Abstract
Cardiovascular disease (CVD) is the leading cause of death in women aged 65 years and older. Sex hormones have been implicated as having a critical role in the evolution of CVD, with the focus mainly on estrogens in women. Available data also indicate that low testosterone blood levels may be detrimental to cardiovascular function in women. At blood concentrations considered normal for premenopausal women, testosterone has favorable effects on blood vessel function (relaxation and contraction), much of which is determined by the endothelial cells that line the inside of blood vessels. Testosterone enhances endothelium-dependent and independent brachial artery vasodilation and has an acute systolic blood pressure-lowering effect in postmenopausal women. Advantageous effects of testosterone in animal models have been seen for myocardial function and cardiac electrical signaling. Human data are mainly limited to observational and mechanistic studies, which mostly demonstrate beneficial effects of testosterone on cardiovascular health. Few studies of testosterone use in women, with cardiovascular endpoints as primary outcomes, have been published.
摘要
心血管疾病(CVD)是65岁及以上女性的主要死因。性激素被认为在CVD的发展中发挥着关键作用, 主要集中在女性体内的雌激素。可用数据还表明, 血中睾酮水平过低可能对女性心血管功能产生不利影响。在孕前女性被认为正常的血浆浓度下, 睾酮对血管功能(舒张和收缩)产生了有利的影响, 其中大部分是由内皮细胞决定的, 这些细胞覆盖在血管内部。睾酮增强依赖于内皮和独立于内皮的肱动脉舒张, 对绝经后女性有降低急性收缩压的作用。在动物模型中观察到睾酮对心肌功能和心脏电信号传导的有益影响。有关睾酮在女性中使用并以心血管终点为主要结果的研究较少。现有的人类数据主要限于观察性和机制研究, 其中大多数研究表明睾酮对心血管健康有益。已发表的涉及睾酮在女性中使用并以心血管为主要终点的研究很少。
Introduction
With the steady increase in the life expectancy of older women, longevity is accompanied by more years lived with disease and disability. Globally, cardiovascular disease (CVD), including ischemic heart disease, heart failure and stroke, is the leading cause of death in women aged 65 years and older [Citation1]. Risk factors for CVD, common to both sexes, include hypertension, hyperlipidemia, diabetes, obesity, smoking and family history. While CVD is more prevalent in men before the age of 50 years, the age-related incidence of CVD increases more steeply in women after age 50 years, with an acceleration in risk, particularly of coronary artery disease (CAD), around the age of menopause [Citation2]. Sex hormones have been implicated as having a critical role in the development and evolution of age-associated disease, with the focus in women mainly on estrogens. However, in women, testosterone, the main female androgen, is an essential precursor for estrogen production and exerts important physiological effects in its own right. The role of endogenous testosterone in the pathogenesis of ischemic and non-ischemic CVD and cardiovascular death remains uncertain, although available data suggest that testosterone may exert protective cardiovascular effects. This article reviews the available evidence for the associations between testosterone and cardiovascular health.
Overview of androgen physiology and changes with age
Traditionally androgens are defined as 19-carbon (C19) steroid hormones associated with maleness and the induction of male secondary sexual characteristics, specifically testosterone and its more potent metabolite dihydrotestosterone (DHT). Blood levels of DHT are low or undetectable in postmenopausal women [Citation3], as in the cells in which it acts, DHT is broken down into metabolites that are then released into the circulation () [Citation4].
Figure 1. Testosterone biosynthesis. AKR1C3, aldo-keto reductase 1, member C3; DHEA, dehydroepiandrosterone; DHEAS, DHEA sulfate; DHT, dihydrotestosterone; HSDB32, β-hydroxysteroid dehydrogenase; SULT2A1, sulfotransferase 2A.
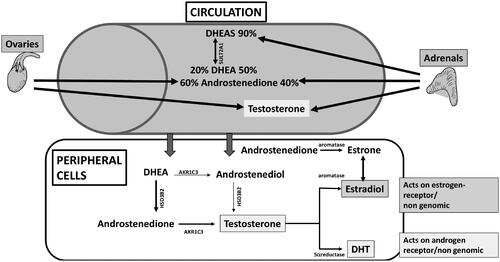
The pro-hormones that are the precursors of testosterone production, dehydroepiandrosterone (DHEA) and androstenedione, are made by both the adrenals and the ovaries [Citation4]. DHEA sulfate (DHEAS) is also made by the adrenals and acts as a reservoir for peripheral DHEA availability [Citation4]. In terms of tissue exposure to androgens, the pro-hormones of adrenal and ovarian origin can be converted in extra-gonadal target tissues such as the brain, bone and adipose either by aromatization to estrone or by 5α-reduction to testosterone, with the latter being converted to either estradiol or DHT in the same cells () [Citation5]. Thus, tissue sensitivity to androgens will vary according to the amount and activity of the enzymes 5α-reductase and aromatase, which may vary considerably between individuals and between tissues, and tissue androgen receptor sensitivity. Thus, even with highly sensitive assays for the measurement of testosterone and DHT such as liquid or gas chromatography with tandem mass spectrometry (LCMS/GCMS), blood concentrations only provide an indication of androgen availability, but not an absolute measure of tissue exposure or tissue sensitivity and responsiveness. This is a major limitation of observational data pertaining to the role of androgens in the cardiovascular system.
