Abstract
Cathepsin B is a vitally important enzyme in various physiological processes and in tumor invasion and metastasis. A cathepsin B inhibitor, HCB-SunI, was identified and purified from sunflower seeds, Helianthus annuus, using ammonium sulfate precipitation and two steps of conventional chromatography. The molecular mass of HCB-SunI was estimated to be 12 kDa by SDS-PAGE and 12.32 kDa by MALDI TOF MS. Its N-terminal amino acid sequence was determined to be: PYGGGGTESG. HCB-SunI not only inhibited Helicoverpa cathepsin B (HCB) but also decreased the growth of HeLa and glioma cells by 7 ∼ 27% and 6 ∼ 22%, respectively, when the cells were grown in a final concentration of 0.002 ∼ 0.008 μM inhibitor.
Introduction
Protein proteinase inhibitors (PIs) are low molecular weight proteins that are commonly found in the organs and tissues of many plants and animals and many PIs have been isolated from animal or human tissues. PIs play important roles in regulating protease activity in physiological processes under normal or disease conditions. For example, the balance of matrix metalloproteinase-1 (MMP-1) and the endogenous tissue inhibitor of metalloproteinase-1 (TIMP-1) is important for maintaining the health of periodontal tissue [Citation1,Citation2]. Overexpression of some proteases may lead to the invasion and metastasis of tumor cells. Various types of proteinases are implicated in the malignant progression of human and animal tumors [Citation3]. Cathepsin B is especially known as a major protease that is involved in tumor invasion and may be a marker for malignancy in the more aggressive type of meningiomas. Cathepsin B is directly involved in glioma cell invasion and inhibitors of cysteine proteases reduce invasion of glioma cells in two in vitro models [Citation4]. Therefore, the development of new cathepsin inhibitors could lead to treatments that prevent tumor invasion and reduce the local spread of a tumor [Citation5].
A large amount of scientific evidence shows that eating fruits and vegetables lowers the risk of cancer. A potential mechanism for this is inhibition of proteasomal chymotrypsin-like activity that is associated with cancer cell apoptosis [Citation6]. Many protease inhibitors are candidates for developing treatments for tumor therapy. Some naturally occurring cysteine proteinase inhibitors were investigated for their inhibitory effects on tumors. A potato cysteine proteinase inhibitor (PCPI 8.7), a potent inhibitor of cathepsin L, inhibits invasion of murine melanoma cells by 21% at a concentration of 1 μg/ml, and reduced cathepsin B activity to 22% at a concentration of 10 μg/ml. Because the inhibitors of cathepsin B are generally more efficient at reducing invasion, cathepsin B is thought to play a more important role than cathepsin L in murine melanoma cell invasion [Citation3].
The sunflower, Helianthus annuus, is a major agricultural crop. Its seeds are widely used for the production of edible oil and food. Various protease inhibitors have been identified and purified from this plant. Two cysteine protease inhibitors (CPI) from sunflower seeds, named Sca (sunflower cystatin A), and Scb (sunflower cystatin B), were reported and characterized in terms of protein structure and inhibitory activity [Citation7,Citation8]. SFTI-1, a potent inhibitor of trypsin with a bicyclic 14 amino acid peptide was originally isolated from the seeds of the sunflower H. annuus. Its active site is homologous to the well-known family of serine protease inhibitors known as the Bowman-Birk trypsin inhibitors [Citation9]. Another report showed that hydrolysates of sunflower seed proteins contain the peptide Phe-Val-Asn-Pro-Gln-Ala-Gly-Ser, which is an inhibitor of angiotensin-I converting enzyme (ACE) and is a potential source of ACE inhibitory peptide. This peptide corresponds to a fragment of helianthinin, the 11S globulin from sunflower seeds, which is the main storage protein in sunflowers [Citation10]. These studies offer the prospect of using protease inhibitors from sunflower seeds for developing anti-tumor drugs.
