Abstract
Aim: This study aimed to evaluate the effect of physiological testosterone replacement on male aged rats with orchiectomy-induced osteoporosis in advanced stage.
Methods: Thirty male rats (Rattus norvegicus albinus, Holtzman lineage) were randomly distributed into 3 groups (n = 10): 1-sham, 2-orchiectomy (OCX), 3-OCX + testosterone replacement (OCX + T). On day 0, a sham or orchiectomy surgery was performed according to the groups. Thirty and sixty days after surgeries, the animals from OCX + T group received testosterone intramuscularly, and the rats in all groups were euthanized on day 77. The femurs were removed for micro-CT scanning and biomechanical test.
Results: Orchiectomy resulted in a marked trabecular bone damage (p < 0.05), which was not reversed with testosterone treatment (OCX + T group). The femoral strength was lower in orchiectomized animals (p < 0.05), while the bone strength in OCX + T group was similar to that observed in the sham animals (p > 0.05) and correlated to this parameter the deformation of rupture was smaller in OCX + T group.
Conclusion: In conclusion, testosterone depletion induced by orchiectomy established an osteoporotic environment, mainly affecting the trabecular bone. Moreover, even though testosterone treatment did not enhance these variables, the hormonal replacement improved the femoral fracture strength and promoted beneficial effects on the biomechanical parameters compromised by castration in femoral bone.
Introduction
The incidence of osteoporotic fractures in men and women has increased rapidly, as both women and men have been living increasingly longer [Citation1]. Osteoporosis is a skeletal disease characterized by low bone mass and bone architecture disruption, resulting in loss of bone strength and increased fracture risk. Usually, osteoporosis presents no symptoms and has its diagnosis established after the first fracture occurrence [Citation2]. “Primary” osteoporosis or osteoporosis derived from primary causes refers to a bone disease resulting from physiologic bone loss associated with aging; for women, this increased loss can be related to menopause. Osteoporosis resulting from other disorders or medication exposures is referred to as “secondary” osteoporosis or osteoporosis resulting from secondary causes [Citation3]. It is more frequent in male than in female patients [Citation4], and alcohol abuse, use of corticosteroids, diabetes mellitus and hypogonadism are the most commonly related factors [Citation5,Citation6].
It is well established that androgen can influence bone strength by binding directly to androgen receptors, or indirectly to estrogen receptors via testosterone aromatization to estrogen [Citation7]. In women, bone loss has been correlated with a normal decrease of estrogen in the onset of menopause [Citation8]. In contrast, as men do not have menopause the decrease in testosterone may result from any other disorder, such as hypogonadism, or a therapeutic castration due to diseases like prostate cancer [Citation5]. Risk factors for the development of osteoporosis in women are best documented than in men [Citation1], and even though fracture prevalence is higher in women, fractures constitute a significant public health issue in older men [Citation7]. Furthermore, among patients suffering from fractures, men have higher mortality than women [Citation9].
The effects of testosterone on bone occur by direct and indirect mechanisms [Citation7,Citation10,Citation11]. These mechanisms involve several cells within the bone, including human mesenchymal stem cells, osteoblasts and osteocyte (those that express androgen receptors), which are target cells for testosterone [Citation8]. Moreover, the indirect mechanism of androgens on bone cells may involve the expression of several cytokines and the local production of growth hormone (GH) and insulin-like growth factor 1 [Citation12].
Therefore, it seems plausible to correlate low bioavailable testosterone levels in aging men with loss in mineral bone density (BMD), bone volume and increased fracture risk, similar to women in menopause. The clinical or in vivo literature regarding low bioavailable testosterone and hormonal replacement in correlation with bone loss and increased risk of fracture in men is still limited [Citation13–19]. Although there are some clinical trials showing an improvement of BMD by testosterone replacement treatment, Lee et al. [Citation15] described that most studies evaluating testosterone replacement in hypogonadal men have been uncontrolled, performed in a short term and involving a small sample size with a variety of causes for their hypogonadism. Bone health in men is sometimes a neglected health issue, as it is less investigated than in women [Citation12], justifying the lack of studies exploring the effect of testosterone therapy on bone homeostasis. Thereby, our study aimed to evaluate the physiological testosterone replacement effect on male aged rats with orchiectomy-induced osteoporosis in advanced stage.