Figure 2. Testosterone (T) declines 25% premenopause [Citation10] but increases 11% between age 70 and 85+ years [Citation3]. *p ≤ 0.05, **p ≤ 0.01, adjusted for age and body mass index; premenopause also adjusted for menstrual cycle phase. Modified from Skiba et al. 2019 [Citation10] and Davis et al. 2019 [Citation3].
![Figure 2. Testosterone (T) declines 25% premenopause [Citation10] but increases 11% between age 70 and 85+ years [Citation3]. *p ≤ 0.05, **p ≤ 0.01, adjusted for age and body mass index; premenopause also adjusted for menstrual cycle phase. Modified from Skiba et al. 2019 [Citation10] and Davis et al. 2019 [Citation3].](/cms/asset/d0a0b055-755e-4d8a-991c-d7a49a1f373a/icmt_a_2250252_f0002_b.jpg)
Approximately two-thirds of blood testosterone is bound to sex hormone binding globulin (SHBG), almost one-third is weakly bound to albumin and minor amounts are weakly bound to other plasma proteins or circulate unbound in plasma [Citation6,Citation7]. Evidence that the biologically active fraction of testosterone is the 2–3% not bound to plasma proteins is lacking. It has been suggested that unbound testosterone is the most readily degradable fraction, and therefore the least metabolically active component of blood testosterone [Citation8]. In addition, the measurement of unbound testosterone is complicated by the poor precision and low specificity of most methods, and the formulae commonly used to estimate the unbound fraction lack validity and provide inconsistent results [Citation7]. An international panel concluded that total testosterone, hereafter referred to as testosterone, remains the best estimate of testosterone exposure in women [Citation9].
Using LCMS, testosterone and androstenedione concentrations have been shown to vary across the menstrual cycle, peaking midcycle, whereas DHEA concentrations do not change meaningfully during the menstrual cycle [Citation10]. Serum concentrations of all C19 steroids decline between 25% and 35% with age during the reproductive years [Citation10], do not appear to acutely change at menopause [Citation11,Citation12], but reach a nadir during the early sixth decade [Citation11,Citation13]. After this age, testosterone blood levels tend to increase in women. Consequently, testosterone concentrations in women age 70 years and older have been found to be similar to the concentrations seen in premenopausal women () [Citation3,Citation13,Citation14]. The apparent increase in testosterone beyond the age of 70 years does not appear to be simply a survivor effect as we have shown that this increase occurred in paired samples of serum from individuals taken 3 years apart [Citation15].
Mechanistic studies of cardiovascular effects of testosterone
Vascular endothelial dysfunction plays a role in the pathogenesis of hypertension and atherosclerosis [Citation16]. Estrogen insufficiency has been shown to result in loss of vascular endothelial function in premenopausal [Citation17] and postmenopausal [Citation18] women. Estrogen therapy increases endothelial nitric oxide production via genomic and non-genomic mechanisms [Citation19], and this restores endothelial function and vascular reactivity in postmenopausal women [Citation20]. This effect declines with age and years since menopause [Citation21]. Endogenous testosterone was positively associated with vascular endothelial function in postmenopausal women with a mean age of 56.9 years and on average 9.4 years postmenopause [Citation22]. Similar findings have been reported for endogenous testosterone in surgically menopausal women who were up to 10 years postmenopausal [Citation23]. Exogenous parenteral testosterone significantly increased endothelial-dependent and independent vasodilation in postmenopausal women, mean age 52 years, stabilized on estrogen therapy [Citation24]. In a mechanistic study, testosterone increased the production of nitric oxide in rat aortic endothelial and smooth muscle cells [Citation25]. These effects were blocked by finasteride, an androgen receptor antagonist, but not by inhibition of the aromatase enzyme, which converts testosterone to estradiol [Citation25]. This indicates an androgen receptor-mediated effect of testosterone on endothelial nitric oxide production or release. Testosterone also appears to have an endothelium-independent vasodilatory effect, possibly via direct non-genomic actions [Citation24,Citation26,Citation27]. When administered to postmenopausal women, testosterone causes acute vasodilation, evidenced by one study showing a mean drop in systolic blood pressure of 10 mmHg in the first 5 min post dose across a range of doses [Citation28]. This rapid effect supports a non-genomic effect of testosterone on vascular function.
The effects of testosterone on myocardial ischemia have been studied in mouse and rat models. Cardiac myocytes have aromatase and can make estradiol from testosterone and androstenedione [Citation29]. The expression of estrogen receptor (ER)-α differs between males and females whereas ER-β does not exhibit sexual dimorphism [Citation29]. In a mouse model, androstenedione and testosterone are not only precursors for estradiol biosynthesis, but also stimulate nitric oxide synthetase production, and hence nitric oxide levels, in cardiac myocytes [Citation29].
