ABSTRACT
In the attempt to reduce greenhouse gas emissions from steel production, several large industry decarbonization projects have emerged in Europe. The commercialization of low-emission steel technology, however, faces systemic barriers such as a lack of infrastructure and unclear demand for greener steel. As part of its new commitment to climate-neutrality, the European Commission has announced plans to more actively create and reshape markets for green basic materials. The approach is inspired by the recent success story of renewable energy, where market interventionist policy has successfully led to cost reductions and supported the diffusion of wind and photovoltaics. However, the applicability of this type of policy to decarbonize basic materials has so far not been investigated. In this study, we evaluate the effectiveness, feasibility, efficiency and fairness of early commercialization policy support for the decarbonization transition of steel. We compare two approaches: demand side market creation and direct production subsidies through carbon contracts for difference. We find that the subsidy approach can more effectively enable the realization of primary green steel production. A complementary use of market creation policy instruments can reduce the production subsidy volumes needed and aid the global diffusion of new production methods. Although effective, we find that production subsidies will distribute the costs and benefits of the transition unequally. In order to improve effectiveness and fairness of the policy, parallel programmes such as electricity price guarantees and transitional assistance policies for disadvantaged regions are needed.
Key policy insights
Carbon contracts for difference are the most promising policy instrument to commercialize low-emission primary steel but are likely to lead to unequal distribution of transition costs.
Market creation policies can support the global diffusion of low-emission primary steelmaking.
Material efficiency and demand reduction can reduce the need for primary steel production by more than 50%.
Regions without access to large amounts of renewable electricity are especially disadvantaged.
Unclear EU ETS benchmarks currently create a perverse incentive that keeps firms from investing in breakthrough technologies.
1. Introduction
In order to limit global warming to well below 2°C, net anthropogenic greenhouse gas (GHG) emissions must reach zero between 2050 and 2070 and become negative thereafter (IPCC, Citation2018). Steel production is a major emitter of CO2, both globally (7% of energy-related CO2 emissions in 2017) and within the EU (4% in 2017), and the process of primary ironmaking (from which steel is derived) is responsible for over 80% of those emissions (EEA, Citation2018; IEA, Citation2017). The industry has responded to climate policy with efficiency improvements (Eurofer, Citation2013), but the potential for further emission reductions through efficiency is limited, and a more rigorous approach is therefore needed to meet climate targets. Deep decarbonization of steel production requires systemic change of the steel production process and the energy system it is embedded in. The technologies required are not yet commercially available, and are currently under development in a series of mostly European innovation projects. However, since 2016, several incumbent firms have announced the building of demonstration plants, and some have communicated plans to sell ‘green’ steel within the next decade.
As the decarbonization of European steel production enters the early-commercialization phase, ideas for complementary and more interventionist forms of policy support have surfaced. So far, the EU emissions trading system (EU ETS) has not sufficiently supported the transformation of energy-intensive industries. Climate targets and R&D support at the national and EU levels have elicited a series of company roadmaps and pilot plants to reduce steel's climate impact. The EU Innovation Fund builds on this by planning to support the scale-up of novel technologies to demonstration stage. However, despite the large R&D support, significant risks regarding the business case for greenFootnote1 steel production remain. In other words, low-emission steel production is not cost-competitive, neither within Europe nor with non-European producers, and needs public support to come into being (Kushnir et al., Citation2020). Consequently, both energy-intensive industries and the European Commission have begun to call for a policy approach that actively creates and reshapes markets to support strategic value chains such as steel. In its Industrial Strategy the Commission sets a target for a climate-neutral industry by 2050 (EC, Citation2020), while the European Green Deal announces the Commission's goal of commercializing zero-carbon steel production by 2030 (EC, Citation2019). The EU's High-Level Group on Energy-Intensive Industries has also made market creation for climate-neutral products one of its core recommendations (HLG-EII, Citation2019).
The more active role of policy in the transition to create and reshape markets is inspired by its success in renewable energy, where policy instruments such as feed-in tariffs, renewable portfolio standards and contracts for difference have reduced costs and supported the deployment of wind and solar power (Quitzow et al., Citation2014). However, the production of basic materials follows different logics with respect to markets, trade, scale and value chains, which makes the translation of policy from renewables to energy-intensive industry far from trivial. Despite recent calls for exclusive markets for green basic materials by the Commission (EC, Citation2019, Citation2020) and industry (Eurofer, Citation2019b; HLG-EII, Citation2019), this is the first study that evaluates this approach in detail. Previous research papers have focussed on the concept of contracts for difference (Richstein, Citation2017; Sartor & Bataille, Citation2019), or provided overviews across multiple industries (Elkerbout & Egenhofer, Citation2018; Neuhoff et al., Citation2019; Wyns et al., Citation2019), and across all phases of the innovation process (Neuhoff et al., Citation2014). This paper aims to contribute to this body of research by focussing on one specific phase of the innovation process – early commercialization – and on a single sector, acknowledging the complexity of markets for steel. To do so, we first identify and then evaluate policy approaches to reduce the risk for investments into low-emission primary steel production. Thereby we aim to show how policy makers can enable and guide the commercialization of green steel production in the EU. This provides a more granular picture of the implications of different approaches and offers some guidance regarding the design and implementation of effective and just measures.
This article proceeds as follows: First, we outline issues relating to the transformation of the EU steel industry and the types of policy support needed to realize it. In section 3 we describe our analytical framework and suggest a typology of policy instruments for the early-commercialization phase. Section 4 presents the evaluation of policy approaches articulated with respect to our framework. We end with a discussion of the main political choices and trade-offs in section 5 and conclude thereafter.
2. The EU steel transition
The principal source of GHG emissions in the steel sector is ironmaking in blast furnaces. The production of steel from iron ore is typically referred to as the primary route, whereas steel recycling is called the secondary route. Even under the most ambitious circular economy scenarios some primary steel production will be required in the future. illustrates a schematic path to a carbon-neutral steel industry in Europe by pursuing two distinct strategies: material efficiency and development of zero-emission primary production technologies. A broad literature has formed around the benefits of increasing the material efficiency of steel production and use (Allwood & Cullen, Citation2012; Allwood et al., Citation2017; Hertwich et al., Citation2019; Kallis, Citation2017; Material Economics, Citation2018; Milford et al., Citation2013; Söderholm & Tilton, Citation2012). However, these issues have largely not made it onto the political agenda (Cooper-Searle et al., Citation2018). The extent to which the share of secondary production can be increased is limited by several factors, such as the availability of scrap and the maintenance of scrap quality (Pauliuk, Milford, et al., Citation2013; Xylia et al., Citation2018), as well as the ability to produce high quality steels in the secondary route. The availability of scrap depends on the interaction of several parameters, including steel product lifetimes, historical growth rates and recovery rates (Pauliuk, Wang, et al., Citation2013). In theory, many different qualities of steel can be produced from scrap, if scrap of controlled quality is available. In practise, access is limited, and scrap stock quality is deteriorating due to copper accumulation (Daehn et al., Citation2017).
