, a period of 500 ms passed, after which a target probe briefly appeared at one of the 10 locations for 100 ms. The location of this probe will be referred to as the answer location. Participants then typed in their answer by keyboard. Responses were shown on screen and could be changed until participants confirmed their answer by pressing the Enter key or the Space bar. The subset of letters was chosen due to the location of the letters on the keyboard, which eased the difficulty of typing in the dark. To further facilitate this, participants received feedback on their performance at the end of every block of trials. To reduce fatigue in participants, at the end of every block of trials the participants had the opportunity to take a short break. We also gave the participants a 10 minute break outside of the lab when they were halfway through the experiment.
Figure 2. Sequence of events as they appeared in Experiment 1. Dashed lines with arrows indicate eye movement and the eye graphic indicates eye position. The tone played is either a high tone, indicating a saccade to the right, or a low tone, indicating a saccade to the left. The panels in the figure are presented in inverted contrast and are not drawn to scale.
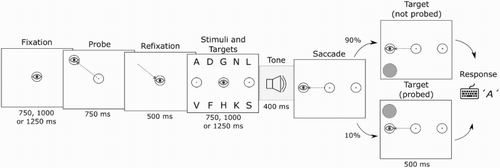
Combinations of onset location and answer location were counterbalanced per participant and appeared equally often, but in quasi-random order. Thus, there was a 10% chance that the answer location and the onset location were the same. Participants were informed that probes were equally likely to appear at any location. The location of the onset was therefore completely uninformative of the location of the answer. Right and leftward saccades were also counterbalanced with the combination of onset and answer locations. Lastly, the stimuli could not appear more than twice in succession at the same location. Locations of onset and answers were re-randomized if an experiment was generated in which stimuli did appear more than twice at the same location in succession.
To determine whether IOR would be induced at the onset location, as expressed in prolonged reaction times, we performed a pilot study with a different set of participants. The same locations, timing and stimuli were used as in Experiment 1. One saccade was made to an onset location and subsequently back to fixation. After this, the stimulus array appeared with one target letter highlighted. The letter was highlighted using a grey circle (2° diameter). Participants were encouraged to type a letter as quickly as possible. A slowed response of 30.6 ms (SD = 125.6 ms) was observed for stimuli that were previously cued, F(1, 11) = 5.09, p < .05. We therefore concluded that IOR, in terms of prolonged reaction times, could be induced with the onset cues used in this paradigm.
Data analysis
Analyses and associated data are available online (Schut, Citation2016). Trials were excluded on the basis of any saccades missing their target by more than 2° or by participants not returning to the central fixation dot after fixating the onset position. Trials were executed on the basis of saccade latencies using a recursive trimming procedure (Van Selst & Jolicoeur, Citation1994). Saccade latencies deviating more than 2.5 SD within the participant’s median latency were excluded. Two participants were excluded from further analysis due to not meeting these criteria on more than 50% of their trials. Of the remaining participants, 14.2% of the trials were excluded due to saccades not landing within 2° of a saccade target, either the cued location, saccade back to fixation or to saccade target. Another 7.5% of the trials were excluded on the basis of saccade latencies.
Answer locations were collapsed vertically to analyze the effect of the location of the onset relative to the saccade direction. This collapsing was performed after tagging the trials as either onset and answer congruent or incongruent trials, to ensure that only the trials in which the participants fixated the exact same location as where the answer appeared were tagged as target congruent trials. By collapsing the positions of the answer in relation to the saccade target, two stimuli appearing on the same vertical axis were encoded as one answer location to a total of five answer locations. The locations were encoded relative to the saccade target: Location 1 being the two answer stimuli appearing above and below the saccade target, in equal steps up to Location 5 (the two stimuli furthest away from the saccade target). The performance is expressed as a proportion of correct answers.
Statistical analyses included a repeated measures analysis of variance and a generalized linear mixed (GLM) model. The repeated measures analysis of variance used the mean proportion of answers correct as dependent variable. Independent variables were location of the answer (five locations, from closest to saccade target to furthest from saccade target) and whether the location of the answer probe had been previously fixated (either true or false). A significance criterion of p < .05 was used for these analyses, additionally effect sizes are reported as eta squared (η2). Post-hoc tests consisted of Holm-Bonferroni corrected paired t-tests, Cohen’s d is reported as a measure of effect size for post-hoc analyses.
GLM analyses allowed us to examine the fit of the participant data to the model, as analyzing proportions per participant may be less informative for the small amount (10) of critical trials per participant in this experiment (Bell et al., Citation2010; Kenward, Roger, Process, Lodge, & Ox, Citation2013; Lindstrom & Bates, Citation1990). GLM models may be a stronger approach in examining participant variance than using proportions, as trial by trial data is not collapsed in a GLM (like in traditional statistics), but added as a random effect to the model. As a result, GLM is less sensitive to unbalanced data than a type 3 sum of squares analysis of variance (Cnaan, Laird, & Slasor, Citation2014; Lindstrom & Bates, Citation1990). The GLM model included correct answers per trial as dependent variable, with location of answer probe as a second degree polynomial and answer probe appearing at previously fixated location as fixed effects. The second degree polynomial was added as the response pattern in the task used typically shows a U shape, where end-point locations are reported better than central locations (Irwin & Gordon, Citation1998). A random intercept per participant was added to the model, to account for variance per observer. Significance of fixed effects were tested by a z test of which the p values are reported. Lastly, the model was compared to a null GLM model, which excluded any interaction between fixed effects. A chi square test was used to test the two models against each other and Bayesian Information Criteria (BIC) were reported for both models.
