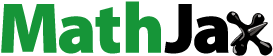
Abstract
Nowadays, the observed trend in road materials technology covers the study of environmentally-friendly modifiers. Therefore, the possibility of modifying bitumen with chitosan and its mixtures with epichlorohydrin was evaluated. The studies were carried out for four different percentages of biopolymer (1.0%, 2.5%, 4.0% and 5.5%) and two percentages (1% and 2%) of crosslinking agents and included the assessment of their influence on the basic binder properties, surface free energy parameters and chemical structure. The results indicate that the appropriately selected quantitative chitosan/epichlorohydrin ratio leads to their crosslinking inside the bitumen causing a softening effect. The study also shows the possible benefits of using biopolymer-modified binders in the production of asphalt mixtures by the enhancement of surface-free energy parameters and reduction of the dynamic viscosity. Chitosan is an environmentally-friendly biodegradable biopolymer, and its chemical crosslinking has a positive effect on the properties of modified bitumen.
1. Introduction
In recent years, there has been a significant development in material technology in the aspect of road surfaces. This is mainly due to the increase in the volume and load on the roads due to growing traffic. Currently known technologies include the use of bitumen modified with polymers and/or copolymers e.g. PE, PP, EVA, EBA, SBS, SIS or SEBS (Zhu et al., Citation2014). These modified road materials are characterised by reduced susceptibility to permanent deformation and more favourable rheological characteristics. Simultaneously, the growing ecological awareness of the builders of road surfaces and road users leads to the development of technologies for material production and road construction based on the principles of sustainable development. Therefore, in recent years there has been a growing number of technologies based on the application of materials originating from renewable sources in bitumen and asphalt mixtures as a bio-modifier (Bao et al., Citation2019), bio-filler in asphalt mixtures (Woszuk et al., Citation2020) and bio-rejuvenator (Holleran et al., Citation2021; Wróbel et al., Citation2020).
Biopolymers, also called ‘green polymers’ synthesised by microorganisms, plants, trees or other living organisms are examples of eco-friendly modifiers for bitumens (Hernández et al., Citation2014). The natural origin of biopolymers makes them environment-friendly, thanks to that they can be a full-value modifier used in road construction in line with sustainable development principles (Malinowski et al., Citation2022), offering lower energy requirements in the manufacturing process and reduced environmental impact. A common biopolymer used in road technologies is cellulose. This biomaterial often acts as a stabiliser in SMA (Stone Mastic Asphalt) mixtures, which are characterised by high bitumen and coarse aggregate content (Gallego et al., Citation2020). Cuadri et. al. carried out the work on the use of ethyl-cellulose for bitumen modification. The obtained results show that the use of this type of biopolymer caused a change in the colloidal structure of bitumen and improved rheological behaviour (Cuadri et al., Citation2020). Another biopolymer, with a wide range of application possibilities in bitumen modification, is lignin. It can be used as an ageing inhibitor, bitumen extender or even bitumen substitute (Pérez et al., Citation2019) showing a stiffening effect (Camargo et al., Citation2021). Furthermore, Wen et al. carried out a wide-temperature rheological characterisation of bitumen modified with natural rubber latex and demonstrated its effectiveness in reducing temperature sensitivity (Wen et al., Citation2017). The literature results are ambiguous as far as the research on bitumen and asphalt mixtures containing natural rubber is concerned, regarding the phase homogeneity of the binder, modification process and the production of rubberised asphalt mix (Porto et al., Citation2019). Tu et al. indicated an improvement in the storage stability of bitumen modified with welan gums (Tu et al., Citation2016). Moreover, the literature describes studies on bitumens with biopolymers based on polymerised soybean oil (Chen et al., Citation2018) Besides, there have been numerous studies on alginate-based capsules (Concha et al., Citation2022) and chitosan-based composites (Li et al., Citation2017) modified bitumen and its self-healing ability.
Chitosan is a naturally occurring polysaccharide that is a partially or fully deacylated form of chitin. As is seen in Figure , the chemical structure of chitosan consists of β-(1→4) 2-acetamido-2-deoxy-D-glucose units. Structurally, chitosan is similar to cellulose; however the primary amine group is responsible for its unique properties (Hu & Luo, Citation2021).
An important element in bitumen modification is the change of its surface properties, which have a bearing on the subsequent behaviour of the asphalt mixture under the influence of loads and changing weather conditions, especially including destructive action of water and frost. A verification method of bitumen parameters in terms of resistance to these factors is the evaluation of the surface-free energy, its components and derived parameters of cohesion, wettability and adhesion concerning the properties of aggregates (Andrzejuk et al., Citation2020; Habal & Singh, Citation2021) using the Surface-Free Energy theory (Chen et al., Citation2020).
The studies presented in this paper aim to determine the applicability of biopolymer in the form of chitosan and/or epichlorohydrin crosslinking agent for use as a novel bitumen modifier. The objective of the studies presented in this paper was to answer the following questions:
whether the biopolymer in the form of chitosan can be used as a bitumen modifier,
whether the biopolymer chemical cross-linking enhances the properties of the modified bitumen,
whether chitosan and its chemically cross-linked forms affect the bitumen surface properties and its interaction with the mineral aggregate,
whether it is suitable to use chitosan-modified bitumen for asphalt mixtures containing RAP?
In addition to the existing studies, this paper presents the possibility of biopolymer cross-linking inside bitumen. Conventional binder tests, such as penetration, softening point and dynamic viscosity as well as verification of the cohesive and adhesive properties of the bitumen modified with cross-linked chitosan, were performed. The overall scheme of the research plan is presented in Figure .
