Abstract
Antibodies and antigen-binding fragments (Fabs) can be used to modify the surface of nanoparticles for enhanced target binding. In our previous work, site-specific conjugation of Fabs to polymeric micelles using conventional methods was limited to approximately 30% efficiency, possibly due to steric hindrance related to macromolecular reactants. Here, we report a new method that enables conjugation of Fabs onto a micelle surface in a controlled manner with up to quantitative conversion of nanoparticle reactive groups. Variation of (i) PEG spacer length in a heterofunctionalized cross-linker and (ii) Fab/polymer feed ratios resulted in production of nanoparticles with a range of Fab densities on the surface up to the theoretical maximum value. The biological impact of variable Fab density was evaluated in vitro with respect to cell uptake and cytotoxicity of a drug-loaded (SN38) targeted polymeric micelle bearing anti-EphA2 Fabs. Fab conjugation increased cell uptake and potency compared with non-targeted micelles, although a Fab density of 60% resulted in decreased uptake and potency of the targeted micelles. Altogether, our findings demonstrate that conjugation strategies can be optimized to allow control of Fab density on the surface of nanoparticles and also that Fab density may need to be optimized for a given cell-surface target to achieve the highest bioactivity.
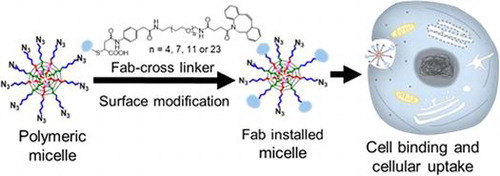
1. Introduction
Functionalizing nanoparticles with targeting biomolecules provides biofunctionality, which allows access to natural biological substrates and/or processes that could improve their desired function [Citation1–3]. Covalent attachment of biomolecules to nanoparticles has great potential for generating therapeutics with advanced properties such as cell targeting and penetration through biological barriers [Citation4–6]. However, these macromolecular reactions usually present low yield and conjugation efficiencies, as they are more challenging than analogous small-molecule reactions due to factors such as steric hindrance, diffusion limitations and high solvent sensitivity [Citation7–9]. Thus, bioconjugation approaches that allow controlled nanoparticle modification with high yield will be key for development of novel diagnostic and therapeutic applications that demand tunable nanoparticle properties.
Among macromolecular ligands, antibodies and antibody fragments (Fabs) present exceptional advantages for nanoparticle biofunctionalization, including tunable binding affinity to antigens and high specificity [Citation10]. Moreover, the relatively smaller size of Fabs compared with antibodies is a substantial benefit for modifying the surface of nanoparticles without significantly altering the dimensions of the systems. Installation of Fabs onto nanoparticles has been accomplished by reaction of active esters with random Fab lysine amine groups [Citation11], by Michael addition of maleimide to cysteine thiols in either reduced Fab disulfide, or genetically engineered Fabs [Citation12], or by using heterofunctionalized cross-linkers introducing alkyne groups to Fabs that enable click chemistry with azide-functionalized surfaces [Citation13,14]. Genetically engineered Fabs offer the advantage of site-specific conjugation, which allows control of Fab orientation on the nanoparticle surface. We have previously explored these strategies for installing Fabs onto the poly(ethylene glycol) (PEG) shell of polymeric micelles [Citation15,16], although the number of Fabs on the micelles by using these methods was limited to 20–30% modification of the PEG chains. Thus, new conjugation strategies are required for improving Fab installation efficiency while also maintaining targeting ability.
Herein, we studied a series of heterobifunctional cross-linkers containing PEG spacers of different lengths designed to alleviate steric effects associated with the Fab-nanoparticle conjugation and improve installation efficiency. Heterobifunctional cross-linkers were synthesized to contain a phenylmaleimide functional group for stable conjugation to Fab-thiols followed by a PEG spacer with variable length, i.e. 4, 7, 11 and 23 -CH2CH2O- units, and a terminal strained alkyne group (DBCO) for reaction with azide-containing nanoparticles. First, the effect of PEG length on conjugation efficiency was studied on model micelles based on cross-linked polyion complex (PIC) micelles bearing azide groups on their surface [Citation15]. Next, we developed polymeric micelles containing the anticancer agent SN38 linked through enzymatically sensitive bonds, which can provide selective drug activation and minimal drug leakage in biological settings. These SN38-loaded micelles (SN38/m) were further modified with 1C1 Fabs (directed to the Ephrin receptor EphA2 on cancer cells) by using the heterobifunctional linkers having 4 or 23 unit PEG spacers. The cellular uptake and cytotoxicity of the SN38/m was evaluated, focusing on the effect of the PEG spacer and Fab density on the surface of micelles. Our results showed that the elongation of the PEG spacer improved the conjugation efficiency on the surface of micelles, while sustaining similar micelle binding ability, cellular uptake and cytotoxicity under the same Fab density. In addition, it was found that installation of Fabs improved the activity of the drug-loaded micelles, even for those prepared with the longest PEG spacer, although biological activity was impaired at high Fab density. This study demonstrates that conjugation of Fabs to nanoparticles can be optimized by linker design and provides insight into the practical formulation of effective ligand-installed nanomaterials.
2. Methods
2.1. Materials
α-azido-ω-amino poly(ethylene glycol) (N3-PEG-NH2, Mn: 12,000 Da) was purchased from NOF Co., Inc. (Tokyo, Japan). N-carboxyl anhydride of γ-Benzyl-L-Aspartate (NCA-BLA) and N-carboxyl anhydride of γ-trifluoacetyl-L-Lysine (NCA-LL(TFA)) were bought from Chuo Kaseihin Co., Inc. (Tokyo, Japan). Benzene, dimethyl formamide (DMF), dimethyl sulfoxide (DMSO), diethyl ether and phosphate buffered saline (PBS) were obtained from Wako Pure Chemical Co., Inc. (Osaka, Japan) and used as received. Furfurylamine was purchased from Tokyo Chemical Industry Co., Ltd (Tokyo, Japan) and purified with conventional distillation. Dulbecco’s modified Eagle’s medium (DMEM), fetal bovine serum (FBS) and penicillin-streptomycin were bought from Sigma (St. Louis, Missouri, USA). Heterofunctionalized cross-linkers with maleimide and DBCO moiety spaced by different lengths of PEG (named PMP4D, PMP7D, PMP11D and PMP23D) were purchased from SynChem (Elk Grove Village, Illinois, USA). SN38 prodrug functionalized with maleimide reactive moiety and enzyme-cleavable phenylalanine-lysine-p-aminocarbamate (Phe-Lys-PABC) linker was provided at 62% purity (HPLC) by SynChem (Elk Grove Village, Illinois, USA). Alexa Fluor 555-NHS and Alexa Fluor 647-NHS were purchased from Invitrogen (Carlsbad, California, USA). Alexa Fluor 647-DBCO was purchased from Sigma Aldrich (St. Louis, Missouri, USA). 1-Ethyl-3-(3-dimethylaminopropyl) carbodiimide hydrochloride (EDC), tris(2-carboxyethyl) phosphine (TCEP) and dehydroascorbic acid (DHA) were brought from Life Technology Co. (Grand Island, USA).
