Motor unit double discharges, or doublet firings, have been described as two consecutive motor unit discharges that occur with short interspike intervals. By the use of electrical stimulation protocols, short interspike intervals inserted at the beginning of a stimulation train have been shown to increase both the peak force and the rate of rise of force production [S.J. Garland and L. Griffin, Motor unit double discharges: statistical anomaly or functional entity? Can. J. Appl. Physiol. 24 (1999), pp. 113–130]. The aim of this article is to estimate possible mechanical effects of simulated motor unit doublets in selected motor unit pools (MUPs) during the initial isometric contractions. Five different MUPs with varying ranges were simulated considering important nonlinearities in the force response to pairs of stimuli according to Thomas et al. [C.K. Thomas, R.S. Johansson, and B. Bigland-Ritchie, Pattern of pulses that maximize force output from single human thenar motor units. J. Neurophysiol. 82 (1999), pp. 3188–3195]. The results support the hypothesis that double discharges represent a functional entity: expected increases depend on the properties of the MUP as a whole. Relative timing of the doublet discharges occurs, but has only little effect.
1. Introduction
When human motor units are recruited in a ballistic voluntary contraction they often start to fire with two closely spaced impulses, termed ‘doublets’, followed by longer impulse intervals that maintain the contraction [Citation1–3, cf. 4]. Impulse patterns of one or two short interpulse intervals followed by longer intervals maximize the force–time integral from triceps surae motor units of the cat [Citation5–8, cf. 4]. Likewise, single human thenar motor units show twitch force summation up to half of the maximum tetanic force when stimulated intraneurally by a short interpulse interval of 5–15 ms [Citation4]. Interestingly, both the peak and force–time integral elicited by a ‘doublet’ seem to be depending on several properties of the motor units: in relation to initial twitch values, twitch/tetanic force ratios and contraction time (CT) correlations show slope coefficients different from zero (see [Citation4]).
Also, there is evidence in the literature that extra doublets (not only at the onset of the series of spikes) contribute to an increase in the speed of voluntary contraction after dynamic training in human ankle dorsi flexor muscles [Citation9]. Thus the pattern by which motoneurons fire when initially recruited – and during brief contractions there are only a few spikes observable – strongly influences the force motor units subsequently generated [Citation4].
Therefore, the aim of this article was to investigate possible effects of simulated motor unit doublets in selected motor unit pools (MUPs) during isometric contractions to gain a deeper understanding of within-muscle activation patterns during movement initiations.
2. Methods
This study involved computer simulations that were based on parts of models previously described in detail by Fuglevand et al. [Citation10–12], extended by the results experimentally obtained by Thomas et al. [Citation4] and the observations made by Van Cutsem et al. [Citation9].
In general, the model represents the isometric force production for motor units with known physiological properties of a human hand muscle, modified by coefficients involving nonlinearities because of activation history (short-duration interspike intervals) depending on their mechanical properties [peak twitch force, contraction time (CT)]. The simulations involve the prediction of the isometric muscle force responses for different MUP populations to a pair of stimuli 5 ms apart from each other (‘doublet firing’). Such doublets have been seen, for example, by Garland et al. [Citation13] who found motor units that discharge with an initial doublet at the onset of movement but did not discharge again during the movement commonly occurred in elbow extension movements.
2.1. Motor unit force model
The theoretical background of the isometric force model for motor units used in this study was previously described [Citation11,Citation14,Citation15]. Briefly, the time course of the single twitch response of a motor unit was regarded as the impulse response of a critically damped second-order system. The distribution of peak twitch forces across the MUP was assumed as an increasing exponential function with the lowest threshold motor unit assigned to have the smallest force and the highest threshold unit to have the greatest force. Fuglevand et al. [Citation13] assigned a variation of twitch forces over a 100-fold range; this study involves the addition of variations in the range of twitch forces at 10, 20, 40, 60, 80, 120 and 140 to estimate the influence of the range of peak twitch forces. The relationship between twitch force and CT was approximated as an inverse power function with the CT for the weakest motor unit being 90 ms, and for the strongest unit 30 ms. The impulse response of a motor unit, fi (t), can be expressed as
To estimate the influence of the distribution of the single motor unit contractile properties, five MUPs were generated: MUP1 with the number of Fuglevand's model (120 motor units); MUP2 with a number of 60 motor units by first-half of the population (i.e. weakest MU1 to MU60); MUP3 with a number of 60 motor units by second-half of the population (i.e. MU61 to strongest MU120); MUP4 with a number of 180 motor units by duplicating first-half of the population (i.e. weakest MU1 to MU60); and MUP5 with a number of 180 motor units by duplicating second-half of the population (i.e. MU61 to strongest MU120).
