Abstract
Protein tyrosine phosphatase 1B (PTP1B) is considered as a therapeutic target for the treatment of diabetes and obesity. In our preliminary screening study, a MeOH extract of the aerial part of Siegesbeckia glabrescens was found to inhibit PTP1B activity at 30 μg/mL. Bioassay‐guided fractionation led to the isolation of two active diterpenes, ent-16βH,17-isobutyryloxy-kauran-19-oic acid (1) and ent-16βH,17-acetoxy-18-isobutyryloxy-kauran-19-oic acid (2), along with ent-16βH,17-hydroxy-kauran-19-oic acid (3). Compounds 1 and 2 inhibited the PTP1B activity with IC50 values of 8.7 ± 0.9 and 30.6 ± 2.1 μM, respectively. Kinetic studies suggest that both 1 and 2 are non-competitive inhibitors of PTP1B. However, compound 3 substituted with a hydroxyl group at C-17 in kaurane-type showed no inhibitory effects towards PTP1B.
Introduction
Binding of insulin to the extracellular α-subunit of the insulin receptor (IR) triggers a conformational change that activates the intrinsic tyrosine kinase activity of the β-subunit via autophosphorylation of specific tyrosine residues. This results in the phosphorylation of IR substrates (IRS) 1–4, which then activates several signaling cascades that give a biological response, such as glucose transport into the cell and glycogen synthesis [Citation1,Citation2]. Protein tyrosine phosphatases (PTPs) are responsible for the dephosphorylation of tyrosine residues, and are considered as negative regulators of insulin signaling. Although several PTPs such as PTP-α, leukocyte antigen‐related tyrosine phosphatase (LAR), SH2-domain‐containing phosphotyrosine phosphatase (SHP2) have been implicated in the regulation of insulin signaling, there is substantial evidence supporting protein tyrosine phosphatase 1B (PTP1B) as the critical PTP controlling insulin signaling pathway [Citation1,Citation2]. The overexpression of PTP1B has been shown to inhibit the IR signaling cascade and occurs in insulin‐resistant states [Citation3,Citation4]. Furthermore, recent genetic evidence has demonstrated that PTP1B gene variants are associated with changes in insulin sensitivity [Citation5,Citation6]. As with the insulin signaling pathway, the leptin signaling pathway can be attenuated by PTPs and there is compelling evidence that PTP1B is also involved in this process [Citation1,Citation2,Citation7]. Therefore, it has been suggested that compounds that reduce PTP1B activity or expression levels could not only be used for treating type 2 diabetes but also obesity [Citation1,Citation2]. Although there have been a number of reports on the design and development of synthetic PTP1B inhibitors [Citation1,Citation8], only a few studies have been reported on PTP1B inhibitors derived from plants [Citation9,Citation10].
In our preliminary screening study, a methanol extract of the aerial part of Siegesbeckia glabrescens (Compositae) was found to inhibit PTP1B activity at 30 μg/ml [Citation11]. The genus Siegesbeckia has been used as a traditional medicine, ‘Hi-Chum’ in Korea (common name: Siegesbeckia herb), to treat inflammatory diseases [Citation12]. Previous phytochemical investigations on this genus have resulted in the isolation of sesquiterpenes and diterpenes [Citation13,Citation15]. The aqueous extract of S. glabrescens has been reported to reduce the mast cell-mediated anaphylactic reaction and inhibit the immunoglobulin E (IgE) production [Citation16,Citation17]. Since there has been no study with regard to its inhibitory effect on PTP1B, we searched for the PTP1B-inhibitory compounds from this plant. Bioassay-guided fractionation of the methanol extract resulted in the isolation of two active diterpenes, ent-16βH,17-isobutyryloxy-kauran-19-oic acid (1) and ent-16βH,17-acetoxy-18-isobutyryloxy-kauran-19-oic acid (2), along with ent-16βH,17-hydroxy-kauran-19-oic acid (3) (). Although these diterpenes have been isolated from some other species, they have not previously been reported in this plant.