2.2. Cells
Human prostate PC3 cancer cells were obtained from Riken (Wako, Saitama, Japan). Cells were cultured at 37 °C and 5% CO2 in DMEM containing 10% fetal bovine serum and 1% penicillin-streptomycin. Cells were passaged until 90% confluence at a ratio of 1:10 by using trypsin (Wako, Saitama, Japan) and the culture medium was changed the next day.
2.3. Polymer synthesis
N3-PEG-b-poly(benzyl-L-aspartate) (N3-PEG-PBLA) and N3-PEG-b-poly(L-Lysine(TFA)) (N3-PEG-PLL(TFA)) copolymers were synthesized by ring-opening polymerization initiated by the amine group of N3-PEG-NH2 as described before [Citation17,18]. Briefly, N3-PEG-NH2 (1 g, 0.083 mmol) was lyophilized in benzene overnight followed by dissolving in anhydrous DMF. Then NCA-BLA (0.934 g, 3.75 mmol) or NCA-LL(TFA) (1.005 g, 3.75 mmol) were dissolved in anhydrous DMF. NCA solutions were transferred to PEG solutions under Argon. The reaction was kept at 35 °C for 3 days and the polymer products were collected as white powder following precipitation in diethyl ether. Degree of polymerization of PBLA and PLL(TFA) blocks was determined to be 40 and 41 respectively by proton nuclear magnetic resonance (1H-NMR, 400 MHz, DMSO-d6, 80 °C. δ(ppm) = 3.5–3.8 (–CH2CH2O–, PEG backbone), 4.2–4.4 (–CH–, PBLA backbone), 4.9–5.1 (–CH2–, PBLA side chain), 7.1–7.3 (–C6H5, PBLA side chain), 1.2–2.0 (–CH2CH2CH2CH2NH–, PLL(TFA) side chain)). The narrow degree of polydispersity of both polymers was confirmed by gel permeation column analysis (DMF containing 10 mM LiCl, 40 °C). N3-PEG-b-poly(aspartatic acid) (N3-PEG-PAsp) and N3-PEG-b-poly(L-Lysine) (N3-PEG-PLL) were obtained by removing protecting groups in 0.1 N NaOH solution followed by dialysis against Milli-Q water. N3-PEG-b-PLL was fluorescently labeled by mixing with Alexa Fluor 555-NHS at equimolar concentration, and purified by PD-10 column (GE Healthcare; Little Chalfont, UK).
2.4. Preparation of PIC micelles
N3-PEG-PLL labeled with Alexa Fluor 555 and N3-PEG-PAsp block copolymers were combined to form PIC micelles as described previously [Citation15]. Briefly, the optimal polymer ratio was determined to be 1:1 using dynamic light scattering (DLS) analysis (Zetasizer, Malvern, UK). Micelles were cross-linked using EDC and cross-linking effectiveness was confirmed by adding NaCl and using DLS to determine whether the micelles remained intact. Size, polydispersity index (PDI), and derived count rate (kcps) of dye-labeled cross-linked micelles were also measured using DLS. The polymer association number of the micelles was determined to be approximately 10 by static light scattering (SLS) measurement (Wyatt Technology, Santa Barbara, USA) [Citation15].
2.5. Preparation of Fab-cross-linker
Anti-EphA2 (1C1) containing an engineered cysteine at position A114 was used in this study. The Fab was engineered to contain a HIS-tag, which was cleaved by using thrombin prior to conjugation, as described in our previous work [Citation15]. The disulfide-protected A114 cysteine was uncapped with TCEP and digested light chains and heavy chained were re-oxidized into reactive Fabs with DHA. Four different cross-linkers containing PEG chains of different lengths (PEG4, PEG7, PEG11, and PEG23) were conjugated to 1C1 Fab via Michael addition of thiol on Fab and maleimide on the cross-linker. The product was purified by ceramic hydroxyapatite column chromatography to remove excess cross-linker. Mass spectrometry was used to confirm that conjugation was successful, as well as to determine whether the cross-linker conjugated specifically to the heavy chain of the Fab. A mock Fab with similar amino acid sequence, but no specific binding affinity to EphA2, was modified in the same method. Conjugation efficiencies determined by mass spectrometry were similar for all cross-linkers, i.e. ≥90%.
2.6. Conjugation of Fab to PIC micelles
Fab-cross-linker conjugates (10 mg/ml based on Fab in PBS) were mixed with cross-linked, azide-bearing PIC micelles (2 mg/ml based on polymer in PBS) and reacted at 37 °C under shaking overnight, followed by removal of excess Fab using ultrafiltration (MWCO: 10 kDa). Conjugation reactions were carried out at Fab/azide molar feeds of 0.1, 0.5, 1, 2, 4, and 8 for each cross-linker. Two different methods were used to determine how many Fabs were conjugated to each PIC micelle. First, UV-vis absorbance analysis was used to quantify the ratio of Alexa Fluor 555-labeled PIC micelles to Fabs, i.e. absorptions at 555 nm and 280 nm, respectively, which was then used to calculate how many Fabs were installed on each micelle as previously described [Citation15]. Second, excess Alexa Fluor 647-DBCO was added to the Fab-installed micelles to quench the remaining free azides. The number of dye molecules per nanoparticle was calculated by comparing the ratio of the UV absorption of Alexa Fluor 647 dyes to Alexa Fluor 555. Thus, the number of Fabs and the number of free azides should theoretically add up to 10, since each micelle contains 10 polymers as mentioned above.
2.7. Preparation of enzyme-sensitive SN38-loaded micelles
Carrier polymer N3-PEG-Poly(Aspartate-Furan) was synthesized by aminolysis of N3-PEG-PBLA based on the previous method with slight modification [Citation19]. Briefly, N3-PEG-PBLA (100 mg, 5 μmol) was dissolved in benzene and freeze-dried overnight. Then N3-PEG-PBLA was dissolved in 3.8 ml of anhydrous DMF and furfurylamine (1.2 ml, 12 mmol) was added under Ar protection. The reaction was kept at 35 °C for 12 h and the product was obtained as white powder through diethyl ether followed by filtration and vacuum drying. The substitution extent of furan moiety on the side chain was characterized by 1H-NMR (400 MHz, DMSO-d6, 80 °C. δ(ppm) = 3.5–3.8 (–CH2CH2O–, PEG backbone), 4.2–4.4 (–CH–, polyaspartate backbone), 6.1–6.4 (–CCH=CHCHO–, furan ring), 7.4–7.5 (–CCH=CHCHO–, furan ring)). The results showed that all benzyl groups were substituted by furan groups without any degradation of the polymer backbone. Polymer was further labelled with Cy5-NHS (Lumiprobe, Hallandale Beach, Florida, USA) in 1 ml DMF and unreacted dye was removed in open chromatography column (∅1.5 cm × H60 cm) with Sephadex LH-20 (GE Healthcare; Little Chalfont, UK) using DMF as the elution solution.