Material and methods
Animals
All experimental protocols were approved by the Ethics Committee from the Araraquara School of Dentistry at UNESP (Process CEUA/FOAr #20/2016). The procedures were conducted in compliance with the Brazilian National Council for the Control of Animal Experimentation, with ethical standards that fully comply with Animal Research: Reporting of In Vivo Experiments (ARRIVE) guidelines.
The sample size (n) was calculated to be 10 animals/group. A total of thirty male rats (Rattus norvegicus albinus, Holtzman lineage), aged approximately 11–12 months old were maintained at a temperature of 21°C ± 1, humidity of 65–75% and a controlled light cycle (12 h light-12 h dark), with feed and water available ad libitum. The animals were randomly distributed into 3 groups (n = 10): 1-sham, 2-orchiectomy (OCX), 3-OCX + testosterone replacement (OCX + T). On day 0, a sham or orchiectomy surgery was performed according to the groups. Thirty and sixty days after surgeries, the animals from OCX + T group received testosterone intramuscularly, and the rats in all groups were euthanized on day 77. The groups and experimental conditions are shown in .
Figure 1. Experimental design. Thirty rats were distributed into 3 groups (n=10/group) - Sham group (no testicle removal), Orchiectomy group (OCX) and OCX + Testosterone replacement group (OCX+T). Orchiectomy (testicle removal) was performed on day 0 (week 1), and the testosterone administration (62.5 mg/kg body weight of Nebido) was performed in two moments: 30 and 60 days after orchiectomy. The testicles of the animals in sham groups were exposed and manipulated, but not removed. The rats were euthanized on day 77, when completed 11 weeks.
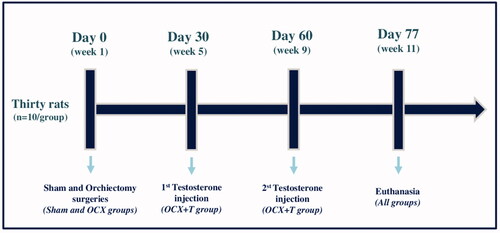
Androgen deprivation model
Briefly, 20 rats (OCX group) under anesthesia with ketamine (100 mg/kg body weight, intramuscular – Ceva Animal Health, Paulínia, Brazil) and xylazine (8 mg/kg body weight, intramuscular – Ceva Animal Health, Paulínia, Brazil) and in sterile conditions underwent a scrotal incision for bilateral testicular removal (orchiectomy). The rats received Ketoprofen (0.05 mg/rat, intramuscular – MERIAL, Campinas, Brazil) for postoperative pain relief, and Pentabiotic® (200 mg/Kg body weight, intramuscular – Zoetis, São Paulo, Brazil). The sham procedure was performed in 10 rats through testicle exposure and manipulation (without removal), replacement, and suturing (Sham group). After the procedure, the animals were kept in separate cages for recovery for 1 week [Citation20] ().
Hormonal replacement with testosterone
Thirty days after surgery, the rats (OCX + T group) received testosterone undecanoate (Nebido® intramuscularly, 62.5 mg/kg body weight – Bayer, Socorro, Brazil). On day 60, a second exogenous testosterone administration was performed until day 77, as previously described, for the maintenance of physiological levels of testosterone [Citation20,Citation21] ().
Serum testosterone evaluation
A blood sample was collected from all animals at the beginning of the experiment, before the surgical procedure (baseline). A second and third caudal blood collections were performed in all animals 25 days after the first testosterone replacement (TR) and repeated 48 h after the second TR. A final blood collection was performed with cardiac puncture at euthanasia to assess testosterone levels. The blood samples were centrifuged at 3.000 rpm for 10 min, and the serum was collected and kept at −80 °C until the analysis. Each serum sample was analyzed for total testosterone levels using a chemiluminescence-based immunoassay, and the concentration was expressed in ng/mL (Immulite 2000, Siemens Healthcare Diagnostics, Gwynedd, United Kingdom) [Citation20]. The purpose of the second and third harvestings was to verify the pharmacokinetics of the exogenous testosterone administered, comparing the hormone concentration in this group with the sham and orchiectomized animals.
