Abstract
Estradiol (E2) is women’s dominant ‘bone hormone’ since it is essential for development of adolescent peak bone mineral density (BMD) and physiological levels prevent the rapid (3-week) bone resorption that causes most adult BMD loss. However, deceasing E2 levels trigger bone resorption/loss. Progesterone (P4) is E2’s physiological partner, collaborating with E2 in every cell/tissue; its bone ‘job’ is to increase P4-receptor-mediated, slow (3–4 months) osteoblastic new bone formation. When menstrual cycles are normal length and normally ovulatory, E2 and P4 are balanced and BMD is stable. However, clinically normal cycles commonly have ovulatory disturbances (anovulation, short luteal phases) and low P4 levels; these are more frequent in teen and perimenopausal women and increased by everyday stressors: energy insufficiency, emotional/social/economic threats and illness. Meta-analysis shows that almost 1%/year spinal BMD loss occurs in those with greater than median (∼31%) of ovulatory disturbed cycles. Prevention of osteoporosis and fragility fractures requires the reversal of stressors, detection and treatment of teen-to-perimenopausal recurrent cycle/ovulatory disturbances with cyclic oral micronized progesterone. Low ‘Peak Perimenopausal BMD’ is likely the primary risk for fragility fractures in later life. Progesterone plus estradiol or other antiresorptive therapies adds 0.68%/year and may be a highly effective osteoporosis treatment. Randomized controlled trials are still needed to confirm progesterone’s important role in women’s bone formation.
Introduction
Bone is a complex, adaptable tissue with a protein ‘matrix’ that becomes strong with mineralization. Each bone, of over 200 in our bodiesCitation1, grows to its particular size, shape and structure for its role(s) in mobility, protection of vital organs and emergency calcium availability. The two main types of bone – cancellous and cortical – have different roles and rates of turnover. The calcium stored in cancellous bone (honeycomb-like bone in the center of vertebrae and long bones) is essential to catalyze heart, nerve, brain, and muscle functions. Dense outer cortical bone of limbs and others is necessary for strength.
Bone tissues renew/renovate through a complex ‘remodeling’ process across the lifespan. Bone adaptably responds to nutritional inputs, gravity, exercise and hormonal signals; its bone-negative responses to illness, pain and weight loss emergencies are kept short. The overall lifecycle bone health goal is to build a large, well-mineralized and strong skeleton (bone mineral density, BMD), so age-related normal losses will not cause osteoporosis, manifest by fragility fractures (bone breaks with ≤ force of a fall from a standing height).
Both estradiol (E2, ‘women’s hormone’) and progesterone (P4), E2’s ovarian partner hormone, play essential roles in bone balance. E2 slows bone resorption and P4 stimulates bone formationCitation2. That P4 is important for bone is controversial. One reason is that E2 prevents bone resorption, the primary reason for BMD lossCitation3 (Box 1). But bone remodeling/renovation is a two-part, balanced process; bone resorption is fast while formation is slow. The balance in bone remodeling during the menstruating years likely determines lifelong bone health (Box 1).
The purposes of this review are to describe the E2–P4 partnership in normal bone, review P4’s practical and clinical roles in osteoporosis prevention and added to antiresorptive therapies for treatment of increased fragility fracture risk. To understand P4 and bone health, we need to understand women’s ‘bone renovation’, remodeling, system (Box 1). It is also initially necessary to appreciate bone’s changes across the reproductive phases of women’s life cycle ().
Figure 1. This theoretical diagram describes the ideal ‘life cycle’ of lumbar spine (L1–4) areal bone mineral density (BMD). It initially shows bone modeling-related gains through childhood and adolescenceCitation10; following peak BMD during the menstruating (premenopausal) years, remodeling ideally has a balance of resorption and formationCitation17,Citation21; during perimenopause following a skipped period, bone resorption overbalances bone formation with life’s most rapid normal bone lossCitation26; menopause (starting 1 year after last menstruation) initially has rapid BMD loss that becomes more gradual with healthy agingCitation32; when frailty begins in older ages, rapid bone loss again occursCitation32 and fragility fractures increaseCitation35.
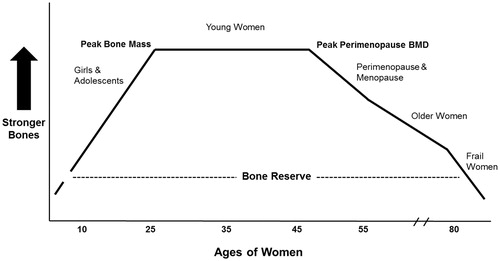
Integrating women’s reproduction with the bone life cycle
shows idealized spinal BMD changes over time and will serve as a guide through the description of women’s reproductive lifespan. Since ovarian hormone patterns vary across menstrual cycles and across life phases, and E2 and P4 strongly influence bone health, it is necessary to understand how key hormones relate to bone remodeling (Box 2).
Box 1 Bone renovation.
The five main phases of women’s life cycle (adolescence, premenopause, perimenopause, menopause, frail aging, ) differ in normal rates of bone change due to differences in women’s ‘bone renovation system’.
Adult bone is renovated (within small packets of bone throughout the body called ‘bone mineralization units’, BMU) through a two-phase, unidirectional process of initial removal of old bone called bone resorption; this is followed by replacement with new osteoid (bone matrix) in bone formation followed by its mineralization.
Women’s bone grows to ‘peak bone mass’ in adolescence through ‘modeling’ in which biomarkers for both resorption and formation are elevated but formation is not preceded by resorption.
The two phases of bone renovation differ in timing and cellular actors. Resorption is quick (over 3 weeks) and accomplished by bone marrow osteoclast cells. Formation is slow (over 3–4 months) achieved by bone marrow derived osteoblast cells.
The two phases of bone renovation, resorption and formation, are linked together (called ‘coupling’) so when resorption increases, formation also increases. Bone mineral density is neither lost nor gained when resorption and formation are in balance.