SN38 prodrug was covalently attached to a carrier polymer through a Diels-Alder reaction between polymer furans and drug maleimides. Briefly, N3-PEG-Poly(Aspartate-Furan) (10 mg, 0.5 μmol) and SN38 prodrug (2 mg, 20 μmol) were dissolved in 1 ml DMF. The mixture of polymer and prodrug was reacted at 50 °C for 2 days. The product was purified by passing through Sephadex LH-20 column. The purity of polymer-prodrug conjugate was confirmed by dialysis against DMF (MWCO: 3000 Da, Spectrum Laboratories, Inc., Rancho Dominguez, California, USA) and measurement of UV absorbance at 370 nm outside the dialysis tube. SN38 prodrug loading was calculated based on the concentration of polymer obtained by freeze drying and the concentration of SN38 determined by UV absorbance at 370 nm. Results showed that one polymer carried 10 molecules of SN38 prodrug. SN38/m were formed by self-assembly of polymer-prodrug conjugate in aqueous solution. Briefly, the polymer-prodrug conjugate was dissolved in DMF at 10 mg/ml and added to chilled pure water under stirring. The micelle solution was further purified by dialysis against pure water (MWCO: 3,000 Da) to remove organic solvent and passed through a 0.22 μm syringe filter (Millipore, Billerica, Massachusetts, USA) to remove particulates. Micelle size and polydispersity index was measured by DLS. For determining the molecular weight of SN38/m, the micelles were diluted sequentially with PBS, and the static light scattering (SLS) intensity was measured by using Zetasizer. For calculating the molecular weight, the dn/dc value of SN38/m was assumed to be the same as that of PIC micelles, that is, 0.162 [Citation15]. Moreover, bovine serum albumin (Sigma, St. Louis, Missouri, USA) was used as the reference sample to check the measurement quality. The association number of bare SN38/m was calculated by dividing the molecular weights of micelle with that of the SN38-conjugated polymer. In addition, for measuring the critical micelle concentration (CMC) of SN38/m, the fluorescence intensity of the SN38-conjugated polymers was measured at SN38 concentrations between 0.001 and 0.1 μM (FP6500, Jasco, Tokyo, Japan; excitation wavelength: 350 nm, emission wavelength: 538 nm). The CMC was defined as the concentration where the fluorescence signal drops and loses linearity against SN38 concentration, indicating the quenching of the SN38 fluorescence.
2.8. Installation of Fab on SN38-loaded micelles
SN38/m was adjusted to a concentration of 3 mg/ml based on polymer and 1C1 Fab PMP23D or mock Fab PMP23D was added at a DBCO/azide ratio of 1 and 3 to prepare micelles with high Fab density and micelles with low Fab density, respectively. The reaction was kept at 37 °C for 4 h. The product was purified by Sephadex G-150 (GE Healthcare; Little Chalfont, UK) and Vivaspin ultrafiltration (MWCO: 100 kDa, Sartorius, Göttingen, Germany). The micelles were purified until no protein was detected in the filtrates determined by micro BCA assay (Thermo Fisher Scientific, Waltham, Massachusetts, USA). Fab content in micelle samples was determined by comparing the concentration of Fab obtained by micro BCA assay and the concentration of polymer. Results indicated that Fab installation ratio was 60% of the PEG chains modified with Fab for the high Fab density micelles and 30% of the PEG chains modified with Fab for the low Fab density micelles. Moreover, SN38/m micelles were modified with 1C1 Fab PMP4D in a similar manner to study the effect of the PEG spacer on the activity of the micelles. These 1C1 Fab PMP4D-installed SN38/m showed 30% of the PEG chains modified with Fab for the low Fab density micelles.
2.9. Micelle binding and cellular uptake of Fab installed micelles
PC3 cells were seeded into eight-well chambered slide systems (Thermo Fisher Scientific, Waltham, Massachusetts, USA) at 10,000 cells per well. Culture medium was changed on the second day and Fab-installed micelles were added to each well. Incubation time for micelle binding was 10 min and cellular uptake was 6 h. After incubation, cells were fixed with 4% paraformaldehyde and counterstained with Hoechst (Dojindo, Kumamoto, Japan). Four images from a random field were taken for each group by confocal laser scanning microscopy (CLSM) using a LSM780 microscope (Carl Zeiss, Oberkochen, Germany). The Cy5 fluorescence intensity was obtained by using ImageJ software, and each cell was regarded as an ROI based on the Hoechst staining. Cell binding and cellular uptake of Fab installed micelles were evaluated after subtracting blank intensity and normalized with bare micelle intensity. The Tukey-Kramer test was applied simultaneously to all groups to find significant differences.
2.10. In vitro cytotoxicity
PC3 cells were seeded into 96-well plates at 5000 cells/well. Twenty-four hours later, irinotecan, which is the water-soluble prodrug of SN38 standardly used in the clinic, or SN38/m were added at different drug concentrations. After 6 h of drug treatment, the cells were washed twice with PBS, and further incubated for another 24-, 48- or 72-h in whole culture medium. The cell viability was determined after the post-incubation step by adding 10 μl of CCK8 (Dojindo, Kumamoto, Japan) into each well, culturing for 2 h at 37 °C, and measuring the absorbance at 450 nm by using Infinite M1000 PRO microplate reader (Tecan Trading AG, Switzerland).
3. Results and discussion
3.1. Conjugation of cross-linkers to Fab
The first step in the process of conjugating Fabs to nanoparticles was to introduce appropriate reactive groups into Fabs that allow conjugation to the biorthogonal chemistry contained on nanoparticles. This was achieved by functionalizing cysteine-engineered Fab targeting EphA2 with a maleimide-PEG-DBCO cross-linker. Reaction of cross-linker maleimide with Fab thiol introduced a free DBCO functional group for further reaction with nanoparticle surface azides. Cross-linkers comprising different PEG lengths (4, 7, 11 and 23 ethylene glycol units, named PMP4D, PMP7D, PMP11D and PMP23D, respectively) were conjugated to Fabs to study the effect of linker length on Fab-nanoparticle conjugation efficiency. Cross-linker conjugation to Fabs was confirmed by mass spectrometry (Figure ), indicated by an increase in Fab molecular weight in the following manner: 737 Da for PMP4D, 869 Da for PMP7D, 1045 Da for PMP11D and 1574 Da for PMP23D. Observed mass increases were in precise agreement with the molecular weight of cross-linkers. Single peaks in the intact mass spectra indicate that the cross-linkers functionalized all the Fabs in each sample. Furthermore, site-specific conjugation of the cross-linker onto Fabs was evaluated by mass spectrometry under reducing conditions to separate Fab and Fab-cross-linker conjugates into heavy chain and light chain species. Site-specific linker attachment to the engineered cysteine at position A114 in the Fab heavy chain was confirmed by the observed increase in the molecular weight of the Fab heavy chain while the peak corresponding to the light chain was not changed.