As saccades are somewhat inaccurate (Coëffé & O’Regan, Citation1987; Cohen & Ross, Citation1978) and foveated points thus differ between trials, we controlled for saccade landing and starting position. It may be possible that these properties that differ between trials would bias which information is acquired. To this end, we added the deviation from the perfect starting and landing position (with regards to the saccade that was cued by tone to a saccade target) as fixed effects to the GLM model to test their predictive qualities. Saccade latencies were similarly controlled for and investigated using a repeated measures analysis of variance.
Results
The main results found are shown in the Data points of . A main effect was found for answer location in relation to saccade target F(4, 44) = 11.84, p < .01, η2 = 0.29. Post-hoc tests exploring the main effect for location show that participants were more accurate at the location closest to the saccade target (Location 1: M = 0.49, SD = 0.50) as compared to locations in the middle of the array: Location 2 (M = 0.19, SD = 0.39), Location 3 (M = 0.17, SD = 0.38) and Location 4 (M = 0.21, SD = 0.41), p < .01, 0.67 < d ≤ 0.72. Participants were more accurate at the location furthest from the saccade target as compared to the locations in the middle of the stimulus array, p < .05, 0.39 < d ≤ 0.44. Importantly, participants were more accurate at Location 1 (the location closest to the saccade target) than at Location 5 (furthest from the saccade target, M = 0.36, SD = 0.48), p < .01, d = 0.27.
Figure 3. Generalized linear mixed model and mean data for mean proportion of answers correct in relation to saccade target between onset appearing at same location as the answer and onset not appearing at the same location as the answer. The shaded region represents the 95% confidence interval for the model, error bars around the data points show standard error. The x axis shows an illustration of where the answer probe appeared. The dashed black line shows performance at chance level (0.08). In the figure the two conditions are horizontally offset as to not overlap visually.
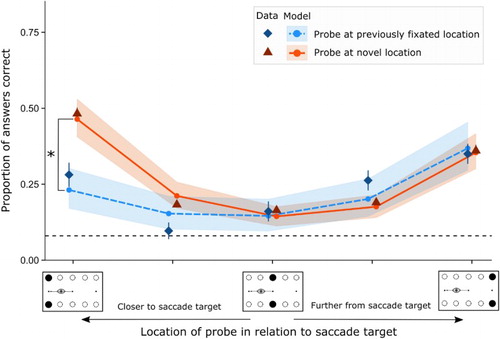
No main effect was found for onset location F(1, 11) = 1.93, p = .19, η2 = 0.02. An interaction effect was present for answer location and onset location F(4, 44) = 6.14, p < .01, η2 = 0.09. Post-hoc tests compared the onset location with each answer location in relation to the saccade target. Visually examining the data (as shown in ) suggests that there is an effect present for the location closest to the saccade target. The interaction effect comparing performance for answer at Location 1 with onset at the same location and answer at Location 1 with onset at a different location was significant (difference in mean proportion = −0.21, p = .04, d = 0.40). Comparisons for the other four locations (e.g., answer at location x, onset at exact same location or at a different location) did not show significance (ranging all ps > .8, .02 < d ≤ 0.17). These results suggest that only the information closest to the saccade target is significantly less acquired by prior orienting to the onset. An additional post-hoc analysis shows that performance at Location 1 is significantly better than at Location 5 in trials where the onset and probe were presented at different locations, p < .01, Cohen’s d = 0.27. In contrast, in trials in which the onset and the probe appeared at the same location there was no significant performance difference between Location 1 and Location 5, p = .18, d = 0.12. Therefore, participants only scored significantly higher at the location closest to the saccade target in trials in which the answer probe was not presented at a previously fixated location.
Data for individual participants is shown in . This figure shows individual scores for the interaction effect we found, which is the location closest to the saccade target. A logistic regression on the participant data suggests that half of the participants show significant reduction in their performance when onset and probe were presented at the same location, p < .05, as shown in . To further quantify the contribution of individual participants a GLM model was fit, the results of which are shown Model points of . This model shows significance for the fixed effects: answer probe at same location as the onset, z = 3.73, p < .01 and probe location in relation to saccade target, z = −7.89, p < .01. The interaction effect between the two fixed factors were similarly significant, z = −2.51, p < .01. We found that this model (BIC = 5435) significantly outperformed a null model (BIC = 5910) in which no interaction effects were present, χ2 = 474.79, p < .01. Thus, the results from this analysis converge with our previous results. Visually inspecting the mean data suggests that the model fits the data well. Analyzing the fit of this model per participant indicated that six out of 12 participants significantly fitted the model (odds ratio > 1.2). Furthermore, investigating the fit of the participants for the location closest to the saccade target reveals that seven out of 12 participants fit the model for this location (odds ratio > 1.2).
Figure 4. Mean proportion of answers correct per participant at the location closest to the saccade target. Each orange and blue bar, separated by ticks at the bottom of the graph, represents one participant. Significant differences between the onset appearing at the location at the onset or a new location are indicated by asterisk for each participant. For visualization purposes, error bars are standard error calculated from a binomial probability function. The dashed black line is performance at chance level (0.08).