Figure 1. Historic data and outlook for EU steel production. Climate neutrality in 2050 means total emissions (left axis) and emission intensities (right axis) must approach zero. Material efficiency improvements and overall steel demand reduction can lower the amount of needed primary steel production significantly (see primary route – min.). 2050 data from Fleiter et al. (Citation2019), Material Economics (Citation2018); van Ruijven et al. (Citation2016); historic emissions from EEA (Citation2018), steel production data from World Steel Association (Citation2009, Citation2019).
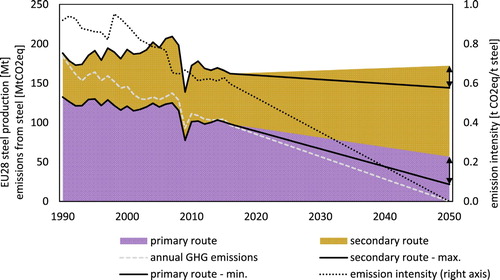
A review of scenario models identifies a maximum possible share of secondary steel production in Europe at 85% of total production by 2050 (Material Economics, Citation2018). Achieving such a high share of recycling without offshoring emissions would require ‘that nearly all available scrap is utilized, high-quality steel production from scrap is feasible, and copper contamination is resolved’ (ibid.:72). In such a stylized scenario, only 22 Mt of primary steel instead of 57 Mt would need to be produced by 2050. Assuming an average blast furnace capacity of 1.5 Mt, the number of operating blast furnaces that need to be converted into clean primary production processes would decline from today's 65 down to 38, or as low as 15 if secondary production is maximized. Since 2016, several steelmakers have announced plans to abandon their blast furnaces and switch to hydrogen-based processes.Footnote2 These commitments currently add up to a capacity of 30 Mt green steel production – more than needed if full material efficiency and demand reduction potentials are exhausted.
2.1. Past and present climate governance of the EU steel industry
GHG emissions from the steel sector are mainly regulated through the EU Emissions Trading System (EU ETS), which sets the pace for emission reductions up to 2050. Trade-exposed sectors such as steel have been receiving free allocation of emission allowances to prevent carbon leakage. Steel firms have in the past downplayed their ability to pass through costs to their customers and successfully lobbied the EU for complete free allocation (Okereke & McDaniels, Citation2012). Although the support volumes are based on a benchmark that reflects the most emission-efficient installations, the industry has been receiving more free allowances than necessary to cover its GHG emissions since the start of the EU ETS (Carbon Market Watch, Citation2016; Richards et al., Citation2018; Sandbag, Citation2018).
Up until 2010, EU climate policy for energy-intensive industries was mostly concerned with efficiency improvements and marginal emission reductions, conserving industrial structure rather than encouraging the systemic change needed for zero emission (Åhman & Nilsson, Citation2015). After 2010, the focus shifted to advancing technology and innovation as a means of enabling deep decarbonization. Innovation programmes so far stretch from laboratory-scale (e.g. in Horizon 2020 and Horizon Europe) up to demonstration plant funding (e.g. NER300 and the Innovation Fund). Correspondingly, industry has started to develop visions and innovative ideas to reduce emissions to essentially zero by mid-century (Eurofer, Citation2019b; HLG-EII, Citation2019; Jernkontoret, Citation2018).
Recent studies have estimated the production cost increase of these novel production methods to 68–80 EUR per tonne CO2 (Mandova et al., Citation2019; Vogl et al., Citation2018). Although uncertainty is high and cost-competitiveness is not static (see section 2.2), the EU ETS in its current form does not provide sufficiently high prices, and its volatility makes investments into capital-intensive projects risky. In addition, as technologies are not yet commercial and associated infrastructure is not in place, first movers face high technological risk and large investments. Thus, while the EU ETS plays an important role in driving the adoption of best available technology, it is not sufficient to remove the risks for first-of-a-kind investments into low-emission primary production technologies.
2.2. Policy for the early-commercialization stage
The EU has the opportunity and responsibility to lead innovation efforts for low-emission alternatives in primary steel production. A transition to green steel requires a systemic change of core elements of the production process. To fully decarbonize primary steel production, GHG emissions must either be fully avoided through electrification, or they must be captured and stored, while at least some of the fossil fuel energy input must be substituted with sustainable bioenergy, as carbon capture and storage (CCS) does not capture all emissions (Mandova et al., Citation2019). Both pathways increase the electricity demand of primary steel production. The production costs of novel processes are dependent on the energy systems and the new value chains they are embedded in. Depending on the technological path chosen, important factors include access to and costs of renewable electricity, green hydrogen, access to good quality metallurgical coal and biofuels, and the carbon price. Consequently, uncertainty about the economic viability of new technologies is high, with some studies concluding near cost-competitiveness, while others argue that the price for low-quality steel grades could almost double (Hölling & Gellert, Citation2018).
The cost-competitiveness of new technologies should not be understood as static but depends on the co-evolution of technological systems and the institutions that support and shape them (Unruh, Citation2002). Innovations such as producing green steel face a range of systemic disadvantages that are caused by lock-in, such as missing infrastructure, institutions specialized in the current system and risk-averse incumbents and finance (Unruh, Citation2000). Removing these barriers is a matter of industrial policy and affects the economic viability of current and future production methods. A policy strategy that aims to minimize the costs of deploying low-emission steelmaking will therefore pursue three distinct objectives: (1) Increase the share of secondary production while reducing overall steel demand; (2) improve conditions that enable low-emission steel, for example by nurturing demand, and by providing grid infrastructure and hydrogen supply; and (3) once new technologies seem viable, break the lock-in of the current production system, based on blast furnaces and coke, and thereby remove systemic barriers for new technologies.
A transition from one production system to another requires various sequential and overlapping policy approaches (Bataille, Citation2019). Initially, the up-scaling and commercial deployment of a new technology faces a series of risks that motivate public support. During the early stages of innovation, the technical feasibility of novel unit processes and new production systems is in focus. The closer the technology moves towards commercialization, the more financial risks come to the fore. These encompass both internal factors, such as a company's access to finance, and external ones, such as a customer's willingness to pay for a green premium or the risk of high future electricity prices. A first-of-a-kind industrial plant might initially face significantly higher production costs compared to established technologies. However, over time, this disadvantage wanes as learning and process improvements on the producer side, and reduced uncertainties and network effects on the user side, improve the performance of the novel production process (Sandén & Azar, Citation2005). Further risks that condition the viability of new versus old production systems are political, such as future climate policy and expectations concerning its level of ambition and continuity, as well as social, such as public reactions to the unequal distribution of transitional costs.
It is generally agreed upon in innovation studies that successful innovation policy needs both pushes and pulls, although demand-pull policy has been of varying importance to different innovations in different industrial sectors (Åhman, Citation2006; Mowery & Rosenberg, Citation1979; Nemet, Citation2009). In this study we evaluate policy options that push and pull a technological innovation (low-emission steel production) into an early commercial market. These policies aim to create a protective space, in which the innovation can become commercially feasible by being shielded, nurtured and empowered (Smith & Raven, Citation2012). Niches provide an opportunity for further technological improvement and can lead to cost reductions through increased experience and adoption (Sandén & Azar, Citation2005). Over time, niche markets in a country might evolve into lead markets (Beise, Citation2005).