3.2. Installation of Fabs onto model PIC micelles
Model micelle nanoparticles were assembled by PIC formation between PEG-P(Asp) with 40 aspartic acid units and PEG-PLL with 41 lysine units (Supplementary Figure S1), mixed at 1:1 PEG-polyanion:PEG-polycation molar ratio according to our previous report [Citation15]. PIC micelle cores were cross-linked by the addition of EDC to form amides between carboxyls and amines in PEG-P(Asp) and PEG-PLL, respectively. Samples were dialyzed after the cross-linking procedure and then passed through a 0.22 μm syringe filter to obtain micelles at approximately 26 nm with a moderate PDI. Fab-cross-linkers were installed onto the surface of micelles through copper-free click chemistry of the DBCO group on the cross-linker and the azide moiety on the PEG α-terminus. Feed ratios of Fab-linker:azide groups were varied (Fab/azide molar ratio at 0.1, 0.5, 1, 2, 4 and 8) to investigate the effect of reaction stoichiometry on installation efficiency. After each reaction, the excess of Fab-cross-linker in the mixture was removed by ultrafiltration and the absorbance profile of purified micelles was measured. The number of Fabs on each micelle was calculated using the UV absorbance of Fab at 280 nm and micelles at 555 nm [Citation15]. Results showed that the number of Fabs installed on each micelle increased with reaction feed for all Fab-cross-linkers (Figure ), although increases were lower for the Fab modified with PMP4D cross-linker. At the Fab/azide feed ratio of 8, Fabs modified with PMP11D and PMP23D reached the theoretical maximum installation of 10 Fabs per micelle based on the average polymer association number of micelles, i.e. 10 polymers.
Figure 2. Fab content in nanoparticle-Fab conjugates prepared using PMP4D, PMP7D, PMP11D and PMP23D bifunctional PEG linkers at Fab-linker/azide feed molar ratios of 0.1, 0.5, 1, 2, 4 and 8 during the conjugation reaction. The theoretical maximum is set as 10 (dashed line) based on the polymer association number of each nanoparticle.

To further quantify Fab content in nanoparticles following conjugation, unreacted azide moieties were quenched with an excess amount of Alexa 647-DBCO dye. For this experiment, the Fab/azide feed ratio was fixed at 2 and conjugation efficiency was evaluated as a function of PEG linker length in Fab-linker constructs. After the designated reaction time, samples were purified by ultrafiltration and further reacted with Alexa Fluor 647-DBCO and again subjected to purification by ultracentrifugation. The numbers of dyes and Fabs per molecule were calculated based on the UV-vis absorbance values of micelles and micelle-Fab conjugates (Figure (A)). Before micelle modification, the UV-vis absorbance profile of micelles showed one major peak at 555 nm corresponding to labeled polymer and one minor peak at 280 nm corresponding to the polyamino acid core (Figure (B)). After reaction with Fab-cross-linkers, the 280 nm absorbance significantly increased, indicating successful installation of Fab (Figure (C)). Moreover, the unreacted azide group on the surface of micelles was further modified with the DBCO-bearing dye, as indicated by the appearance of the peak at 647 nm associated with the Alexa dye (Figure (D)). An additional control experiment was carried out where micelle azide groups were quenched with a non-dye DBCO moiety and then subsequently reacted with Alexa 647-DBCO, which showed no conjugation of Alexa 647-DBCO, thus confirming that Alexa 647-DBCO did not react non-specifically or physically absorb to micelle structures (data not shown).
Figure 3. (A) Schematic overview of experiment to determine Fab- and unreacted azide-content by optical absorption. Fab content was determined by A280 measurement, whereas azide content was determined by A647 measurement after backfilling with Alexa Fluor 674-DBCO (A647). (B-D) UV-vis absorbance spectra of (B) unmodified micelles, (C) Fab-linker reacted micelles, and (D) Fab-linker and Alexa Fluor 647-DBCO reacted micelles.

Dye and Fab absorbance values for each micelle-Fab conjugate prepared at Fab-linker:azide ratio of 2 were used to calculate the number of Fabs and Alexa 647 dyes per nanoparticle (Figure ). For unmodified micelles, i.e. non-Fab-reacted, the number of installed dyes per micelle was 10, which is consistent with the theoretical maximum number of reactive azides based on polymer association number. For micelles modified with Fab-PMP4D, the number of installed Fabs was about two to three and the number of dyes was approximately eight. Increasing the length of the linker from four to seven ethylene glycol units allowed an increased the number of Fabs per micelle to approximately three to four, whereas the dyes on the surface of the micelles were reduced to around six. The calculated sum of Fabs and dyes on each micelle for all the Fab-cross-linkers were in good agreement with the known polymer association number of micelles, i.e. 10. Considering both calculated Fab content and calculated Alexa647-DBCO content in reacted micelle samples, increased Fab conjugation efficiency correlated with decreased Alexa647-DBCO conjugation efficiency, thus confirming different Fab-linker reactivities by direct (A280) and indirect (A647) measurements.
Figure 4. Fab/NP and Dyes/NP conjugation efficiency using different bifunctional PEG linker (PMP4D, PMP7D, PMP11D and PMP23D) at Fab/azide feed molar ratio 2.
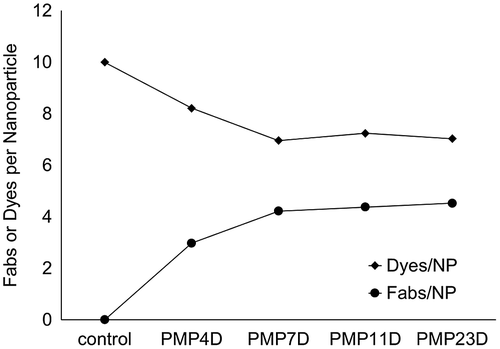
Altogether, our results show that Fab-linkers prepared with PEG lengths of 7–23 units conjugate more efficiently to nanoparticles than Fab-linkers prepared with PEG4 and also that unreacted azide groups after Fab-linker conjugation can be further modified in a second conjugation reaction. Increased reactivity of Fab-linkers with >7 PEG units could be attributed to several factors such as increased distance of DBCO reactive groups from the Fab surface, increased flexibility of PEG spacers with higher molecular weights, or improved solubility of the DBCO group resulting from increased linker hydrophilicity with longer PEGs. One, or the combination, of these factors resulted in increased reaction efficiency between Fab-linker-DBCO and micelle-azides in the current work. However, the DBCO-azide reaction is still somewhat inefficient. A DBCO:azide molar feed ratio of 2 resulted in ~40% modification of azides, corresponding to ~20% reaction of Fab DBCO groups. Thus, higher molar ratios of DBCO:azide (eight or more molar equivalents) are needed to achieve complete conversion of nanoparticle azide groups.
Compared with our previous method for conjugating Fabs to micelles using DBCO-bearing cross-linkers with a short PEG4 spacer (which was limited to ~30% conversion of micelle azides), these new Fab-cross-linker conjugates can be applied to modify the surface of nanoparticles in a more controlled manner. Such control of ligand density is critical for evaluating the effects of ligand density on cellular uptake and targeting efficiency, which could be highly variable depending on ligand affinity, receptor availability, and the rate of receptor internalization [Citation20–23]. Controlled installation of Fabs onto nanoparticle systems has been challenging thus far and the relationship between Fab density and cellular uptake of nanoparticles (particularly at high levels of modification) is for the most part poorly defined. The Fab-nanoparticle conjugation method described here provides an effective platform for pursuing such studies. Furthermore, results confirmed that Fab modification of the micelle surface occurred without affecting reactivity of remaining azide groups, thus allowing for further chemical modification after completion of the Fab conjugation step. This property of the current nanoparticle-Fab conjugation process could be useful for attachment of more than one type of ligand onto micelles for dual targeting and conjugation of ligands and fluorophores onto micelles for function and biodistribution studies.