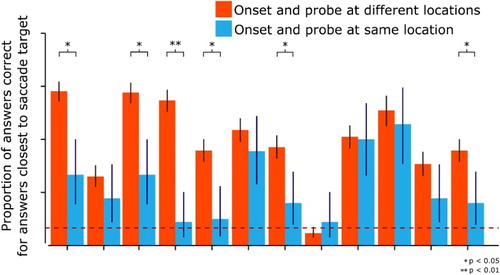
To examine whether saccade starting and landing positions biased the information that was reported in any way we tested our previously constructed GLM model to a model that also included saccade starting and landing position as fixed effects. We found that the GLM model without saccade landing position or starting position (BIC = 5435), as used in the previous analysis, outperformed the models that included saccade landing (BIC = 5890) or saccade starting position (BIC = 5880). Thus, we conclude that the actual starting and landing position of the saccade to a cued saccade target did not affect the ability to report information near that saccade target.
To control for saccade latency we analyzed the effect of onset location on saccade onset. As IOR may affect saccade latency (Briand, Larrison, & Sereno, Citation2000; Klein & MacInnes, Citation1999) it was crucial to exclude a possible interaction between delayed saccades towards a location associated with IOR. No indications of an effect was found for onset location on saccade latency, F(4, 44) = 0.54, p = .48, η2 < 0.01. Median latency for Location 1 was 314 ms with a standard deviation of 328 ms, average latency for the other locations was 325 ms with a standard deviation of 333 ms.
To follow-up on our findings, we investigated whether the reduction of accuracy, when answers and onset appeared in close proximity to the saccade target, would also mean that less elements were retained overall. Our results thus far imply that when the onset appeared near the saccade target a reduction of accuracy is shown for elements that appear at the same location as the onset. Thus, we recoded the onset position, and assumed that this loss of information was always present when the onset appeared near the saccade target. This assumption had to be made due to restrictions in our data set, as only one answer/onset location combination was reported per trial. The onset position was recoded to be either near the saccade target (onset appeared at Location 1) or away from it (onset appeared at Locations 2 to 5). This allowed us to examine whether Locations 2 through 5 received an increase in accuracy from the onset appearing near the saccade target. To analyze the effect of the recoded onset position on accuracy we used a 2 × 5 repeated measures analysis of variance as before. We entered two factors into the analysis: the recoded onset location (2 levels: onset at Location 1 or onset at Location 2 through 5) and location of answer probe (Locations 1 through 5). As before, the analysis showed a main effect for location F(4,44) = 12.41, p < .01, η2 = 0.38. No main effect was observed for the recoded onset probe locations, F(1,11) = 1.03, p = .33, η2 < 0.01. Lastly, no interaction effect was identified between the recoded onset probe locations and the answer locations, F(4,44) = 0.47, p = .90, η2 = 0.01 suggesting that no difference was observed when onset appeared at the location closest to the saccade target or further away from it in terms of overall accuracy for information at Locations 2 to 5. Therefore, locations further from the saccade target received no compensation in information acquisition from the reduction of information acquisition after the onset appeared near the saccade target.
Discussion
In this experiment we studied the association between IOR and transsaccadic memory using an adapted transsaccadic memory paradigm previously used by Irwin and Andrews (Citation1996). We hypothesized that IOR would interact with transsaccadic memory by reducing the information acquisition at a previously cued location. Our results indicate an interaction between IOR and transsaccadic memory: we found a reduction of correct answers for elements associated with IOR compared to elements that were not associated with IOR. This effect was only present for the elements at the closest proximity to the saccade target and was not present for elements further from the saccade target. This indicates that processes prior to the execution of the saccade were affected by IOR which resulted in a reduction of accuracy for elements near a saccade target.
In the current experiment IOR only affected transsaccadic memory for elements in near proximity to a saccade target. To explore how this interaction affects the overall memory capacity of transsaccadic memory we recoded our data relative to the location where the onset appeared. In this manner, we could investigate whether the less accurately reported information, that was previously fixated, was compensated for by higher accuracy for items that were not previously fixated. Our results show that no benefit was observed for the other locations when the information near the saccade target was previously cued and thus less correctly reported. These results suggest that the reduction of accuracy, when the onset and stimuli both appeared at the same location close to the saccade target, did not relocate attentional resources to other locations.
IOR has been associated with increasing in saccade latency when saccading to a previously cued location (Briand et al., Citation2000). For this reason we wanted to examine the possibility that our results could be explained through differences in saccade latencies. IOR being associated with a location within the stimulus array did not cause delays in saccade execution to the saccade targets and does therefore not add any explanatory value to our results. Similarly, we investigated whether information acquisition in the paradigm used influenced by saccade landing and starting position. We found no such effect, which is in line with the studies indicating that the presaccadic shift of attention moves to the intended location of an eye movement, rather than the actual landing position (Deubel & Schneider, Citation1996; Van der Stigchel & De Vries, Citation2015).
We note that in the previous implementations of the paradigm (Irwin, Citation1991; Irwin & Andrews, Citation1996; Irwin & Gordon, Citation1998) the stimuli to be attended to via the presaccadic shift of attention were located in closer proximity than in our current study. Although the distance between the saccade target and the stimuli were increased (from a maximum of 2.2° in the original paradigm to 3.0° in the current paradigm) we found a pattern of results that matched the results found previously (i.e., information near saccade end-points is better reported when blanked during saccadic suppression). This effect is not entirely unexpected, as early research has shown that task demands may dictate the size of the attentional focus (Castiello & Umiltà, Citation1990). Thus, we have shown that the presaccadic shift of attention can at least have an asymmetric spread of 3.0° with the task described in our current study.