Peak bone mineral density
Bones need to grow to the optimal size, shape and strength – this is called ‘modeling’; unlike remodeling in adult bone, modeling does not start with resorptionCitation1. Before birth, in infancy, during childhood and in adolescence, bone grows to optimally suit its skeletal roles. ‘Peak BMD’ that differs by the particular bone as well as by sex and ageCitation4, is key to understanding prevention of fragility fractures. Peak BMD is the densest and strongest bone we ever achieve. For women, peak total hip and femoral neck BMDs accrue during ages 16–19 years in prospective random population dataCitation5; lumbar spine peak BMD accrues during ages 30–40Citation5,Citation6.
Many variables are associated with higher peak BMD. These include geneticsCitation7, age at menarcheCitation8,Citation9, more physical activity/less sedentary timeCitation7, higher calcium/vitamin D3 intakes and less intense eating ‘restraint’ attitudesCitation10, and likely how soon following menarche ovulation and P4 production are establishedCitation11. Evidence is growing that use of combined hormonal contraception (CHC) during adolescenceCitation12,Citation13 and young adulthoodCitation11,Citation14 may interfere with optimal peak BMD accrual, perhaps by suppressing necessary bone turnover.
Box 2 Hormonal roles in the bone remodeling cycle.
Estradiol is the most important hormone for the bone of women since, when it is in moderate or high physiological levels, its role is to decrease bone resorptionCitation3 (through osteoprotegerin osteoblast production, increased intestinal calcium absorption and other mechanisms) and thus to prevent bone loss.
When estradiol levels drop, this triggers a cascade of cytokines (most important being RANK-L) that stimulates a rapid increase in bone resorption.
Progesterone sits on specific osteoblast receptorsCitation41,Citation42 and stimulates new osteoblasts to be made from mesenchymal stem cells (instead of into adipocytes) and also stimulates osteoblasts to create more bone matrixCitation2. It is women’s bone formation-stimulating hormone.
Testosterone is metabolized into dihydrotestosterone in osteoblasts and stimulates bone formation but it is also metabolized into estradiol. It is men’s primary bone formation-stimulating hormone.
Cortisol, the adrenal stress hormone present in both men and women, although absolutely essential for human life, in high levels both increases bone resorption (through inhibiting calcium absorption and central suppression of gonadal steroid production causing declining estradiol levels) and paralyzes bone formation by inhibiting osteoblast progesterone and testosterone effects.
Premenopause
During the primary menstruating years, BMD is ideally maintained (). To understand why and appreciate P4’s role in women’s bone health, we must take a closer look at the normal menstrual cycle. The idealized cycle () shows that, during flow, both E2 and P4 levels are low. However, E2 levels then gradually rise to a mid-cycle peak about 240% higherCitation15 that suppresses bone resorption (Box 2). However, E2 then declines, increasing bone resorption. The E2 peak stimulates the luteinizing hormone (LH) peak that triggers ovulation/egg release and luteal onset. With ovulation, the ovarian follicle cells that initially made E2 are transformed to also make P4. Unlike our ‘cultural picture’ based on the unit-less diagrams in Google, P4 rises to a high peak that is 1400% above its flow nadirCitation15 (). E2 is in pmol/l and P4 in the 1000-fold higher nmol/l units.
Figure 2. (A) A simple, idealized depiction of estradiol (solid line) and progesterone (dashed line) changes occurring across the normal menstrual cycle as depicted by the common unit-less diagrams that are readily available. (B) The percentage changes from follicular-phase low levels in estradiol and in progesterone that illustrate their relative changes across the normally ovulatory menstrual cycle. Redrawn from a diagram by NielsenCitation15.
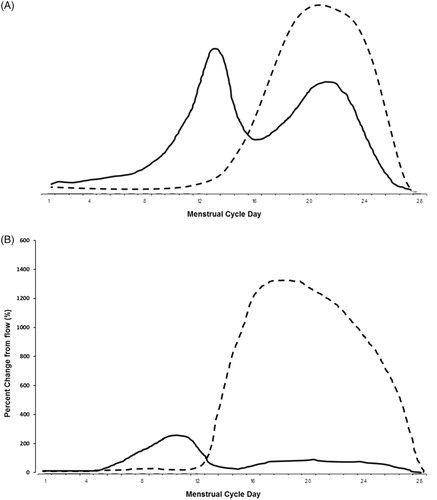
The normally ovulatory cycle provides bone balance because, as E2 levels rise, bone resorption is inhibited (Box 2). This is followed by a P4 peak that stimulates bone formation. Although the decreasing E2 levels from peak to menstrual flow trigger a small increase in bone resorption (Box 2)Citation16, P4’s bone formation–stimulation counter-balances that to result in a net, stable BMDCitation17,Citation18. For optimal P4-related bone formation, however, the ovulatory cycle appears to need a luteal phase length of 10–14 days by quantitative basal temperatureCitation19,Citation20 or 12–16 days by LH peakCitation21.
That silent ovulatory disturbances occurring within clinically normal cycles are related to BMD loss was first shown in a prospective, observational 1-year study in 66 women. These women were pre-screened for normal cycle/luteal phase lengths in two consecutive cycles (); in addition, the women were of normal/stable weight, non-smokers and aged 20–42 yearsCitation17. Those who continued to be normally ovulatory or experienced only one short luteal cycle per year maintained cancellous BMD. However, those with one or more short luteal phases or any anovulatory cycles significantly lost BMD at rates of 4–6%/yearCitation17.
Figure 3. Cancellous spinal bone mineral density (BMD) changes over 1 year by year-long ovulatory characteristics within regular, normal-length menstrual cycles in 66 weight-stable, healthy, initially normally cycling and ovulatory premenopausal women. BMD change in the solid bar (n = 13) includes women with all normally ovulatory cycles; the slant-hatched bar (n = 12) includes women with one short luteal phase/year; the stippled bar (n = 28) includes women with two or more short luteal phase cycles/year; the cross-hatched bar (n = 13) shows women with any anovulatory cycles. Reprinted with permission from the New England Journal of MedicineCitation17.