2. Materials and methods
2.1. Materials
Road bitumen 50/70 produced by Orlen Asfalt S.A. company was selected as a base material for modification in the experiments. The modification process was carried out using biopolymer in the form of chitosan with a chemical structure seen in Figure . To obtain cross-linking phenomena the extra pure grade (>99%) epichlorohydrin (1-chloro-2,3-epoxypropane), with an empirical formula of C3H5ClO, a density of 1.1812g/cm3, a melting point of 25.6°C and a boiling point of 117.9°C obtained from Fluka Analytical, was used. In FTIR analysis the pure grade (>99%) tetrahydrofurane with an empirical formula (CH2)4O was used.
2.2. Bitumen modification and sample preparation
50/70 bitumen was modified in three different variants: (I) without a cross-linking agent, (II) with a 1% addition of a cross-linking agent and (III) with a 2% addition of a cross-linking agent. The amount of crosslinking agent was calculated in relation to the weight of the modified bitumen. In these three variants, four following percentages of chitosan in relation to the weight of bitumen were applied for the preparation of modified binders: 1%, 2.5%, 4% and 5.5% w/w. The labels of obtained modified bitumen samples are summarised in Table . Directly before modification base bitumen was heated to 160°C, an appropriate amount of biopolymer and/or epichlorohydrin was added and homogenised by mechanical stirring keeping the temperature in the range of 160 ± 5°C for 60 min using an IKA T-50 ULTRA-TURRAX. To eliminate the effect of thermal history on the physical and mechanical properties of the modified asphalt binders, they were heated twice for the same period and at the same temperature Then, the samples were prepared according to the respective test standards. The samples for the contact angle test were prepared on 5 × 5 cm glass plates by spilling 5 g of hot binder on them until a flat surface was reached and then the samples were kept under cover until the test.
Table 1. Labelling of samples studied in the work.
2.3. Chemical analysis
The FTIR spectra of reference and modified bitumen were acquired using a NICOLET 380 spectrometer applying the DRIFT technique from 4000 to 600 cm−1 using 1024 scans and a resolution of 4 cm−1. Samples of bitumen were dissolved in tetrahydrofurane directly before measurement to obtain the solution with a concentration of 0.33 g/mL. Then, the 100 µL of bitumen solution was dropped on 300 mg of thoroughly dried KBr and left at 80°C for 20 min for the solvent to evaporate.
2.4. Conventional bitumen testing
The penetration of bitumen samples was determined at 25°C according to the EN 1426:2015 standard. The softening point was carried out in the ring and ball apparatus in a water bath according to the EN 1427:2015 standard. The tests of dynamic viscosity were performed in a Brookfield’s viscometer at 160°C according to the ASTM D 4402 standard. Each test was performed on three separate samples and the final result is their average value.
The penetration index (PI) was calculated on the basis of penetration and softening point test according to the formula (1).
(1)
(1) where
—softening point, (°C) and
—penetration determined in 25 °C, (0.1 mm).
2.5. SFE theory and obtained parameters
The sessile drop method was used to investigate the SFE (Surface-Free Energy) of bitumen and derived parameters related to water and frost resistance. The static contact angles were recorded using an OCA25 optical measurement system from DataPhysics Instrument with three measuring liquids: distilled water, ethylene glycol and diiodomethane (Mishra et al., Citation2020). The dosed droplet volume for each measuring liquid was about 2 µl. The properties of the measurement liquids used for the calculation of the parameters were adopted from the database of the OCA25 software. To ensure measurement accuracy, in addition to optimal liquid selection, tests were performed on three samples in three measurements.
Considering the basis of the van Oss-Chaudry-Good acid–base theory, the total surface-free energy of any material consists of the following components: Non-polar Lifshitz-van der Waals (LW), Lewis acid and Lewis base components, which can be determined using three measuring liquids. On the basis of the above-mentioned theory, the SFE of bitumen, its components and related parameters (work of cohesion (), work of adhesion under dry conditions (
) and adhesion at the bitumen-aggregate interface in the presence of water (
)) can be determined with the calculation procedure described in detail in (van Oss et al., Citation1988).
According to the applied theory, two more indicators can be determined (Bhasin et al., Citation2006; Jiménez del Barco Carrión et al., Citation2019): ER1 directly related to the water and frost resistance of the combination of a given binder–aggregate system and ER2, an index that reports the wettability effect of an aggregate with a given binder. The SFE properties for the aggregate-bitumen system were determined based on the aggregate SFE components adapted from the literature, which allowed this work to analyse the effects of biopolymer-modified bitumen in relation to different types of aggregates i.e. limestone and granite (Arabani et al., Citation2020).
3. Results and discussion
3.1. Chitosan and cross-linked chitosan effect on bitumen properties
Figure shows the effect of the addition of chitosan, epichlorohydrin and their mixtures on the penetration of the modified binders. The study shows that the modification of bitumen with chitosan leads to small changes in the penetration of the binders obtained. An increase in penetration of 4.0% was observed for 1.0% (REF_1.0) and 2.5% (REF_2.5) of the biopolymer addition. For a higher biopolymer percentage of 4.0% (REF_4.0), no change in the penetration of the bitumen after modification was observed in relation to the reference sample. While an 8.0% decrease in the penetration of the bitumen was observed when the biopolymer was used at 5.5% (REF_5.5). The obtained results show that the highest increase in penetration is observed for small biopolymer percentages, while at higher biopolymer contents a hardening effect of binder is observed.
Figure 3. Effect of chitosan and epichlorohydrin content [%] on the penetration of modified bitumen.