Euthanasia and sample harvesting
After completing the experimental period, all rats were euthanized using general anesthesia with ketamine (100 mg/kg body weight, intramuscular – Ceva Animal Health, Paulínia, Brazil) and xylazine (8 mg/kg body weight, intramuscular – Ceva Animal Health, Paulínia, Brazil), followed by sample collection. Femurs were removed, fixed in 10% buffered formalin (pH 7.2) for 48 h and kept in 70% ethanol before micro-CT scanning. For the quantitative three-dimensional analysis, both femurs of all animals were scanned using a micro-CT system (Skyscan, Aartselaar, Belgium), and then submitted to the compressive strength evaluation.
Microcomputed tomography (µCT) analysis
The bone samples were wrapped in humid paper to prevent artifacts caused by dehydration during the scanning process. The femurs were scanned in the micro-computed tomography (μ-CT) equipment using a microfocus X-ray CT system (Skyscan 1176, Artselaar, Belgium). The chosen scanning parameters were: X-ray generator operating at 50 kVp, beam current of 500 μA, attenuation filter of 0.5 mm, rotation step of 0.5° and frame averaging of 3. The three-dimensional images were reconstructed using the software NRecon 1.6.9.8 (SkyScan N. V. Belgium). Data Viewer 1.5.0 (SkyScan, Belgium) and CTAnalyser (CTAn − 2003-11SkyScan, 2012 Bruker MicroCT, 1.13.11.0 version) were used for image re-orientation and analysis, respectively.
The region of interest (ROI) included 100 slices in the proximal metaphysis of the femur for both trabecular and cortical bone analyses. To standardize the ROI for all samples, the first 350 slices from the epiphysis were discarded. The bone microarchitecture parameters evaluated were bone volume fraction (BV/TV, %), trabecular thickness (Tb.Th), trabecular number (Tb.N), trabecular separation (Tb.S), trabecular and cortical connectivity density (Conn.D), cortical thickness (C.Th), cortical total porosity (Po.tot) and bone mineral density (BMD). The calibration for bone mineral density (BMD) measurements was performed with two calcium hydroxyapatite (CaHA) phantom rods containing two different concentrations of 0.25 and 0.75 g CaHA/cm3, respectively. The phantom rods were scanned and reconstructed using the same parameters as those used for the femur, as described above. The attenuation coefficient was entered into the calibration dialogue window in CTAn to determine BMD (mg/cm3) [Citation19,Citation20,Citation22].
Biomechanical evaluation
The biomechanical evaluation was performed using the MTS 810 mechanical test machine (Material Test System – MTS 810; MTS System Corporation, Minneapolis, USA) by a three-point bending test using a compressive force.
The three-point bending strength was set with 2 cm between the two support arms. The femurs were positioned horizontally, and the tip of the compressive force was applied perpendicular to the center of the femoral diaphysis, the area in which the mechanical fracture occurred. A constant crosshead speed of 0.5 mm/min was applied, and the data were obtained from Test Star II software (MTS System Corporation, Minneapolis, USA). The mechanical parameters evaluated were the compressive strength (maximum load force – N/mm), young modulus (GPa), strain (MPa), ultimate stress (MPa) and deformation of rupture (%). [Citation23]
Data analysis
Data obtained from each evaluation were analyzed using the statistical software GraphPad Prism 6 (GraphPad Software Inc., San Diego, CA, USA). The distribution was evaluated by the Shapiro-Wilk test; one-way ANOVA and post-hoc Tukey tests were performed for multiple comparison, and t-test was carried out in order to compare testosterone levels at the beginning and end of the experiment. All tests were applied with a confidence level of 95%. Data are expressed as mean ± SD.
Results
Adverse events
Orchiectomy or exogenous testosterone treatment did not promote any behavioral change, physical issues (e.g. skin lesions, hair loss), significant weight loss or feeding impairment.
Orchiectomy and exogenous testosterone administration were effective in reproducing the testosterone decrease and its physiological replacement
Animals showed homogeneity in the testosterone serum concentration at the beginning of the experiment. After 77 days, the animals in sham and orchiectomy + testosterone (OCX + T) groups presented no difference in the testosterone serum level, showing similar concentration compared to the respective groups on day 0. However, orchiectomy (OCX) promoted a significant decrease in hormone concentration in comparison with the other groups on day 77 and the respective group on day 0 (p < 0.05) ().