Aromatase knockout (ArKO) mice cannot make any estrogens, and like other rodents do not make DHEA [Citation30]. Because they cannot convert testosterone to estradiol, ArKO mice have higher testosterone concentrations than wild-type mice. Hence, ArKO mice serve as a model for studying the effects of sex steroids in various systems. The effects of ischemia and reperfusion of the myocardium have been studied in ArKO versus wild-type mice. Despite being completely estrogen deplete, during cardiac reperfusion after an episode of ischemia, the ArKO mice hearts exhibited greater systolic performance, less diastolic dysfunction and less LDH release, suggesting less cardiac muscle damage [Citation31]. A study of oophorectomized rats complement these findings. In this second study, oophorectomized rats with no hormone replacement, and rats treated with estrogen alone or estrogen plus testosterone, were compared with sham operated mice [Citation32]. After induction of acute myocardial ischemia, the animals were sacrificed and their hearts immediately reperfused. Whereas low-dose estradiol did not restore left ventricular end diastolic pressure, testosterone alone and testosterone with estradiol did [Citation32]. The dose of testosterone used increased the serum testosterone to 1.29 ± 0.06 ng/ml, which was slightly lower than that of the sham treated animals (1.69 ± 0.09 ng/ml) [Citation32]. Together these studies suggest testosterone may afford myocardial protection against ischemia. In addition, testosterone has been shown to favorably influence cardiac muscle action potential and calcium homeostasis [Citation33,Citation34]. Specifically, testosterone enhances cardiac myocyte contractility through regulation of calcium channels [Citation35].
Testosterone and CVD risk markers in women
Studies of the associations between testosterone and blood lipids have provided inconsistent findings. Endogenous testosterone concentrations were not independent predictors of lipid levels and made little contribution to total cholesterol or low-density lipoprotein (LDL)-cholesterol in a multinational study of postmenopausal women aged 40–70 years [Citation36]. Conversely, a German study reported a positive association between testosterone and total cholesterol, LDL-cholesterol and high-density lipoprotein (HDL)-cholesterol [Citation37]. Consistently, however, low SHBG concentrations have been independently associated with a more adverse lipid profile with higher concentrations of triglycerides and lower concentrations of HDL-cholesterol [Citation36,Citation38,Citation39]. Low SHBG, but not high serum testosterone, has been associated with greater visceral fat [Citation40]. Low SHBG is an independent marker of insulin resistance [Citation41], and has been associated with an increased risk of type 2 diabetes [Citation37,Citation42,Citation43]. Conversely, testosterone measured by LCMS was not associated with an increased risk of diabetes in a large Dutch study [Citation43].
Observational studies of testosterone and ischemic heart disease
Older observational studies of testosterone and ischemic cardiovascular events and mortality are limited by the use of immunoassays that lack precision for the measurement of testosterone in women, recruitment of convenience clinic-based samples, case–control design and long intervals between the time of blood draw and cardiovascular events [Citation44–48]. In most studies that have used immunoassays to measure testosterone concentrations, low endogenous testosterone was associated with an increased risk of ischemic cardiac events [Citation45,Citation46,Citation49], or a greater risk of ischemic stroke (greater carotid artery intima-media thickness and atherosclerosis) [Citation50–52]. In the Miami Heart Study, testosterone was not associated with any coronary artery plaque or high-risk plaque [Citation53]. Conversely, high endogenous testosterone was found to be associated with a greater risk of CVD and death in two studies [Citation44,Citation48]. In both studies, testosterone was measured many years before events were recorded. In a study of all-cause and cause-specific mortality in perimenopausal and postmenopausal women aged 45–82 years, Schederecker et al. found no significant associations between testosterone measured by LCMS and cardiovascular death or all cause-mortality [Citation54]. Meun et al. reported for a sample of women with a mean age of 70 years that having a blood testosterone concentration measured by LCMS in the highest quartile, compared with the middle two quartiles, was not associated with a greater likelihood of CVD [Citation55]. Whether a testosterone concentration in the lowest quartile was associated with CVD risk was not reported [Citation55].
The findings for testosterone, measured by LCMS, in women aged 70–94 years at recruitment were reported for the Sex Hormone and Older Women Study [Citation56]. To be eligible for this study, women were required not to have previously experienced a major adverse cardiovascular event, which included fatal coronary heart disease (excluding heart failure), non-fatal myocardial infarction and fatal or non-fatal ischemic stroke [Citation56]. Women were excluded from the analysis if they were known to be using any medication that might influence their sex hormone blood levels. Over a 4.5-year follow-up of 5535 women, 144 women experienced a major adverse cardiovascular event and there were 200 deaths [Citation56]. After adjusting for age, body mass index, smoking, alcohol consumption, diabetes, hypertension, dyslipidemia, impaired renal function and aspirin use, a blood testosterone concentration in the highest two quartiles was associated with an approximately 40% lower likelihood of a major adverse cardiovascular event, with the benefit emerging about 3 years from the baseline blood draw [Citation56]. As in the study by Schederecker et al. [Citation54], no association was seen for testosterone and all-cause mortality [Citation56]. Taken together, the overall data suggest low testosterone is associated with a greater likelihood of ischemic CVD in older women.