![Figure 3. Effect of chitosan and epichlorohydrin content [%] on the penetration of modified bitumen.](/cms/asset/e3f70ef3-fd0f-4a82-903b-ac72bd1e5733/trmp_a_2174357_f0003_ob.jpg)
The 1.0% (CHMB_0.0_E1) and 2.0% (CHMB_0.0_E2) cross-linking agent addition in the form of epichlorohydrin without biopolymer caused an increase in the penetration of 4.0%. A considerably more evident softening effect on bitumen was observed during their modification with a biopolymer in the presence of a cross-linking agent. The studies showed that a 1.0% (CHMB_1.0_E1) and 2.5% (CHMB_2.5_E1) addition of biopolymer modified in the presence of a 1.0% cross-linking agent increased the penetration of the bitumen by 23.0% and 17.0%, respectively. For higher chitosan percentages of 4.0% (CHMB_4.0_E1) and 5.5% (CHMB_5.5_E1) in the presence of a 1.0% cross-linking agent, a decrease in penetration was observed, but the modified bitumen was still softer than the reference sample. As is seen in Figure , the most notable changes in penetration were observed for bitumen modified in the presence of a 2.0% addition of the cross-linking agent. The greatest increase in the penetration of 39.0% was observed for the 2.5% (CHMB_2.5_E2) addition of chitosan. Smaller penetration increases of 26.0%, 29.0% and 24.0% were observed for bitumen containing 1.5% (CHMB_1.5_E2), 4.0% (CHMB_4.0_E2) and 5.5% (CHMB_5.5_E1) chitosan, respectively. The results indicate that the amount of biopolymer has a direct effect on the penetration of the modified bitumen.
The analysis of the results obtained on bitumen samples containing biopolymer suggests that bitumen modification with a small amount of chitosan leads to the incorporation of its molecules between the flat asphaltene structures, similar to nanomaterials (Wang et al., Citation2018). However, the chitosan linear structure does not allow sufficient separation of the asphaltene structures, making it impossible to obtain a more noticeable softening effect on the bitumen. This effect was observed at different levels when bitumen samples were modified with a mixture of chitosan and epichlorohydrin. Furthermore, the rate of bitumen softening is dependent on both the amount of biopolymer and the cross-linking agent. For a 1.0% addition of the cross-linking agent, the highest softening effect was observed for the lowest addition of chitosan. This is probably due to the stoichiometry of the biopolymer cross-linking reaction shown in Figure , which can lead to the formation of layered polymer networks responsible for the observed softening effect of the bitumen by distancing the asphaltene molecules from each other. When the binder contains a higher amount of biopolymer, the uncrosslinked excess behaves as a solid additive leading to a decrease in the penetration of the modified bitumen (Nciri et al., Citation2018). This thesis can also be confirmed from the results obtained for binders modified with the biopolymer in the presence of a higher amount of epichlorohydrin (2.0%), where the highest softening effect on the bitumen was observed for a chitosan content of 2.5%.
The observed increase in the penetration of the bitumen can also be explained based on the colloidal structure of the bitumen. The microstructure of bitumen contains micelles composed of asphaltene particles surrounded by a resinous phase (dispersed phase) and a dispersing phase-containing maltenes (Eberhardsteiner et al., Citation2015). The bitumen consistency and its properties depend mainly on the size of these micelles. Cross-linking of the biopolymer with epichlorohydrin can also lead to the formation of three-dimensional spherical polymer structures (Yu et al., Citation2021), which can be incorporated between the asphaltene molecules during the modification process, leading to the observed bitumen softening effect.
As shown in Figure , the modification of bitumen with chitosan and/or cross-linking agent also affects their softening point. The highest increase in the softening point of 2.8°C was observed for the bitumen containing 2.5% (REF_2.5) and 5.5% (REF_5.5) chitosan. However, the softening point of bitumens modified with the cross-linking agent alone was not changed. The obtained results indicate also that the softening point of biopolymer-modified bitumen containing both 1.0% (CHMB_0.0_E1) and 2.0% (CHMB_0.0_E2) epichlorohydrin increased by only 1.2°C compared to the reference sample. A slight decrease in the softening point was observed for bitumens modified with chitosan in the presence of a 1.0% cross-linking agent. The observed decrease was not significant and reached 1.2°C for bitumen containing 4.0% (CHMB_4.0_E1) chitosan. In the remaining samples the decrease was lower and ranged from 0.4°C to 0.8°C. The softening point of the bitumen containing 5.5% chitosan did not change compared to the sample with the same biopolymer percentage containing 1.0% epichlorohydrin. The higher decreases in softening point were observed for bitumens modified with chitosan in the presence of 2.0% epichlorohydrin. The studied results showed that the samples containing 1.0% (CHMB_1.0_E2) and 2.5% (CHMB_2.5_E2) of chitosan had a lower softening point by 3.0°C and 3.6°C, respectively, compared to the binder modified with the cross-linking agent only. On the other hand, the use of a higher amount of biopolymer caused a decrease in the softening temperature by 1.2°C and 2.6°C for 4% (CHMB_4.0_E2) and 5.5% (CHMB_5.5_E2) chitosan, respectively.
Figure 5. Effect of chitosan and epichlorohydrin content [%] on softening point of the modified bitumen.
![Figure 5. Effect of chitosan and epichlorohydrin content [%] on softening point of the modified bitumen.](/cms/asset/47227d83-3c10-472d-ab1f-317f058f3016/trmp_a_2174357_f0005_ob.jpg)
The observed changes in the softening point are not considerable and do not directly influence the functional properties of modified bitumens, but they suggest a conversion in their nanostructure at the molecular level. Lesueur, Citation2009 indicates that the rheological characteristics including softening point are directly related to the resin content in the bitumen. The author indicates that the increase in the softening point of bitumen is responsible for the increase in the content of resins, which are directly involved in the formation of the colloidal microstructure of bitumens acting as stabilisers. The resins are aromatic structures made up of 6–10 benzene rings containing most of the heteroatoms found in bitumens which are responsible for their polarity. The increase in the softening point of bitumens modified with chitosan and/or epichlorohydrin can be explained based on their chemical structure. As mentioned in the introduction, the chitosan molecule consists of parts A and D showing hydrophilic and hydrophobic properties, respectively. Therefore, it can be stated that chitosan behaves like bitumen resins and possibly incorporate into the structure of bitumen micelles or to form new micelles with larger diameters. This mechanism for increasing the softening point is also possible due to the potential formation of cross-linked chitosan beads filled with asphaltene structures which will also behave like resins and lead to an increase in temperature, A decrease in the softening point observed for bitumen binders modified with chitosan in the presence of a cross-linking agent can also be a confirmation of the occurring cross-linking reactions of the biopolymer. The chemical structure of chitosan contains external -OH and -NH2 groups with dipole moments of 1.70 and 1.30 D, respectively. These values indicate that the -OH groups are mainly responsible for the biopolymer polarity. Chitosan cross-linking chemical reaction in presence of epichlorohydrin, as is shown in Figure , occurs with the formation of an ether group. Reduction by chemical reaction of the number of -OH groups leads to a change in the electron cloud distribution of the biopolymer and a decrease in its polarity. Subsequently, it limits the possibility of incorporating/forming micelle structures in the bitumen volume and consequently lowers the softening point of the modified bitumen.