Figure 2. Serum testosterone concentration. The animals in all groups showed a similar testosterone level on day 0 (week 1). After 77 days (weel 11), orchiectomy (OCX) and testosterone treatment (OCX+T) promoted, respectively, the complete depletion and physiological replacement of hormone (chart A). Over time, as showed in the chart B, the testosterone level remained at physiological levels in both Sham and OCX+T groups, although the higher concentrations in the animals that received exogenous testosterone, meanwhile the hormone concentration was completed reduced by OCX during the time. Chart A - *P < 0.05 when compared to the respective group on day 0 (week 1), **P < 0.05 between groups on day 77 (week 11). Chart B - Different letters indicate statistical difference between the groups (Sham, OCX, OCX+T) in the same timepoint, and identical letters show no difference; Asterisks indicate statistical difference within a group over time. ***P<0.05 in comparison to the timepoint of 48hrs post-2nd testosterone replacement. Data are shown as mean ± SEM. Sham - No testicle removal.
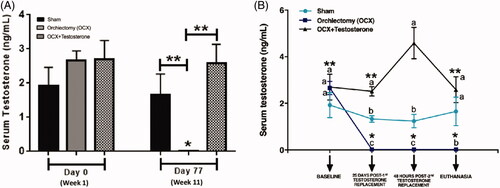
Over time, the testosterone level remained at physiological levels in both sham and OCX + T groups despite the higher concentrations in the animals that received exogenous testosterone. Meanwhile, the hormone concentration was completed reduced by OCX. Although statistically significant, the increase in serum concentration of testosterone in the OCX + T group was considered small. In addition, the hormonal levels remained at physiological levels until the end of the experimental period.
Orchiectomy compromised trabecular microarchitecture and testosterone did not promote any significant improvement in bone parameters
Testosterone depletion (OCX group) reduced cortical thickness (p < 0.05) but did not impact bone volume and bone mineral density (BMD) when evaluating the cortical bone (). However, when compared to the sham group, the orchiectomized animals treated with testosterone showed a reduced percentage of cortical bone volume and a higher percentage of total porosity (p < 0.05). In contrast, orchiectomy markedly reduced trabecular bone volume, trabecular thickness, trabecular number, connectivity density and mineral density when compared to the sham group (p < 0.05). Testosterone administration (OCX + T) did not reverse the trabecular bone parameters evaluated (p > 0.05). However, trabecular number and thickness tended to be higher in OCX + T compared to the OCX group, remaining similar to the sham group ().
Figure 3. Microcomputed tomographic evaluation of femoral bone. The microarchitecture parameters of trabecular (Panel A) and cortical bone (Panel B) evaluated were bone volume fraction (BV/T V, %), Trabecular thickness (Tb.Th), Trabecular number (Tb.N), Trabecular separation (Tb.S), Trabecular and Cortical Connectivity density (Conn.D), Cortical thickness (C.Th), Cortical Total porosity (Po.tot) and bone mineral density (BMD). The 3D reconstructed images of femurs are representative of each group and illustrate the results of the graphs. Data are shown as mean ± SEM. The bars represent the average, while the vertical lines indicate the standard deviation. *p<0.05 compared to the sham group. Sham - no testicle removal.
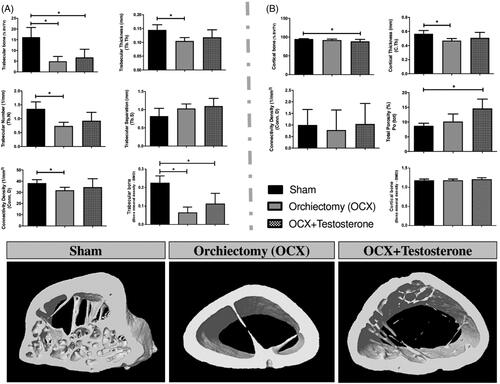
Testosterone administration promoted beneficial effects on the biomechanical parameters compromised by castration in femoral bone
The biomechanical test showed that the femoral strength as well as the strain and stress parameters were significantly lower in orchiectomized animals (OCX) compared to the sham group (p < 0.05) even though the young modulus and deformation of rupture parameters were not different when comparing both groups. The physiological testosterone replacement (OCX + T) resulted in a bone strength similar to that observed in the sham animals, and correlated to this parameter the young modulus and stress were found to be higher in the OCX + T group than in the OCX group (p < 0.05) and similar to the sham animals, while the deformation of rupture was smaller in the OCX + T group ().