Testosterone and heart failure
Heart failure has become a major public health problem, as it is now the most common cause of hospitalization for people aged >65 years [Citation57]. Heart failure comprises the structural or functional impairment of ventricular filling or ejection of blood, with the former known as heart failure with preserved ejection fraction (HFpEF) and the latter as heart failure with reduced ejection fraction [Citation57]. HFpEF occurs when the left ventricle does not fully fill with blood between contractions. Hence, the fraction of blood pumped out with each contraction (ejection fraction) is preserved, but the total volume available to be pumped out is less than the body needs. HFpEF now accounts for more than 50% of heart failure cases, is three times more common in women and is increasing in incidence [Citation58,Citation59]. Factors believed to contribute to this risk disparity include sex differences in myocardial structure and metabolism, and vascular function [Citation60]. Common risk factors for HFpEF, including obesity, hypertension, diabetes mellitus, sleep disorders, chronic obstructive pulmonary disease, atrial fibrillation and age [Citation61], confer a greater risk of HFpEF in women [Citation60]. Iron deficiency, also a HFpEF risk factor, is more common in women, while pregnancy and pre-eclampsia are risk factors unique to women [Citation60]. Age-related changes in physical function and muscle loss further increase the risk of HFpEF in women, and low cardiorespiratory fitness in midlife independently predicts future heart failure, as described in the following [Citation59].
The main symptom of HFpEF is exercise intolerance, which can be quantified by the peak amount of oxygen used during intense/maximal exercise effort (VO2max) [Citation62]. Skeletal muscle mass and function are critical determinants of VO2max, such that a decline in muscle function reduces VO2max [Citation62]. An estimated 9% of women aged over 65 years have reduced muscle mass and function, which contributes to the pathogenesis and progression of HFpEF [Citation62]. Consistent with this, women with HFpEF have reduced skeletal muscle mass, with fat infiltration and impaired muscle oxidative capacity, and consequently lowered cardiorespiratory fitness (low VO2max) [Citation63]. Other symptoms of HFpEF include dyspnea and fatigue which impair quality of life [Citation57].
Recently, treatment with the SGLT2 inhibitor dapagliflozin has been shown to improve symptomatic HFpEF [Citation64]. Symptomatic HFpEF is preceded by asymptomatic pre-heart failure (stages A and B) such that intervention to prevent progression should ideally commence early in the disease. Supervised exercise training, which improves VO2max, has shown promise in preventing progression to symptomatic HFpEF [Citation65]. But such programs are expensive and not always feasible.
Compared with the immediately preceding years, HFpEF increases four-fold in women aged 55–64 years, when blood testosterone concentrations are at the adult female nadir [Citation11,Citation58]. In a large community-based study, low DHEAS was associated with a statistically significant increased likelihood of HFpEF across all ages, while a statistically significant association between low testosterone and an increased risk of HFpEF was limited to women aged younger than 65 years [Citation66]. These findings are limited by the measurement of testosterone by immunoassay [Citation66]. Potentially, testosterone therapy might improve HFpEF progression via salutatory effects on peripheral vascular resistance, myocardial function and skeletal muscle performance. This merits consideration.
Data from clinical trials
Few clinical trials have examined the effects of testosterone on CVD risk markers or cardiovascular events as primary outcomes. Most of the available data come from randomized controlled trials (RCTs) of testosterone for the treatment of hypoactive sexual desire dysfunction, or other conditions. Our systematic review and meta-analysis of published clinical trials with treatment of testosterone and comparator for a duration of at least 3 months revealed that oral testosterone therapy lowers total cholesterol, HDL-cholesterol and triglycerides, whereas non-oral testosterone therapy has no effect on lipid and lipoproteins [Citation67]. Meta-analysis showed no influence of transdermal testosterone therapy on blood pressure, glucose, insulin or C-reactive protein [Citation67]. Analysis of data from seven published RCTs of the transdermal testosterone patch [Citation68–74], and two unpublished testosterone patch RCTs, showed no increase in myocardial infarction, stroke or venous thromboembolic events with testosterone compared with placebo [Citation67]. In a 6-month pilot double-blind RCT of women, mean age 69 years, with heart failure with reduced ejection fraction, testosterone therapy improved VO2max, performance on the 6-min walk test and insulin sensitivity [Citation75]. No studies in women with HFpEF have been reported. However, we are presently recruiting women with asymptomatic HFpEF to determine the effects of testosterone versus placebo on VO2max and myocardial structure and function. In doses that approximate physiological concentrations for premenopausal women, testosterone has not been associated with serious adverse events [Citation67].
Conclusions
The world has an aging population, with an over-representation of older women. CVD is not only a cause of personal disability but incurs enormous health-care costs. Understanding the factors that keep women healthy as they age is vital, considering the average life expectancy of a girl born in 2030 is likely to be greater than 90 years [Citation76]. Sex hormones influence cardiovascular health, but compared with estrogens, testosterone has been overlooked as a hormone with favorable cardiovascular effects in women. The restoration of testosterone concentrations in older women to levels seen in younger women provokes thought as to why this occurs [Citation3,Citation11,Citation13,Citation14]. While available data from observational and mechanistic studies suggest this physiological change may afford cardiovascular protection, RCTs examining the cardiovascular effects of testosterone in women are scant. The findings from this review indicates that robust RCTs to determine the effects of testosterone in women with heart failure, both heart failure with reduced ejection fraction and HFpEF, and at increased atherogenic CVD risk are warranted.