The penetration index (PI) is an indicator of the binder sensitivity to temperature changes (Zaumanis et al., Citation2013). As shown in Figure , the PI of bitumen modified with chitosan and/or epichlorohydrin is ranging from −1.13 to −0.35. The purple, green and red lines on the graph represent the cross-linking agent addition, whereas the values on the external outline represent the amount of chitosan used for binder modification. These rules apply to all of the radar charts presented in this study. The calculated values of PI indicate that bitumen modification with chitosan does not change the bitumen type in terms of its colloidal structure, regardless of the percentage addition of the cross-linking agent. Both the reference and modified bitumen are mixed sol–gel-type binders. Modification of bitumens with chitosan in the amount of 1.0% (REF 1.0) and 2.5% (REF 2.5) causes an increase in PI by 35% and 69%, respectively. At higher biopolymer amounts a decrease in the penetration index is observed. This means that the binder containing 2.5% of chitosan will be the most resistant to temperature fluctuations. As shown in Figure , the 35% increase in PI determined for both 1% and 2% epichlorohydrin addition also suggests the improved resistance to temperature changes of such modified bitumen binders. When bitumens were modified with the biopolymer in the presence of the cross-linking agent, an improvement in temperature susceptibility was also observed, but the changes were not as significant as in the case of modification of the bitumen with the biopolymer alone.
Figure 6. Effect of chitosan and epichlorohydrin addition on penetration index of the modified bitumen.
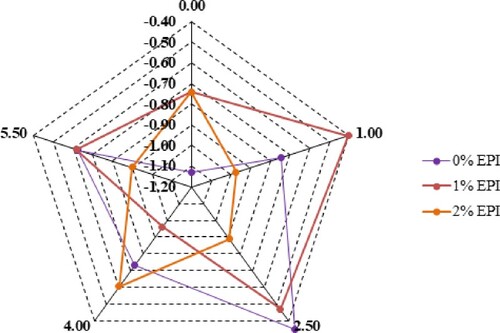
Figure shows the dependence of the dynamic viscosity of bitumens on the percentage of biopolymer and the cross-linking agent. The obtained results show that modification of the bitumen with chitosan causes an increase in the viscosity of the modified bitumen binder. The observed increase is proportional to the amount of added biopolymer chitosan and equals 20, 55, 75 and 117 mPa·s for bitumen with chitosan contents of 1.0% (REF 1.0), 2.5% (REF 2.5), 4.0% (REF 4.0) and 5.5% (REF 5.5). Taking into account the production of mineral-asphalt mixtures, the obtained increases in the bitumen viscosity are not favourable due to the need of heating the bitumen to higher operating temperatures to properly coat the aggregate. However, the modification of bitumen with chitosan and epichlorohydrin leads to a decrease in their dynamic viscosity compared to binders with the same content of the biopolymer alone. Figure shows that the application of a 1.0% addition cross-linking agent also causes a proportional increase in dynamic viscosity, but the observed increase is not as dynamic as in the case of modification of the binder using the chitosan without epichlorohydrin addition. For bitumen modified with biopolymer in the presence of a 2% crosslinking agent, a further decrease in viscosity was observed in comparison to both the reference sample and bitumen modified with epichlorohydrin only. The results show that the highest decrease in dynamic viscosity of 62 mPa·s was obtained for the bitumen containing 1.0% (CHMB 1.0 E2) and 2.5% (CHMB 2.5 E2) of chitosan. The results show that the chitosan modification of the bitumen in the presence of a cross-linking agent leads to a decrease in its viscosity, thereby providing technological advantages in terms of mixture production. The observed reduction in viscosity is seen and at the same time it confirms the chitosan cross-linking process with epichlorohydrin taking place inside bitumen according to the scheme presented in Figure .
3.2. Chemistry of the modified bitumen
The chemical changes occurring in the bitumen molecular structure during its modification with biopolymer and/or cross-linking agent were investigated using FTIR spectroscopy. As shown in Figures (a–c), FTIR spectra of both the reference and modified bitumen show typical bands for this type of material. At a wavenumber of about 2900 and 2800 cm−1 there are two characteristic peaks corresponding to vibrations of the -CH2 and -CH3 groups, respectively (Malinowski et al., Citation2019). At a wavelength of about 1570 cm−1 there is a small peak from the vibrations of the aromatic structures of the bitumens. The polyaromatic structures can be seen from four characteristic peaks in the wavelength range 900–700 cm−1. The FTIR spectrum of asphaltenes also contains a band at about 1000 cm−1 corresponding to vibrations of the -S = O group (Hou et al., Citation2018).
Figure 8. FTIR spectra of bitumen modified with different chitosan contents with the use of 0% (a), 1%(b) and 2%(c) of epichlorohydrin.