The effect of Fab-linker conjugation on micelle size was evaluated by dynamic light scattering (DLS) measurement (Table ). The hydrodynamic diameter of 1C1 Fab is approximately 5 nm [Citation24] and thus micelle size should increase after Fab installation, which was confirmed by DLS measurement (Figure ). When the Fab/azide ratio was fixed at 2, the nanoparticle diameter increased 4 to 5 nm for PMP4D, PMP7D, PMP11D and PMP23D. Increasing the Fab/azide feed ratio to 8 resulted in a diameter increment of more than 8 nm, which is larger than a single Fab, suggesting that the installed Fabs are surrounding the micelles. Measured size increases of micelle nanoparticles after Fab conjugation were in good agreement with the size and dimension of Fabs, with size increases of ~4–10 nm corresponding to one and two Fab lengths. These results suggest that Fabs were installed onto nanoparticles in a controlled manner.
Table 1. Z-average diameter and PDI of micelles.
3.3. Preparation of Fab-installed SN38-loaded micelles
The biological impact of controlled Fab conjugation to nanoparticles was evaluated using functional drug-loaded micelle nanoparticles. Micelles were prepared with the active metabolite of irinotecan, SN-38, an anti-cancer agent with potent anti-tumor effects and currently used in the development of antibody-drug conjugates [Citation25–27]. SN38 was functionalized with a protease cleavable linker (phenylalanine-lysine-p-aminocarbamate) for cathepsin B activation and also a maleimide group for covalent conjugation to furans on the carrier block polymer. The carrier polymer of SN38 prodrug was synthesized by aminolysis of N3-PEG-PBLA (MWPEG = 12,000; PBLA units: 40) with furfurylamine to introduce furan moieties onto amino acid side chains. The synthetized block copolymers were found to be monodisperse with narrow polydispersity (Mw/Mn close to 1; Supplementary Figures S2 and S3). Moreover, the 1H-NMR results indicated quantitative conversion of all 40 benzyl groups on the PBLA side chain to furan moieties after aminolysis. Next, SN38 prodrug was conjugated onto furan-containing polymer through Diels-Alder reaction of the maleimide and furan groups to yield the desired polymer-prodrug conjugate (Supplementary Figure S4). UV absorbance measurement confirmed that one polymer conjugated 10 molecules of SN38 prodrug. Diels-Alder conjugation was used in this study rather than the conventional thiol-maleimide Michael reaction due to the stability of the conjugation product, as the thiol-maleimide system is susceptible to retro-Michael addition by thiol-bearing biomolecules, such as glutathione and albumin [Citation28], leading to an unspecific release of the cargo. Thus, taking advantage of Diels-Alder reaction between maleimide and furan, the prodrug activation is exactly defined at the enzyme-cleavable linker position, which is of much importance for the selectivity of prodrug activation [Citation29]. By using these polymers, SN38/m with 38 nm diameter and a narrow PDI (0.188) were self-assembled by dialysis in water (Supplementary Figure S5). The association number of SN38/m, that is, the number of polymers forming one micelle, was determined to be 80 by SLS (Supplementary Figure S6). Moreover, the CMC of SN38/m was analyzed by using the fluorescence signal of the SN38 conjugated to the polymers as an indicator of the polymer association, since the assembly of the SN38-conjugated polymers into micelles quenches the fluorescence of SN38. Thus, at polymer concentrations below 2 nM, the fluorescence intensity of SN38-conjugated polymers increased linearly with polymer concentration, suggesting that the polymers are not associated. However, at polymer concentrations higher than 2 nM, the linearity between the fluorescence signal from the SN38-conjugated polymers and the polymer concentration was lost, with the fluorescence signal being reduced, which suggests the association of the polymers and fluorescence quenching. Thus, we concluded that the CMC of SN38/m was around 2 nM based on polymer concentration (Supplementary Figure S7), which is consistent with our previous reports for micelles loading hydrophobic drugs [Citation19].
To investigate the effect of Fab density on the bioactivity of micelles, 1C1- and mock-Fabs functionalized with PMP4D or PMP23D were reacted with SN38/m at Fab/azide ratios of 1 and 3 for high and low installation levels, respectively. Unreacted Fab was removed by size exclusion chromatography followed by ultrafiltration, as confirmed by BCA protein analysis of the ultrafiltrate. Fab installation density was obtained by comparing the molar concentration of Fab measured by BCA protein assay with the concentration of azide groups calculated from the known polymer concentration. It was found that at low feed ratio of Fab/azide, the Fab density on micelles was 30% for both PMP4D and PMP23D, that is, 24 Fabs per micelle based on the association number of SN38/m, which was the maximum Fab/azide ratio in our previous report [Citation15]. Increasing the reaction feed ratio of Fab/azide to 3 for the PMP4D-functionalized Fab-linkers only increased the conjugation efficiency to 60% Fab, i.e. 48 Fabs per micelle based on the association number of SN38/m. These results are comparable to those obtained for PIC micelles (Figure ). Moreover, because the Fab density change was significantly increased by using the PMP23D-functionalized Fabs, we applied these micelles for studying the effect of the Fab density on the bioactivity of micelles.
The diameter of the SN38/m was slightly increased after modification with the Fabs (Table ). Compared with bare SN38/m, the diameter of micelles installed with 30% Fab had an increment of around 4 nm, while the diameter of the micelles installed with 60% Fab increased around 10 nm, which is consistent with findings obtained with model PIC micelle nanoparticles in the previous section (Table ). In addition, all the micelles presented moderate PDI (Tables and ).
Table 2. Characterization of SN38-loaded micelles (SN38/m).
3.4. In vitro activity of Fab-installed SN38-loaded micelles using PMP4D and PMP23D
The effect of conjugating Fab-linkers through either PMP4D- or PMP23D-linkers on the bioactivity of Fab-installed SN38/m was studied in human prostate PC3 cancer cells. Installing ligands on the surface of nanoparticles may enhance cell uptake compared with bare nanoparticles, which is usually related to ligand-mediated endocytosis mechanisms [Citation30–32]. First, the effect of cross-linker length on cellular binding and internalization was investigated with SN38/m prepared with PMP4D- or PMP23D-Fab at the same Fab density, i.e. 30%. In the current study, cell binding and internalization processes were evaluated using CLSM after treating the cells with Cy5-labeled micelles for 10 min and 6 h for cellular binding and uptake studies, respectively. After 10 min incubation, fluorescence signals for both 30% 1C1-PEG4- and 30% 1C1-PEG23-SN38/m were detected on the surface of receptor (+) cancer cells, with intensities significantly higher than that of bare SN38/m, indicating rapid binding of the Fab-installed micelles (Figure (A)). Moreover, both 30% 1C1-PEG4- and 30% 1C1-PEG23-SN38/m showed comparable fluorescence intensities after 10 min, indicating a similar ability to bind receptors on PC3 cells (Figure (B)). In addition, cellular uptake of the micelles after 6 h incubation showed that internalization of both 1C1 Fab-installed SN38/m was higher than that of bare SN38/m (Figure (C) and (D)). As expected, no significant binding difference was observed between the 1C1 Fab-installed micelles (Figure (D)). After blocking EphA2 receptors by addition of free 1C1 Fab to cells before micelle treatment, the cellular uptake of both 1C1 Fab-installed micelles decreased, confirming that EphA2-mediated endocytosis increased uptake. These findings demonstrate that PMP4D- and PMP23D-linkers themselves did not affect cellular binding and uptake of 1C1 Fab-installed micelles, and that the enhanced cellular interaction of both Fab-installed micelle constructs was due to EphA2 recognition.