In this paradigm, overall target discrimination was best at the location closest to the saccade target. This effect has been previously observed and attributed to the presaccadic shift of attention in the study by Irwin and Andrews (Citation1996). However, the proportion of correct answers on the location furthest away from the saccade target was also better than for stimuli that appeared in the middle of the stimulus array. We suggest that these results might have been due to the design of the paradigm. To reiterate, in the current study the stimulus array was shown after saccading to a cued location. The stimulus array and saccade targets were then visible until a saccade was executed to a saccade target. These saccades were cued with a tone that had a mean onset of 1000 ms after stimulus presentation. The participants may have been pre-emptively attending both saccade targets before the onset of the tone, as the saccade targets were relevant to perform the task correctly. This form of attending in preparation of the tone would explain the increase in performance at locations furthest from saccade target as compared to stimuli that appeared in the middle of the array. Our results show that overall performance was significantly higher at the location closest to the saccade target when compared to the location furthest from the saccade target. This indicates a possible added benefit in memory acquisition of executing the saccade. Interestingly, no effect was present for target discrimination when onset and answer appeared furthest from the saccade target and at the same location. Therefore, we postulate that IOR may affect attentional processes linked to the saccade, such as the presaccadic shift of attention, by disrupting the benefit in information acquisition of executing a saccade to the saccade target.
However, our model of the effect may be supported by the similar properties between the presaccadic shift of attention and exogenously captured attention. IOR has been associated with exogenous cueing (Posner & Cohen, Citation1984), which directs attention automatically. Similarly, the presaccadic shift of attention is drawn involuntarily to a saccade location. The area that the presaccadic shift of attention is directed to has a range that is bigger than the target itself (Deubel & Schneider, Citation1996; Irwin & Andrews, Citation1996; Irwin & Gordon, Citation1998). Through this, the participants were better able to report information not only at the saccade target, but also near a saccade target. The increased performance for stimuli near the saccade target suggests that the location that was cued and subsequently associated with IOR also falls within this range of attention. Research has shown that the size and shape of the attentional window can be modulated and can contain fields in which information is unattended (Castiello & Umiltà, Citation1990; Müller & Hübner, Citation2002). This leads us to postulate that information acquisition in transsaccadic memory may be affected by IOR through inhibition of the presaccadic shift of attention. We do note that IOR is an effect which may be disentangled in a motor and attentional component (Kingstone & Pratt, Citation1999; Martín-Arévalo, Kingstone, & Lupiáñez, Citation2012). In the current study IOR was induced by the participants executing a saccade to a probe, rather than through attentional orienting alone. Although our current design would most likely elicit both attentional and oculomotor IOR, it is possible that the effect found may be specific to IOR induced by executing a saccade to a location.
It should be noted that we base our conclusions on a relative effect that is the combination of two effects. First, we observed an increase in performance near the saccade target relative to the performance furthest from the saccade target, which may be attributed to presaccadic attentional processes. Second, we observed an absence of this increase when IOR was induced near the saccade target that we suggest affects the initial presaccadic shift of attention. To verify the validity of our conclusions, we wanted to ensure that the observed increase in performance near the saccade targets was not due to confounding factors. As noted by Irwin and Gordon (Citation1998), performance is generally increased for targets at the end positions in this task and in linear arrays in general. Irwin and Gordon argue that this advantage is due to less visual crowding, since the stimuli at the endpoints have only one stimulus besides them. As we wanted to control for this possibility, a follow-up experiment was performed to examine the effects of flanking stimuli on the observed increase in performance near the saccade target. Our results thus far indicate that stimuli that are in the middle of the stimulus array are less accurately reported and may therefore be less attended to, due to not receiving a presaccadic attentional benefit. We propose that flanker stimuli will not receive this presaccadic benefit in a similar manner and therefore not interfere with performance in this paradigm. However, visual crowding has been linked to reducing the ability to identify stimuli (Whitney & Levi, Citation2011) and thus adding more stimuli to the array would reduce the accuracy for the elements at the end positions. To test for this possibility we added four flanker stimuli next to the end positions in the stimulus array.
Experiment 2
Method
Participants and procedure
The procedure for the second experiment was identical to Experiment 1 except for the following details. The study consisted of 12 participants from the Utrecht University community (11 female) aged 18 to 25, M = 21.8, that completed 120 trials in one 30 minute session. We chose this amount of trials for Experiment 2 to closely match the amount of critical trials in Experiment 1. All participants reported normal or corrected-to-normal vision.
The procedure used for the experiment is shown in . The main difference is that we only included a crowding condition, where in half of the trials four additional stimuli appeared next to the end point stimuli. In the remaining half of the trials the four additional stimuli did not appear. The crowding stimuli were interchanging between the letter T and the letter Q. We opted for these crowding stimuli as they are highly similar to the target stimuli (a necessity for crowding to occur; Kooi, Toet, Tripathy, & Levi, Citation1994; Levi, Citation2008; Whitney & Levi, Citation2011). With regards to Experiment 1, the onset stimulus has been removed and thereby the IOR condition. Timing for the experiment remained unchanged as compared to Experiment 1.