Although this type of early commercialization policy is new for green basic materials it has worked in other sectors. For renewable energy, successful market creation policy instruments such as feed-in premiums, renewable portfolio standards and contracts for difference have provided stable conditions for investments into solar cells and wind turbines (Nemet, Citation2009; Wilson & Grubler, Citation2011). Learning effects across the value chain played a big part in the decrease of the levelised cost of wind power (−38% onshore, −29% offshore) and solar PV (−82%) since 2010 (IRENA, Citation2020). The extent to which learning can be expected for new steel production processes is not yet known, but learning rates are typically higher for modular small-scale technologies compared to large processes (Neij, Citation2008).
3. Methodology
Our analysis is based on an ex ante evaluation of policy approaches for the commercialization of low-emission steel production. We define the policy goal as realizing the first commercial-scale production of greenFootnote3 steel in Europe. With regards to this goal, we evaluate two principal policy options against effectiveness, feasibility, efficiency, and fairness.
Effectiveness denotes the degree to which the policy goal is met and is sometimes referred to as goal attainment (Huitema et al., Citation2011; Vedung, Citation1997). In our study, an effective policy must reduce the competitiveness gap between current and clean technologies while not causing carbon leakage. It should reduce risks for investments and not add any other significant risks. It also must not pose any barriers to reaching zero emissions in the sector in the long run.
The legal and institutional feasibility of a policy refers to how a policy instrument can effectively work and can be implemented in a specific institutional and political context. Here, legal feasibility concerns the legality of a policy in the EU and all its member states, if relevant. For a policy to be institutionally feasible it must fit with the current policy framework, such as the EU ETS which we assume to be long-lasting. We do not consider political feasibility, that is, finding political majorities for a policy.
Efficiency is not used uniformly in the evaluation literature, with some distinguishing between cost-effectiveness and efficiency (Huitema et al., Citation2011) or between cost–benefit and cost-effectiveness (Mickwitz, Citation2003; Vedung, Citation1997). In our study, we evaluate efficiency according to whether the policy encourages actors to meet the target with as low cost as possible (cost-effectiveness), avoiding overcompensation and excessive administration efforts.
The fairness of a policy, sometimes called equity (Arvizu et al., Citation2011; Mickwitz, Citation2003), considers how the costs and benefits of that policy are distributed in society. It should avoid or compensate for detrimental side effects as far as possible. Fairness issues can be found at different levels: between member states, between regions, between companies and between private and public actors.
We categorize policy instruments according to point of intervention, the regulated variable and whether it works as a stick or carrot. The point of intervention describes which part of the value chain is regulated. We consider interventions that support the supply (production) of finished steel or that increase or secure a volume of demand at the point of delivery to the manufacturer of end consumer products (e.g. white goods, cars, buildings). Supply-side measures thus support the production of green steel, while demand-side measures support its market uptake. For regulations we further distinguish between regulating prices and quantities (Hepburn, Citation2006). Either can act as carrots, incentivising greener production or consumption, or as sticks, discouraging more polluting production or consumption. The full typology including a non-exhaustive list of policy instruments is shown in .
Table 1. Typology of policy instruments for the early-commercialization phase of green steel production. The two main approaches are supply-side pricing and demand-side market creation. Instruments in italic text are not evaluated in this study.
The policy approaches under study were identified based on a review of recent literatureFootnote4 on steel decarbonization policies, which is mainly grey literature originating from climate advocacy organizations (e.g. European Climate Foundation), industrial associations (e.g. Eurofer), research institutes and the European Commission. The review focussed on instruments that aim at the creation or stimulation of demand for low- or zero- emission steel as their primary objective. Some polices related to steel decarbonization are thus beyond the scope of this study. For example, the material consumption charge laid out by Pollitt et al. (Citation2019) does incentivise reduced material use and recycling, but does not specifically target low-emission primary steelmaking as it would take the form of a uniform charge on steel based on a benchmark, not differentiating on the basis of an actual carbon footprint. Emission standards for production were excluded on the same grounds of not sufficiently targeting low-emission primary steelmaking. Further, we exclude demand-side pricing policies due to the administrative effort involved. Different products contain different amounts and qualities of steel, which makes the setup of schemes such as tax credits or buyers’ premiums highly complex. Finally, border carbon adjustments have been discussed at length by othersFootnote5 and will be referred to here only to provide context.
4. Policy evaluation
We distinguish two main approaches to early-commercialization policy: supply-side production subsidies and demand-side market creation. For each approach we first provide a description of policy instruments, followed by an evaluation presented according to the four variables of the analytical framework.
4.1. Carbon contracts for difference
A carbon contract for difference (CCfD) is a subsidy agreement between the regulator and a steel producer aiming to realize a decarbonization project. The volume of the annual subsidy payment is determined by the difference between the carbon price and a negotiated or tendered strike price and by the emissions reductions achieved by the proposed project. The strike price represents the carbon price that the project requires to become economically viable. By guaranteeing a constant carbon price for 20–30 years, a CCfD aims to reduce the carbon price risk for the steel producer (Elkerbout & Egenhofer, Citation2018; Helm & Hepburn, Citation2005). This elevation of the carbon price allows the commercial operation of technologies that require higher carbon prices than found on the current market. If the carbon market price exceeds the strike price, the relationship is inversed and the difference is paid back.Footnote6 If, for example, a firm were to compare two investments – the refurbishment of its blast furnace versus the construction of a hydrogen-based process – and it could realize the latter with a carbon price of 70 EUR per tonne CO2, this would be the strike price needed. The firm would then receive the difference between the strike price and the average annual carbon price in the EU ETS for each tonne of CO2 that is not emitted as the result of the lower-carbon investment option.
Effectiveness: Production subsidies through CCfDs can be an effective way to commercialize low-emission primary steel production if designed right. The effectiveness of the approach depends on the volume of monetary support offered by the regulator, which in turn determines how many projects can be realized. Sartor and Bataille (Citation2019) have argued that only first-of-a-kind projects should be subsidized. However, this would create only a small market for green steel and thus allow for only limited technology learning. On the other hand, competitive tendering requires that only some producers benefit from the scheme. Irrespective of the support volume, eligibility criteria must avoid carbon lock-in in the medium term. Even if a supported project can reduce the emission intensity of steel production by half, eventually it must become carbon neutral by 2050. Such a climate neutrality ‘stress test’ could take the shape of the regulator requiring a production-site-based roadmap to zero emissions in the application process. Furthermore, a CCfD can reduce the financing cost for a project, as the stable cashflow increases a project's access to debt (Neuhoff et al., Citation2019). However, CCfDs alone might not suffice to reduce the investment risks for low-emission primary steel production. Especially for producers moving into electrification, future electricity prices become a key determinant of production costs. A stable carbon price through a CCfD might thus not be enough to guarantee a business case. In this instance, government-backed electricity price guarantees can be a complementary policy to CCfD. Most EU energy-intensive industries already benefit from exemptions from taxes and renewable levies when procuring electricity (Åhman & Nilsson, Citation2015).