Figure 4. Biomechanical evaluation of femurs on day 77 (week 11). The biomechanical evaluation was performed using the MT S 810 mechanical test machine by a three-point bending testing and the mechanical parameters evaluated were the compressive strength (maximum load force - N/mm), young modulus (GPa), strain (MPa), ultimate stress (MPa) and deformation of rupture (%). Testosterone administration prevented the reduction of femoral bone strength promoted by castration. Data are shown as mean ± SEM. The bars represent average, while vertical lines indicate the standard deviation. *P<0.05 compared to the sham group; **P<0.05 compared to the orchiectomy group. Sham - no testicle removal.
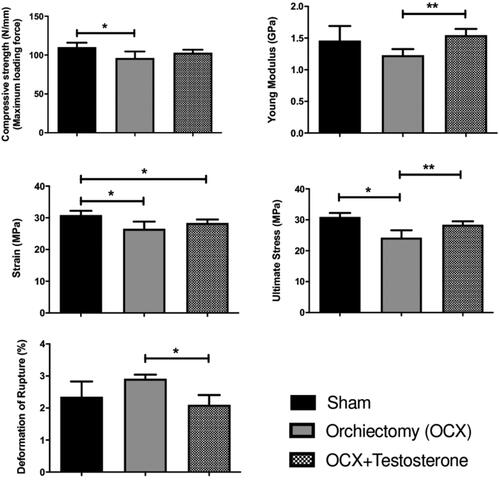
Discussion
The available evidence regarding the testosterone role in bone health has been described in different methods, and despite the fact that the results demonstrate that the hormone absence is related to a decrease in the bone quality, the information reporting the effect of testosterone therapy on the improvement of bone parameters are still scarce [Citation13–19]. In our study, we evaluated the effect of physiological testosterone replacement on male aged rats with advanced stage of osteoporosis induced by physical castration. We emphasize that the relevance of this study is mainly the physiological use of testosterone as well as the advanced model of osteoporosis femur in comparison with other experimental studies using shorter periods of time [Citation14,Citation24]. Orchiectomy in rats was found to be an important model to study the effects of androgen deficiency or replacement, leading to an environment similar to that found in male osteoporosis [Citation14,Citation25,Citation26]. Also, the World Health Organization (WHO) validated the orchiectomy model in studies that evaluate bone loss to simulate osteoporosis in men [Citation18].
The current study showed that long-term orchiectomy promoted a complete testosterone depletion, culminating in remarkable alterations in the bone remodeling process with a significant deterioration of the trabecular bone, thus proving to be sufficient to cause osteoporosis induced by hormone depletion. In general, the findings provided by µCT (microcomputed tomography) analysis are in accordance with the literature, which shows that the trabecular bone is the most affected in osteoporosis [Citation19,Citation27]. Considerable differences in cortical bone volume could be found in longer periods, according to previous studies reporting that orchiectomy significantly affects the cortical bone mass in only 4 months [Citation28,Citation29]. However, the cortical microarchitecture damage initiates earlier, as observed by the significant cortical thickness decrease already in 11 weeks after orchiectomy.
Hormonal treatment was not able to revert bone deterioration, even after 7 weeks of testosterone replacement following orchiectomy. A systematic review and meta-analysis evaluating the preventive use of testosterone in osteoporotic fractures and the bone mass improvement in hypogonadal and elderly men with androgen decline have shown that intramuscular testosterone moderately increases lumbar bone density; however, the results on femoral neck bone density were inconclusive [Citation13]. In the present study, the measurements were limited to the femoral bone, and an evaluation of other structures (e.g. lumbar spine, tibia) could possibly provide the observation of beneficial and significant results due to testosterone treatment. We should also consider that testosterone replacement, if performed in a longer period or initiated in a shorter period of time after orchiectomy (and consequently in the earlier stage of bone damage), could promote some improvements in the femoral bone parameters. A previous study evaluating the supraphysiological testosterone administration in the femoral trabecular structure in rats for 8 weeks after 1 week of orchiectomy showed that the hormone prevented bone damages such as those observed in castrated animals [Citation14]. Thus, it suggests that in an animal model of premature osteoporosis the exogenous testosterone administration promotes a preventive effect on trabecular bone impairment. Nevertheless, this study can be seen as inconclusive if considered time- or dose-dependent, or both. The absence of preceding in vivo studies using different experimental design, for instance, the evaluation of long or short-term physiological testosterone replacement in an early or late osteoporotic condition, makes any comparison impossible.