Figure 5. Sequence of events as they appeared in Experiment 2. Dashed lines with arrows indicate eye movement and the eye graphic indicates eye position. Top panel second from the left shows an example trial with crowding stimuli. The figure is presented in inverted contrast and is not drawn to scale.
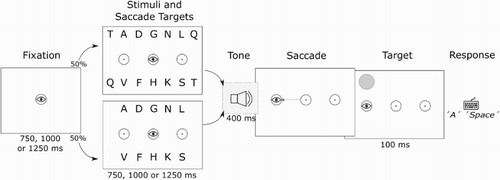
Data analysis
Trials were excluded on basis of the saccade missing its target by more than 2° or saccade latencies greater than 2.5 standard deviations from the median saccade latency. By these criteria 10.8% of the trials were excluded. Similar to Experiment 1, statistical analyses included a GLM model and a repeated measures analysis of variance. The fixed factors in the GLM model were location (five levels, from closest to saccade target to furthest) and whether the trial contained crowding stimuli or not (two levels). A random effect was added for participant. For analyses between locations we used Holm-Bonferroni corrected paired t-tests.
Results
We investigated the effects that crowding stimuli have on the linear array used in Experiment 1. To this end, we added stimuli next to the end point positions of the linear array in half of the trials and compared these with performance on trials without the extra stimuli. The results are shown in . First results for the GLM show a main effect was found for location of answer probe, z = 1.99, p = .04, the repeated measures analysis confirmed this, F(1,11) = 11.69, p < .01, η2 = 0.08. Post-hoc paired t-tests show that the proportion of letters correct is higher at the location closest to the saccade target (Location 1, M = 0.51, SD = 0.50) as compared to locations further from the saccade target (Locations 2 through 4, M = 0.24, SD = 0.43) with p < .01, d = 0.58. Moreover, performance at the location furthest from the saccade target was significantly higher (M = 0.42, SD = 0.50) than at the centre locations, p = .01, d = 0.39. Performance at the location closest to the saccade target was significantly better than at the location furthest from the saccade target (Location 5), p = .03, d = 0.18. No main effect was present for presence of the crowding stimuli, z = −0.12, p = .89 and no interaction effect was present for location of answer probe and presence of crowding stimuli, z = −0.20, p = .84. A repeated measures analysis of variance confirmed these results: no significant effect of crowding stimuli, F(1,11) = 0.02, p = .89, η2 < 0.01 and no evidence for an interaction effect between presence of crowding stimuli and location of answer, F(1,11) = 0.03, p = .87, η2 < 0.01.
Figure 6. Mean proportion of correct answers for locations in relation to saccade target between crowding stimuli and no crowding stimuli. Error bars show standard error. The dashed black line shows performance at chance level (0.08). In the figure the two conditions are horizontally offset as to not overlap visually.
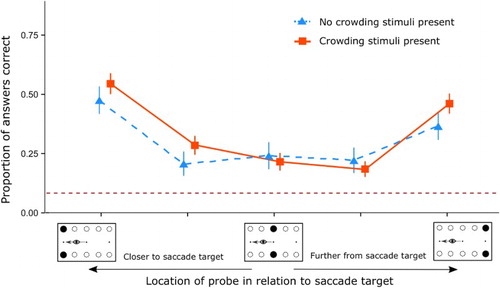
To provide further evidence that the added crowding stimuli did not interfere with performance on the task we performed a Bayesian analysis. We choose a Bayesian approach since it allowed us to further examine whether the null hypothesis (i.e., crowding does not affect accuracy on the transsaccadic memory paradigm) was rightly rejected or that our test was inconclusive (Dienes, Citation2011). This distinction cannot be made by using p values. To quantify this concept: if the Bayes factor is less than 0.33 it indicates evidence for the null hypothesis and there is evidence to support the alternative hypothesis when the Bayes factor is greater than 3. Conversely, if the Bayes factor is between 0.33 and 3 it indicates a lack of sensitivity and neither the null hypothesis nor the alternative hypothesis is supported. We conservatively modelled the prior distribution as a uniform distribution with a lower bound of 0 (no correct answers) and an upper bound of 1 (all answers correct). This analysis indicated significant support for the null hypothesis, BF = 0.08. Therefore, the null hypothesis was rightly accepted, as our data was 12.5 times as likely to be explained by the null hypothesis over the alternative hypothesis. We conclude that the crowding stimuli did not affect the performance on the transsaccadic memory task.
Discussion
We added crowding stimuli to the paradigm described in Experiment 1 to assess the influence that endpoint advantages have for correctly reporting the stimuli near the saccade targets. Our conclusions in Experiment 1 may be confounded by the finding that the end point stimuli have an advantage over the centre stimuli by lack of visual crowding. Our results for Experiment 2 indicate that endpoint advantages, due to a lack of crowding, did not influence performance. To elucidate these results: in both Experiment 1 and Experiment 2 a main effect for location was found, where elements in nearest proximity to the saccade targets were reported more accurately than elements further from the saccade target. These results are in line with the findings in the original experiment by Irwin and Andrews (Citation1996). The stimuli that are further away from the saccade target are not reported as accurately. The flanker stimuli appeared at a similar distance from the saccade target as the stimuli that were further from the saccade target. We suggest that crowding stimuli were not selected by the presaccadic shift of attention in a similar manner as the less accurately reported stimuli in the middle of the stimulus array. Thus, the crowding stimuli would not compete with the stimuli closest to the saccade target for storage in transsaccadic memory and thereby not elucidate effects of performance on the transsaccadic memory task.