Helicoverpa cathepsin B (HCB) is a cysteine proteinase purified from the eggs of Helicoverpa armigera and was identified as a cathepsin B-like protease by N-terminal amino acid sequence [Citation11,Citation12]. Its mature peptide is 63% identical to human cathepsin B. Our previous work established an efficient activity assay for HCB. Therefore, we used this proteinase as a molecular model to screen potential inhibitors of cathepsin B from sunflower seeds. We identified an inhibitor and studied its effects on glioma cells and HeLa cells. Glioma cells are known to express high levels of cathepsin B, which is a key factor for invasion and metastasis [Citation4]. This paper reports a new cathepsin B inhibitor from sunflower seeds.
Materials and methods
Fresh sunflower seeds, Helianthus annuus Linn., were purchased from the market in Jinan, China. Bovine hemoglobin (Hb) was purchased from the DongFeng Company (Shanghai, China). Bovine serum albumin (BSA) was from TaKaRa (Japan). Sephadex G-75 was purchased from ChangZheng FuMin (Shanghai, China). DEAE-Toyopearl was produced by TOSOH (Japan). Glioma cells and HeLa cells were purchased from the Institute of Zoology, Academy of China (Beijing). Standard protein was purchased from Amersham Biosciences Company (Buckinghamshire, England). MTT (Thiazoyl blue tetrazolium bromide) was produced by Amresco (United States). DMEM (high glucose) was purchased from Hyclone (Logan, United States). All other chemicals were analytical grade for biochemical research.
Purification of cathepsin B from H. armigera (HCB)
The cathepsin B was purified from the ovaries and fat bodies of H. armigera according to the method of Zhao et al. [Citation11]. Purified HCB was used in all experiments in this study.
Inhibitory activity assay of HCB-SunI
An HCB-BSA-degradation assay was used to detect inhibitory activity. 10 μl of sunflower seed extract or purified HCB-SunI (0.5 mg/ml in Buffer A, 10 mM Tris-HCl, 2 mM 2-mercaptoethanol and 1 mM EDTA, pH 7.5) was mixed with 10 μl purified HCB (0.5 mg/ml in buffer A) and adjusted to pH 3.6 by adding 2 μl of acetate buffer (1 M). After 15 min of incubation at room temperature, 20 μl BSA were added to the solution (1 mg/ml in acetate buffer, 0.1 M, pH 3.6), mixed and incubated at 37°C for 1 h. The degradation of BSA was examined by SDS-PAGE.
Purification of HCB-SunI
Husked fresh sunflower seeds, Helianthus annuus (100 g) were degreased with ether and homogenized in buffer A. The meal was then suspended in 400 ml buffer A, and stirred for 2 h at 4°C. After centrifugation at 10,000 rpm for 10 min, the supernatant was precipitated by adding ammonium sulfate to 50% saturation. The precipitate was dissolved in 30 ml buffer A and dialyzed against 10 volumes of the same buffer overnight at 4°C. After centrifugation at 10,000 rpm for 10 min at 4°C, the dialyzed sample was run on a Sephadex G-75 column (2.5 × 100 cm) that was equilibrated with buffer A. Fractions which inhibited HCB were collected and applied to a DEAE-Toyopearl column (1 × 30 cm, equilibrated with buffer A). The protein was eluted with a linear NaCl gradient from 0 to 0.5 M in 200 ml buffer A.
Protein quantification and sequencing
The amount of protein was determined by the Lowry method [Citation13]. Purified HCB-SunI was transferred to a PVDF membrane via an electric current and the N-terminal amino acid sequence was determined by Edman degradation on an Applied Biosystems model 491 protein sequencer.
Determination of molecular weight of HCB-SunI
The molecular weight of HCB-SunI was determined using the Laemmli method [Citation14] on a 15% gel and matrix-assisted laser desorption ionization time-of-flight mass spectrometry (MALDI TOF). The purified HCB-SunI was mixed with matrix (Sinapinic acid, 3-5-dimethoxy-4-hydroxycinnamic acid, 10 mg/ml in 30% acetonitrile/0.01% TFA) 1:4 and detected by Voyager™ Linear-DE/K, Perseptive Biosystems, Biospectrometry Workstation (Framington, MA, USA). The measurement condition, were 5 × 10− 6 mbar vacuum, 25 kV accelerating voltage and 2500 laser intensity.