The feasibility of CCfDs depends on the evolution of existing policies such as the EU ETS, State Aid rules, and on how they can be funded. As an extension of the EU ETS, CCfDs are co-dependent with its development, especially the fate of free allocation and the respective benchmark levels. If benchmarks are lowered, and the amount of allowances freely allocated across the sector is reduced according to the principle of competition-neutrality, the beneficiary might effectively receive less support than expected. CCfD support levels can be determined through negotiations, strike price offers by the regulator or tendering, which could be implemented either on EU or member state level. Some authors have suggested that government-determined strike prices could come into conflict with current EU State Aid rules (Richstein, Citation2017; Sartor & Bataille, Citation2019). At the EU level, funding can either be based within or outside the EU budget, for example through ‘explor[ing] whether part of the funding being liquidated under the European Coal and Steel Community can be used’ (EC, Citation2019, pp. 8–9), through more auctioning of emission allowances or through consumption taxes (cf. Pollitt et al., Citation2019).
CCfDs are feasible irrespective of whether leakage protection under the EU ETS happens through free allocation or through border carbon adjustments (BCA), which we illustrate in . BCA are tariffs on imported goods, such as steel, according to its carbon content and thereby seek to even out the costs that the EU ETS imposes on European producers. In theory, BCA are an alternative to free allocation of emission allowances; a simultaneous use of both schemes, as requested by some (Eurofer, Citation2020), would overcompensate European producers. However, steel exported from the EU would be disadvantaged if BCA do not contain a reverse adjustment at the border in return, for example in the form of an export credit. At the same time, EU premium markets disadvantage non-EU producers of green steel that do not receive support under an equal domestic CCfD scheme.
Figure 2. Schematic illustration of steel production costs for EU producers under CCfD support versus non-EU producers. Left side: production of conventional steel. Right side: production of green steel. Top: current EU ETS setup with free allocation. Bottom: EU ETS with border carbon adjustment. Equal production costs for EU and non-EU producers were assumed.
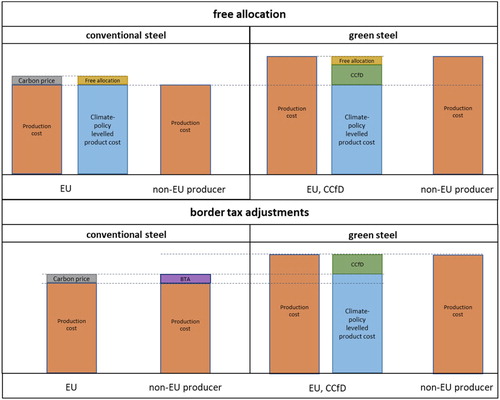
Efficiency is highest when CCfDs are awarded through tenders. Determining the strike price levels through tenders reduces information asymmetry for governments and thus increases cost-efficiency. Competitive tenders are more likely to work at the EU level than at the national level, as many EU member states are only home to one or a small number of primary steelmakers that could take part in such tenders. However, national tenders could include several sectors to reach the necessary scale. CCfDs can build on the EU ETS and its monitoring, reporting and verification system which lowers the administrative effort needed (Richstein, Citation2017). Finally, there is a risk of overcompensation if the ability to pass-through costs increases while strike price levels remain the same. This is especially relevant once the bulk of the steel industry moves towards cleaner production methods and the end consumer demand for green steel grows, which would increase the producer's ability to pass-through costs.
Fairness: CCfDs will likely distribute costs unequally. The tendering of CCfD would benefit powerful EU members with low electricity prices or good access to renewable energy, biomass or carbon storage opportunities at scale, while regions without these conditions would be disadvantaged. A tender will necessarily yield some primary producers that do not win support. These producers and the regions and communities they are situated in might require additional policy support in the transition. Furthermore, the length of contract periods faces a trade-off between reducing the uncertainty associated with political decisions that can discourage technology investment (Richstein, Citation2017), and burdening future administrations and generations with subsidy obligations.
4.2. Demand-side market creation
Creating markets for green steel through regulatory or voluntary measures relies on information about the embodied emissions of steel products or goods containing steel. We distinguish between two ways to create and share this information: tradable certificates and carbon footprint tracing. In the first case, producers of green steel can issue certificates that can be purchased by users on a certificate market, akin to tradable renewable certificates (IRENA, IEA & REN21, Citation2018). Depending on supply and demand, certificate prices vary. While users willing to buy green steel can procure steel from any producer, the purchase of certificates ensures that the green premium goes to the producer of green primary steel. The second case of tracing of carbon footprints requires data collection at each stage in the value chain, including information about the import of raw materials, and semi-finished and finished steel products into the EU. This information must be continually updated, verified and made available to all potential users.
Once a system for collection and sharing of information is in place, it can be used for regulatory policies and voluntary actions by actors wanting to use green steel. Regulatory approaches either obligate the use of steel with low embodied emissions in certain goods, which we call quota schemes, or restrict or forbid the use of steel with a high footprint. A legal scheme regulating quantities typically consists of three parts: (1) Setting a quantity, (2) allocating certificates, and (3) enacting a compliance mechanism (Hepburn, Citation2006). Both approaches can use either certificates or traced data.
Green public procurement (GPP) follows the same logic. Reliable information about the embodied emissions is needed in order to procure climate-friendlier materials. GPP can then be practiced on a voluntary basis or combined with quotas or restrictions. Practical examples of GPP already exist; for example, the Swedish Transport Administration set upper limits for allowed material climate impacts (Toller & Larsson, Citation2017). Another policy instrument depending on a system for tracing carbon footprints is labelling, which aims at making externalities visible to the consumer. Several industry labels exist today that communicate environmental and climate performance, such as industry-led initiatives like ResponsibleSteel and SustSteel (Eurofer), and the European Union Product Environmental Footprint Category Rules (PEFCR).
Effectiveness: Neither certificate-based nor tracing-based market creation policy is likely to be very effective with respect to the policy goal of supporting low-emission primary steel production. Policies based on certificates add an additional certificate price risk for investors, besides already existing risks such as raw material market prices and the variation in the carbon price (Mitchell et al., Citation2006). Regulation based on footprinting and tracing suffers from limited knowledgeFootnote7 regarding which goods mainly or only use primary steel; a quota for such goods would successfully impact primary steel production. If a quota were imposed on a sector at random, it is likely that producers will seek to use secondary steel instead of primary due to the lower carbon footprint of the secondary route. Public procurement is a case in point. In total, 14% of the EU GDP is spent through public procurement (European Commission, Citation2017), using approximately 14% of steel (based on UK numbers: UK BEIS, Citation2019). Sectors in which public procurement dominates are defence and the construction and maintenance of infrastructure such as highways and railroads, energy or water (Marron, Citation2004). Most of these sectors mainly use long products, especially rebar, rail and steel sections, which are typically already produced through the secondary route (Sekiguchi, Citation2017). Thus, public procurement of green steel is unlikely to have a large impact in terms of support for low-emission primary steelmaking.
The effectiveness of quotas and restrictions depends on how well steel is specifically targeted by the regulation. Although the most effective choice to support markets for green steel would be to directly regulate the steel used in goods, this would likely lead to material substitution and large administrative efforts, e.g. if separate policies are needed for all the materials of a car. In contrast, horizontal policies such as performance-based standards that set upper limits for emission per kilometre travelled, despite being more cost-efficient, have only little impact on primary steel production, as other emission reductions might be easier or cheaper to achieve than using green steel.