General discussion
In this study we investigated how IOR affects information acquisition into transsaccadic memory. To this end we used a transsaccadic memory paradigm previously used by Irwin and Gordon (Citation1998) with an added exogenous cue to which an additional saccade had to be made. In both our experiment and the original paradigm it was found that information that is presented near a saccade target is reported more accurately, which has been attributed to the presaccadic shift of attention. The presaccadic shift of attention has been described as an effect that facilitates information acquisition, whereas IOR is seen as a negative attentional effect, increasing reaction times and reducing target discrimination. Our main finding was that IOR near a saccade target reduces accuracy for stimuli at that same location. This implies that these elements were less accurately acquired by transsaccadic memory and thus affected the participants’ ability to report this information. We did not observe a reduced performance for stimuli that were positioned further from the saccade target, or when IOR was induced further away from the saccade target. These results may implicate the attentional benefit provided by the presaccadic shift of attention being reduced by the negative attentional effect of IOR.
As we can only observe a relative effect in the paradigm used, we examined the validity of our conclusions by investigating possible confounding factors. Linear stimulus arrays, as the one used here, have been associated with endpoint advantages (Irwin & Gordon, Citation1998). Thus, we needed to exclude the possibility that the increased performance for stimuli at the endpoint positions in Experiment 1 was not due to a lack of visual crowding. In Experiment 2 we investigated the effects of crowding stimuli on the linear stimulus array used in Experiment 1, by using crowding stimuli which were similar to the experimental stimuli. We found that the linear array used was not affected by added visual crowding. This indicates that the increased performance at the endpoint positions in Experiment 1 were not due endpoint advantages. Thus, even though the effect we observed in Experiment 1 is relative, it seems unaffected by the lack of crowding for the elements near the saccade targets. However, the effect we have observed in Experiment 1 was found by inducing IOR through instructing the participants to execute a saccade to a probe and refixating the centre stimulus. Therefore, we cannot disentangle the oculomotor and attentional component of IOR in our current series of experiments. We hypothesize this effect to be most strongly present in the design as described in Experiment 1, as the effects of IOR may be reduced in the absence of saccadic eye movements (Kingstone & Pratt, Citation1999).
Previous research suggests that a limited amount of objects are stored in transsaccadic memory (Irwin & Gordon, Citation1998; Luck & Vogel, Citation1997; Ma, Husain, & Bays, Citation2014; Woodman, Vogel, & Luck, Citation2012). Thus, we analyzed whether the reduction of accuracy when a location near a saccade target is associated with IOR has an effect on the total amount of elements stored in transsaccadic memory. To this end we recoded the data relative to the onset position. Our results show that when the elements near a saccade target are less accurately reported, other elements remain unaffected and are reported as accurately as otherwise. This implicates that lessened information acquisition, in close proximity to a saccade target, is not compensated for by increased performance at other locations. This suggests that IOR not only modulates the accuracy of information acquisition, but also may reduce the amount of elements that are acquired between saccades.
The nature of transsaccadic memory has been a point of discussion since it was named. Similarities between visual short-term memory (VSTM) and transsaccadic memory have been noted since the term has arisen (Irwin, Citation1991). VSTM is the memory storage where a small amount of visual information is held for a limited time (Alvarez & Cavanagh, Citation2004; Cowan, Citation2001). Transsaccadic memory is described to exclusively store this visual information between saccades (Deubel, Schneider, & Bridgeman, Citation1996; Irwin & Andrews, Citation1996). Research has shown that transsaccadic memory carries many similarities to VSTM, such as the limited amount of objects retained and information being selected by similar attentional processes (for a review, see Luck & Hollingworth, Citation2008). As such, transsaccadic memory may be a subsystem of VSTM (Hollingworth, Richard, & Luck, Citation2008). Importantly, previous studies have linked VSTM and IOR closely together. Both VSTM and IOR are thought to be used in visual search tasks, as to respectively remember the locations of previous fixations and to inhibit revisiting previously attended locations (Castel et al., Citation2003; Klein & Ivanoff, Citation2000; McCarley, Wang, Kramer, Irwin, & Peterson, Citation2003). Although our study does not necessarily support the notion that VSTM and transsaccadic memory may consist of the same system, our study adds that previously fixated locations within the field of the presaccadic shift of attention are affected in terms of information acquisition, which may implicate both VSTM and transsaccadic memory.
To summarize, this study provides evidence that IOR and transsaccadic memory are functionally linked. Our results are in line with the notion that IOR prioritizes information presented at uninspected locations (Pratt et al., Citation2001), and adds that there is less benefit from the attentional processes that are closely linked to execution of saccades at locations associated with IOR. This loss of benefit is not compensated for by increased performance at other locations in the visual field and thus results in lessened acquisition of information.
Disclosure statement
No potential conflict of interest was reported by the authors.