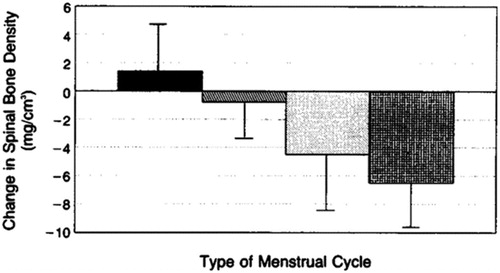
Premenopausal cycles in population-based women older than 25 years are usually predictable, about 3–5 weeks apartCitation22; ovulatory characteristics are not so stable. A prospective 2-year study in 123 healthy, regularly cycling, young adult women showed that an average of 39% of their cycles in 1 year were ovulatory-disturbedCitation21. Examined by higher vs. lower proportion of ovulatory disturbances, spine and hip BMD changes differed significantly (). A population-based study that measured P4 and E2 on a single day in a random cycle showed these cycle-timed values indicated anovulation over one-third of the time in more than 3000 women aged 20–49 years (mean 42 years)Citation23.
Finally, a meta-analysis of spinal BMD change that monitored 436 women aged 18–422 years for cycles and ovulation, showed that, within each study, those with less than a median proportion of normally ovulatory cycles were losing almost 1% (−0.86%) of spinal BMD/yearCitation24 (). Therefore, over the 30–45 years of menstruating life, if an ideal peak BMD is not gainedCitation4,Citation6,Citation11 or if BMD is lost at −0.86%/year, a woman may reach the time of rapid bone loss in late perimenopause (’Peak Perimenopausal BMD’) thinking she has strong bones but having already lost more than 10–20% ().
Figure 4. Mean 2-year areal bone mineral density (BMD) changes (dual-energy X-ray absorptiometry) by whether women had higher (striped bars) or lower (solid bars) experiences of subclinical ovulatory disturbances, based on a median split (39% of all cycles), within regular menstrual cycles (∼30 days) in 123 women ages 20–35 (average 22 years). BMD % change data shown as mean ± SEM adjusted for: change in lean body mass in kg, baseline gynecological age and body mass index (kg/m2)Citation21. Significant differences: *p = 0.034; **p = 0.001. Drawn from data in this publicationCitation21.
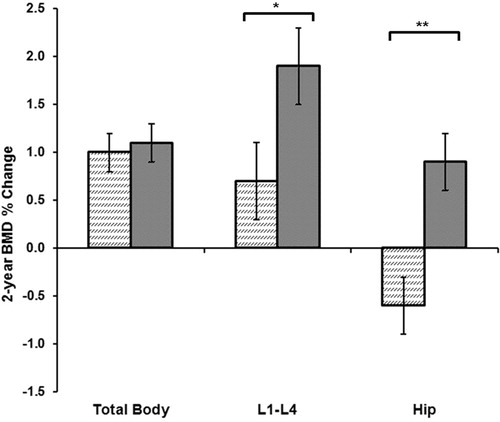
Figure 5. Forest plot from a meta-analysis of six prospective, observational studies in 436 premenopausal women tracking 1-year menstrual cycles, ovulatory characteristics and percentage changes in bone mineral density (BMD). Results show a highly heterogeneous (I2 = 80.2%) random effects model with -0.86%/year more spinal BMD loss in those with worse ovulatory disturbancesCitation24. Reprinted with permission of Epidemiologic ReviewsCitation24.
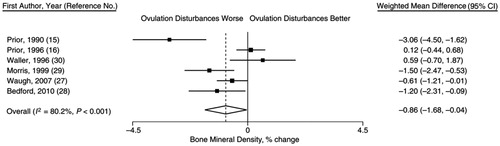
Perimenopause
Perimenopause, a long transitional between premenopause and menopause, is characterized by major hormonal changes: (1) higher, erratic E2 levelsCitation25 and decreasing ovulatory P4 levelsCitation26 with increased ovulatory disturbancesCitation27,Citation28; (2) hypothalamic–pituitary–ovary hormonal feedback disturbancesCitation26,Citation28; and (3) increased inflammatory markerCitation29 and stress hormone levelsCitation30. All of these changes (Box 2) are negative for bone balance. In perimenopause (), rapid bone loss beginsCitation26 in the late menopausal transitionCitation31 (with the first skipped cycle). Perimenopausal spinal BMD loss rates exceed those in early menopause (−1.83 ± 4.49% vs. −1.22 ± 3.14%; p = 0.005)Citation26,Citation32, as confirmed in prospective, population dataCitation32. Given that perimenopausal E2 levels are significantly higherCitation26, high bone resorption must relate to repeatedly declining E2 levelsCitation25 plus disturbed ovulationCitation28 and decreased bone formationCitation33 (Box 2)Citation26,Citation32,Citation34.
Menopause
The former belief that rapid bone loss was due to menopause-related ‘estrogen deficiency’ explained women’s greater fragility fracture rates versus menCitation35. As already discussed, peak BMD levelsCitation4, young adulthoodCitation24 and perimenopausalCitation32 rates of BMD change all contribute to BMD/bone strength at ‘Peak Perimenopausal BMD’ (). The influence of these earlier life bone factors on incident menopausal fragility fractures is not yet known. The rate of menopausal bone loss slows gradually () until it averages about −0.5%/year while women remain healthy, mobile and without quality-of-life-altering diseasesCitation32. Note, this slowed BMD loss rate occurs despite years of low E2 and P4 levels.
Rapid menopausal bone loss begins againCitation32, however, and increased fractures occur when women become frail in older adulthoodCitation36; frailty and rapid bone loss are often triggered by a critical illnessCitation37. We also know that major osteoporotic fractures (fragility fractures of hip, clinical spine, forearm and humerus) are associated with increased rates of bone lossCitation38. Again, according to , the level of BMD/bone strength at onset of frailty in elderly women is related to preceding peak BMD and subsequent losses prior to menopause onset.