Materials and methods
Plant material
The aerial parts of S. glabrescens was purchased from Il-sin Pharmaceutical Co., Ltd. in Daejeon, Korea. A voucher specimen (KRIBB No. 1144) has been deposited in the authors' laboratory.
Extraction and isolation
The dried aerial parts of S. glabrescens (2 kg) was extracted with MeOH (10 L × 3) for 6 h at room temperature. The dried MeOH extract (680 g) was suspended in water and partitioned with hexane, EtOAc, and BuOH, sequentially. Since the hexane‐soluble fraction exhibited the strongest activity (80% inhibition at 30 μg/mL), a portion of this fraction (1.45 g) was further separated by silica gel column chromatography (5.5 × 45 cm, 0.063–0.200 mm particle size) eluting with a stepwise gradient of hexane-EtOAc (from 100: 0 to 0: 100), to afford 22 subfractions (Fr.1 – Fr. 22). Of the subfractions that showed PTP1B inhibitory activity, Fr. 9 (eluted with 85% hexane in EtOAc, 63.4 mg) was further purified by preparative reverse phase HPLC using a gradient from 80% – 100% AcCN in 0.1% formic acid (aq.) over 40 min [RS tech Optima Pak C18 column (Korea); 250 × 10 mm; 10 μm particle size; 2 mL/min; UV detection at 210 nm], to yield compound 1 (9.3 mg, tR 29.5 min). The other active subfraction, Fr. 15 (eluted with 70% hexane in EtOAc, 68.4 mg), was also subjected to preparative reverse phase HPLC on Shiseido Capcell Pak C18 column (250 × 10 mm; 5 μm particle size; Japan), eluted with a gradient from 80%–92% AcCN in 0.1% formic acid (aq.) over 30 min (2 mL/min; UV detection at 210 nm), to afford compounds 2 (9.7 mg, tR 21.3 min) and 3 (11.8 mg, tR 10.3 min).
Ent-16βH,17-isobutyryloxy-kauran-19-oic acid (1)
white amorphous powder; ESI-MS m/z: 389 [M − H]+; 1H-NMR (600 MHz, pyridine-d5) δ: 4.01 (2H, br d, J = 8.4 Hz, H-17), 2.61 (1H, sept, J = 6.6 Hz, H-2′), 2.50 (1H, d, J = 12.6 Hz, H-3), 2.14 (1H, m, H-16), 2.06 (1H, br s, H-13), 1.88 (2H, d, J = 12.0 Hz, H-1 and H-14), 1.37 (3H, s, H-18), 1.19 (6H, d, J = 6.6 Hz, H-3′ and H-4′), 1.16 (3H, s, H-20), 1.08 (1H, m, H-3), 1.05 (1H, br d, J = 11.4 Hz, H-14), 0.84 (1H, m, H-1); 13C-NMR (150 MHz, pyridine-d5) δ: 41.59 (C-1), 20.34 (C-2), 39.18 (C-3), 44.36 (C-4), 57.47 (C-5), 23.64 (C-6), 42.34 (C-7), 45.60 (C-8), 55.88 (C-9), 40.39 (C-10), 19.42 (C-11), 32.01 (C-12), 39.42 (C-13), 37.84 (C-14), 45.74 (C-15), 40.48 (C-16), 68.98 (C-17), 29.86 (C-18), 180.52 (C-19), 16.45 (C-20), 177.24 (C-1′), 34.73 (C-2′), 19.65 (C-3′), 19.64 (C-4′).