Additional information
Funding
References
- Alvarez, G. A., & Cavanagh, P. (2004). The capacity of visual short-term memory is set both by visual information load and by number of objects. Psychological Science: A Journal of the American Psychological Society, 15(2), 106–111. doi:10.1167/2.7.273
- Bays, P. M., & Husain, M. (2008). Dynamic shifts of limited working memory resources in human vision. Science, 321(5890), 851–854. doi:10.1126/science.1158023
- Bell, B. A., Morgan, G. B., Schoeneberger, J. A., Loudermilk, B. L., Kromrey, J. D., & Ferron, J. M. (2010). Dancing the sample-size limbo with mixed models: How low can you go? SAS Global Forum, 4, 11–14.
- Briand, K. A., Larrison, A. L., & Sereno, A. B. (2000). Inhibition of return in manual and saccadic response systems. Perception & Psychophysics, 62(8), 1512–1524. doi:10.3758/BF03212152
- Castel, A. D., Pratt, J., & Craik, F. I. M. (2003). The role of spatial working memory in inhibition of return: Evidence from divided attention tasks. Perception & Psychophysics, 65(6), 970–981. doi:10.3758/BF03194827
- Castiello, U., & Umiltà, C. (1990). Size of the attentional focus and efficiency of processing. Acta Psychologica, 73(3), 195–209. doi:10.1016/0001-6918(90)90022-8
- Cnaan, A., Laird, N. M., & Slasor, P. (2014). Using the general linear mixed model to analyse unbalanced repeated measures and longitudinal data. Journal of Neuroscience Methods, 509(1), 805–820. doi:10.1002/(SICI)1097-0258(19971030)
- Coëffé, C., & O’Regan, J. K. (1987). Reducing the influence of non-target stimuli on saccade accuracy: Predictability and latency effects. Vision Research, 27(2), 227–240. doi:10.1016/0042-6989(87)90185-4
- Cohen, M. E., & Ross, L. E. (1978). Latency and accuracy characteristics of saccades and corrective saccades in children and adults. Journal of Experimental Child Psychology, 26(3), 517–27. doi:10.1016/0022-0965(78)90130-3
- Cowan, N. (2001). The magical number 4 in short-term memory: A reconsideration of mental storage capacity. Behavioral and Brain Sciences, 24, 87–114. doi: 10.1017/S0140525X01003922
- Dalmaijer, E. S., Mathôt, S., & Van der Stigchel, S. (2013). PyGaze: An open-source, cross-platform toolbox for minimal-effort programming of eyetracking experiments. Behavior Research Methods, 46(4), 1–16. doi:10.3758/s13428-013-0422-2
- Deubel, H., & Schneider, W. X. (1996). Saccade target selection and object recognition: Evidence for a common attentional mechanism. Vision Research, 36(12), 1827–1837. doi:10.1016/0042-6989(95)00294-4
- Deubel, H., Schneider, W. X., & Bridgeman, B. (1996). Postsaccadic target blanking prevents saccadic suppression of image displacement. Vision Research, 36(7), 985–996. doi:10.1016/0042-6989(95)00203-0
- Dienes, Z. (2011). Bayesian versus orthodox statistics: Which side are you on? Perspectives on Psychological Science, 6(3), 274–290. doi:10.1177/1745691611406920
- Hilchey, M. D., Klein, R. M., & Satel, J. (2014). Returning to “inhibition of return” by dissociating long-term oculomotor IOR from short-term sensory adaptation and other nonoculomotor “inhibitory” cueing effects. Journal of Experimental Psychology. Human Perception and Performance, 40(4), 1603–16. doi:10.1037/a0036859
- Hoffman, J. E., & Subramaniam, B. (1995). The role of visual attention in saccadic eye movements. Perception & Psychophysics, 57(6), 787–795. doi:10.3758/BF03206794
- Hollingworth, A., & Henderson, H. J. (1998). Does consistent scene context facilitate object perception? Journal of Experimental Psychology: General, 127(4), 398–415. doi: 10.1037/0096-3445.127.4.398
- Hollingworth, A., Richard, A. M., & Luck, S. J. (2008). Understanding the function of visual short-term memory: Transsaccadic memory, object correspondence, and gaze correction. Journal of Experimental Psychology, 137(1), 163–181. doi: 10.1037/0096-3445.137.1.163
- Ihaka, R., & Gentleman, R. (1996). R: A language for data analysis and graphics. Journal of Computational and Graphical Statistics, 5(3), 299–314. doi:10.2307/1390807
- Irwin, D. E. (1991). Information Integration across saccadic eye movements. Cognitive Psychology, 23, 420–456. doi: 10.1016/0010-0285(91)90015-G
- Irwin, D. E., & Andrews, R. V. (1996). Integration and accumulation of information across saccadic eye movements. Attention and performance: Information integration in perception and communication, 16, 125–155.
- Irwin, D. E., & Gordon, R. D. (1998). Eye Movements, attention and trans-saccadic memory. Visual Cognition, 5, 127–155. doi:10.1080/713756783
- Johnson, J. S., Hollingworth, A., & Luck, S. J. (2008). The role of attention in the maintenance of feature bindings in visual short-term memory. Journal of Experimental Psychology, 34, 41–55.