Progesterone for prevention of bone loss in adolescent, pre- and perimenopause
Oral micronized progesterone (progesterone) that is identical to ovarian luteal-phase P4 production is now available for cyclic therapyCitation39. However, progesterone therapy only became available in France in the 1980sCitation39 and North America in the mid-1990s. Before that time, synthetic progestins were used in lieu of progesterone. However, progestins have varying chemical structures and such heterogeneous effects on other tissues that they have no non-reproductive class effectsCitation40. Since progestins only need to transform a proliferative to secretory endometrium and preserve a pregnancy to be so classified, before using them in lieu of progesterone we must ensure that they act like progesterone in bone. It has been proven that medroxyprogesterone acetate (MPA) in vitro activated the osteoblast P4 receptor causing increased osteoblast cell maturation from mesenchymal stem cellsCitation41. MPA also, especially in estrogen-co-therapy (or likely with a longer cell culture exposure) increased the differentiation of osteoblast cells into those making alkaline phosphataseCitation41 associated with bone matrix formation. Only osteoblasts from female rats, however, were P4-responsiveCitation42.
Although cyclic progesterone may be appropriate therapy, in every situation a woman needs to be holistically assessed for the other important general and bone health variables such as overall nutritionCitation43, body mass index (BMI, kg/m2), adequacy of energy intake for energy expenditureCitation44, proteinCitation45, calcium and vitamin DCitation46 intakes, sedentary behaviorCitation47 or physical activityCitation9, adequacy of sleep and circadian rhythmCitation48, and the psychosocial environment to rule out stress, threat, abuse, depressionCitation49 and cognitive dietary restraintCitation21 (). This section will discuss life phases before menopause during which cyclic progesterone may prevent osteoporosis.
Adolescent women at risk for osteoporosis
The years from menarche to 20 years (adolescence) include both a reproductive transition (from childhood to reproductive status)Citation50 and a bone health transition to achieve the majority of peak BMDCitation4–6. Early menarche (age ≤11 years) in the population is associated with a higher adult weight/obesityCitation51, a normal adult BMD, and with increased mortalityCitation52. It is likely that maturation to ovarian E2 production exceeds the development of ovulation and P4 release for those with early menarcheCitation53.
If an adolescent experiences hypothalamic oligo- or amenorrhea, as 1–4% in the population mayCitation54,Citation55, then cyclic progesterone (in a luteal-phase equivalent doseCitation56 of 300 mg at bedtime for 14 days) is an ideal therapy. In amenorrhea, it is given 14 days on and 14 days off. In regular cycles, it is best on cycle days 14–27. Although no data on oligo-/amenorrhea, long-term, cyclic progesterone treatment are available in adolescents, a cyclic MPA randomized controlled trial (RCT) (discussed below) showed 50% 1-year menstrual cycle/ovulatory recoveryCitation57. Cyclic progesterone information for women/health-care providers is available (http://www.cemcor.ca/resources/cyclic-progesterone-therapy). If an adolescent with early menarche is overweight, taking cyclic progesterone may assist in weight normalization because weight-stable women eat an additional ∼300 kilocalories/day during the luteal phaseCitation58; P4 requires energy for its temperature-increasing action.
Although secondary amenorrhea is rare in the populationCitation55, oligomenorrhea is not uncommonCitation54 and both are associated with bone loss during a time of expected bone gainCitation16,Citation59 or with increased fracture riskCitation60. Increasingly we understand that hypothalamic reproductive disturbances relate to one or more ‘threats’ and central stress responsesCitation61. They may involve loss of menstrual cycles (amenorrhea) or longer than 35-day cycle intervals (oligomenorrhea), and these are associated with lower E2 and P4 productionsCitation62. Short luteal phases and anovulation within regular, normal-length cycles (called ‘subclinical ovulatory disturbances’) involve low P4 but normal E2 levels; they are also usually hypothalamic stress-related and reversibleCitation62,Citation63. Although one might think, as clinicians often doCitation64, that those with hypothalamic cycle/ovulatory disturbances require estradiol ‘replacement’, those adolescent/young women treated with supraphysiological estrogen with progestin in CHC experienced half the rate of cycle recovery as those who were untreated or prescribed menopausal-like hormone therapyCitation65.
Premenopausal women at risk for negative bone changes
Subclinical ovulatory disturbances are common in the population, are reversibleCitation63 and related to negative BMD effectsCitation17,Citation21,Citation23,Citation24. In confirmation, a cross-sectional, population-based study in over 500 premenopausal women found that those with BMD values in the lowest 10th percentile had significantly lower P4 and occasional subnormal E2 levels versus those with normal BMD levelsCitation66. Therefore, it is logical to provide cyclic progesterone ‘replacement’ therapy for women with hypothalamic cycle/ovulatory disturbances.
In the 1990s, we recruited a cohort of 73 healthy, non-smoking, normal-weight women aged 21–45 years (mean 32 years) with hypothalamic disturbances of cycle length (amenorrhea, oligomenorrhea) or ovulation (anovulation and recurrent short luteal phases) to a 1-year randomized, double-blind, placebo-controlled 2 × 2 factorial trial of cyclic MPA (prior to oral micronized progesterone availability) and/or supplemental calciumCitation57. Sixty-one women completed the study; there were no serious adverse effectsCitation57. Women were stratified by reproductive disturbances into four groups treated with cyclic MPA (10 mg for 10 days/month or cycle) and calcium carbonate (500 mg twice daily) or their respective placebos () with 14–16 women/groupCitation57. The primary outcome of spinal BMD change showed significant +2–3% annual mean gains in cyclic MPA groups, no change on calcium alone and a significant −2% loss on double placebo; 2 × 2 analysis of variance, MPA: F = 19.43, p = 0.0001; calcium: F = 3.34, p = 0.073 without significant interaction ()Citation57. Thus MPA acting through the P4 osteoblast receptor caused a significant annual gain in spinal BMD in active women with hypothalamic disturbances, ranging from amenorrhea to regular cycles with repeated short luteal phases. During the cycle following experimental treatment, almost half had recovered perfectly normal cycle and luteal-phase lengthsCitation57. Note that this study used 10 mg of MPA cyclically which, unlike Depo-MPA (150 mg intramuscularly every 3 months), is not high enough to either suppress endogenous E2 levels (causing increased bone resorption, Box 2) or to act negatively on bone formation through the glucocorticoid receptor.