2.2. Nonlinear properties to a pair of stimuli
The most novel part of this article is the consideration of the nonlinearities in the force response to a pair of stimuli (‘doublet’) that were based on the experimental findings described by Thomas et al. [Citation4]. The authors found that for all 21 units, the ‘doublet’ forces averaged 3.5 ± 1.6 times the initial twitch force and were 48 ± 13% of the maximum tetanic force: the force–time integrals of the ‘doublets’ were 5.6 ± 3.6 times those of the initial twitches. The greatest summation both in peak force (‘doublet’ force/twitch force) and force–time integral occurred in units with weak twitch forces and small force–time integrals. This nonlinear behaviour was incorporated into the model by allowing the enhancement of ‘doublet’ force response to vary as a function of twitch force. On the basis of the data of Thomas et al. [Citation4] and in an analogous manner as described in Fuglevand et al. [Citation11] for CT, the relationship between twitch force of the ith motor unit Pi and ‘doublet’ force/twitch force ratio d was approximated as an inverse power function in the form
The parameter D
max represented the largest ‘doublet’ force/twitch force value, the coefficient a was determined from the expression a = logRTRP, where RP was the range of motor unit peak twitch forces and RT was the range of CTs desired. For example, if a threefold range in CT was desired for a pool of units that had a 100-fold range in twitch forces, then a = log3100 = 4.2. If the largest ‘doublet’ force/twitch force value desired for the pool was 6.6, then EquationEquation (2)(2) became
Another observation of Thomas et al. [Citation4] was that the ratio of ‘doublet’ CT to initial twitch CT was greatest for fast units (about twofold) and almost the same for slow units. This behaviour was incorporated into the model by allowing the increase of ‘doublet’ CT to vary as a function of CT and twitch force. On the basis of the data of Thomas et al. [Citation4], the relationship between CT and ‘doublet’ CT/twitch CT ratio DCT was approximated as an exponential function in the form
In summary, the force–time behaviour after the second stimulus in unit i, f
id, depended on the CT and the peak twitch force. Both factors, the ‘doublet’ force/twitch force ratio di
and the ‘doublet’ CT/twitch CT ratio DCT
i
were used to adapt the motor unit impulse response (
EquationEquation 1(1)) after the second stimulus as
The mechanical coupling that may occur between active motor units and that may lead to nonlinear force summation [Citation16,Citation17] was not considered in the model. The mechanical actions of motor units were assumed to be independent of one another; thus the total force in the muscle without a second stimulus (‘doublet’), F M(t), was determined as the sum of the individual motor unit forces:
The total force in the muscle with a second stimulus, F Md(t), was equivalent to the sum of the individual motor unit forces and the sum of the adapted motor unit force response after the second stimulus:
The value of f id(t–t d) represents the force response to discharge after the interstimulus time interval t d of the unit. In these simulations, the ‘doublet’ time interval t d was set to 5 ms, because of the following reasons: (1) during voluntary contractions, motor unit interspike intervals of 2–10 ms are observable in vivo [Citation9] and (2) most of the units stimulated by Thomas et al. [Citation4] generated maximal force when the two pulses were delivered 5 ms apart and their main findings about the ‘doublet’ values used in this study are based on that and (3) at interstimulus intervals <5 ms, the force seems to be depressed relative to that evoked by a 5 or 10 ms interstimulus interval [see Citation4,Citation5].
2.3. Analysis
The outcome variables of the simulation are the single twitch force and the ‘doublet’ force as function of time for each unit. From these, the following parameters were computed for each of the 5 MUP × 8 ranges = 40 simulations:
-
the peak/force–time integral enhancement from doublets as multiples of the single twitch force,
-
the rate of rise in force enhancement from doublets as multiples of the single twitch force rate,
-
the percentage enhancement of the sum of all peaks of the single twitch forces (indicating consecutive discharges of the MU in a way that all units reach peak force values at the same time = maximum force capacity of the simulated MUP) relative to the peak of summated single twitch forces of all MU (indicating simultaneous discharges). This is called time index TI [ EquationEquation (8)
(8)]:
(8)
(d) The same procedure as in (b) was done for ‘doublet’ forces.