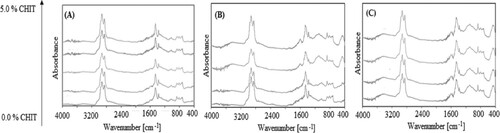
The FTIR spectra shown in Figure (a) indicate that bitumen modification with biopolymer does not lead to any changes in their chemical structure. All spectra contain the same bands with similar intensities and mutual proportions. However, some structural changes of bitumens can be observed after their modification with biopolymer in the presence of 1% crosslinking agent addition. Figure (b) shows that modification of the bitumen with 1% (CHMB 1.0 E1) chitosan does not cause any evident changes in the FTIR spectra, but increasing the amount of biopolymer to 2.5% (CHMB 2.5 E1) causes a new peak forming at a wavelength of about 500 cm−1 and an increase in the intensity of the peak occurring at about 900 cm−1. The spectra of bitumens modified with 4% (CHMB 4.0 E1) and 5.5% (CHMB 5.5 E1) biopolymer addition also show an increase in the intensity of the band occurring at the wavelength of 1600 cm−1. These differences suggest that some changes in the structure of bitumen occurred, probably due to the incorporation of cross-linked biopolymer. In the wavelength range 1200–1000 cm−1, broadband changes were also observed. They may be indicative of the formation of connections between chitosan and/or epichlorohydrin and S atoms occurring naturally in bitumen (Hou et al., Citation2018). Similar changes are also seen for bitumens containing more biopolymer in the presence of 1% cross-linking agent and for all tested bitumens obtained by modification in the presence of 2.0% cross-linking agent addition. The additional band seen on the FITR spectrum occurring at about 1130 cm−1 may suggest the presence of –CH–OH groups, and the additional band seen at about 960 cm−1 may indicate the presence of –O–CH2 groups. The simultaneous presence of these two bands may confirm the chemical cross-linking process of chitosan taking place in the environment of the liquefied asphalt binder. On the other hand, the presence of a band at a wavelength of about 880 cm−1 may indicate the presence of a cross-linking agent that has not undergone a cross-linking reaction.
3.3. Energy parameters obtained from the SFE method
The effect of chitosan and/or epichlorohydrin on the cohesive and adhesive properties of the modified bitumens was then analysed based on the surface-free energy components. Figure shows the effect of biopolymer and/or cross-linking agent content on the cohesion energy of the tested bitumens. The process of chitosan cross-linking leads to a decrease in the cohesion energy of the bitumen. But it should be noted that for lower percentages of the modifier, the dynamics of change is lower than after exceeding 2.5% w/w chitosan. The cohesion energy describes the interactions that occur between the components of bitumen and directly affects the ability to prevent the detrimental effects of water on the properties of designed asphalt mixtures (Liu et al., Citation2017). The obtained values of cohesion energy of binders modified with chitosan indicate that an increase in its content improves the intermolecular interactions occurring in the volume of the bitumen.This effect can be explained at the level of chemical interactions occurring between the modifier and the components of the bitumen. As mentioned in Section 3.1, during bitumen modification with chitosan, its molecules can incorporate between the asphaltene structures leading to only a slight increase in penetration with simultaneous changes in the cohesion energy of the binder. Such results suggest that the incorporation of biopolymer molecules has a positive effect on the intermolecular interactions occurring between bitumen components presumably by deforming their electron cloud (Mousavi et al., Citation2016). This may result in the formation of local positive and negative charges, which may increase the cohesion energy of the bitumen through electrostatic interactions. The cohesion energy reduction observed for biopolymer-modified binders in the presence of a cross-linking agent indicates that the resulting three-dimensional structures of crosslinked chitosan may cause the observed changes in two ways. Firstly, the electron cloud deformation of the asphaltene components may still occur, but their considerable distancing results in a decrease in electrostatic interactions between them and consequently a decrease in the cohesion energy of the modified binder. Secondly, considering the colloidal structure of bitumens, the resulting cross-linked biopolymer can cause an increase in micelle size (Fawcett & McNally, Citation2003) by embedding itself between asphaltene structures while decreasing the interaction strength between them (Sheu, Citation1996). This, in turn, leads to a decrease in dynamic viscosity and an increase in penetration characterised in Section 3.1. ANOVA statistical analysis (Table and Table ) showed that changing the EPI content did not significantly affect the cohesion energy of the modified bitumen (P-value > 0.05). However, the statistical data collected in Table show a statistically significant effect of chitosan and the interaction between chitosan and epichlorohydrin content on the cohesion energy of the investigated bitumens. In both cases, the P-value = 0.000 was obtained.
Table 2. Analysis of variance for cohesion energy.
Table 3. Analysis of variance for dry and wet adhesion energy.
Table 4. Analysis of variance for ER1 and ER2.
Modelling of adhesion in dry (Wdry) and wet conditions (|Wwet|) was performed for two different aggregates i.e. limestone and granite. Figures (a–b) show that bitumen modification with biopolymer and/or cross-linking agent affects the adhesion of the binders obtained to the aggregate, with significantly lower adhesion obtained in the bitumen-limestone system (Figures (a) and (b)). The dependencies observed for both granite and limestone indicate that for bitumen modified with the biopolymer, the Wdry value is dependent on the amount of chitosan added. The greatest increase in adhesion for both aggregates analysed was obtained for bitumen-containing 2.5% chitosan addition (REF 2.5). Slightly different Wdry dependencies were obtained for bitumen modified with biopolymer in the presence of 1.0% cross-linking agent addition, where no considerable changes in dry adhesion were observed for both studied aggregates at 1% (CHMB 1.0 E1) and 2.5% (CHMB 2.5 E1) biopolymer content. However, for its higher additions of 4.0% (CHMB 4.0 E1) and 5.5% (CHMB 5.5 E1), decreases in adhesion to the limestone of 1.55% and 1.89% were observed, respectively. For granite, lower decreases in adhesion were observed for the modified aggregate of 1.27% and 1.52%, respectively, compared to modification with the cross-linking agent. However, for bitumens modified with biopolymer at 2% epichlorohydrin a decrease in adhesion was observed for both aggregates proportional to increasing biopolymer content. In summary, it can be concluded that bitumen modification with a cross-linking agent improves adhesion to both limestone and granite aggregates. ANOVA analysis showed that a statistically significant effect on Wdry was observed for changes in the percentages of EPI and CHIT as well as their interaction. In addition, Wdry also statistically significantly depended on the type of mineral aggregate used in the analyses. However, its interaction with the percentage of EPI and CHIT is not statistically significant. Statistical analysis also showed that the interaction between all the independent variables analysed in this work is not statistically significant.