Potential conflict of interest
S.R.D. reports honoraria from Besins Healthcare, Mayne Pharma, Health Ed, BioSyent, Lawley Pharmaceuticals and Que Oncology; has served on Advisory Boards for Mayne Pharma, Astellas Pharmaceuticals, Theramex and Gedeon Richter; and has been an institutional investigator for Que Oncology and Ovoca Bio.
Source of funding
This review did not receive any specific grant from funding agencies in the public, commercial or not-for-profit sectors. S.R.D. is an NHMRC Investigator [Grant No. 2016627].
Acknowledgements
The author declares that artificial intelligence software had no role in the authorship of this review.
References
- Stramba-Badiale M, Fox KM, Priori SG, et al. Cardiovascular diseases in women: a statement from the policy conference of the european society of cardiology. Eur Heart J. 2006;27(8):994–1005. doi: 10.1093/eurheartj/ehi819.
- Gorodeski GI. Update on cardiovascular disease in post-menopausal women. Best Pract Res Clin Obstet Gynaecol. 2002;16(3):329–355. doi: 10.1053/beog.2002.0282.
- Davis SR, Bell RJ, Robinson PJet al. Testosterone and estrone increase from the age of 70 years; findings from the sex hormones in older women study. J Clin Endocrinol Metab. 2019;104(12):6291–6300. doi: 10.1210/jc.2019-00743.
- Davis SR, Panjari M, Stanczyk FZ. DHEA replacement for postmenopausal women. J Clin Endocrinol Metab. 2011;96(6):1642–1653. doi: 10.1210/jc.2010-2888.
- Labrie F, Luu-The V, Labrie C, et al. Endocrine and intracrine sources of androgens in women: inhibition of breast cancer and other roles of androgens and their precursor dehydroepiandrosterone. Endocr Rev. 2003;24(2):152–182. doi: 10.1210/er.2001-0031.
- Dunn JF, Nisula BC, Rodbard D. Transport of steroid hormones. Binding of 21 endogenous steroids to both testosterone-binding globulin and cortico-steroid-binding globulin in human plasma. J Clin Endocrinol Metab. 1981;53(1):58–68. doi: 10.1210/jcem-53-1-58.
- Goldman AL, Bhasin S, Wu FCW, et al. A reappraisal of testosterone’s binding in circulation: physiological and clinical implications. Endocr Rev. 2017;38(4):302–324. doi: 10.1210/er.2017-00025.
- Handelsman DJ. Free testosterone: pumping up the tires or ending the free ride? Endocr Rev. 2017;38(4):297–301. doi: 10.1210/er.2017-00171.
- Davis SR, Baber R, Panay N, et al. Global consensus position statement on the use of testosterone therapy for women. Climacteric. 2019;22(5):429–434. doi: 10.1080/13697137.2019.1637079.
- Skiba MA, Bell RJ, Islam RM, et al. Androgens during the reproductive years, what’s normal for women? J Clin Endocrinol Metab. 2019;104(11):5382–5392. doi: 10.1210/jc.2019-01357.
- Davison SL, Bell R, Donath S, et al. Androgen levels in adult females: changes with age, menopause, and oophorectomy. J Clin Endocrinol Metab. 2005;90(7):3847–3853. doi: 10.1210/jc.2005-0212.
- Burger HG, Dudley EC, Robertson DM, et al. Hormonal changes in the menopause transition. Recent Prog Horm Res. 2002;57(1):257–275. doi: 10.1210/rp.57.1.257.
- Cappola AR, Ratcliffe SJ, Bhasin S, et al. Determinants of serum total and free testosterone levels in women over the age of 65 years. J Clin Endocrinol Metab. 2007;92(2):509–516. doi: 10.1210/jc.2006-1399.
- Aribas E, Roeters van Lennep JE, De Rijke YB, et al. Sex steroids and sex steroid-binding globulin levels amongst middle-aged and elderly men and women from general population. Eur J Clin Invest. 2022;52(12):e13866. doi: 10.1111/eci.13866.
- Islam RM, Bell RJ, Handelsman DJ, et al. Longitudinal changes over three years in sex steroid hormone levels in women aged 70 years and over. Clin Endocrinol. 2021;94(3):443–448. doi: 10.1111/cen.14401.
- Biegelsen E, Loscalzo J. Endothelial function and atherosclerosis. Coron Artery Dis. 1999;10(4):241–256.
- Meczekalski B, Katulski K, Czyzyk A, et al. Functional hypothalamic amenorrhea and its influence on women’s health. J Endocrinol Invest. 2014;37(11):1049–1056. doi: 10.1007/s40618-014-0169-3.
- Taddei S, Virdis A, Ghiadoni L, et al. Menopause is associated with endothelial dysfunction in women. Hypertension. 1996;28(4):576–582. doi: 10.1161/01.hyp.28.4.576.