Figure 6. This dot-plot depicts individual spinal bone mineral density BMD changes/year in 61 healthy, normal-weight, active, non-smoking women ages 21–45 stratified by their hypothalamic menstrual cycle (amenorrhea, oligomenorrhea) or ovulatory disturbances within normal cycles (anovulation, short luteal phases) into in a randomized, double-blind, placebo-controlled 2 × 2 factorial design trial of cyclic medroxyprogesterone (MPA, 10 mg/day, 10 days/month or cycle) or supplemental calcium (500 mg twice/day). Groups by therapy: A = cyclic MPA, calcium; B = cyclic MPA, calcium placebo; C = MPA placebo, calcium; D = placebos for MPA, calcium. The short horizontal line is the group mean. With permission from the American Journal of MedicineCitation57.
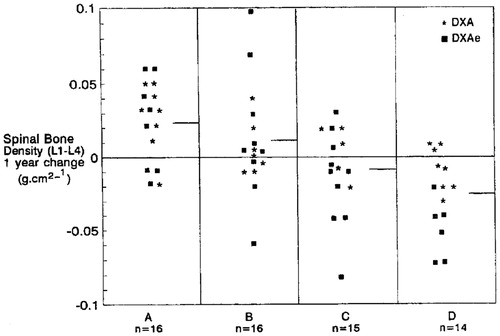
Perimenopausal women at risk for osteoporosis
Almost no bone-therapy studies are available in perimenopause. There is some observational evidence that CHC use may prevent femoral BMD loss; weekly nitrogen bisphosphonate (alendronate 70 mg/week) plus vitamin D may prevent spine and femoral neck but not total hip BMD lossesCitation67. Neither of these therapies is proven safe in perimenopause since perimenopausal CHC risks for thromboembolic disease are at least tripled and bisphosphonates are contraindicated in potentially fertile women. To our knowledge, cyclic or daily progesterone therapy has not been tested in perimenopausal women at risk for osteoporosis.
Progesterone for osteoporosis treatment in menopausal women
Following our understanding of bone remodeling (Box 1) and the complementary bone roles played by E2 and P4 (Box 2), to increase BMD and prevent fracture it is likely that co-treatment would be optimalCitation68. A comparative 1-year RCT tested daily conjugated equine estrogen (CEE, 0.6 mg/day) vs. MPA (10 mg/day) in 41 women randomized at hospital discharge (∼1 week) after premenopausal surgical menopauseCitation69. At study onset, the N-telopeptide (bone resorption marker) was already ≥2 standard deviations above premenopausal normsCitation69. Significant cancellous spinal BMD loss occurred on CEE and MPA and was marginally greater on MPA (p = 0.04)Citation69. CEE, however, prevented significant BMD loss at the whole body and femoral neck sites but MPA did notCitation69. Thus the formation effects of MPA or progesterone can increase BMD only when resorption levels are normal; they do not suppress the resorption ‘driver’ of bone loss (Box 1).
Menopausal hormone therapy (MHT) is estrogen-based; current guidelines instruct that only menopausal women without a hysterectomy be treated with added progestin/progesteroneCitation70. Estrogen alone, estrogen with progestin, and MPA/progesterone alone all effectively improve hot flushes and night sweatsCitation71. Therefore we need to know: does BMD change differ during treatment with estrogen alone or estrogen–progestin/progesterone? Our recent meta-analysis of RCTs that directly randomized more than 1000 menopausal women (mean age 55 years) to estrogen alone or estrogen–progestin/progesterone, while measuring 1-year spinal BMD change says it does ()Citation72. CEE (0.625 mg/day) was the usual estrogen and MPA (2.5 mg/day) the progestin. Of the five RCTs, eight dose-paired comparisons were analyzed by weighted mean percent BMD difference in a random effects modelCitation72. Results showed a significantly greater BMD gain (+0.68%/year; 95% CI 0.38–0.97%) (p = 0.00001) on CEE plus MPACitation72. Thus MPA added to the BMD and likely bone strength gains from estrogen alone.
Figure 7. Meta-analysis of spinal areal spinal bone mineral density (BMD) changes in five controlled comparative trials that randomized more than 1000 menopausal women directly to estrogen therapy alone (ET) or estrogen–progestin therapy (EPT) without regard to hysterectomy status. The weighted mean difference in a random effects model of BMD change is reported as g/cm2 over 1 yearCitation72. Reprinted with permission from the Journal of Musculoskeletal and Neuronal InteractionsCitation72.
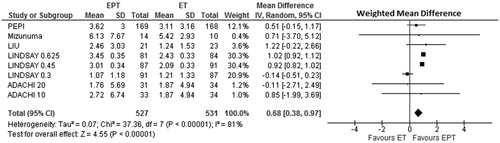
The next logical question is whether progesterone adds to BMD gains when combined with non-estrogen antiresorptive agents. MHT’s risks may outweigh its benefitsCitation73 and estrogen plus MPA (but not estradiol plus progesterone) is associated with significantly increased breast cancer risk, as indicated by the longitudinal observational studies in menopausal women in the French E3N studyCitation74. The only available data on this question are from a random sample of 19 clinical charts of women with osteoporosis treated with a non-nitrogen bisphosphonate plus MPA/progesteroneCitation75. Over 2% annual spinal BMD gain on etidronate plus MPA/progesterone (+2.57; 95% CI 1.85–3.54) was higher than with etidronate alone from an RCT meta-analysis (+1.42; 95% CI 0.89–1.32)%/yearCitation75; comparative results for femoral neck BMD on etidronate–MPA (+1.83; 95% CI 0.89–3.56) vs. etidronate alone (+0.73; 95% CI 0.14–1.32)Citation75,Citation76 suggest progesterone would add important BMD benefits to stronger antiresorptive osteoporosis therapies.
RCTs of CEE aloneCitation73 and CEE/MPACitation77 showed fracture prevention in the Women’s Health Initiative hormone therapy trials. However, there are no head-to-head fracture prevention trials of estrogen alone vs. estrogen plus MPA/progesterone or of antiresorptives alone vs. antiresorptives plus bone formation stimulatorsCitation78. Based on the results of a network meta-analysis, parathyroid hormone (PTH, teriparatide) acting primarily as a bone-formation stimulating agent, was more effective at fracture prevention than the strong antiresorptivesCitation78. Increasingly, agents acting on bone formation and resorption are combinedCitation68 since antiresorptives alone suppress bone formation through coupling (Box 1) and are associated with significant long-term risksCitation79.