Analogous to price regulation as discussed above, eligibility criteria determining which producers receive certificates or are endorsed with labels can lead to medium-term carbon lock-in (see also section 4.1). Finally, quantity regulation should be planned well ahead in order to create an expectation of stable demand and thereby investor certainty.
Feasibility: Although in principle feasible within the existing EU policy framework, in order to comply with World Trade Organisation rules, certificate markets might have to be extended to non-EU countries. Tracing of embodied emissions can build on existing EU programmes such as the PEFCR (see above) or the Ecodesign Directive (EU, Citation2009). Furthermore, the steel industry has so far not shown much agreement on methodology for life-cycle assessment (LCA). Different LCA guidelines have been suggested to determine the carbon footprint of primary and secondary steel, but ultimately the definition of ‘green steel’ and which methods and system boundaries to use, is a political decision (Vogl & Åhman, Citation2019).
Efficiency: Regulating quantities entails large administrative efforts. Certificate-based quantity regulation rests on the creation of a certificate market that needs monitoring, reporting and verification, as well as sanctions for non-compliance. Setting up a carbon footprint tracing system requires capacity for continuous data collection, handling and verification within and outside the EU. The cost-efficiency of market creation can be quite low if the policy leads to significant spill-overs outside of the jurisdiction. This is the case if domestic markets are to a large extent supplied by goods not produced domestically. On the upside, these spill-overs stimulate the development of a cleaner steel industry even outside Europe, which is in line with the EU's responsibility to lead the transition under the United Nations Framework Convention on Climate Change (UNFCCC).
The main advantage of a certificate-based over a tracing system is the built-in trading scheme, which adds flexibility for producers and consumers. This is especially important in complex markets when certain goods that meet the requirements of the quota might not be available from a certified producer. Through obtaining certificates, the real carbon footprint of a product does not matter for the end consumer.
Market creation policy can be phased in on a voluntary basis and later evolve into regulation. Certificates can start as voluntary certificate trade between producers and users willing to buy ‘greener’ steel. Building codes can be a first step to mandatory emission standards in construction. Labelling can be used as a supplementary policy to stricter regulation, for example as a first step in regulating a previously untouched sector by serving as a base upon which further regulation can be built upon (Somanathan et al., Citation2014). Once labels are in place, ratcheting up regulation becomes easier through several mechanisms. Firstly, introducing labelling sheds light on previously invisible problems, which might induce innovation or increase consumer demand for the endorsed product. Secondly, a data collection, monitoring and verification scheme needs to be put in place for the implementation of labels which further regulation can draw on. Labels face the same limitations regarding LCA-based tracing of carbon footprints discussed above.
Fairness: Imposing quotas or standards increases the costs of steel for the end consumer. This can lead to resistance by sectors or businesses obliged to purchase green steel. Although the share of steel in the cost structure of final products is typically below 1% (Rootzén & Johnsson, Citation2016), significant cost increases can be expected for construction, where steel can become a significant cost factor that is passed through to end consumers (Andersson, Citation2020).
5. Discussion
Here we discuss the main political choices and side effects of early-commercialization policy for low-emission primary steelmaking in Europe. We have identified and analyzed two principal approaches: (1) production subsidies through CCfDs; and (2) demand-side market creation for green steel.
CCfDs (1) are an effective instrument to realize the first industrial-scale production of low-emission steel production. However, as many of the discussed technologies are highly sensitive to electricity costs, changes in electricity price are a high risk even for producers under CCfD support, which could be mitigated through long-term electricity price guarantees. The creation of markets (2) for green steel is not as effective in promoting clean primary steelmaking. Market creation instruments either add additional risks, as is the case with quota schemes based on tradable certificates, or do not fully deliver the benefit to the intended producers due to the complexity of steel markets. However, market creation policy can still be warranted by other policy goals such as creating international demand for green basic materials and thereby incentivising climate action in other countries. The global spill-overs that market formation necessarily causes could trigger investments into low-emission steel production outside the EU. Making information available and fostering premium markets early can increase the willingness to pay for green steel. While this would reduce the strike price levels needed for CCfDs, it can also lead to overcompensation.
CCfDs for the steel industry have several fairness implications. If CCfDs operate under the current practice of free allocation in the EU ETS, there is a risk of exacerbating the already existing problem of overcompensation due to cost pass-through. Furthermore, the main drawback of production subsidies is the distribution of transitional costs. Inter-firm competition in tenders implies that only some projects can be supported. Considering the increasing electricity demand of novel steel production technologies, these will likely be located in regions with good access to large amounts of cheap electricity. At the same time, the EU seeks to make its economy more circular, which necessitates increased recycling and a reduction of total steel demand and production. This means that some mills that currently produce primary steel either will have to close or convert their operations to scrap recycling, thereby retaining their downstream metalworking operations. It is plausible that regions that are adversely positioned in the competition for CCfD support are the very same as those most affected by global overcapacity and a move to more secondary steel production. A fair and politically feasible policy package for the early-commercialization phase of steel should consider providing transitional assistance to affected regions and workforces (Green & Gambhir, Citation2019), either in converting their plants to secondary production or even for closure.
Workers are a particularly vulnerable group in the steel transition. According to Eurofer (Citation2019a), the European steel industry directly employs 330,000 people, with more than 2 million jobs indirectly associated with the sector. The replacement of Europe's old steel plant stock with state-of-the-art steel mills is likely to increase the degree of automation and digitalization in steel plants. This could lead to a decrease in labour intensity in primary production. On the other hand, a more circular steel industry could see additional jobs in recycling, remanufacturing and reuse. In this case, retraining programmes for the current workforce could cushion the impact. Further, if prices for basic materials increase, sectors like construction may respond by increasing prices and reducing wages (Andersson, Citation2020).
In the European Commission's own words, ‘[i]t takes 25 years – a generation – to transform an industrial sector and all the value chains. To be ready in 2050, decisions and actions need to be taken in the next five years’ (EC, Citation2019, p. 7). Based on previous estimations, a full switch to alternative production methods will increase production costs by 1.7–4.5 bn EUR depending on the size of primary steelmaking in Europe.Footnote8 Material efficiency and demand reduction measures can significantly reduce the overall transition costs (Hernandez et al., Citation2018). Climate targets will only be met if the supported projects are able to move to deep decarbonization in the medium term. Carbon lock-in is a risk that cuts across all mentioned policy strategies and is even relevant for R&D policy such as the Innovation Fund. This implies that firms must be able to demonstrate that their projects can meet long-term climate goals. Such a climate stress test should build on the latest knowledge of technological options and should be continually updated.
Irrespective of the chosen policy approach, the perceived transition risk at firm-level strongly depends on the fate of the EU ETS. A major obstacle to the transition is uncertainty about free allocation levels if producers move away from the blast furnace and thus might be subject to another benchmark. If a company fears losing its free allocation by moving to a low-emission production process, then not only the incentive that carbon pricing should create is non-existent, but a perverse incentive is created that keeps firms from investing into breakthrough technologies. Urgent clarification and a commitment by the Commission on the allocation levels for breakthrough technologies is needed. Furthermore, technology-specific benchmarks pick winners if allocation levels are different for the same emissions reduction volumes. This would be the case if, for example, a steelmaker can choose to achieve a 50% emissions reduction target by retrofitting CCS or through a new process (e.g. hydrogen enriched direct reduction) but would receive different amounts of free allowances for these projects.