- Chambliss KL, Shaul PW. Estrogen modulation of endothelial nitric oxide synthase. Endocr Rev. 2002;23(5):665–686. doi: 10.1210/er.2001-0045.
- Leiberman EH, Gerhard M, Uehata A, et al. Estrogen improves endothelium-dependent, flow-mediated vasodilation in postmenopausal women. Ann Intern Med. 1994;121:936–941.
- Vitale C, Mercuro G, Cerquetani E, et al. Time since menopause influences the acute and chronic effect of estrogens on endothelial function. Arterioscler Thromb Vasc Biol. 2008;28(2):348–352. doi: 10.1161/ATVBAHA.107.158634.
- Montalcini T, Gorgone G, Gazzaruso C, et al. Endogenous testosterone and endothelial function in postmenopausal women. Coron Artery Dis. 2007;18(1):9–13. doi: 10.1097/01.mca.0000236290.79306.d1.
- Rech CM, Clapauch R, de Souza M, et al. Low testosterone levels are associated with endothelial dysfunction in oophorectomized early postmenopausal women. Eur J Endocrinol. 2016;174(3):297–306. doi: 10.1530/EJE-15-0878.
- Worboys S, Kotsopoulos D, Teede H, et al. Parental testosterone improves endothelium-dependent and independent vasodilation in postmenopausal women already receiving estrogen. J Clin Endocrinol Metab. 2001;86(1):158–161. doi: 10.1210/jcem.86.1.7103.
- Campelo AE, Cutini PH, Massheimer VL. Cellular actions of testosterone in vascular cells: mechanism independent of aromatization to estradiol. Steroids. 2012;77(11):1033–1040. doi: 10.1016/j.steroids.2012.05.008.
- Navarro-Dorado J, Orensanz LM, Recio P, et al. Mechanisms involved in testosterone-induced vasodilatation in pig prostatic small arteries. Life Sci. 2008;83(15–16):569–573. doi: 10.1016/j.lfs.2008.08.009.
- Wynne FL, Khalil RA. Testosterone and coronary vascular tone: implications in coronary artery disease. J Endocrinol Invest. 2003;26(2):181–186. doi: 10.1007/BF03345150.
- Davison S, Thipphawong J, Blanchard J, et al. Pharmacokinetics and acute safety of inhaled testosterone in postmenopausal women. J Clin Pharmacol. 2005;45(2):177–184. doi: 10.1177/0091270004269840.
- Grohe C, Kahlert S, Lobbert K, et al. Expression of oestrogen receptor alpha and beta in rat heart: role of local oestrogen synthesis. J Endocrinol. 1998;156(2):R1–R7. doi: 10.1677/joe.0.156r001.
- van Weerden WM, Bierings HG, van Steenbrugge GJ, et al. Adrenal glands of mouse and rat do not synthesize androgens. Life Sci. 1992;50(12):857–861. doi: 10.1016/0024-3205(92)90204-3.
- Bell JR, Mellor KM, Wollermann AC, et al. Aromatase deficiency confers paradoxical postischemic cardioprotection. Endocrinology. 2011;152(12):4937–4947. doi: 10.1210/en.2011-1212.
- Fu L, Liu Y, Wang J, et al. Cardioprotection by low-dose of estrogen and testosterone at the physiological ratio on ovariectomized rats during ischemia/reperfusion injury. J Cardiovasc Pharmacol. 2017;70(2):87–93. doi: 10.1097/FJC.0000000000000497.
- Bidoggia H, Maciel JP, Capalozza N, et al. Sex differences on the electrocardiographic pattern of cardiac repolarization: possible role of testosterone. Am Heart J. 2000;140(4):678–683. doi: 10.1067/mhj.2000.109918.
- Dunlay SM, Roger VL. Gender differences in the pathophysiology, clinical presentation, and outcomes of ischemic heart failure. Curr Heart Fail Rep. 2012;9(4):267–276. doi: 10.1007/s11897-012-0107-7.
- Curl CL, Delbridge LMD, Canny BJ, et al. Testosterone modulates cardiomyocyte Ca(2+) handling and contractile function. Physiol Res. 2009;58(2):293–297. doi: 10.33549/physiolres.931460.
- Worsley R, Robinson PJ, Bell RJ, et al. Endogenous estrogen and androgen levels are not independent predictors of lipid levels in postmenopausal women. Menopause. 2013;20(6):640–645. doi: 10.1097/GME.0b013e318279bd4a.
- Kische H, Gross S, Wallaschofski H, et al. Clinical correlates of sex hormones in women: the study of health in pomerania. Metabolism. 2016;65(9):1286–1296. doi: 10.1016/j.metabol.2016.05.011.
- Mudali S, Dobs AS, Ding J, et al. Endogenous postmenopausal hormones and serum lipids: the atherosclerosis risk in communities study. J Clin Endocrinol Metab. 2005;90(2):1202–1209. doi: 10.1210/jc.2004-0744.
- Bell RJ, Davison SL, Papalia MA, et al. Endogenous androgen levels and cardiovascular risk profile in women across the adult life span. Menopause. 2007;14(4):630–638. doi: 10.1097/GME.0b013e31802b6cb1.