Inhibitory activity assay of HCB-Sun in chromatography
Bovine hemoglobin (Hb, 1 g) was dissolved in 100 ml of 1 M acetate buffer (pH 3.6), and then dialyzed in 0.1 M acetate buffer (pH 3.6) overnight. After centrifugation, this solution was used as the substrate for HCB. 50 μl of the chromatography fractions of HCB-Sun, 1 were mixed with 10 μl HCB (0.5 mg/ml in buffer A), and 40 μl acetate buffer (0.1 M pH 3.6) was added to adjust the pH to 3.6. This inhibitor-enzyme mixture was incubated at 37°C for 15 min. Then 200 μl 1% Hb substrate was added, mixed and incubated for 1 h at 37°C. The reaction was stopped with 0.5 ml 5% TCA. The mixture was centrifuged at 10,000 rpm for 10 min, and 200 μl of the supernatant was neutralized with 50 μl 1N NaOH. The absorbance at 750 nm was measured by the Lowry method [Citation13]. The control was treated in the same manner but the inhibitor was replaced with the same volume of buffer A. One inhibitory unit is defined as 0.001 decrease in absorbance at A750 per min when HCB degrades Hb.
Effects of HCB-SunI on glioma cells and HeLa cells
The growth of glioma cells and HeLa cells was measured by the MTT (Thiazoyl blue tetrazolium bromide) method [Citation15]. Glioma cells and HeLa cells were cultured in DMEM medium with 10% calf serum (pH 7.5) at 37°C, with 5% CO2 and saturated humidity. Actively growing cells were collected by digestion with 0.25% trypsin. The cells were plated in a 96 well plate with 100 μl per well (104 cells/ml). After 24 h culture the medium was discarded and replaced with 90 μl fresh DMEM. 10 μl of various concentrations 0.02, 0.04 and 0.08 μM of HCB-SunI in buffer A was added to each well. For a control the cells were cultured in the same way, however, the inhibitor was replaced with the same volume of buffer A. A cell-free culture control treated in the same way but with no cells was used as a background control during the absorbance measurement. After 72 h of culture, 20 μl MTT (5 mg/ml in water) was added to each well and the mixture cultured for another 4 h. DMSO (100 μl) was added to resolve the violet precipitate and 10 min later the absorbance of the culture medium and cells was measured at 490 nm with a ELX800 Universal Microplate Reader (Bio-EK Instruments INC). Six wells were used for each treatment and the data were analyzed statistically.
Results
Identification and purification of HCB-SunI from sunflower seed
Using BSA as a substrate, inhibitory activity to HCB was detected in sunflower seed extracts. When BSA was incubated alone under acidic conditions, it was not degraded, which suggests that BSA is not degraded at this acidic pH. In contrast, BSA was degraded when it was incubated with HCB, which is due to the proteolytic activity of HCB. However, BSA degradation was obviously reduced when sunflower seed extract was added. This implied that there an inhibitor in the sunflower seed extract, which inhibited the degradation of BSA by HCB () and to confirm this, we purified the inhibitor from the sunflower seed extract.
Figure 1 SDS-PAGE showing the inhibition of sunflower seed extract on HCB activity. A, (1) 20 μl BSA incubated with 10 μl buffer A; (2) 20 μl BSA plus 10 μl HCB (0.5 mg/ml in buffer A); (3) 20 μl sunflower seed extract with 10 μl buffer A (0.5 mg/ml in buffer A); (4) 20 μl BSA plus 10 μl HCB saturated with 10 μl sunflower seed extract at pH 3.6 (0.5 mg/ml,). BSA (1 mg/ml) is in acetate buffer (0.1 M, pH 3.6). 12.5% gel. B, purity of HCB used for study.