All simulations were supposed to be in the plateau region of the force–length model, that is, over which the force is maximal.
3. Results
The force responses of representative motor units to pairs of stimuli (‘doublets’) in relation to the initial twitch force for i = 1, 40, 80 and 120 (RP = 100) are shown in .
Figure 1. Simulated isometric force–time curves and ‘doublet’ force summation of four representative motor units (i = 1, 40, 80 and 120). Notice the different scales on the force axes. (a) Smaller units (i = 1, 40) have greater ‘doublet’ force summation and smaller increases in time to ‘doublet’ force in relation to twitch contraction time, (b) faster units (i = 80, 120) the opposite way around.
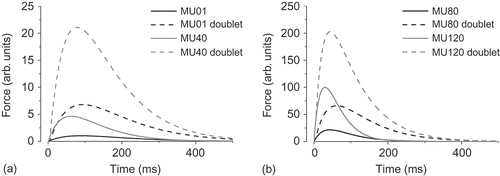
For all 120 units, the peak ‘doublet’ forces averaged 3.9 ± 1.3 times the initial peak twitch forces, the force–time integrals of the ‘doublets’ were 5.0 ± 0.9 times those of the initial twitches independent of the range of twitch forces (RP). shows the peak/force–time integral enhancement from doublets as multiples of the single twitch force for all 40 simulations according to (a).
Table 1. Peak and force–time integral enhancement values from doublets as multiples of the single twitch force from (a)
shows the rate of rise in force enhancement from doublets according to (b). As expected, largest enhancement occurred in MUP4, the pool with mostly weak motor units and therefore largest peak force enhancements.
shows the index TI for single twitch/doublet forces according to (b) and (c). The percentage increase of the sum of all peaks of the single twitch forces relative to the peak of summated single twitch forces ranges from 0.91% to 4.55% with the highest values for the smallest range (10). The percentage increase of the sum of all peaks of the doublet forces relative to the peak of summated doublet forces ranges from 0.34% to 2.51% with the highest values not for the smallest range but for the range of 20.
Table 2. Time index TI from (c) and (d) as percentage enhancements of single twitch and doublet forces
4. Discussion
The simulation procedures presented in this article supports the hypothesis that double discharges represent a functional entity because of the mechanical advantages during the types of contractions with changing amplitudes and rates of force production.
There is evidence that
-
MUPs with small ranges of twitch forces have greater benefit from doublets as MUPs with higher ranges of twitch forces;
-
MUPs with small slow-twitch motor units have greater benefit from doublets as MUPs with large fast twitch motor units;
-
The effect of relative ‘timing’, that is, consecutive discharges with highest peak force versus simultaneous discharges, seems to be smaller (+0.34% to +2.51%) versus timing in single twitch force (+0.91% to +4.55%) but exists.
In this study, two observations showed unexpected results:
-
For MUP4, the percentage enhancement of doublet forces (see ) shows a maximal value at range 20 contrary to the appending single twitch forces. In all other simulations the smallest range shows the highest values.
-
With increasing range, the benefit from timing in single twitch forces versus doublet forces decreases. The decline itself depends on the distribution of the single motor unit contractile properties (see ).
Figure 3. The benefit from timing in single twitch forces versus doublet forces decreases with higher range of twitch forces in MUP. The decline itself depends on the distribution of the single motor unit contractile properties.
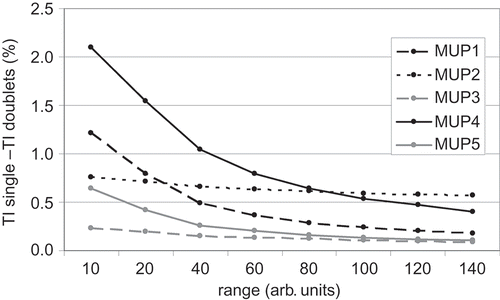
Both observations suggest that besides the paradigms described above, from a motor control strategy point of view, the occurrence of doublets could be associated with the mechanical properties of the whole MUP itself. The question is possibly not whether small or large motor units are more likely to demonstrate double discharges than others. Rather the properties of the MUP as a whole, plus the task to produce force levels in short times, seem to be accountable for the occurrence of doublets. From a mechanical point of view, there is evidence that optimal distributions of motor unit types exist with individual properties for the best benefit of doublet firings. This could help to explain some discrepancy in the literature, particularly in nonhuman experiments, as to whether double discharges occur more often in high- or low-threshold motor units [Citation18].