- Janssen I, Powell LH, Kazlauskaite R, et al. Testosterone and visceral fat in midlife women: the Study of Women’s Health across the Nation (SWAN) fat patterning study. Obesity. 2010;18(3):604–610. doi: 10.1038/oby.2009.251.
- Davis SR, Robinson PJ, Moufarege A, et al. The contribution of SHBG to the variation in HOMA-IR is not dependent on endogenous oestrogen or androgen levels in postmenopausal women. Clin Endocrinol. 2012;77(4):541–547. doi: 10.1111/j.1365-2265.2011.04301.x.
- Ding EL, Song Y, Manson JE, et al. Sex hormone-binding globulin and risk of type 2 diabetes in women and men. N Engl J Med. 2009;361(12):1152–1163. doi: 10.1056/NEJMoa0804381.
- Muka T, Nano J, Jaspers L, et al. Associations of steroid sex hormones and sex hormone-binding globulin with the risk of type 2 diabetes in women: a population-based cohort study and meta-analysis. Diabetes. 2017;66(3):577–586. doi: 10.2337/db16-0473.
- Benn M, Voss SS, Holmegard HN, et al. Extreme concentrations of endogenous sex hormones, ischemic heart disease, and death in women. Arterioscler Thromb Vasc Biol. 2015;35(2):471–477. doi: 10.1161/ATVBAHA.114.304821.
- Laughlin GA, Goodell V, Barrett-Connor E. Extremes of endogenous testosterone are associated with increased risk of incident coronary events in older women. J Clin Endocrinol Metab. 2010;95(2):740–747. doi: 10.1210/jc.2009-1693.
- Sievers C, Klotsche J, Pieper L, et al. Low testosterone levels predict all-cause mortality and cardiovascular events in women: a prospective cohort study in German primary care patients. Eur J Endocrinol. 2010;163(4):699–708. doi: 10.1530/EJE-10-0307.
- Schaffrath G, Kische H, Gross S, et al. Association of sex hormones with incident 10-year cardiovascular disease and mortality in women. Maturitas. 2015;82(4):424–430. doi: 10.1016/j.maturitas.2015.08.009.
- Zhao D, Guallar E, Ouyang P, et al. Endogenous sex hormones and incident cardiovascular disease in post-menopausal women. J Am Coll Cardiol. 2018;71(22):2555–2566. doi: 10.1016/j.jacc.2018.01.083.
- Naessen T, Sjogren U, Bergquist J, et al. Endogenous steroids measured by high-specificity liquid chromatography-tandem mass spectrometry and prevalent cardiovascular disease in 70-year-old men and women. J Clin Endocrinol Metab. 2010;95(4):1889–1897. doi: 10.1210/jc.2009-1722.
- Debing E, Peeters E, Duquet W, et al. Endogenous sex hormone levels in postmenopausal women undergoing carotid artery endarterectomy. Eur J Endocrinol. 2007;156(6):687–693. doi: 10.1530/EJE-06-0702.
- Bernini GP, Sgro’ M, Moretti A, et al. Endogenous androgens and carotid intimal-medial thickness in women. J Clin Endocrinol Metab. 1999;84(6):2008–2012. doi: 10.1210/jcem.84.6.5824.
- Golden SH, Maguire A, Ding J, et al. Endogenous postmenopausal hormones and carotid atherosclerosis: a case-control study of the atherosclerosis risk in communities cohort. Am J Epidemiol. 2002;155(5):437–445. doi: 10.1093/aje/155.5.437.
- Kwapong YA, Sharma G, Valero-Elizondo J, et al. The association of sex-specific hormones with coronary artery plaque characteristics from Miami Heart (MiHeart) study. Am J Prev Cardiol. 2023;14:100479. doi: 10.1016/j.ajpc.2023.100479.
- Schederecker F, Cecil A, Prehn C, et al. Sex hormone-binding globulin, androgens and mortality: the KORA-F4 cohort study. Endocr Connect. 2020;9(4):326–336. doi: 10.1530/EC-20-0080.
- Meun C, Franco OH, Dhana K, et al. High androgens in postmenopausal women and the risk for atherosclerosis and cardiovascular disease: the Rotterdam study. J Clin Endocrinol Metab. 2018;103(4):1622–1630. doi: 10.1210/jc.2017-02421.
- Islam RM, Bell RJ, Handelsman DJ, et al. Associations between blood sex steroid concentrations and risk of major adverse cardiovascular events in healthy older women in Australia: a prospective cohort substudy of the ASPREE trial. Lancet Healthy Longev. 2022;3(2):e109–e18. doi: 10.1016/S2666-7568(22)00001-0.
- Ziaeian B, Fonarow GC. Epidemiology and aetiology of heart failure. Nat Rev Cardiol. 2016;13(6):368–378. doi: 10.1038/nrcardio.2016.25.
- Chan YK, Tuttle C, Ball J, et al. Current and projected burden of heart failure in the Australian adult population: a substantive but still ill-defined major health issue. BMC Health Serv Res. 2016;16(1):501. doi: 10.1186/s12913-016-1748-0.