Figure (b) shows the results of adhesion modelling under wet conditions. |Wwet| value describes the water and frost resistance of the mixture. The smaller that value, the more water and frost resistant the asphalt mixture. All the |Wwet| values obtained on both granite and limestone samples are negative indicating the possibility of debonding at the aggregate-binder interface in water. The results shown in Figure (b) indicate that the mineral-asphalt mixtures obtained with the use of cross-linked chitosan-modified bitumen would be more resistant to water and frost. For the bitumens modified with the cross-linking agent, a decrease in |Wwet| was observed as the percentage of the cross-linking agent increased. This effect is stronger for limestone, where almost twice the decrease of about 1.3% was obtained. A similar dependence of cross-linking agent percentage on |Wwet| was observed for binders modified with 1% chitosan addition. In the case of bitumen modified with 2.5% (CHMB 2.5 E1) chitosan addition for limestone and granite different dependences were observed. For the first aggregate, an increase in the cross-linking agent content resulted in a decrease and an increase in the |Wwet| value for the second aggregate. For bitumens modified with biopolymer above 2.5% and increasing amounts of a cross-linking agent, an increase in |Wwet| values was observed for both limestone and granite. In contrast, the statistical analysis conducted for |Wwet| indicates that the adhesion of the investigated modified bitumen with water is statistically significantly affected by the type of applied mineral aggregate, the percentage content of CHIT as well as the interaction between the percentage content of EPI and CHIT. Interestingly, changing the percentage of a cross-linking agent does not cause statistically significant changes in the |Wwet| values of the analysed bitumen. ANOVA analysis also showed that there was no statistically significant effect of the interaction of mineral aggregate and percentage of EPI and CHIT with the determined |Wwet| values.
The water and frost resistance of mineral-asphalt mixtures can be evaluated based on the ER1 and ER2 coefficients determined by equations described in section 3.5. Higher values of these coefficients indicate higher resistance of the mineral-asphalt mixture to water and frost damage. The ER1 coefficient determines the work of debonding when water displaces bitumen from the bitumen-aggregate interface, while the ER2 coefficient describes the wettability of aggregate by the bitumen (Liu et al., Citation2017). As indicated by the results of analyses (Figure (a)), the modification of bitumen with sole biopolymer leads to an increase in the ER1 coefficient value, while the highest increase in ER1 coefficient value was observed for the biopolymer content of 2.5% (REF 2.5) and 4% (REF 4.0). When the binder was modified with a higher amount of chitosan, a decrease in the analysed coefficient was observed, but the value was still higher than for the reference sample. This trend is observed for both limestone and granite. Modification of bitumen with epichlorohydrin also leads to an increase in the work of debonding of bitumen, which is indicated by the increasing value of the ER1 coefficient observed for both aggregates. However, the studies indicate that in relation to bitumen modified with cross-linking agent only, the addition of biopolymer causes a decrease in the work of debonding on both limestone and granite surfaces. The results show that a small addition of biopolymer to bitumen at the level of 1% does not affect its wettability on both limestone and granite surfaces with respect to the ER2 coefficient (Figure (b)). On the other hand, an increase in the percentage of biopolymer in bitumen causes a decrease in the wettability of selected aggregates. A similar trend was observed for bitumen binders modified with the cross-linking agent alone. However, it is worth noting that its combination with the biopolymer causes a systematic increase in wettability proportional to both the amount of chitosan and epichlorohydrin. In the case of both ER1 and ER2, the effect of the used aggregate, the percentage content of EPI and CHIT as well as the interaction between the content of biopolymer and cross-linking agent proved statistically significant. On the other hand, interactions between the mineral aggregate and the percentage of EPI and CHIT proved to be statistically insignificant on the ER1 and ER2 values.
5. Conclusions
The basic research on bitumen showed that non-cross-linked and chemically-cross-linked chitosan can be successfully applied as a modifier. With the addition of non-cross-linked biopolymer the softening effect of bitumen is observed; however, it is much lower than in the case of bitumen with chemically cross-linked biopolymer. The formation of cross-linked chitosan structures causes the asphaltenes to be more separable, which translates into measured penetration values of the modified bitumens. At the same time, the cross-linking reaction of chitosan in the volume of bitumens leads to a lower softening point by about 2.5°C, which can be reflected in lower resistance to permanent deformation. Modification of bitumen with the biopolymer alone leads to an increase in its viscosity by about 125 mPa·s. However, the chitosan crosslinking leads to a decrease in viscosity of the modified bitumen by about 50 mPa·s, which directly translates into technological advantages related to coating the aggregate at a lower temperature. Moreover, according to the investigations carried out, the modification of bitumen with either a biopolymer or a mixture of biopolymer and cross-linking agent in an appropriate ratio causes an increase in its cohesion energy by a maximum of 7%. The modification of bitumen with epichlorohydrin showed an increase in ER1 parameters for combinations with limestone and granite by about 3. Based on the results, it can be concluded that the best effect of bitumen modification with biopolymer was obtained with 2.5% chitosan and 1% epichlorohydrin. This is because at this ratio of biopolymer and cross-linking agent, the cross-linking process that is responsible for the observed changes in bitumen properties is already visible.