Treatment for symptomatic menopause with hot flushes is significantly more effective with estrogen–progestin than estrogen aloneCitation80; RCT evidence suggests that progesterone alone will be effective and safeCitation81. Since many menopausal women will have both hot flushes and fracture risks requiring treatment, combined antiresorptive plus progesterone therapy may yield amplified benefitsCitation71.
Conclusion
Progesterone is estradiol’s partner hormone in bone. P4 appears to play important roles in achievement of an ideal peak bone mineral density (BMD) in adolescence and young adulthood and in the prevention of bone loss during pre- and likely perimenopausal life phases, leading to a normal peak perimenopausal BMD. For women with hypothalamic disturbances of cycles and ovulation, cyclic progesterone therapy (based on RCT data with cyclic MPA) is an effective BMD-increasing therapy and may also facilitate reproductive recovery. P4 will likely safely add BMD/strength gains to antiresorptive therapy in menopausal and frail elderly women at increased fracture risk.
Conflict of interest
The author reports no conflict of interest. The author alone is responsible for the content and writing of this paper.
Source of funding
Nil.
Acknowledgements
Thanks to Dharani Kalidasan MSc for creating and enhancing figures as well as facilitating the accomplishment of this review. I appreciate the bone health mentorship I have received over the years from Drs D. Harold Copp, M. Parfitt, S. Wimalawansa and Bert Cameron. Thanks to Besins Healthcare International who has repeatedly, arms-length, provided oral micronized progesterone and placebo for RCTs.
References
- Clarke B. Normal bone anatomy and physiology. Clin J Am Soc Nephrol 2008;3(Suppl 3):S131–9
- Seifert-Klauss V, Prior JC. Progesterone and bone: actions promoting bone health in women. J Osteoporos 2010;2010:845180
- Khosla S, Melton LJ, III, Riggs BL. The unitary model for estrogen deficiency and the pathogenesis of osteoporosis: is a revision needed?. J Bone Miner Res 2011;26:441–51
- Weaver CM, Gordon CM, Janz KF, et al. The national osteoporosis foundation's position statement on peak bone mass development and lifestyle factors: a systematic review and implementation recommendations. Osteoporos Int 2016;27:1281–386
- Berger C, Goltzman D, Langsetmo L, et al. Peak bone mass from longitudinal data: implications for the prevalence, pathophysiology, and diagnosis of osteoporosis. J Bone Miner Res 2010;25:1948–57
- Boot AM, de Ridder MA, van der Sluis IM, et al. Peak bone mineral density, lean body mass and fractures. Bone 2010;46:336–41
- Prior JC, Hitchcock CL, Vigna YM, Seifert-Klauss V. Premenopausal trabecular bone loss is associated with a family history of fragility fracture. Geburtshilfe Frauenheilkd Sci 2016;76:895–901
- Chevalley T, Bonjour JP, Van Rietbergen B, Rizzoli R, Ferrari S. Fractures in healthy females followed from childhood to early adulthood are associated with later menarcheal age and with impaired bone microstructure at peak bone mass. J Clin Endocrinol Metab 2012;97:4174–81
- Gabel L, MacDonald HM, Nettlefold L, McKay HA. Physical activity, sedentary time, and bone strength from childhood to early adulthood: a mixed longitudinal HR-pQCT study. J Bone Miner Res 2017;32:1525–36
- Barr SI, Petit MA, Vigna YM, Prior JC. Eating attitudes and habitual calcium intake in peripubertal girls are associated with initial bone mineral content and its change over 2 years. J Bone Min Res 2001;16:940–7
- Kalyan S, Barr SI, Alamoudi R, Prior JC. Is development of ovulatory cycles in adolescence important for peak bone mass? J Bone Miner Res 2007;22:S494–511
- Polatti F, Perotti F, Filippa N, Gallina D, Nappi RE. Bone mass and long-term monophasic oral contraceptive treatment in young women. Contraception 1995;51:221–4
- Scholes D, Hubbard RA, Ichikawa LE, et al. Oral contraceptive use and bone density change in adolescent and young adult women: a prospective study of age, hormone dose, and discontinuation. J Clin Endocrinol Metab 2011;96:E1380–7
- Brajic TS, Berger C, Schlammerl K, et al. Combined hormonal contraceptives use and bone mineral density changes in adolescent and young women in a prospective population-based Canada-wide observational study. J Musculoskelet Neuronal Interact 2017;in press
- Nielsen HK, Brixen K, Bouillon R, Mosekilde L. Changes in biochemical markers of osteoblastic activity during the menstrual cycle. J Clin Endocrinol Metab 1990;70:1431–7
- Kalyan S, Prior JC. Bone changes and fracture related to menstrual cycles and ovulation. Crit Rev Eukaryot Gene Expr 2010;20:213–33
- Prior JC, Vigna YM, Schechter MT, Burgess AE. Spinal bone loss and ovulatory disturbances. N Engl J Med 1990;323:1221–7
- Prior JC. Progesterone as a bone-trophic hormone. Endocr Rev 1990;11:386–98
- Prior JC, Vigna YM, Schulzer M, Hall JE, Bonen A. Determination of luteal phase length by quantitative basal temperature methods: validation against the midcycle LH peak. Clin Invest Med 1990;13:123–31
- Vollman RF, The menstrual cycle. In: Friedman EA, ed. Major problems in obstetrics and gynecology. Vol 7. 1 ed. Toronto: W.B. Saunders Company; 1977:11–193
- Bedford JL, Prior JC, Barr SI. A prospective exploration of cognitive dietary restraint, subclinical ovulatory disturbances, cortisol and change in bone density over two years in healthy young women. Jcem 2010;95:3291–9
- Munster K, Schmidt L, Helm P. Length and variation in the menstrual cycle–a cross-sectional study from a Danish county. Br J Obstet Gynaecol 1992;99:422–9