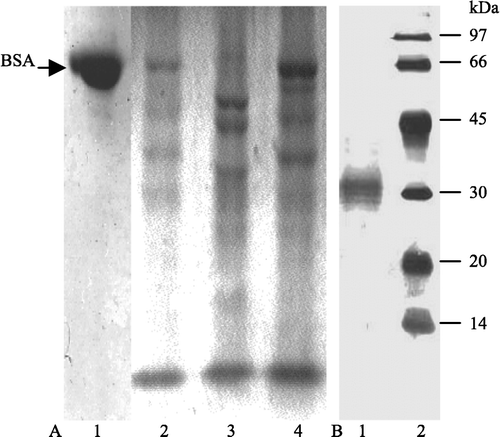
After Sephadex G-75 chromatography, the sunflower seed homogenate was primarily separated into two parts. Most of the proteins were in part I, but the major part of the inhibitory potency is in part II where there was much less protein than in part I ( A). The fractions with inhibitory activity (fractions 50–60) were collected and further separated by DEAE-Toyopearl chromatography. Two peaks with inhibitory activity were eluted with a linear sodium chloride gradient. The two peaks correlated with two protein peaks, which suggested that the proteins were effectively separated ( B). The later peak which eluted when the concentration of sodium chloride reached 0.3 M, exhibited obviously higher inhibitory activity compared with the other peak and therefore the fractions of the later peak, from fractions 65 to 75, were collected for analysis.
Figure 2 Chromatography. A. Sephadex G-75 column (2.5 × 100 cm). ‐‐•, Quantity of protein (absorbance measured at 750 nm); —○, Inhibitory activity, 4 ml per fraction with flow rate 0.3 ml/min. B. DEAE-Toyopearl column (1 × 30 cm), ‐‐•, Quantity of protein (absorbance measured at 750 nm); —○, Inhibitory activity, 2 ml per fraction with flow rate 0.2 ml/min. Italic line is the gradient of sodium chloride. The reletive inhibitory activity is calculated as: the absorbance in every fraction÷the lowest absorbance in the fractions × 100% (The zero is adjusted by the reaction solution of Hb and HCB without inhibitor).
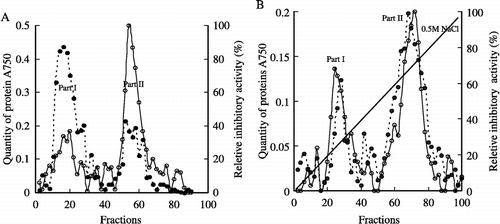
SDS-PAGE showed that the sunflower seed homogenate contained large amounts of various proteins. After Sephadex G-75 chromatography, most of the proteins were separated from the inhibitor, which indicated that the gel filtration with Sephadex G-75 efficiently separated the inhibitor. The few remaining contaminating proteins were effectively removed by further anion exchange chromatography with DEAE-Toyopearl. SDS-PAGE showed that after chromatography with DEAE-Toyopearl the inhibitor was purified from the original protein mixture. The molecular mass of the purified product was measured to be 12 kDa on a 15% SDS-PAGE gel and 12.32 kDa by MALDI TOF mass spectrometry ( A, B).
Figure 3 Analysis of the purity of HCB-SunI and determination of the molecular mass. A, 15% gel. (1) ammonium sulfate precipitation of the sunflower seed; (2) product after Sephadex G-75 chromatography; (3) final product after DEAE-Toyopearl column; (4) protein standard.
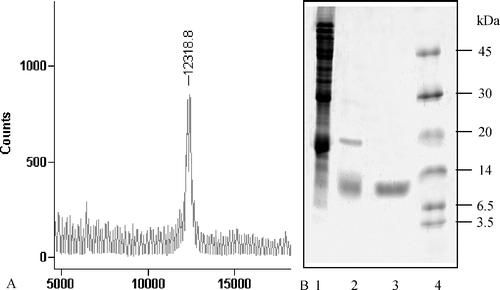
The yield of inhibitor was calculated (); from 100 g of sunflower seed, 200 mg of crude protein was isolated. After gel filtration with Sephadex G-75 only, 65 mg of protein remained which demonstrated how important this step was in removing contaminating proteins. After anion exchange chromatography with DEAE-Toyopearl, 6 mg of purified cathepsin B inhibitor was finally obtained. The inhibitory activity decreased from 68 to 8 units with the purification steps but the specific activity of the inhibitor increased from 0.39 to 1.33 units/mg. The purification yield was 12%.