The policies discussed here constitute one part of a policy mix to decarbonize the steel industry. Besides innovation support, once viable technological alternatives to current fossil fuel-based production systems are feasible, a dedicated phase-out policy (e.g. retirement programme, bans, sunset clauses) must ensure that low-carbon alternatives do not co-exist alongside their fossil fuel precursors. Finally, not all European steel sites might win out in a low-carbon transition. In particular, areas without access to plentiful renewable energy or renewable hydrogen might lose out in the process. The Just Transition Fund could assist these regions and local populations.
6. Conclusion
The European Commission has committed to a transformation of industry in line with climate neutrality. Inspired by the success of wind and solar energy policy, a more interventionist policy style using subsidies and market creation has been promoted by energy-intensive industries and European policy makers. In order to fully decarbonize the steel sector by 2050, the first industrial-scale steel mills producing green steel should be commercialized within the next decade. Due to various risks and likely increases in production costs, this requires policy interventions.
We compare two principal policy approaches to support commercialization: production subsidies through CCfDs and demand side market creation. CCfDs can effectively enable the commercialization of breakthrough technologies, while market creation can reduce the subsidy volumes needed and aid the global diffusion of new production methods. Subsidies through CCfDs are feasible and most efficient if awarded in competitive tenders. However, as electricity demand is set to increase in the steel sector, long-term electricity price arrangements should be considered to complement CCfDs. At the same time, production subsidies will lead to an unequal distribution of transition costs and benefits. Regions and member states with little access to large amounts of inexpensive renewable energy are likely to be disadvantaged in the transition.
Early-commercialization policies are one part of a policy mix to decarbonize the steel industry. Continued supply push efforts, such as innovation support or investment grants, are needed. Once viable technological alternatives to current fossil fuel-based production systems are feasible, a dedicated phase-out policy must ensure high emitters do not continue to operate alongside the low-carbon alternatives. Finally, to ensure a just transition, assistance will be required for disadvantaged regions and their communities.
Acknowledgement
This work was supported by the Swedish Energy Agency under the HYBRIT-RP1 project and by H2020 under Grant 730053 REINVENT. The authors thank Karin Ericsson and Bengt Johansson for their comments on a previous draft.
Disclosure statement
No potential conflict of interest was reported by the author(s).
Correction Statement
This article has been republished with minor changes. These changes do not impact the academic content of the article.
Additional information
Funding
Notes
1 For the remainder of this article we will use the terms low-emission and green to refer to novel steel production methods with very low or no CO2 emissions.
2 Steelmakers with hydrogen plans and/or net-zero goals in the EU: SSAB, Voestalpine, Salzgitter, ThyssenKrupp Steel, ArcelorMittal Hamburg. Other technologies are also being explored, e.g. electrowinning and different carbon capture and storage or utilisation approaches.
3 The decision of what counts as green is essentially a political one and should be aligned with the Paris Agreement (Vogl & Åhman, Citation2019).
4 The main sources of our review were: EC (Citation2019); HLG-EII (Citation2019); IRENA, IEA and REN21 (Citation2018); Neuhoff et al. (Citation2014); Neuhoff et al. (Citation2018); Neuhoff et al. (Citation2019); Wyns et al. (Citation2019). Other sources are cited as appropriate in section 4.
5 See literature on BCA, e.g. Ismer and Neuhoff (Citation2007); Monjon and Quirion (Citation2011) and Eckersley (Citation2011).
6 A recent contribution by Sartor and Bataille (Citation2019) deviates from this. In their version, once the carbon price exceeds the strike price, no or only partial payments occur.
7 Besides simple heuristics, no scientific literature is available that assigns steel products to primary and secondary production route.
8 Based on production outlooks in section 2 and cost increases of 80 EUR/t steel (Mandova et al., Citation2019; Vogl et al., Citation2018).
References
- Åhman, M. (2006). Government policy and the development of electric vehicles in Japan. Energy Policy, 34(4), 433–443. https://doi.org/10.1016/j.enpol.2004.06.011
- Åhman, M., & Nilsson, L. J. (2015). Decarbonising industry in the EU – Climate, trade and industrial policy strategies. In S. Oberthur & C. Dupont (Eds.), Decarbonisation in the European Union: Internal policies and external strategies (pp. 92–114). Palgrave Macmillan.
- Allwood, J. M., & Cullen, J. M. (2012). Sustainable materials: With both eyes open. UIT Cambridge Ltd.
- Allwood, J. M., Gutowski, T. G., Serrenho, A. C., Skelton, A. C. H., & Worrell, E. (2017). Industry 1.61803: The transition to an industry with reduced material demand fit for a low carbon future. Philosophical Transactions of the Royal Society A: Mathematical, Physical and Engineering Sciences, 375(2095), 20160361. https://doi.org/10.1098/rsta.2016.0361
- Andersson, F. N. G. (2020). Effects on the manufacturing, utility and construction industries of decarbonization of the energy-intensive and natural resource-based industries. Sustainable Production and Consumption, 21, 1–13. https://doi.org/10.1016/j.spc.2019.10.003
- Arvizu, D., Bruckner, T., Chum, H., Edenhofer, O., Estefen, S., Faaij, A., Fischedick, M., Hansen, G., Hiriart, G., Hohmeyer, O., Hollands, K. G. T., Huckerby, J., Kadner, S., Killingtveit, Å, Kumar, A., Lewis, A., Lucon, O., Matschoss, P., Maurice, L., … Zwickel, T. (2011). Technical Summary. In O. Edenhofer, R. Pichs-Madruga, Y. Sokona, K. Seyboth, P. Matschoss, S. Kadner, & T. Zwickel (Eds.), IPCC special report on renewable energy sources and climate change mitigation (pp. 1–132). Cambridge University Press.
- Bataille, C. G. F. (2019). Physical and policy pathways to net-zero emissions industry. WIREs Climate Change, 2019, e633. https://doi.org/10.102/wcc.633
- Beise, M. (2005). Lead markets, innovation differentials and growth. International Economics and Economic Policy, 1(4), 305–328. https://doi.org/10.1007/s10368-004-0013-x
- Carbon Market Watch. (2016). Industry windfall profits from Europe’s carbon market.
- Cooper-Searle, S., Livesey, F., & Allwood, J. M. (2018). Why are material efficiency solutions a limited part of the climate policy agenda? An application of the multiple streams framework to UK policy on CO2 emissions from cars. Environmental Policy and Governance, 28(1), 51–64. https://doi.org/10.1002/eet.1782
- Daehn, K. E., Cabrera Serrenho, A., & Allwood, J. M. (2017). How will copper contamination constrain future global steel recycling? Environmental Science & Technology, 51(11), 6599–6606. https://doi.org/10.1021/acs.est.7b00997
- EC. (2017). Making public procurement work in and for Europe (vol. 572).
- EC. (2019). The European green deal.