- Prior JC, Naess M, Langhammer A, Forsmo S. Ovulation prevalence in women with spontaneous normal-length menstrual cycles–a population-based cohort from HUNT3, Norway. PLoS One 2015;10:e0134473
- Li D, Hitchcock CL, Barr SI, Yu T, Prior JC. Negative spinal bone mineral density changes and subclinical ovulatory disturbances–prospective data in healthy premenopausal women with regular menstrual cycles. Epidemiol Rev 2014;36:137–47
- Hale GE, Hughes CL, Burger HG, Robertson DM, Fraser IS. Atypical estradiol secretion and ovulation patterns caused by luteal out-of-phase (LOOP) events underlying irregular ovulatory menstrual cycles in the menopausal transition. Menopause 2009;16:50–9
- Prior JC. Perimenopause: the complex endocrinology of the menopausal transition. Endocr Rev 1998;19:397–428
- Prior JC, The ageing female reproductive axis II: ovulatory changes with perimenopause. In: Chadwick DJ, Goode JA, eds. Endocrine facets of ageing. 242 edn. Chichester (UK): John Wiley and Sons Ltd;2002:172–86
- Weiss G, Skurnick JH, Goldsmith LT, Santoro NF, Park SJ. Menopause and hypothalamic-pituitary sensitivity to estrogen. JAMA 2004;292:2991–6
- Ishii S, Cauley JA, Greendale GA, et al. C-reactive protein, bone strength, and nine-year fracture risk: data from the Study of Women's Health Across the Nation (SWAN). J Bone Miner Res 2013;28:1688–98
- Ballinger CB, Browning NC, Smith AHW. Hormonal profiles and psychological symptoms in peri-menopausal women. Maturitas 1987;9:235–51
- Harlow SD, Gass M, Hall JE, et al. Executive summary of the stages of reproductive aging workshop +10: addressing the unfinished agenda of staging reproductive aging. Climacteric 2012;15:105–14
- Berger C, Langsetmo L, Joseph L, et al. Change in bone mineral density as a function of age in women and men and association with the use of antiresorptive agents. Can Med Assoc J 2008;178:1660–8
- Seifert-Klauss V, Schmidmayr M, Hobmaier E, Wimmer T. Progesterone and bone: a closer link than previously realized. Climacteric 2012;15(Suppl 1):26–31
- Seifert-Klauss V, Fillenberg S, Schneider H, Luppa P, Mueller D, Kiechle M. Bone loss in premenopausal, perimenopausal and postmenopausal women: results of a prospective observational study over 9 years. Climacteric 2012;15:433–40
- Prior JC, Langsetmo L, Lentle BC, et al. Ten-year incident osteoporosis-related fractures in the population-based Canadian Multicentre Osteoporosis Study - comparing site and age-specific risks in women and men. Bone 2014;71:237–43
- Kennedy CC, Ioannidis G, Rockwood K, et al. A frailty index predicts 10-year fracture risk in adults age 25 years and older: results from the Canadian Multicentre Osteoporosis Study (CaMos). Osteoporos Int 2014;25:2825–32
- Orford NR, Lane SE, Bailey M, et al. Changes in bone mineral density in the year after critical illness. Am J Respir Crit Care Med 2016;193:736–44
- Berger C, Langsetmo L, Joseph L, et al. Association between change in BMD and fragility fracture in women and men. J Bone Miner Res 2009;24:361–70
- Dennerstein L, Spencer-Gardner C, Gotts G, Brown JB, Smith MA. Progesterone and the premenstrual syndrome: a double blind crossover trial. Br Med J 1985;290:1617–21
- Prior JC. Progesterone or progestin as menopausal ovarian hormone therapy: recent physiology-based clinical evidence. Curr Opin Endocrinol Diabetes Obes 2015;22:495–501
- Verhaar HJJ, Damen CA, Duursma SA, Schevens BAA. A comparison of the actions of progestins and estrogens on growth and differentiation of normal adult human osteoblast-like cells in vitro. Bone 1994;15:307–11
- Ishida Y, Heersche JN. Progesterone stimulates proliferation and differentiation of osteoprogenitor cells in bone cell populations derived from adult female but not from adult male rats. Bone 1997;20:17–25
- Langsetmo L, Hanley DA, Prior JC, et al. Dietary patterns and incident low-trauma fractures in postmenopausal women and men aged >/= 50 y: a population-based cohort study. Am J Clin Nutr 2011;93:192–9
- Mountjoy M, Sundgot-Borgen J, Burke L, et al. The IOC consensus statement: beyond the female athlete Triad-Relative Energy Deficiency in Sport (RED-S)). Br J Sports Med 2014;48:491–7
- Langsetmo L, Barr SI, Berger C, et al. Associations of protein intake and protein source with bone mineral density and fracture risk: a population-based cohort study. J Nutr Health Aging 2015;19:861–8
- Ross AC, Manson JE, Abrams SA, et al. The 2011 report on dietary reference intakes for calcium and vitamin D from the institute of medicine: what clinicians need to know. J Clin Endocrinol Metab 2011;96:53–8
- Gebel K, Pont S, Ding D, et al. Patterns and predictors of sitting time over ten years in a large population-based Canadian sample: findings from the Canadian Multicentre Osteoporosis Study (CaMos). Prev Med Rep 2017;5:289–94
- Joseph F, Chan BY, Durham BH, et al. The circadian rhythm of osteoprotegerin and its association with parathyroid hormone secretion. J Clin Endocrinol Metab 2007;92:3230–8
- Calarge CA, Butcher BD, Burns TL, Coryell WH, Schlechte JA, Zemel BS. Major depressive disorder and bone mass in adolescents and young adults. J Bone Miner Res 2014;29:2230–7
- Prior JC. Adolescents' use of combined hormonal contraceptives for menstrual cycle-related problem treatment and contraception: evidence of potential lifelong negative reproductive and bone effects. Women's Reproductive Health 2016;3:73–92
- Harris MA, Prior JC, Koehoorn M. Age at menarche in the Canadian population: secular trends and relationship to adulthood BMI. J Adolesc Health 2008;43:548–54