Figure 6. (A) Fluorescence microscopy images of PC3 cells (blue) after 10 min treatment of Cy5-labeled SN38-loaded micelles (red) installed with 1C1 Fab-linkers prepared with PEG4 or PEG23 cross-linkers. (B) Average Cy5 fluorescence intensity of each cell normalized to bare micelles. (C) Fluorescence microscopy images of PC3 cells (blue) after 6 h incubation with Cy5-labeled SN38-loaded micelles (red) installed with 1C1 Fabs having cross-linkers containing 4 or 23 units of PEG. (D) Average Cy5 fluorescence intensity of each cell normalized to bare micelles. Cell nuclei were stained with Hoechst (blue). Scale bar: 50 μm. In (B) and (D), data are shown as average ± standard deviation (SD) from four images taken in random fields and compared with Tukey-Kramer test. ** p < 0.01; n.s.: not significant.
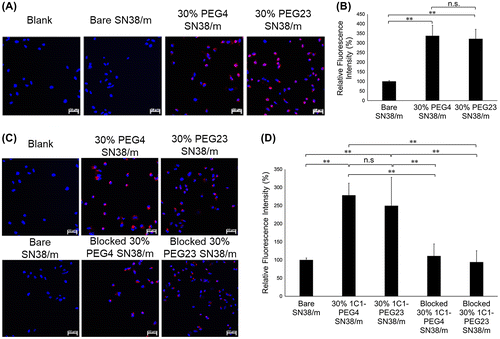
After internalization, micelles are trafficked to lysosomes, where cathepsin B can cleave the phenylalanine-lysine dipeptide linker in polymer-conjugated SN38 prodrug [Citation26]. The potency of SN38/m is likely related to internalization efficiency and subcellular trafficking. Thus, the cytotoxicities of 30% 1C1-PEG4- and 30% 1C1-PEG23-SN38/m were evaluated compared with free irinotecan and bare SN-38/m. PC3 cells were exposed to the drug for 6 h, washed with PBS, and post-incubated for another 24, 48 or 72 h. Results demonstrated that 1C1 Fab-installed micelles were consistently more active than irinotecan and bare SN-38/m (Figure ), which corroborates with increased cellular uptake after 6 h (Figure ). Moreover, the cytotoxicity of 30% 1C1-PEG4-SN38/m was comparable with that of 30% 1C1-PEG23-SN38/m at any time point (Figure ). In vitro 50% inhibitory concentration (IC50) values for all the drugs at the different incubation times are summarized in Table . These cytotoxicity results, together with the cellular binding and uptake observations, indicate that installation of Fabs through PMP4D- and PMP23D-linkers did not affect the in vitro activity of 1C1 Fab-installed micelles.
Figure 7. In vitro cytotoxicity of irinotecan, bare SN-38/m,30% 1C1-PEG4- and 30% 1C1-PEG23-SN38/m against PC3 cells after (A) 24, (B) 48 and (C) 72 h incubation. Data are shown as average ± SD from four experiments.
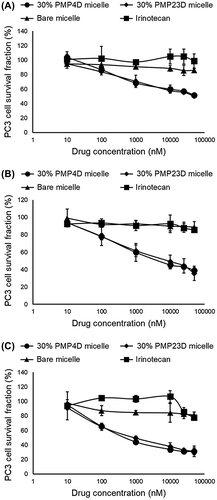
Table 3. Fifty-percent in vitro inhibitory concentration (IC50) values of irinotecan and SN38-loaded micelles (SN38/m) obtained after 6 h drug exposure and 24, 48 and 72 h post-incubation.
3.5. In vitro activity of Fab-installed SN38 micelles with 30% and 60% Fab density
The Fab density on the surface of nanoparticles can affect the bioactivity of Fab-installed nanoparticles [Citation15,33–36]. Previously, we reported that higher Fab densities promote receptor binding and cellular internalization [Citation15], although the maximum studied Fab-density was 30% and the effect of higher Fab densities remains unknown. Here, the cell binding and uptake of 30% 1C1-PEG23- and 60% 1C1-PEG23-SN38/m were investigated in PC3 cells by using CLSM and Cy5-labeled micelles. After incubating cells with SN38/m for 10 min, the binding of 30% 1C1-PEG23-SN38/m was comparable to that of 60% 1C1-PEG23-SN38/m (Figure ), and the binding of both micelles was much higher than that of bare SN38/m. When EphA2 on PC3 cells was blocked by the addition of free 1C1 Fab, the binding of both 30% Fab- and 60% Fab-installed micelles decreased (Figure ).
Figure 8. (A) Fluorescence microscopy images of PC3 cells (blue) after 10 min treatment of Cy5-labeled SN38-loaded micelles (red) installed with 30% or 60% 1C1 Fabs-linker prepared with PEG23 cross-linker. Cells nuclei were stained with Hoechst (blue). Scale bar: 50 μm. (B) Average fluorescence intensity of each cell normalized to bare micelles. Data are shown as average ± SD from four different images, and compared with Tukey-Kramer test. * p < 0.05; ** p < 0.01; n.s.: not significant.
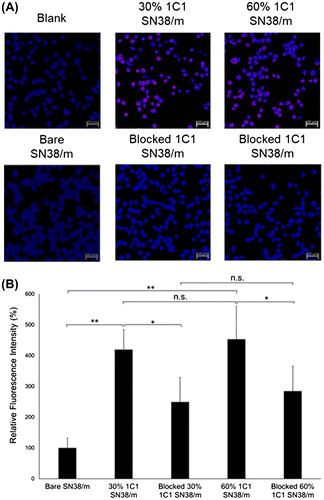
Cellular uptake of SN38/m was further evaluated after 6 h incubation. Bare micelles and micelles installed with the mock Fab (Figure ) showed low cell uptake, whereas SN38/m with 30% 1C1 Fab showed higher cellular uptake, again demonstrating effective targeting of EphA2 on cells. However, increasing the level of 1C1 Fab on micelles to 60% in 1C1-PEG23-SN38/m reduced cellular uptake compared with the 30% 1C1-PEG23-SN38/m (Figure ). Incubation of PC3 cells with free 1C1 Fab for 1 h to block EphA2 receptors, reduced cellular uptake of both 30% and 60% 1C1 Fab-installed micelles (Figure ), again confirming that uptake of both micelles was mediated by interaction with the receptor as demonstrated in the previous section. Since free 1C1 Fabs effectively blocked the cellular binding and uptake of 60% 1C1-PEG23-SN38/m (Figures and ), unspecific interaction of these micelles with the PC3 cells could be ruled out as the possible mechanism for the reduced cell internalization. Alternatively, the drop in the cellular uptake of 60% 1C1-PEG23-SN38/m could be related to strong multivalent binding to the EphA2 on the surface of PC3 cells and exhaustion of the available receptors for further micelle internalization.