- EC. (2020). A new industrial strategy for Europe, COM(2020) 102 final.
- Eckersley, R. (2011). The politics of carbon leakage and the fairness of border measures. Ethics & International Affairs, 24(4), 367–393. https://doi.org/10.1111/j.1747-7093.2010.00277.x
- EEA. (2018, December 11). EU Emissions Trading System (ETS) data viewer. https://www.eea.europa.eu/data-and-maps/dashboards/emissions-trading-viewer-1
- Elkerbout, M., & Egenhofer, C. (2018). Tools to boost investment in low-carbon technologies: Five possible ways to create low-carbon markets in the EU. CEPS.
- EU. (2009). Directive 2009/125/EC of the European Parliament and the Council of 21 October 2009 establishing a framework for the setting of ecodesign requirements for energy-related products.
- Eurofer. (2013). A steel roadmap for a low carbon Europe 2050.
- Eurofer. (2019a). European steel in figures 2019.
- Eurofer. (2019b). Low carbon roadmap: Pathways to a CO2-neutral European steel industry.
- Eurofer. (2020). EU green deal on steel: EU climate leadership requires waterproof carbon leakage measures (vol. 3).
- Fleiter, T., Herbst, A., Rehfeldt, M., & Arens, M. (2019). Industrial innovation part 2: Scenario analysis and pathways to deep decarbonisation. Fraunhofer ISI.
- Green, F., & Gambhir, A. (2019). Transitional assistance policies for just, equitable and smooth low-carbon transitions: Who, what and how? Climate Policy, 1–20. https://doi.org/10.1080/14693062.2019.1657379
- Helm, D., & Hepburn, C. (2005). Carbon contracts and energy policy: An outline proposal. Oxford University.
- Hepburn, C. (2006). Regulation by prices, quantities, or both: A review of instrument choice. Oxford Review of Economic Policy, 22(2), 226–247. https://doi.org/10.1093/oxrep/grj014
- Hernandez, A. G., Cooper-Searle, S., Skelton, A. C. H., & Cullen, J. M. (2018). Leveraging material efficiency as an energy and climate instrument for heavy industries in the EU. Energy Policy, 120, 533–549. https://doi.org/10.1016/j.enpol.2018.05.055
- Hertwich, E. G., Ali, S., Ciacci, L., Fishman, T., Heeren, N., Masanet, E., Asghari, F. N., Olivetti, E., Pauliuk, S., Tu, Q., & Wolfram, P. (2019). Material efficiency strategies to reducing greenhouse gas emissions associated with buildings, vehicles, and electronics—a review. Environmental Research Letters, 14(4), 043004. https://doi.org/10.1088/1748-9326/ab0fe3
- HLG-EII. (2019). Masterplan for a competitive transformation of EU energy-intensive industries enabling a climate-neutral, circular economy by 2050.
- Hölling, M., & Gellert, S. (2018). Direct reduction: Transition from natural gas to hydrogen? [Paper presentation]. ICSTI 2018, Vienna.
- Huitema, D., Jordan, A., Massey, E., Rayner, T., van Asselt, H., Haug, C., Hildingsson, R., Monni, S., & Stripple, J. (2011). The evaluation of climate policy: Theory and emerging practice in Europe. Policy Sciences, 44(2), 179–198. https://doi.org/10.1007/s11077-011-9125-7
- IEA. (2017). Renewable energy for industry.
- IPCC. (2018). Summary for policymakers. In: Global warming of 1.5°C. An IPCC special report on the impacts of global warming of 1.5°C above pre-industrial levels and related global greenhouse gas emission pathways, in the context of strengthening the global response to the threat of climate change, sustainable development, and efforts to eradicate poverty.
- IRENA. (2020). Renewable power generation costs in 2019.
- IRENA, IEA & REN21. (2018). Renewable energy policies in a time of transition.
- Ismer, R., & Neuhoff, K. (2007). Border tax adjustment: A feasible way to support stringent emission trading. European Journal of Law and Economics, 24(2), 137–164. https://doi.org/10.1007/s10657-007-9032-8
- Jernkontoret. (2018). Klimatfärdplan – För en fossilfri och konkurrenskraftig stålindustri i Sverige. Stockholm, Sweden: Jernkontoret.
- Kallis, G. (2017). Radical dematerialization and degrowth. Philosophical Transactions of the Royal Society A: Mathematical, Physical and Engineering Sciences, 375(2095), 20160383. https://doi.org/10.1098/rsta.2016.0383
- Kushnir, D., Hansen, T., Vogl, V., & Åhman, M. (2020). Adopting hydrogen direct reduction for the Swedish steel industry: A technological innovation system (TIS) study. Journal of Cleaner Production, 242, 118185. https://doi.org/10.1016/j.jclepro.2019.118185
- Mandova, H., Patrizio, P., Leduc, S., Kjärstad, J., Wang, C., Wetterlund, E., Kraxner, F., & Gale, W. (2019). Achieving carbon-neutral iron and steelmaking in Europe through the deployment of bioenergy with carbon capture and storage. Journal of Cleaner Production, 218, 118–129. https://doi.org/10.1016/j.jclep.2019.01247 doi: 10.1016/j.jclepro.2019.01.247
- Marron, D. (2004). Greener public purchasing as an environmental policy instrument. OECD Journal on Budgeting, 3(4), 71–105. https://doi.org/10.1787/budget-v3-art23-en
- Material Economics. (2018). The circular economy: A powerful force for climate mitigation. Stockholm, Sweden: Materials Economics.
- Mickwitz, P. (2003). A framework for evaluating environmental policy instruments: Context and key concepts. Evaluation, 9(4), 415–436. https://doi.org/10.1177/135638900300900404
- Milford, R. L., Pauliuk, S., Allwood, J. M., & Muller, D. B. (2013). The roles of energy and material efficiency in meeting steel industry CO2 targets. Environmental Science & Technology, 47(7), 3455–3462. https://doi.org/10.1021/es3031424
- Mitchell, C., Bauknecht, D., & Connor, P. M. (2006). Effectiveness through risk reduction: A comparison of the renewable obligation in England and Wales and the feed-in system in Germany. Energy Policy, 34(3), 297–305. https://doi.org/10.1016/j.enpol.2004.08.004
- Monjon, S., & Quirion, P. (2011). Addressing leakage in the EU ETS: Border adjustment or output-based allocation? Ecological Economics, 70(11), 1957–1971. https://doi.org/10.1016/j.ecolecon.2011.04.020
- Mowery, D., & Rosenberg, N. (1979). The influence of market demand upon innovation: A critical review of some recent empirical studies. Research Policy, 8(2), 102–153. https://doi.org/10.1016/0048-7333(79)90019-2
- Neij, L. (2008). Cost development of future technologies for power generation—A study based on experience curves and complementary bottom-up assessments. Energy Policy, 36(6), 2200–2211. https://doi.org/10.1016/j.enpol.2008.02.029
- Nemet, G. F. (2009). Demand-pull, technology-push, and government-led incentives for non-incremental technical change. Research Policy, 38(5), 700–709. https://doi.org/10.1016/j.respol.2009.01.004
- Neuhoff, K., Acworth, W., Ancygier, A., Branger, F., Christmas, I., Haussner, M., Ismer, R., Rooij, A., Sartor, O., Sato, M., & Schopp, A. (2014). Carbon control and competitiveness post 2020: The steel report. London , UK: Climate Strategies.