The preliminary findings of this study present a different perspective, as our experimental protocol emphasizes a therapeutic – and not preventive – role of testosterone administration in an advanced osteoporotic condition. Taking into account the clinical situation, we should highlight that different stages of osteoporosis, from initial bone damage to advanced resorption, can be seen. Therefore, it is important to investigate if the hormonal replacement acts in the prevention (anti-resorptive activity) or reversion (stimulation of bone formation) of the osteoporotic damage. Evidently, to better understand these specific mechanisms, a measurement of resorption or formation markers (bone turnover) would be necessary – which was not the focus of our study. By evaluating the effects of orchiectomy and supraphysiological testosterone administration on the trabecular bone structure and the gene expression in male rats we found that the hormonal treatment did not change the expression of most genes related to bone remodeling, whereas the expression of the gene receptor activator of nuclear factor kappa β-ligand (RANKL) and osteoprotegerin (OPG) significantly decreased [Citation14]. From these results, we could suggest an anti-resorptive preventive activity promoted by testosterone treatment.
Furthermore, the effect of physiological testosterone replacement was also investigated using an orchiectomy-induced osteoporosis model in male rats. The histomorphometry of femurs revealed that when the orchiectomized rats received testosterone the bone volume was restored, similar to what was observed in uncastrated animals [Citation24]. Despite the similarity with our study, the testosterone treatment was conducted just for 6 weeks, starting on the same day of castration, evidencing the preventive target of the study.
Testosterone replacement (OCX + T group) affected the bone fracture strength and other parameters in femurs of orchiectomized rats, as found in the compressive strength analysis. This biomechanical evaluation was repeatedly used to directly indicate the material strength [Citation19,Citation30,Citation31]. In the present study, the femur samples were fixed in formalin (48 h) before the mechanical test, consisting in a suitable and safe method according to recent studies reporting the effect of formalin preservation on the mechanical properties of bone, describing that formalin fixation up to 12 months does not significantly change the mechanical properties of bone [Citation32,Citation33]. The previous findings showed that formalin preservation of bovine femoral cortical bones at a lower temperature and less than 4 weeks is recommended for biomechanical testing [Citation32], while short-term chemical fixation with formalin of C57BL/6 mice femur samples initiates some changes in collagen structure but are minimally influencing bone material properties measured with Raman spectroscopy [Citation33]. Moreover, we emphasize that formalin solution concentration, preservation temperature, preservation time, specimen shape and other factors might affect the mechanical properties of bone as described previously [Citation32], and we also highlight that in the hypothesis of a minimal alteration occurred due the formalin fixation, all the samples in our study were submitted to the same conditions and the results would present the same bias. Thus, the effect of testosterone depletion or replacement on bones strength, evaluated by the biomechanical analysis, shouldn’t be interpreted in a maximized or minimized view.
It is worth mentioning that the orchiectomy + testosterone group (OCX + TR) revealed a slight increase in bone volume and bone mineral density (BMD), although without a significant difference, which seemed to modify the bone strength and improve the other biomechanical parameters such as stress and deformation of rupture. No changes were found in the BMD of the cortical bone, revealing that trabecular bone alterations were sufficient to reduce the femoral strength. However, previous studies described that BMD could not be used to reflect osteoporosis-associated fracture risk [Citation34,Citation35]. Additionally, given the limitations of comparison, a clinical retrospective study was used to evaluate the effects of testosterone replacement therapy on BMD in postoperative hypogonadal patients with pituitary tumors. The findings showed a significant improvement in the BMD of lumbar spine compared to the baseline BMD. Nevertheless, no significant changes in the femur neck or total femur BMD were noted [Citation15].