The incidence of double discharges has been discussed controversially. In their review, Garland and Griffin [Citation18] list a lot of different findings within a range of muscles, types of contraction, motor unit types and between subjects. At least one similarity seems to be the existence of paradigms that incorporate changing amplitudes and rates of force production. They are associated with the occurrence of double discharges. Griffin et al. [Citation19] report that 33 of 34 motor units discharged with double discharges during elbow extension movements, not always at the onset but around peak velocity and movement end. Because of that no subdivision of entire MUPs were made but all simulations included complete MUPs.
The reasons for the increases in peak force and force–time integral observed as a result of a double discharge may vary. On the one hand it is well accepted that ‘… myofilaments are activated by calcium release from sarcoplasmatic reticulum (SR) during depolarisation of the muscle membrane, … and with double discharges the second stimulus triggers the intracellular release of an additional amount of calcium at a time when the SR sink has not yet removed all the free calcium liberated by the preceding spike’ [Citation20]. On the other hand, doublets occurring at the very start of an impulse train are the most common [Citation21]. At the onset of the movement the series elastic and parallel elastic components of the whole muscle–tendon complex at both macroscopic and microscopic levels are in the toe region, in which the tissue elongates with a small increase in load as the wavy collagen fibres straightened out [see Citation22]. It has been shown for several tissues that the unloaded collagen fibres have a wavy configuration whereas the collagen fibres have straightened out under load [Citation23]. As a result of collagen fibril alignment occurring after first discharge, the elastic components of the motor unit are now in the ‘linear’ region of the load–elongation curve and the second discharge can upload the energy storage of the unit with best benefit.
Concerning maximal and/or ballistic voluntary contractions during brief movements, the importance of a quick increase in force is evident. Movement times in striking and throwing skills, for example, vary between 0.1 and 0.4 seconds. Hence, a slow-twitch motor unit with a small twitch/tetanic ratio would never converge at the tetanic region without doublet firings. It may be assumed that even mammalians with predominantly slow-twitch motor units in upper and lower extremities are able to perform those types of contractions.
Fuglevand et al. [Citation11] itemized some important nonlinearities in the force response of motor units due to activation history, for example, posttetanic potentiation [Citation24] or fatigue [Citation22]. These findings, which could influence the mechanics of doublet firings, were not included in the model.
In summary, by simulating motor unit doublet firings, a substantial increase of isometric muscle force in MUPs mainly containing small, slow-twitch motor units can be shown. There is an effect of relative timing among the motor units, which seems, however, to be quite small. Furthermore, the results suggest that the maximum increase of doublet force output as a consequence of relative timing among the units depends on an optimal range of peak twitch forces across the MUPs with fixed motor unit distribution. The results support the view that initial doublets represent a functional entity because of the dimensions of the mechanical benefits, even though the underlying mechanisms are not fully understood yet.
References
- Bawa , P. and Calancie , B. 1983 . Repetitive doublets in human flexor carpi radialis muscle . J. Physiol. , 339 : 123 – 132 .
- Desmedt , J.E. and Godaux , E. 1977 . Ballistic contractions in man: Characteristic recruitment pattern of single motor units of the tibialis anterior muscle . J. Physiol. , 264 : 673 – 693 .
- Kudina , L.P. and Alexeeva , N.L. 1992 . Repetitive doublets of human motoneurones: Analysis of interspike intervals and recruitment pattern . Electroencephalogr. Clin. Neurophysiol. , 85 : 243 – 247 .
- Thomas , C.K. , Johansson , R.S. and Bigland-Ritchie , B. 1999 . Pattern of pulses that maximize force output from single human thenar motor units . J. Neurophysiol. , 82 : 3188 – 3195 .
- Burke , R.E. , Rudomin , P. and Zajac , F.E. 3rd . 1976 . The effect of activation history on tension production by individual muscle units . Brain Res. , 109 : 515 – 529 .