Table I. Purification of HCB-SunI from sunflower seeds (from 100 g seeds).
Characterization of HCB-SunI
The N-terminal amino acid sequence of purified HCB-SunI was determined to be PYGGGGTESG by protein sequencing. A BLAST search for short and nearly exact matches from the www.ncbi.nlm.nih.gov site identified many matches but the first 4 were: an unknown protein from Oryza sativa (japonica cultivar-group), a glycoprotein from Cryptosporidium hominis, a RNA-binding protein precursor from Solanum tuberosum and an N-methylhydantoinase B/acetone carboxylase from Moorella thermoacetica.
The inhibitory activity of the purified HCB-SunI was further examined by HCB-BSA-degradation assay as described in the Methods. Results showed that BSA was actively degraded into fragments with the major band obviously decreasing in the absence of inhibitor. In contrast, the major band of BSA was left undegraded when the purified HCB-SunI inhibitor was added. This evidence indicates that the purified HCB-SunI from sunflower seeds inhibits HCB activity ().
Figure 4 SDS-PAGE showing the inhibition of the HCB-SunI on HCB. 12.5% gel. (1) 20 μl BSA incubated with 10 μl buffer A; (2) 20 μl BSA plus 10 μl HCB in buffer A; (3) 20 μl BSA plus 10 μl HCB that was saturated with 10 μl purified HCB-SunI at pH 3.6; (4) Protein marker. BSA is 1 mg/ml in acetate buffer (0.1 M, pH 3.6). HCB is 0.5 mg/ml in buffer A; HCB-SunI is 0.5 mg/ml in buffer A.
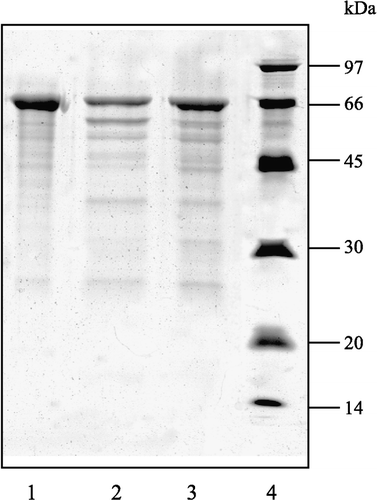
Effects of HCB-SunI on two tumor cell lines
To investigate the potential use of HCB-SunI as a therapy for tumor treatment, we examined the effects of this inhibitor on glioma cells and HeLa cells. Results show that HCB-SunI inhibited the growth of HeLa cells by 7.4, 15 and 27% at final concentrations of 0.002, 0.004 and 0.001 μM, respectively. Similarly, it inhibited the growth of glioma cells by 6.3, 14.3 and 22.2% at the same concentrations (). Statistical analysis shows that the differences between the control (Buffer A alone) and various concentrations of inhibitor treatments are significant (p < 0.05) except for 0.002μM inhibitor in glioma. These results demonstrate the potential for further research on this inhibitor for its development in tumor therapy.
Discussion
Currently the plant inhibitors (PIs) database contains information on 495 inhibitors (plus several isoinhibitors) identified from 129 different plants. They are divided into 10 families according to their primary structure, which includes Bowman-Birk serine protease inhibitors, cereal trypsin/alpha-amylase inhibitors, cysteine protease inhibitors, metallocarboxypeptidase inhibitors, mustard trypsin inhibitors, potato type I protease inhibitors, potato type II protease inhibitors, serpin, soybean trypsin inhibitors (Kunitz) and squash protease inhibitors. Every family contains hundreds of inhibitors. Many transgenic plants with PI genes have been produced, such as transgenetic tobacco, rice, potatos, apples, lettuce, rape seed, strawberries, sunflowers, sweet potatos and tomatoes containing cysteine protease inhibitors (http://bighost.area.ba.cnr.it/PLANT-PIs/).