6. Future perspectives
The results of the pilot study presented in the paper are a starting point for further research. In future, extensive rheological studies of DSR are planned. In addition, we intend to conduct a full analysis of short-term and long-term ageing as well as UV ageing of asphalt binders modified with chemically cross-linked chitosan. This work we intend to carry out on the basis of rheological and chemical ageing indices and microstructural analysis carried out by NMR spectroscopy and AFM microscopy. The research work will also include a study of the stability of chitosan under the influence of environmental factors. In the aspect of mineral-asphalt mixtures with biopolymer-modified asphalt, we intend to conduct further research work in terms of rutting and the formation of low-temperature cracks.
Disclosure statement
No potential conflict of interest was reported by the author(s).
Additional information
Funding
References
- Andrzejuk, W., Szewczak, A., Fic, S., & Łagód, G. (2020). Wettability of asphalt concrete with natural and recycled aggregates from sanitary ceramics. Materials, 13(17), 3799, https://doi.org/10.3390/ma13173799
- Arabani, M., Ranjbar Pirbasti, Z., & Hamedi, G. H. (2020). Investigating the impact of zeolite on reducing the effects of changes in runoff acidity and the moisture sensitivity of asphalt mixtures. Construction and Building Materials, 268, 121071, https://doi.org/10.1016/j.conbuildmat.2020.121071
- Bao, D. X., Yu, Y. Y., & Zhao, Q. M. (2019). Evaluation of the chemical composition and rheological properties of bio-asphalt from different biomass sources. Road Materials and Pavement Design, 21(7), 1829–1843. https://doi.org/10.1080/14680629.2019.1568287
- Bhasin, A., Masad, E., Little, D., & Lytton, R. (2006). Limits on adhesive bond energy for improved resistance of hot-mix asphalt to moisture damage. Transportation Research Record, 1970(1), 3–13. https://doi.org/10.3141/1970-03
- Camargo, I. G. d. N., Dhia, T. b., Loulizi, A., Hofko, B., & Mirwald, J. (2021). Anti-aging additives: Proposed evaluation process based on literature review. Road Materials and Pavement Design, 22(S1), S134–S153. https://doi.org/10.1080/14680629.2021.1906738
- Chen, C., Podolsky, J. H., Williams, R. C., & Cochran, E. W. (2018). Rheological properties and effects of aging on acrylated epoxidised soybean oil monomer-modified asphalt binder. Road Materials and Pavement Design, 21(2), 347–373. https://doi.org/10.1080/14680629.2018.1491883
- Chen, Y., Dong, S., Wang, H., Gao, R., & You, Z. (2020). Using surface free energy to evaluate the fracture performance of asphalt binders. Construction and Building Materials, 240, 118004. https://doi.org/10.1016/j.conbuildmat.2020.118004
- Concha, J. L., Arteaga-Pérez, L. E., Alpizar-Reyes, E., Segura, C., Gonzalez-Torre, I., Kanellopoulos, A., & Norambuena-Contreras, J. (2022). Effect of rejuvenating oil type on the synthesis and properties of alginate-based polynuclear capsules for asphalt self-healing. Road Materials and Pavement Design, 1 . https://doi.org/10.1080/14680629.2022.2092026
- Cuadri, A. A., Navarro, F. J., & Partal, P. (2020). Synergistic ethylcellulose/polyphosphoric acid modification of bitumen for paving applications. Materials and Structures/Materiaux et Constructions, 53(1), 1–13. https://doi.org/10.1617/s11527-019-1437-7
- Eberhardsteiner, L., Füssl, J., Hofko, B., Handle, F., Hospodka, M., Blab, R., & Grothe, H. (2015). Influence of asphaltene content on mechanical bitumen behavior: Experimental investigation and micromechanical modeling. Materials and Structures/Materiaux et Constructions, 48(10), 3099–3112. https://doi.org/10.1617/s11527-014-0383-7
- Fawcett, A. H., & McNally, T. (2003). Polystyrene and asphaltene micelles within blends with a bitumen of an SBS block copolymer and styrene and butadiene homopolymers. Colloid and Polymer Science, 281(3), 203–213. https://doi.org/10.1007/s00396-002-0762-4
- Gallego, J., Rodríguez-Alloza, A. M., & Saiz-Rodríguez, L. (2020). Evaluation of warm rubberized stone mastic asphalt mixtures through the Marshall and Gyratory compactors. Materials, 13(2), 265. https://doi.org/10.3390/ma13020265
- Habal, A., & Singh, D. (2021). Establishing threshold value of surface free energy and binder bond strength parameters for basaltic asphalt mixes. Road Materials and Pavement Design, 23(8), 1877–1899. https://doi.org/10.1080/14680629.2021.1925576
- Hernández, N., Williams, R. C., & Cochran, E. W. (2014). The battle for the “green” polymer. Different approaches for biopolymer synthesis: Bioadvantaged vs. bioreplacement. Organic and Biomolecular Chemistry, 12(18), 2834–2849. https://doi.org/10.1039/c3ob42339e
- Holleran, I., Wilson, D. J., Masad, E., Holleran, G., & Alrashydah, E. (2021). Effect of bio-rejuvenator on recycled porous asphalt mixes. Road Materials and Pavement Design, 340. https://doi.org/10.1080/14680629.2021.2017331
- Hou, X., Lv, S., Chen, Z., & Xiao, F. (2018). Applications of Fourier transform infrared spectroscopy technologies on asphalt materials. Measurement, 121(January), 304–316. https://doi.org/10.1016/j.measurement.2018.03.001