- Parmigiani , F. and Stein , R.B. 1981 . Nonlinear summation of contractions in cat muscles. II. Later facilitation and stiffness changes . J. Gen. Physiol. , 78 : 295 – 311 .
- Zajac , F.E and Young , J.L. 1980 . Properties of stimulus trains producing maximum tension-time area per pulse from single motor units in medial gastrocnemius muscle of the cat . J. Neurophysiol. , 43 : 1206 – 1220 .
- Zajac , F.E. and Young , J.L. 1980 . Discharge properties of hindlimb motoneurons in decerebrate cats during locomotion induced by mesencephalic stimulation . J. Neurophysiol. , 43 : 1221 – 1235 .
- Van Cutsem , M. , Duchateau , J. and Hainaut , K. 1998 . Changes in single motor unit behaviour contribute to the increase in contraction speed after dynamic training in humans . J. Physiol. , 513 : 295 – 305 .
- Fuglevand , A.J. , Winter , D.A. , Patla , A.E. and Stashuk , D. 1992 . Detection of motor unit action potentials with surface electrodes: influence of electrode size and spacing . Biol. Cybern. , 67 : 143 – 153 .
- Fuglevand , A.J. , Winter , D.A. and Patla , A.E. 1993 . Models of recruitment and rate coding organization in motor-unit pools . J. Neurophysiol. , 70 : 2470 – 2488 .
- Yao , W. , Fuglevand , A.J. and Enoka , R.M. 2000 . Motor unit synchronization increases EMG amplitude and decreases force steadiness in simulated contractions . J. Neurophysiol. , 83 : 441 – 452 .
- Garland , S.J. , Cooke , J.D. , Miller , K.J. , Ohtsuki , T. and Ivanova , T. 1996 . Motor unit activity during human single joint movements . J. Neurophysiol. , 76 : 1982 – 1990 .
- Milner-Brown , H.S. , Stein , R.B. and Yemm , R. 1973 . The contractile properties of human motor units during voluntary isometric contractions . J. Physiol. , 228 : 285 – 306 .
- Heller , M. and Witte , K. 2006 . A dynamic approach for modelling and simulation of motor unit discharge behaviour using recurrent fuzzy-techniques . Int. J. Comp. Sci. Sport , 6 ( Edition 1 ) : 30 – 40 .
- Clamann , H.P. and Schelhorn , T.B. 1988 . Nonlinear force addition of newly recruited motor units in the cat hindlimb . Muscle Nerve , 11 : 1079 – 1089 .
- Perreault , E.J. , Day , S.J. , Hulliger , M. , Heckman , C.J. and Sandercock , T.G. 2003 . Summation of forces from multiple motor units in the cat soleus muscle . J. Neurophysiol. , 89 : 738 – 744 .
- Garland , S.J. and Griffin , L. 1999 . Motor unit double discharges: statistical anomaly or functional entity? . Can. J. Appl. Physiol. , 24 : 113 – 130 .
- Griffin , L. , Garland , S.J. and Ivanova , T. 1998 . Discharge patterns in human motor units during fatiguing arm movements . J. Appl. Physiol. , 85 : 1684 – 1692 .
- Desmedt , J.E. and Hainaut , K. 1968 . Kinetics of myofilament activation in potentiated contraction: staircase phenomenon in human skeletal muscle . Nature , 217 : 529 – 532 .
- Kirkwood , P.A. and Munson , J.B. 1996 . The incidence of initial doublets in the discharges of motoneurones of two different inspiratory muscles in the cat . J. Physiol. , 493 : 577 – 587 .
- Bigland-Ritchie , B. and Woods , J.J. 1984 . Changes in muscle contractile properties and neural control during human muscular fatigue . Muscle Nerve , 7 : 691 – 699 .
- Nordin , M. , Lorenz , T. and Campello , M. 2001 . “ Biomechanics of tendons and ligaments ” . In Basic Biomechanics of the Musculoskeletal System , Edited by: Nordin , M. and Frankel , V.H. 102 – 125 . Baltimore : Lippincott Williams & Williams . Part I, Chapt. 3
- Burke , R.E. 1981 . “ Motor units: anatomy, physiology and functional organization ” . In Handbook of Physiology. The Nervous System. Motor Control , Edited by: Brooks , V.B. 345 – 422 . Bethesda, MD : Am. Physiol. Soc . Sect. 1, Vol. II, Chapt. 10