Kouzuma et al [Citation7] reported the characteristics of two cysteine protease inhibitors (CPI) from sunflower seeds, named Sca (Sunflower Cystatin A, Protein data base Q10992) and Scb (Sunflower Cystatin B, Protein data base Q10993). The molecular weights (MW) of these two inhibitors were 9,330 and 11,187 Da, and they inhibited the activity of many cysteine proteases, such as pawpaw protease and fig protease, but only Scb inhibited the activity of cathepsin B. Another cysteine proteinase inhibitor, multicystatin (SMC), was cloned from sunflower seeds by Kouzuma et al. [Citation8]. The deduced amino acid sequence shared 65 ∼ 90% identical residues with Sca. In our work, the molecular weight of HCB-SunI is 12 kDa and the N-terminal amino acid sequence is different from either Scb, Sca or SMC. HCB-SunI is therefore likely to be a new protease inhibitor from sunflower seeds against cathepsin B. Although the N-terminal amino acid sequence is too short for exact identification of this inhibitor, a BLAST (Basic Local Alignment Search Tool) search for short and nearly exact matches showed that HCB-SunI matches an unknown protein from the rice, O. sativa (japonica cultivar-group). Another matched sequence is a glycoprotein from C. hominis, which is a parasite that is one of most common causes of water borne diseases in humans and a potential bioterrorist agent [Citation16]. Other matched sequences are a RNA-binding protein precursor from the potato, S. tuberosum and an N-methylhydantoinase B/acetone carboxylase of the bacteria M. thermoacetica. Final determination of the characteristics of HCB-SunI requires extended amino acid sequencing and molecular cloning.
Konduri et al [Citation17]. showed that cathepsin B activity and protein content levels were higher in glioblastoma cell lines than in anaplastic astrocytoma or low-grade glioma cell lines. Their experiments suggest that inhibition of cathepsin B activity may be useful in cancer therapy [Citation17]. Our preliminary research shows that HCB-SunI has inhibitory activity to HeLa and glioma cells. HCB-SunI decreased growth of the tumor cell lines by 7 ∼ 27% and 6 ∼ 22%, respectively, when used at final concentrations of 0.002 ∼ 0.008μM. Compared with glioma cells, fewer studies have been reported on the relationship between HeLa cells and cathepsin B. The effects of HCB-SunI on the growth of HeLa cells suggest that cathepsin B may also be involved in the proliferation of this tumor cell.
Cathepsin B has both C-terminal peptidyldipeptidase activity that cleaves C-terminal dipeptides sequentially from some polypeptides with broad specificity [Citation18] and endopeptidase activity [Citation19]. HCB was also reported active as an endopeptidase [Citation20]. The mechanism by which HCB-SunI inhibits HCB needs further investigation. During purification of HCB-SunI, peak I and peak II exhibited inhibitory activity to HCB. Peak I is probable another inhibitor because it has higher molecular weight than peak II.
Conclusion
HCB-SunI is a new protease inhibitor from sunflower seeds against cathepsin B. The molecular mass of HCB-SunI was estimated to be 12 kDa by SDS-PAGE and 12.32 Da by MALDI TOF MS. HCB-SunI has inhibitory activity on HeLa and glioma cells growth, which offered a model to further investigate the mechanism of tumor cells proliferation and growth. HCB-SunI was able to inhibit the cathepsin B-like proteinase (HCB) from the cotton boll worm, H. armigera. If this inhibitor can be a possible candidate for developing insect resistance, genetically manipulated crops needs further study.
Acknowledgement
This work was supported by National Natural Science Foundation of China (No: 30330070) and the Natural Science Foundation of Shandong Province (No. Z2003D04). The authors thank Dr. Roberta Greenwood for her editing.