- Neuhoff, K., Chiappinelli, O., Bataille, C., Haußner, M., Ismer, R., Joltreau, E., Jürgens, I., Piantieri, C., Richstein, J., Sartor, O., Singhal, P., & Stede, J. (2018). Filling gaps in the policy package to decarbonise production and use of materials. UK, London: Climate Strategies.
- Neuhoff, K., Chiappinelli, O., Gerres, T., Haussner, M., Ismer, R., May, N., Pirlot, A., & Richstein, J. (2019). Building blocks for a climate-neutral European industrial sector. UK, London: Climate Strategies.
- Okereke, C., & McDaniels, D. (2012). To what extent are EU steel companies susceptible to competitive loss due to climate policy? Energy Policy, 46, 203–215. https://doi.org/10.1016/j.enpol.2012.03.052
- Pauliuk, S., Milford, R. L., Muller, D. B., & Allwood, J. M. (2013). The steel scrap age. Environmental Science & Technology, 47(7), 3448–3454. https://doi.org/10.1021/es303149z
- Pauliuk, S., Wang, T., & Müller, D. B. (2013). Steel all over the world: Estimating in-use stocks of iron for 200 countries. Resources, Conservation and Recycling, 71, 22–30. https://doi.org/10.1016/j.resconrec.2012.11.008
- Pollitt, H., Neuhoff, K., & Lin, X. (2019). The impact of implementing a consumption charge on carbon-intensive materials in Europe. Climate Policy, 20, 74–89. https://doi.org/10.1080/14693062.1605969 doi: 10.1080/14693062.2019.1605969
- Quitzow, R., Walz, R., Köhler, J., & Rennings, K. (2014). The concept of “lead markets” revisited: Contribution to environmental innovation theory. Environmental Innovation and Societal Transitions, 10, 4–19. https://doi.org/10.1016/j.eist.2013.11.002
- Richards, J.-A., Röhrig, K., & McLynn, M. (2018). European fat cats EU energy intensive industries: Paid to pollute, not to decarbonise. CAN Europe.
- Richstein, J. C. (2017). Project-based carbon contracts: A way to finance innovative low-carbon investments. DIW.
- Rootzén, J., & Johnsson, F. (2016). Paying the full price of steel – Perspectives on the cost of reducing carbon dioxide emissions from the steel industry. Energy Policy, 98, 459–469. https://doi.org/10.1016/j.enpol.2016.09.021
- Sandbag. (2018). EU ETS dashboard (2018 ed.). Retrieved February 20, 2019, from http://sandbag-climate.github.io/
- Sandén, B. A., & Azar, C. (2005). Near-term technology policies for long-term climate targets—Economy wide versus technology specific approaches. Energy Policy, 33(12), 1557–1576. https://doi.org/10.1016/j.enpol.2004.01.012
- Sartor, O., & Bataille, C. (2019). Decarbonising basic materials in Europe: How carbon contracts-for-difference could help bring breakthrough technologies to market. IDDRI.
- Sekiguchi, N. (2017). Trade specialisation patterns in major steelmaking economies: The role of advanced economies and the implications for rapid growth in emerging market and developing economies in the global steel market. Mineral Economics, 30(3), 207–227. https://doi.org/10.1007/s13563-017-0110-2
- Smith, A., & Raven, R. (2012). What is protective space? Reconsidering niches in transitions to sustainability. Research Policy, 41(6), 1025–1036. https://doi.org/10.1016/j.respol.2011.12.012
- Söderholm, P., & Tilton, J. E. (2012). Material efficiency: An economic perspective. Resources, Conservation and Recycling, 61, 75–82. https://doi.org/10.1016/j.resconrec.2012.01.003
- Somanathan, E., Sterner, T., Sugiyama, T., Chimanikire, D., Dubash, N. K., Essandoh-Yeddu, J., Fifita, S., Goulder, L., Jaffe, A., Labandeira, X., Managi, S., Mitchell, C., Montero, J. P., Teng, F., & Zylicz, T. (2014). National and sub-national policies and institutions. In O. Edenhofer, R. Pichs-Madruga, Y. Sokona, E. Farahani, S. Kadner, K. Seyboth, A. Adler, I. Baum, S. Brunner, P. Eickemeier, B. Kriemann, J. Savolainen, S. Schlömer, C. von Stechow, T. Zwickel, & J. C. Minx (Eds.), Climate change 2014: Mitigation of climate change. Contribution of Working Group III to the Fifth Assessment Report of the Intergovernmental Panel on Climate Change. Cambridge University Press.
- Toller, S., & Larsson, M. (2017). Implementation of life cycle thinking in planning and procurement at the Swedish transport administration. In Pavement life-cycle assessment – Proceedings of the pavement life-cycle assessment symposium, 2017 (pp. 281–287).
- UK BEIS. (2019). Steel public procurement – Compliance with the steel procurement guidance (PPN 11/16).
- Unruh, G. C. (2000). Understanding carbon lock-in. Energy Policy, 28(12), 817–830. https://doi.org/10.1016/S0301-4215(00)00070-7
- Unruh, G. C. (2002). Escaping carbon lock-in. Energy Policy, 30(4), 317–325. https://doi.org/10.1016/S0301-4215(01)00098-2
- van Ruijven, B. J., van Vuuren, D. P., Boskaljon, W., Neelis, M. L., Saygin, D., & Patel, M. K. (2016). Long-term model-based projections of energy use and CO2 emissions from the global steel and cement industries. Resources, Conservation and Recycling, 112, 15–36. https://doi.org/10.1016/j.resconrec.2016.04.016
- Vedung, E. (1997). Models of evaluation. In Public policy and program evaluation (pp. 35–92). Transaction Publishers.
- Vogl, V., & Åhman, M. (2019). What is green steel? – Towards a strategic decision tool for decarbonising EU steel [Paper presentation]. METEC-ESTAD 2019, Düsseldorf, Germany.
- Vogl, V., Åhman, M., & Nilsson, L. J. (2018). Assessment of hydrogen direct reduction for fossil-free steelmaking. Journal of Cleaner Production, 203, 736–745. https://doi.org/10.1016/j.jclepro.2018.08.279
- Wilson, C., & Grubler, A. (2011). Lessons from the history of technological change for clean energy scenarios and policies. Natural Resources Forum, 35(3), 165–184. https://doi.org/10.1111/j.1477-8947.2011.01386.x
- World Steel Association. (2009). Statistical yearbook 2009.
- World Steel Association. (2019). Steel statistical yearbook 2018.
- Wyns, T., Khandekar, G., Axelson, M., Sartor, O., & Neuhoff, K. (2019). Industrial transformation 2050: Towards an industrial strategy for a climate neutral Europe. European Climate Foundation.
- Xylia, M., Silveira, S., Duerinck, J., & Meinke-Hubeny, F. (2018). Weighing regional scrap availability in global pathways for steel production processes. Energy Efficiency, 11(5), 1135–1159. https://doi.org/10.1007/s12053-017-9583-7