- Pandey A, Cornwell WK, 3rd, Willis B, et al. Body mass index and cardiorespiratory fitness in mid-life and risk of heart failure hospitalization in older age: findings from the cooper center longitudinal study. JACC Heart Fail. 2017;5(5):367–374. doi: 10.1016/j.jchf.2016.12.021.
- Beale AL, Meyer P, Marwick TH, et al. Sex differences in cardiovascular pathophysiology: Why women are overrepresented in heart failure with preserved ejection fraction. Circulation. 2018;138(2):198–205. doi: 10.1161/CIRCULATIONAHA.118.034271.
- Pieske B, Tschope C, de Boer RA, et al. How to diagnose heart failure with preserved ejection fraction: the HFA-PEFF diagnostic algorithm: a consensus recommendation from the Heart Failure Association (HFA) of the European Society of Cardiology (ESC). Eur Heart J. 2019;40(40):3297–3317. doi: 10.1093/eurheartj/ehz641.
- Haykowsky MJ, Tomczak CR, Scott JM, et al. Determinants of exercise intolerance in patients with heart failure and reduced or preserved ejection fraction. J Appl Physiol. 2015;119(6):739–744. doi: 10.1152/japplphysiol.00049.2015.
- Shah SJ, Kitzman DW, Borlaug BA, et al. Phenotype-specific treatment of heart failure with preserved ejection fraction: a Multiorgan roadmap. Circulation. 2016;134(1):73–90. doi: 10.1161/CIRCULATIONAHA.116.021884.
- Nassif ME, Windsor SL, Borlaug BA, et al. The SGLT2 inhibitor dapagliflozin in heart failure with preserved ejection fraction: a multicenter randomized trial. Nat Med. 2021;27(11):1954–1960. doi: 10.1038/s41591-021-01536-x.
- Howden EJ, Sarma S, Lawley JS, et al. Reversing the cardiac effects of sedentary aging in middle age – a randomized controlled trial: implications for heart failure prevention. Circulation. 2018;137(15):1549–1560. doi: 10.1161/CIRCULATIONAHA.117.030617.
- Zhao D, Guallar E, Ballantyne CM, et al. Sex hormones and incident heart failure in men and postmenopausal women: the atherosclerosis risk in communities study. J Clin Endocrinol Metab. 2020;105(10):e3798–e3807.
- Islam RM, Bell RJ, Green S, et al. Safety and efficacy of testosterone for women: a systematic review and meta-analysis of randomised controlled trial data. Lancet Diabetes Endocrinol. 2019;7(10):754–766. doi: 10.1016/S2213-8587(19)30189-5.
- Braunstein GD, Sundwall DA, Katz M, et al. Safety and efficacy of a testosterone patch for the treatment of hypoactive sexual desire disorder in surgically menopausal women: a randomized, placebo-controlled trial. Arch Intern Med. 2005;165(14):1582–1589. doi: 10.1001/archinte.165.14.1582.
- Buster JE, Kingsberg SA, Aguirre O, et al. Testosterone patch for low sexual desire in surgically menopausal women: a randomized trial. Obstet Gynecol. 2005;105(5 Pt 1):944–952. doi: 10.1097/01.AOG.0000158103.27672.0d.
- Simon J, Braunstein G, Nachtigall L, et al. Testosterone patch increases sexual activity and desire in surgically menopausal women with hypoactive sexual desire disorder. J Clin Endocrinol Metab. 2005;90(9):5226–5233. doi: 10.1210/jc.2004-1747.
- Davis SR, van der Mooren MJ, van Lunsen RHW, et al. The efficacy and safety of a testosterone patch for the treatment of hypoactive sexual desire disorder in surgically menopausal women: a randomized, placebo-controlled trial. Menopause. 2006;13(3):387–396. doi: 10.1097/01.gme.0000179049.08371.c7.
- Davis SR, Moreau M, Kroll R, et al. Testosterone for low libido in menopausal women not taking estrogen therapy. N Engl J Med. 2008;359(19):2005–2017. doi: 10.1056/NEJMoa0707302.
- Panay N, Al-Azzawi F, Bouchard C, et al. Testosterone treatment of HSDD in naturally menopausal women: the ADORE study. Climacteric. 2010;13(2):121–131. doi: 10.3109/13697131003675922.
- Shifren J, Davis SR, Moreau M, et al. Testosterone patch for the treatment of hypoactive sexual desire disorder in naturally menopausal women: results from the INTIMATE NM1 study. Menopause. 2006;13(5):770–779. doi: 10.1097/01.gme.0000243567.32828.99.
- Iellamo F, Volterrani M, Caminiti G, et al. Testosterone therapy in women with chronic heart failure: a pilot double-blind, randomized, placebo-controlled study. J Am Coll Cardiol. 2010;56(16):1310–1316. doi: 10.1016/j.jacc.2010.03.090.
- Kontis V, Bennett JE, Mathers CD, et al. Future life expectancy in 35 industrialised countries: projections with a Bayesian model ensemble. Lancet. 2017;389(10076):1323–1335. doi: 10.1016/S0140-6736(16)32381-9.