- Hu, Q., & Luo, Y. (2021). Chitosan-based nanocarriers for encapsulation and delivery of curcumin: A review. International Journal of Biological Macromolecules, 179, 125–135. https://doi.org/10.1016/j.ijbiomac.2021.02.216
- Jiménez del Barco Carrión, A., Carvajal-Muñoz, J. S., Lo Presti, D., & Airey, G. (2019). Intrinsic adhesive and cohesive assessment of the moisture sensitivity of bio-rejuvenated recycled asphalt binders. Road Materials and Pavement Design, 20(sup1), S347–S364. https://doi.org/10.1080/14680629.2019.1588778
- Lesueur, D. (2009). The colloidal structure of bitumen: Consequences on the rheology and on the mechanisms of bitumen modification. Advances in Colloid and Interface Science, 145(1–2), 42–82. https://doi.org/10.1016/j.cis.2008.08.011
- Li, R., Chen, J., Zhou, T., & Pei, J. (2017). Optimal synthetic conditions for oxetane-substituted chitosan (OXE-CHI) and polyethylene glycol (PEG) network self-healing agent. Materials Express, 7(5), 411–416. https://doi.org/10.1166/MEX.2017.1387
- Liu, S., Yu, X., & Dong, F. (2017). Evaluation of moisture susceptibility of foamed warm asphalt produced by water injection using surface free energy method. Construction and Building Materials, 131, 138–145. https://doi.org/10.1016/j.conbuildmat.2016.11.072
- Malinowski, S., Jaroszyńska-Wolińska, J., & Herbert, P. A. F. (2019). Theoretical insight into plasma deposition of laccase bio-coating formation. Journal of Materials Science, 54(15), 10746–10763. https://doi.org/10.1007/s10853-019-03641-2
- Malinowski, S., Wróbel, M., Bandura, L., Woszuk, A., & Franus, W. (2022). Use of new green bitumen modifier for asphalt mixtures recycling. Materials 2022, 15(17), 6070. https://doi.org/10.3390/MA15176070
- Mishra, V., Singh, D., & Habal, A. (2020). Investigating the condition number approach to select probe liquids for evaluating surface free energy of bitumen. International Journal of Pavement Research and Technology, 13(1), 10–19. https://doi.org/10.1007/s42947-019-0097-x
- Mousavi, M., Abdollahi, T., Pahlavan, F., & Fini, E. H. (2016). The influence of asphaltene-resin molecular interactions on the colloidal stability of crude oil. Fuel, 183, 262–271. https://doi.org/10.1016/j.fuel.2016.06.100
- Nciri, N., Shin, T., Lee, H., & Cho, N. (2018). Potential of waste oyster shells as a novel biofiller for hot-mix asphalt. Applied Sciences (Switzerland), 8(3), 415, https://doi.org/10.3390/app8030415
- Pérez, I. P., Rodríguez Pasandín, A. M., Pais, J. C., & Alves Pereira, P. A. (2019). Use of lignin biopolymer from industrial waste as bitumen extender for asphalt mixtures. Journal of Cleaner Production, 220, 87–98. https://doi.org/10.1016/j.jclepro.2019.02.082
- Porto, M., Caputo, P., Loise, V., Eskandarsefat, S., Teltayev, B., & Rossi, C. O. (2019). Bitumen and bitumen modification: A review on latest advances. Applied Sciences (Switzerland), 9(4), 742. https://doi.org/10.3390/app9040742
- Sheu, E. Y. (1996). Physics of asphaltene micelles and microemulsions - theory and experiment. Journal of Physics Condensed Matter, 8(25 SUPPL. A), A125–A141. https://doi.org/10.1088/0953-8984/8/25A/009
- Tu, L. L., Wu, S. P., Liu, G., Zhou, X. X., & Ma, S. K. (2016). Effect of the welan gum biopolymer on rheological properties and storage stability of bitumens. Journal of Testing and Evaluation, 44(6), 2211–2218. https://doi.org/10.1520/JTE20150119
- van Oss, C. J., Chaudhury, M. K., & Good, R. J. (1988). Interfacial Lifshitz—van der Waals and polar interactions in macroscopic systems. Chemical Reviews, 88(6), 927–941. https://doi.org/10.1021/cr00088a006
- Wang, P., Zhai, F., Dong, Z. J., Wang, L. Z., Liao, J. P., & Li, G. R. (2018). Micromorphology of asphalt modified by polymer and carbon nanotubes through molecular dynamics simulation and experiments: Role of strengthened interfacial interactions. Energy and Fuels, 32(2), 1179–1187. https://doi.org/10.1021/acs.energyfuels.7b02909
- Wen, Y., Wang, Y., Zhao, K., & Sumalee, A. (2017). The use of natural rubber latex as a renewable and sustainable modifier of asphalt binder. International Journal of Pavement Engineering, 18(6), 547–559. https://doi.org/10.1080/10298436.2015.1095913
- Woszuk, A., Wróbel, M., Bandura, L., & Franus, W. (2020). Brick debris dust as an ecological filler and Its effect on the durability of asphalt Mix. Materials, 13(21), 5023. https://doi.org/10.3390/ma13215023
- Wróbel, M., Woszuk, A., Ratajczak, M., & Franus, W. (2020). Properties of reclaimed asphalt pavement mixture with organic rejuvenator. Construction and Building Materials, 271, 121514. https://doi.org/10.1016/j.conbuildmat.2020.121514
- Yu, X., Zhang, J., & Zheng, Y. (2021). Perchlorate adsorption onto epichlorohydrin crosslinked chitosan hydrogel beads. Science of the Total Environment, 761(xxxx), 143236. https://doi.org/10.1016/j.scitotenv.2020.143236
- Zaumanis, M., Mallick, R., & Frank, R. (2013). Evaluation of rejuvenator’s effectiveness with conventional mix testing for 100% reclaimed asphalt pavement mixtures. Transportation Research Record, 2370(1), 17–25. https://doi.org/10.3141/2370-03
- Zhu, J., Birgisson, B., & Kringos, N. (2014). Polymer modification of bitumen: Advances and challenges. European Polymer Journal, 54(1), 18–38. https://doi.org/10.1016/j.eurpolymj.2014.02.005