Despite the limitations, our results are novel and preliminary data showing the effect of physiological testosterone replacement on male aged rats with orchiectomy-induced osteoporosis in advanced stage. Our purpose was to investigate the therapeutic role of testosterone in advanced male osteoporosis and to corroborate future studies focused on producing new evidence capable of being transferred to the clinical interventions. However, as previously described [Citation24], it is important to consider that the adverse effects of testosterone could pose a problem to the patients such as sleep apnea, polycythemia, liver toxicity, and more importantly, the risk of developing cancer since hormone-dependent cancers (such as metastatic prostate and breast cancer) may be stimulated during testosterone treatment. Therefore, testosterone is not recommended for those with underlying or high-risk factors for this type of disease [Citation24]. The effect of high circulating testosterone level on the prostate is other one issue of concern, as observed in studies conducted with animals, which report that a supraphysiological dose of testosterone could cause prostate hypertrophy [Citation14,Citation36,Citation37]. However, new studies should be performed to clear the side-effects of testosterone replacement in different protocols, comparing physiological or supraphysiological doses.
In summary, testosterone depletion induced by orchiectomy established an osteoporotic environment, affecting mainly the trabecular bone. Moreover, even though testosterone treatment did not enhance these variables, the hormonal replacement improved the femoral fracture strength and promoted beneficial effects on the biomechanical parameters compromised by castration in the femoral bone.
Disclosure statement
No potential conflict of interest was reported by the author(s).
References
- Misiorowski W. Osteoporosis in men. Prz Menopauzalny. 2017;16(2):70–73.
- Johnston CB, Dagar M. Osteoporosis in older adults. Med Clin North Am. 2020;104(5):873–884.
- Stein E, Shane E. Secondary osteoporosis. Endocrinol Metab Clin North Am. 2003;32(1):115–134.
- Harper KD, Weber TJ. Secondary osteoporosis. Diagnostic considerations. Endocrinol Metab Clin North Am. 1998;27(2):325–348.
- Gennari L, Bilezikian JP. Osteoporosis in men. Endocrinol Metab Clin North Am. 2007;36(2):399–419.
- Napoli N, Chandran M, Pierroz DD, et al. Mechanisms of diabetes mellitus-induced bone fragility. Nat Rev Endocrinol. 2017;13(4):208–219.
- Mohamad NV, Soelaiman IN, Chin KY. A concise review of testosterone and bone health. Clin Interv Aging. 2016;11:1317–1324.
- Golds G, Houdek D, Arnason T. Male hypogonadism and osteoporosis: the effects, clinical consequences, and treatment of testosterone deficiency in bone health. Int J Endocrinol. 2017;2017:4602129.
- Mendoza FA, Le Roux M, Ahmed I. Primary osteoporosis in men: an unmet medical need. Fertil Steril. 2019;112(5):791–798.
- Vanderschueren D, Vandenput L, Boonen S, et al. Androgens and bone. Endocr Rev. 2004;25(3):389–425.
- Clarke BL, Khosla S. Androgens and bone. Steroids. 2009;74(3):296–305.
- Rochira V. Late-onset hypogonadism: Bone health. Andrologia. 2020;8(6):1539–1550.
- Tracz MJ, Sideras K, Bolona ER, et al. Testosterone use in men and its effects on bone health: a systematic review and meta-analysis of randomized placebo-controlled trials. J Clin Endocrinol Metab. 2006;91(6):2011–2016.
- Chin KY, Ima-Nirwana S. The effects of orchidectomy and supraphysiological testosterone administration on trabecular bone structure and gene expression in rats. Aging Male. 2015;18(1):60–66.
- Lee MJ, Ryu HK, An SY, et al. Testosterone replacement and bone mineral density in male pituitary tumor patients. Endocrinol Metab. 2014;29(1):48–53.
- Ryu SJ, Ryu DS, Kim JY, et al. Bone mineral density changes after orchiectomy using a scrotal approach in Rats. Korean J Spine. 2015;12(2):55–59.
- Ryu SJ, Ryu DS, Kim JY, et al. Changes in bone metabolism in young castrated male rats. Yonsei Med J. 2016;57(6):1386–1394.