- Kenward, M. G., Roger, J. H., Process, L. D., Lodge, C. H., & Ox, O. (2013). Small sample inference for fixed effects from restricted maximum likelihood. Biometrics, 53(3), 983–997. doi: 10.2307/2533558
- Kingstone, A., & Pratt, J. (1999). Inhibition of return is composed of attentional and oculomotor processes. Perception & Psychophysics, 61(6), 1046–54. doi:10.3758/BF03207612
- Klein, R. M., & Ivanoff, J. (2000). Inhibition of return. Trends in Cognitive Sciences, 4(4), 138–147. doi: 10.1016/S1364-6613(00)01452-2
- Klein, R. M., & MacInnes, W. J. (1999). Inhibition of return is a foraging facilitator in visual search. Psychological Science, 10(4), 346–352. doi:10.1111/1467-9280.00166
- Kooi, F. L., Toet, A., Tripathy, S. P., & Levi, D. M. (1994). The effect of similarity and duration on spatial interaction in peripheral vision. Spatial Vision, 8(2), 255–279. doi: 10.1163/156856894X00350
- Kuznetsova, A., Brockhoff, P. B., & Christensen, R. H. B. ( 2013). lmerTest: Tests for random and fixed effects for linear mixed effect models (lmer objects of lme4 package). Retrieved from https://cran.r-project.org/web/packages/lmerTest/lmerTest.pdf
- Levi, D. M. (2008). Crowding—An essential bottleneck for object recognition: A mini-review. Vision Research, 48(5), 635–654. doi:10.1016/j.visres.2007.12.009
- Lindstrom, M. J., & Bates, D. M. (1990). Nonlinear mixed effects models for repeated measures data. Biometrics, 46(3), 673–687. doi: 10.2307/2532087
- Luck, S. J., & Hollingworth, A. (2008). Visual memory (S. J. Luck & A. Hollingworth, Eds.). New York, NY: Oxford University Press. doi:10.1177/105345126600100312
- Luck, S. J., & Vogel, E. K. (1997). The capacity of visual working memory for features and conjunctions. Nature, 390(1996), 279–281. doi:10.1038/36846
- Ma, W. J., Husain, M., & Bays, P. M. (2014). Changing concepts of working memory. Nature Neuroscience, 17(3), 347–56. doi:10.1038/nn.3655
- Martín-Arévalo, E., Kingstone, A., & Lupiáñez, J. (2012). Is “Inhibition of Return” due to the inhibition of the return of attention? The Quarterly Journal of Experimental Psychology, 66(2), 1–13. doi:10.1080/17470218.2012.711844
- McCarley, J. S., Wang, R. F., Kramer, A. F., Irwin, D. E., & Peterson, M. S. (2003). How much memory does oculomotor search have? Psychological Science, 14(5), 422–426. doi:10.1111/1467-9280.01457
- Müller, M. M., & Hübner, R. (2002). Can the spotlight of attention be shaped like a doughnut? Evidence from steady-state visual evoked potentials. Psychological Science: A Journal of the American Psychological Society, 13(2), 119–124. doi:10.1111/j.0956-7976.2002.t01-1-.x
- Posner, M. I., & Cohen, Y. (1984). Components of visual orienting. Attention and Performance: Control of Language Processes, 32, 531–556. doi:10.1162/jocn.1991.3.4.335
- Pratt, J., Hillis, J., & Gold, J. M. (2001). The effect of the physical characteristics of cues and targets on facilitation and inhibition. Psychonomic Bulletin & Review, 8(3), 489–495. doi:10.3758/BF03196183
- Prime, S. L., Tsotsos, L., Keith, G. P., & Crawford, J. D. (2007). Visual memory capacity in transsaccadic integration. Experimental Brain Research, 180(4), 609–628. doi:10.1007/s00221-007-0885-4
- Reuter-Lorenz, P. A., Jha, A., & Rosenquist, J. N. (1996). What is inhibited in inhibition of return? Journal of Experimental Psychology: Human Perception and Performance, 22(2), 367–378.
- Sapir, A., Jackson, K., Butler, J., Paul, M. A., & Abrams, R. A. (2013). Inhibition of return affects contrast sensitivity. The Quarterly Journal of Experimental Psychology, 67(7), 1305–1316. doi:10.1080/17470218.2013.859282
- Schut, M. J. (2016). P01: Inhibition of return in transsaccadic memory. doi:10.17605/OSF.IO/56BJE
- Van der Stigchel, S., & De Vries, J. P. (2015). There is no attentional global effect: Attentional shifts are independent of the saccade endpoint. Journal of Vision, 15(15), 1–12. doi:10.1167/15.15.17
- Van Selst, M., & Jolicoeur, P. (1994). A solution to the effect of sample size on outlier elimination. The Quarterly Journal of Experimental Psychology, 47A(3), 631–650. doi:10.1080/14640749408401131
- Whitney, D., & Levi, D. M. (2011). Visual crowding: A fundamental limit on conscious perception and object recognition. Trends in Cognitive Sciences, 15(4), 160–168. doi:10.1016/j.tics.2011.02.005.Visual
- Wolfe, J. M. (1994). Guided search 2.0 a revised model of visual search. Psychonomic Bulletin & Review, 1(2), 202–238. doi: 10.3758/BF03200774
- Wolfe, J. M., Reinecke, A., & Brawn, P. (2006). Why don’t we see changes? The role of attentional bottlenecks and limited visual memory. Visual Cognition, 14(4–8), 749–780. doi:10.1080/13506280500195292
- Woodman, G. F., Vogel, E. K., & Luck, S. J. (2012). Flexibility in visual working memory: Accurate change detection in the face of irrelevant variations in position. Visual Cognition, 20, 1–28. doi:10.1080/13506285.2011.630694