- Jacobsen BK, Heuch I, Kvale G. Association of low age at menarche with increased all-cause mortality: a 37-year follow-up of 61,319 Norwegian women. Am J Epidemiol 2007;166:1431–7
- Vihko R, Apter D. Endocrine characteristics of adolescent menstrual cycles: impact of early menarche. J Steroid Biochem 1984;20:231–6
- van Hooff MH, Voorhorst FJ, Kaptein MB, Hirasing RA, Koppenaal C, Schoemaker J. Relationship of the menstrual cycle pattern in 14-17 year old old adolescents with gynaecological age, body mass index and historical parameters. Hum Reprod 1998;13:2252–60
- Munster K, Helm P, Schmidt L. Secondary amenorrhoea: prevalence and medical contact–a cross-sectional study from a Danish county. Br J Obstet Gynaecol 1992;99:430–3
- Simon JA, Robinson DE, Andrews MC, et al. The absorption of oral micronized progesterone: the effect of food, dose proportionality, and comparison with intramuscular progesterone. Fertil Steril 1993;60:26–33
- Prior JC, Vigna YM, Barr SI, Rexworthy C, Lentle BC. Cyclic medroxyprogesterone treatment increases bone density: a controlled trial in active women with menstrual cycle disturbances. Am J Med 1994;96:521–30
- Barr SI, Janelle KC, Prior JC. Energy intakes are higher during the luteal phase of ovulatory menstrual cycles. Am J Clin Nutr 1995;61:39–43
- Biller BM, Coughlin JF, Saxe VC, Schoenfeld DA, Spratt DS, Klibanski A. Osteopenia in women with hypothalamic amenorrhea: a prospective study. Obstet Gynecol 1991;78:996–1001
- Cooper GS, Sandler DP. Long-term effects of reproductive-age menstrual cycle patterns on peri- and postmenopausal fracture risk. Am J Epidemiol 1997;145:804–9
- Williams NI, Berga SL, Cameron JL. Synergism between psychosocial and metabolic stressors: impact on reproductive function in cynomolgus monkeys. Am J Physiol Endocrinol Metab 2007;293:E270–6
- Prior JC. Physical exercise and the neuroendocrine control of reproduction. Baillieres Clin Endocrinol Metab 1987;1:299–317
- Prior JC, Ho Yeun B, Clement P, Bowie L, Thomas J. Reversible luteal phase changes and infertility associated with marathon training. Lancet 1982;1:269–70
- Chen R, Bejaei F, Shan Y, Vali T, Prior JC. Health care provider hormonal recommendations for treatment of menstrual-cycle related problems–a vignette-based study. BJPR 2016;10:1–12
- Falsetti L, Gambera A, Barbetti L, Specchia C. Long-term follow-up of functional hypothalamic amenorrhea and prognostic factors. J Clin Endocrinol Metab 2002;87:500–5
- Sowers M, Randolph JF, Crutchfield M, et al. Urinary ovarian and gonadotropin hormone levels in premenopausal women with low bone mass. J Bone Min Res 1998;13:1191–202
- Khan A, Dubois S, Khan AA, et al. A randomized, double- blind, placebo- controlled study to evaluate the effects of alendronate on bone mineral density and bone remodelling in perimenopausal women with low bone mineral density. J Obstet Gynaecol Can 2014;36:976–82
- Eastell R, Walsh JS. Is it time to combine osteoporosis therapies?. Lancet 2013;382:5–7
- Prior JC, Vigna YM, Wark JD, et al. Premenopausal ovariectomy-related bone loss: a randomized, double-blind, one-year trial of conjugated estrogen or medroxyprogesterone acetate. J Bone Miner Res 1997;12:1851–63
- Santen RJ, Allred DC, Ardoin SP, et al. Postmenopausal hormone therapy: an endocrine society scientific statement. JCEM 2010;95(Suppl 1):S1–S66
- Prior JC. Progesterone for treatment of symptomatic menopausal women. Climacteric 2018;21:358–65
- Prior JC, Seifert-Klauss VR, Giustini D, Adachi JD, Kalyan S, Goshtasebi A. Estrogen-progestin therapy causes a greater increase in spinal bone mineral density than estrogen therapy–a systematic review and meta-analysis of controlled trials with direct randomization. J Musculoskelet Neuronal Interact 2017;17:146–54
- Anderson GL, Limacher M, Assaf AR, et al. Effects of conjugated equine estrogen in postmenopausal women with hysterectomy: the Women's Health Initiative randomized controlled trial. JAMA 2004;291:1701–12
- Fournier A, Berrino F, Clavel-Chapelon F. Unequal risks for breast cancer associated with different hormone replacement therapies: results from the E3N cohort study. Breast Cancer Res Treat 2008;107:103–11
- Prior JC, Hitchcock CL. Medroxyprogesterone augments positive bone mineral density effects of cyclic etidronate in menopausal women: pilot data from a random sample of clinical charts of menopausal women with osteoporosis. J Bone Mineral Res 2002;17:S474
- Cranney A, Guyatt G, Krolicki N, et al. A meta-analysis of etidronate for the treatment of postmenopausal osteoporosis. Osteoporos Int 2001;12:140–51
- Cauley JA, Robbins J, Chen Z, et al. Effects of estrogen plus progestin on risk of fracture and bone mineral density: the women's health initiative randomized trial. JAMA 2003;290:1729–38
- Murad MH, Drake MT, Mullan RJ, et al. Clinical review. Comparative effectiveness of drug treatments to prevent fragility fractures: a systematic review and network meta-analysis. J Clin Endocrinol Metab 2012;97:1871–80
- Anagnostis P, Paschou SA, Mintziori G, et al. Drug holidays from bisphosphonates and denosumab in postmenopausal osteoporosis: EMAS position statement. Maturitas 2017;101:23–30
- MacLennan AH, Broadbent JL, Lester S, Moore V. Oral oestrogen and combined oestrogen/progestogen therapy versus placebo for hot flushes. Cochrane Database Syst Rev 2004;(4):CD002978
- Hitchcock CL, Prior JC. Oral micronized progesterone for vasomotor symptoms in healthy postmenopausal women–a placebo-controlled randomized trial. Menopause 2012;19:886–93