Figure 9. (A) Fluorescence microscopy images of PC3 cells (blue) after 6 h treatment of Cy5-labeled SN38-loaded micelles (red) installed with 30% or 60% 1C1 Fabs having cross-linkers containing 23 units of PEG. Cell nuclei were stained with Hoechst (blue). Scale bar: 50 μm. (B) Average fluorescence intensity of each cell normalized to bare micelles. Data are shown as average ± SD from four different images, and compared with Tukey-Kramer test. * p < 0.05; ** p < 0.01; n.s.: not significant.
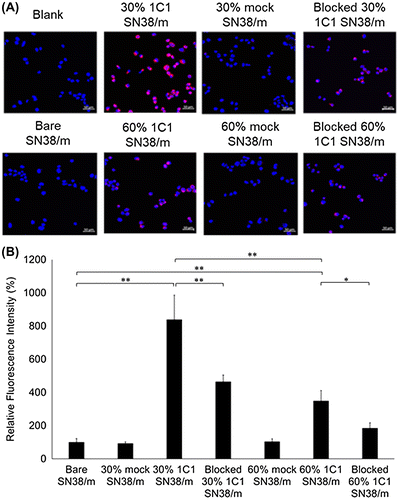
In vitro cytotoxicity of 30% 1C1-PEG23- and 60% 1C1-PEG23-SN38/m, as well as 30% mock-PEG23- and 60% mock-PEG23-SN38/m, were studied by incubating PC3 cells with the drug for 6 h, washing them with PBS, and further incubating for 24, 48 and 72 h in fresh media without SN38/m. SN38/m installed with 1C1 Fab showed high cytotoxicity, while micelles installed with the mock Fab were inactive. Cytotoxicity of 1C1 Fab-installed SN38/m increased with post-incubation time, suggesting that micelle dissociation and enzymatic release of drug is not instantaneous after internalization. One key finding in the cytotoxicity analysis was that 30% 1C1-PEG23-SN38/m was more cytotoxic than 60% 1C1-PEG23-SN38/m, evidenced by IC50 values after 48 and 72 h post-incubation (Figure and Table ). These IC50 values are well above the CMC of SN38/m, suggesting that the micelles exert their cytotoxic effects in micelle rather than as free polymer-drug conjugates. These results also corroborate with the cellular uptake profiles of the micelles, with 30% 1C1-PEG23-SN38/m being more effectively internalized by PC3 cells than 60% 1C1-PEG23-SN38/m (Figure ). High Fab density on nanoparticles could have a particularly strong impact when targeting EphA2 on PC3 cells, as this receptor is relatively low density (~16,000 copies/cell) and the receptor is degraded after internalization [Citation37]. Thus, while the current study shows that lower Fab content results in improved bioactivity, this trend might not hold true for all cell-surface receptors. These findings demonstrate that the introduction of ligands on the surface of nanoparticles must be precisely controlled for achieving optimal activity. In this way, the proposed cross-linkers based conjugation strategy allows precise and tunable installation of Fabs on the surface of nano-scaled platforms, which is a substantial advantage for developing nanosystems with optimal performance.
Figure 10. In vitro cytotoxicity of 30% 1C1-PEG23-, 60% 1C1-PEG23-, 30% mock-PEG23- and 60% mock-PEG23-SN38/m against PC3 cells after (A) 24, (B) 48 and (C) 72 h incubation. Data are shown as average ± SD from four experiments.
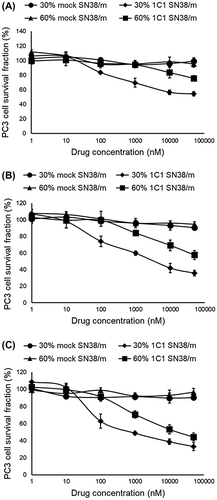
Table 4. IC50 values obtained after 6 h exposure to SN38-loaded micelles (SN38/m) installed with 1C1- or mock Fab-linkers prepared with PEG23 cross-linker, at post-incubation times of 24, 48 and 72 h.
4. Conclusions
In the current study, a method to control Fab installation onto nanoparticles was developed by precise modification of cysteine-engineered Fabs with cross-linkers containing PEG spacers of different lengths. With this strategy, Fab conjugation efficiency to polymeric micelles achieved the theoretical maximum value, with 100% of the conjugation sites on the shell of micelles being modified with Fabs. In general, installation of Fabs on the micelles increased their cellular uptake, and enhanced the cytotoxicity of micelles loading SN38. However, the conjugation strategy with improved conjugation efficiency described here enabled us to explore the effect of preparing micelles with high Fab densities, which was found to be detrimental for their cell internalization and subsequent in vitro activity. This effect was Fab density-specific but not linker-specific as evidenced by comparison of SN38/m prepared with 30% Fab-linker comprising PEG4 or PEG23 linkers. The conjugation strategy described here holds the potential for effectively installing other macromolecular entities onto nanoparticles such as whole antibodies or other large proteins, and could be further extended to various biotechnological applications besides drug delivery, including diagnosis, harvesting of cells and antigens, immunotherapies and vaccination.
Supplemental data
Supplemental data for this article can be accessed https://doi.org/10.1080/14686996.2017.1370361.
Disclosure statement
No potential conflict of interest was reported by the authors.
Funding
This work was partially supported by Grants-in-Aid for Scientific Research B (JP16H03179; HC) and Young Scientists B (JP25750172; HC) from the Japan Society for the Promotion of Science (JSPS), the Project of Cancer Research And Therapeutic Evolution (P-CREATE) (Project No. 16cm0106202h0001; HC and KK) from the Japan Agency for Medical Research and Development (AMED); ‘the Center of Innovation, Science and Technology based Radical Innovation and Entrepreneurship Program (COI STREAM)’ from the Ministry of Education, Culture, Sports, Science and Technology (MEXT) of Japan (KK); and the Japan Science and Technology. SC was financially supported by China Scholarship Council and Kanehara Zaidan. Financial support was also provided by MedImmune, a division of AstraZeneca.
SI.docx
Download MS Word (542 KB)References
- Dand NM, Patel PB, Ayre AP, et al. Polymeric micelles as a drug carrier for tumor targeting. Chron Young Sci. 2013;4:94–101.
- Ling D, Hackett MJ, Hyeon T. Surface ligands in synthesis, modification, assembly and biomedical applications of nanoparticles. Nano Today. 2014;9:457–477.10.1016/j.nantod.2014.06.005
- Katz E, Willner I. Integrated nanoparticle-biomolecule hybrid system: synthesis, properties and application. Angew Chem Int Edit. 2004;43:6042–6108.10.1002/(ISSN)1521-3773
- Allen TM. Ligand-targeted therapeutics in anticancer therapy. Nat Rev Cancer. 2002;2:750–763.10.1038/nrc903
- Wei X, Chen X, Ying M, et al. Brain tumor-targeted drug delivery strategies. Acta Pharm Sin B. 2014;4:193–201.10.1016/j.apsb.2014.03.001
- Mi P, Yanigie H, Dewi N, et al. Block copolymer-boron cluster conjugate for effective boron neutron capture therapy of solid tumors. J Control Release. 2017;254:1–9.10.1016/j.jconrel.2017.03.036
- Peng Y, Li X, Yuan R, et al. Steric hindrance inhibition of strand displacement for homogeneous and signal-on fluorescence detection of human serum antibodies. Chem Commun. 2016;52:12586–12589.10.1039/C6CC06893F
- Kühn T, Ihalainen TO, Hyväluoma J, et al. Protein diffusion in mammalian cell cytoplasm. PLoS One. 2011;6;8:e22962.