- Martin-Fernandez M, Garzon-Marquez FM, Diaz-Curiel M, et al. Comparative study of the effects of osteoprotegerin and testosterone on bone quality in male orchidectomised rats. Aging Male. 2018;2018:1–13.
- Yeh PS, Lee YW, Chang WH, et al. Biomechanical and tomographic differences in the microarchitecture and strength of trabecular and cortical bone in the early stage of male osteoporosis. PLoS One. 2019;14(8):e0219718.
- de Paiva Goncalves V, Ortega AAC, Steffens JP, et al. Long-term testosterone depletion attenuates inflammatory bone resorption in the ligature-induced periodontal disease model. J Periodontol. 2018;89(4):466–475.
- Callies F, Kollenkirchen U, von Zur MC, et al. Testosterone undecanoate: a useful tool for testosterone administration in rats. Exp Clin Endocrinol Diabetes. 2003;111(4):203–208.
- Steffens JP, Coimbra LS, Rossa C, Jr, et al. Androgen receptors and experimental bone loss – an in vivo and in vitro study. Bone. 2015;81:683–690.
- Rosales Rocabado JM, Kaku M, Nozaki K, et al. A multi-factorial analysis of bone morphology and fracture strength of rat femur in response to ovariectomy. J Orthop Surg Res. 2018;13(1):318.
- Tajul Ariff AS, Soelaiman IN, Pramanik J, et al. Effects of eurycoma longifolia on testosterone level and bone structure in an aged orchidectomised rat model. Evid Based Complement Alternat Med. 2012;2012:818072.
- Prakasam G, Yeh JK, Chen MM, et al. Effects of growth hormone and testosterone on cortical bone formation and bone density in aged orchiectomized rats. Bone. 1999;24(5):491–497.
- Shuid AN, El-Arabi E, Effendy NM, et al. Eurycoma longifolia upregulates osteoprotegerin gene expression in androgen- deficient osteoporosis rat model. BMC Complement Altern Med. 2012;12(1):152.
- Ramli R, Khamis MF, Shuid AN. Bone Micro-CT assessments in an orchidectomised rat model supplemented with eurycoma longifolia. Evid Based Complement Alternat Med. 2012;2012:501858.
- Keller TS, Mao Z, Spengler DM. Young’s modulus, bending strength, and tissue physical properties of human compact bone. J Orthop Res. 1990;8(4):592–603.
- Vandenput L, Boonen S, Van Herck E, et al. Evidence from the aged orchidectomized male rat model that 17beta-estradiol is a more effective bone-sparing and anabolic agent than 5alpha-dihydrotestosterone. J Bone Miner Res. 2002;17(11):2080–2086.
- Jamsa T, Jalovaara P, Peng Z, et al. Comparison of three-point bending test and peripheral quantitative computed tomography analysis in the evaluation of the strength of mouse femur and tibia. Bone. 1998;23(2):155–161.
- Vanderschueren D, Van Herck E, Suiker AM, et al. Bone and mineral metabolism in aged male rats: short and long term effects of androgen deficiency. Endocrinology. 1992;130(5):2906–2916.
- Zhang G, Wang S, Xu S, et al. The effect of formalin preservation time and temperature on the material properties of bovine femoral cortical bone Tissue. Ann Biomed Eng. 2019;47(4):937–952.
- Fiedler IAK, Casanova M, Keplinger T, et al. Effect of short-term formaldehyde fixation on raman spectral parameters of bone quality. J Biomed Opt. 2018;23(11):1–6.
- Schmidt C, Priemel M, Kohler T, et al. Precision and accuracy of peripheral quantitative computed tomography (pQCT) in the mouse skeleton compared with histology and microcomputed tomography (microCT). J Bone Miner Res. 2003;18(8):1486–1496.
- Kanis JA, Johnell O, De Laet C, et al. A Meta-analysis of previous fracture and subsequent fracture risk. Bone. 2004;35(2):375–382.
- Borst SE, Conover CF, Carter CS, et al. Anabolic effects of testosterone are preserved during inhibition of 5alpha-reductase. Am J Physiol Endocrinol Metab. 2007;293(2):E507–14.
- Borst SE, Lee JH, Conover CF. Inhibition of 5α-reductase blocks prostate effects of testosterone without blocking anabolic effects. Am J Physiol Endocrinol Metab. 2005;288(1):E222–7.