References
- Sloane BF, Moin K, Krepela E. Cathepsin B and its endogenous inhibitors: the role in tumor malignancy. Cancer Metastasis. Rev. 1990; 9: 333–352
- Domeij H, Modeer T, Yucel-Lindberg T. Matrix metalloproteinase-1 and tissue inhibitor of metalloproteinase-1 production in human gingival fibroblasts: the role of protein kinase C. J. Periodontal. Res. 2004; 39: 308–314
- Sever N, Filipic M, Brzin J, Lah TT. Effect of cysteine proteinase inhibitors on murine B16 melanoma cell invasion in vitro. Biol. Chem. 2002; 383: 839–842
- Demchik LL, Sameni M, Nelson K, Mikkelsen T, Sloane BF. Cathepsin B and glioma invasion. Int. J. Dev. Neurosci. 1999; 17: 483–494
- Levicar N, Nuttall RK, Lah TT, Nutall RK. Proteases in brain tumor progression. Acta. Neurochir 2003; 145: 825–838, (Wien)
- Chen MS, Chen D, Dou QP. Inhibition of proteasome activity by various fruits and vegetables is associated with cancer cell death. In Vivo. 2004; 18: 73–80
- Kouzuma Y, Kawano K, Kimura M. Purification, characterization and sequencing of two cysteine proteinase inhibitors, Scb and Sca, from sunflower (Helianthus annuus) seeds. J. Biochem. (Tokyo) 1996; 119: 1106–1113
- Kouzuma Y, Inanaga H, Doi-Kawano K, Yamasaki N, Kimura M. Molecular cloning and functional expression of cDNA encoding the cysteine proteinase inhibitor with three cystatin domains from sunflower seeds. J Biochem (Tokyo) 2000; 128: 161–166
- Korsinczky ML, Schirra HJ, Craik DJ. Sunflower trypsin inhibitor-1. Curr. Protein. Pept. Sci. 2004; 5: 351–364
- Megi'as C, Yust, der MM, Pedroche J, Lquari H, Giroan-Calle J, Alaiz M, Millaan F, Vioque J. Purification of an ACE inhibitory peptide after hydrolysis of sunflower (Helianthus annuus L.) protein isolates. J. Agric. Food. Chem 2004; 52: 1928–1932
- Zhao X-F, Wang J-X, Wang Y-C. Purification and characterization of a cysteine proteinase from eggs of the cotton boll worm. Helicoverpa armigera. Insect Biochem. Molec. Biol. 1998; 28: 259–264
- Zhao X-F, Wang J-X, Xu X-L, Schmid R, Wieczorek H. Molecular cloning and characterization of the cathepsin B-like proteinase from the cotton boll worm. Helicoverpa armigera. Insect Molec. Biol. 2002; 11: 567–575
- Lowry OH, Rosebrough Al, Farr Al, Randal RJ. Protein measurement with the Folin-phenol reagent. J Biochem. 1951; 193: 265–275
- Laemmli UK. Cleavage of structural proteins during the assembly of the head of bacteriophage T4. Nature. 1970; 227: 265–275
- Denizot F, Lang R. Rapid colorimetric assay for cell growth and survival. J. Immunol. Meth. 1986; 89: 271–277
- Xu P, Widmer G, Wang Y, Ozaki LS, Alves JM, Serrano MG, Puiu D, Manque P, Akiyoshi D, Mackey AJ, Pearson WR, Dear PH, Bankier AT, Peterson DL, Abrahamsen MS, Kapur V, Tzipori S, Buck GA. The genome of Cryptosporidium hominis. Nature 2004; 431: 1107–1112
- Konduri S, Lakka SS, Tasiou A, Yanamandra N, Gondi CS, Dinh DH, Olivero WC, Gujrati M, Rao JS. Elevated levels of cathepsin B in human glioblastoma cell lines. Int. J. Oncol. 2001; 19: 519–524
- Barrett AJ, Kirschke H, Cathepsin B, Cathepsin H, cathepsin L. Meth. Enzymol 1981; 80: 535–561
- Portaro FC, Santos AB, Cezari MH, Juliano MA, Juliano L, Carmona E. Probing the specificity of cysteine proteinases at subsites remote from the active site: analysis of P4, P3, P2′ and P3′ variations in extended substrates. Biochem. J 2000; 347: 123–129
- Zhao X-F, Wang J-X, Li F-X, Sueda S, Kondo H. Analysis of Substrate Specificity and Endopeptidyl Activities of the Cathepsin B-like Proteinase from Helicoverpa armigera. Protein J. 2005; 24, in press