- Kobayashi T, Yokota M, Mitani T, et al. Effects of solvent displacement on sensitivity and specificity of monoclonal antibodies for ABO blood grouping of forensic specimens with an absorption-elution test. Leg Med. 1991;1:68–75.
- Schrama D, Reisfeld RA, Becker JC. Antibody targeted drugs as cancer therapeutics. Nat Rev Drug Discov. 2006;5:147–159.10.1038/nrd1957
- Trilling AK, Beekwilder J, Zuilhof H. Antibody orientation on biosensor surfaces: a minireview. Analyst. 2013;138:1619–1627.10.1039/c2an36787d
- Belbekhouche S, Guerrouache M, Carbonnier B. Thiol-maleimide Michael addition click reaction: a new route to surface modification of porous polymeric monolith. Macromol Chem Phys. 2016;217:997–1006.10.1002/macp.v217.8
- Yamagishi K, Sawaki K, Murata A, et al. A Cu-free clickable fluorescent probe for intracellular targeting of small biomolecules. Chem Commun. 2015;51:7879–7882.10.1039/C4CC09947H
- Kumar S, Aaron J, Sokolov K. Directional conjugation of antibodies to nanoparticles for synthesis of multiplexed optical contrast agents with both delivery and targeting moieties. Nat Protoc. 2008;3:314–320.10.1038/nprot.2008.1
- Florinas S, Liu M, Fleming R, et al. A nanoparticle platform to evaluate bioconjugation and receptor-mediated cell uptake using cross-linked polyion complex micelles bearing antibody fragments. Biomacromolecules. 2016;17:1818–1833.10.1021/acs.biomac.6b00239
- Ahn J, Miura Y, Yamada N, et al. Antibody fragment-conjugated polymeric micelles incorporating platinum drugs for targeted therapy of pancreatic cancer. Biomaterials. 2015;39:23–30.10.1016/j.biomaterials.2014.10.069
- Nakanishi M, Park JS, Jang WD, et al. Study of the quantitative aminolysis reaction of poly(β-benzyl l-aspartate) (PBLA) as a platform polymer for functionality materials. React Funct Polym. 2007;67:1361–1372.10.1016/j.reactfunctpolym.2007.08.009
- Takeda KM, Yamasaki Y, Dirisala A, et al. Effect of shear stress on structure and function of polyplex micelles from poly(ethylene glycol)-poly(l-lysine) block copolymers as systemic gene delivery carrier. Biomaterials. 2017;126:31–38.10.1016/j.biomaterials.2017.02.012
- Quader S, Cabral H, Mochida Y, et al. Selective intracellular delivery of proteasome inhibitors through pH-sensitive polymeric micelles directed to efficient antitumor therapy. J Control Release. 2014;188:67–77.10.1016/j.jconrel.2014.05.048
- Moradi E, Vllasaliu D, Garnett M, et al. Ligand density and clustering effects on endocytosis of folate modified nanoparticles. RSC Adv. 2012;2:3025–3033.10.1039/c2ra01168a
- Elias DR, Poloukhtine A, Popik V, et al. Effect of ligand density, receptor density, and nanoparticle size on cell targeting. Nanomedicine. 2013;9:194–201.10.1016/j.nano.2012.05.015
- Miura Y, Takenaka T, Toh K, et al. Cyclic RGD-linked polymeric micelles for targeted delivery of platinum anticancer drugs to glioblastoma through the blood–brain tumor barrier. ACS Nano. 2013;7:8583–8592.10.1021/nn402662d
- Alam MR, Ming X, Fisher M, et al. Multivalent cyclic RGD conjugates for targeted delivery of small interfering RNA. Bioconjugate Chem. 2011;22:1673–1681.10.1021/bc200235q
- Thirumurugan P, Matosiuk D, Jozwiak K. Click chemistry for drug development and diverse chemical–biology applications. Chem Rev. 2013;113:4905–4979.10.1021/cr200409f
- Ramesh M, Ahlawat P, Srinivas NR. Irinotecan and its active metabolite, SN-38: review of bioanalytical methods and recent update from clinical pharmacology perspectives. Biomed Chrom. 2010;24:104–123.10.1002/bmc.v24:1
- Govindan SV, Cardillo TM, Sharkey RM, et al. Milatuzumab-SN-38 conjugates for the treatment of CD74+ cancers. Mol Cancer Ther. 2013;12:968–978.10.1158/1535-7163.MCT-12-1170
- Dubowchik GM, Firestone RA, Padilla L, et al. Cathepsin B-labile dipeptide linkers for lysosomal release of doxorubicin from internalizing immunoconjugates: model studies of enzymatic drug release and antigen-specific in vitro anticancer activity. Bioconjugate Chem. 2002;13:855–869.10.1021/bc025536j
- Nair DP, Podgórski M, Chatani S, et al. The thiol-michael addition click reaction: a powerful and widely used tool in materials chemistry. Chem Mater. 2014;26:724–744.10.1021/cm402180t
- Lu J, Jiang F, Lu A, et al. Linkers having a crucial role in antibody–drug conjugates. Int J Mol Sci. 2016;17:561.10.3390/ijms17040561
- Ritchie M, Tchistiakova L, Scott N. Implications of receptor-mediated endocytosis and intracellular trafficking dynamics in the development of antibody drug conjugates. Mabs. 2013;5:13–21.10.4161/mabs.22854
- Austin CD, De Mazière AM, Pisacane PI, et al. Endocytosis and sorting of ErbB2 and the site of action of cancer therapeutics trastuzumab and geldanamycin. Mol Biol Cell. 2004;15:5268–5282.10.1091/mbc.E04-07-0591
- Friedman AD, Claypool SE, Liu R. The smart targeting of nanoparticles. Curr Pharm Des. 2013;19:6315–6329.10.2174/13816128113199990375
- Yin L. Measuring binding kinetics of antibody-conjugated goldnanoparticles with intact cells. Small. 2015;11:3782–3788.10.1002/smll.v11.31
- Fay F, Scott CJ. Antibody-targeted nanoparticles for cancer therapy. Immunotherapy. 2011;3:381–394.10.2217/imt.11.5
- Saha B, Evers TH, Prins MWJ. How antibody surface coverage on nanoparticles determines the activity and kinetics of antigen capturing for biosensing. Anal Chem. 2014;86:8158–8166.10.1021/ac501536z
- Bandyopadhyay A, Fine RL, Demento S, et al. The impact of nanoparticle ligand density on dendritic-cell targeted vaccines. Biomaterials. 2011;32:3094–3105.10.1016/j.biomaterials.2010.12.054
- Jackson D, Gooya J, Mao S, et al. A human antibody-drug conjugate targeting EphA2 inhibits tumor growth in vivo. Cancer Res. 2008;68:9367–9374.10.1158/0008-5472.CAN-08-1933