Ent-16βH,17-acetoxy-18-isobutyryloxy-kauran-19-oic acid (2)
white amorphous powder; ESI-MS m/z: 447 [M − H]+, 471 [M+Na]+; 1H-NMR (300 MHz, CDCl3) δ: 4.40 (1H, d, J = 10.5 Hz, H-18a), 3.97 (1H, d, J = 10.5 Hz, H-18b), 3.84 (2H, d, J = 7.2 Hz, H-17), 2.53 (1H, sept, J = 7.2 Hz, H-2′), 2.05 (3H, s, OCOCH3), 1.15 (6H, d, J = 7.2 Hz, H-3′ and H-4′), 0.96 (3H, s, H-20); 13C-NMR (75 MHz, CDCl3) δ: 40.32 (C-1), 18.94 (C-2), 32.53 (C-3), 47.64 (C-4), 52.46 (C-5), 22.54 (C-6), 41.26 (C-7), 44.82 (C-8), 55.51 (C-9), 39.63 (C-10), 18.51 (C-11), 31.39 (C-12), 38.70 (C-13), 37.34 (C-14), 45.03 (C-15), 39.76 (C-16), 68.50 (C-17), 72.38 (C-18), 180.70 (C-19), 15.64 (C-20), 177.61 (C-1′), 34.30 (C-2′), 19.23 (C-3′), 19.23 (C-4′), 170.90 (OCOCH3), 21.01 (OCOCH3).
Ent-16βH,17-hydroxy-kauran-19-oic acid (3)
white amorphous powder; ESI-MS m/z: 319 [M − H]+; 1H-NMR (400 MHz, pyridine-d5) δ: 3.64 (2H, m, H-17), 2.50 (1H, br d, J = 12.6 Hz, H-3), 2.35 (2H, m, H-2), 2.21 (2H, m, H-5 and H-16), 1.92 (1H, m, H-1), 1.37 (3H, s, H-18), 1.18 (3H, s, H-20), 0.75 (1H, td, J = 12.4, 4.8 Hz, H-1); 13C-NMR (100 MHz, pyridine-d5) δ: 42.16 (C-1), 19.90 (C-2), 37.43 (C-3), 45.07 (C-4), 57.10 (C-5), 23.25 (C-6), 41.20 (C-7), 43.90 (C-8), 55.68 (C-9), 39.97 (C-10), 19.23 (C-11), 31.92 (C-12), 38.74 (C-13), 38.76 (C-14), 45.80 (C-15), 44.23 (C-16), 67.03 (C-17), 29.41 (C-18), 180.19 (C-19), 16.05 (C-20).
PTP1B assay
PTP1B (human, recombinant) was purchased from BIOMOL® International LP (USA). The enzyme activity was measured using p‐nitrophenylphosphate (pNPP) as described previously [Citation18]. To each 96 well (final volume: 200 μl) was added 2 mM pNPP and PTP1B (0.05(0.1 μg) in a buffer containing 50 mM citrate (pH 6.0), 0.1 M NaCl, 1 mM EDTA and 1 mM dithiothreitol (DTT) with or without test compounds. Following incubation at 37°C for 30 min, the reaction was terminated with 10 M NaOH. The amount of p‐nitro phenol produced was estimated by measuring the absorbance at 405 nm. The non-enzymatic hydrolysis of 2 mM pNPP was corrected by measuring the increase in absorbance at 405 nm obtained in the absence of PTP1B enzyme.
Dual-specificity protein tyrosine phosphatase (DS-PTP) assay
DS-PTP was assayed with VH1-related human protein (VHR) expressed in Escherichia coli. The reaction mixture containing VHR enzyme, 2 mM pNPP and assay buffer (50 mM succinate, 1 mM EDTA, 140 mM NaCl, 0.05% Tween 20, pH 6.0) was incubated at 37°C for 30 min. The reaction was terminated by the addition of 1 M NaOH, and the dephosphorylation activity measured at 405 nm [Citation18,Citation19].
Protein serine/threonine phosphatase 1 (PP1) assay
The PP1 (Sigma Chemical Co., St. Louis, MO, USA) activity was measured at 37°C using pNPP as a substrate. Reactions were performed for 30 min in the assay buffer (50 mM Tris-HCl, 0.1% β-mercaptoethanol, 1 mM EDTA, 1 mM MnCl2, 20 mM MgCl2, pH 7.6). The reaction was stopped by the addition of 1 M NaOH, and the amount of p-nitro phenol was measured by absorbance at 405 nm [Citation18,Citation19].
Inhibition kinetics
In the kinetic analysis, the reaction mixture consisting of four or five different concentrations of pNPP (0.5, 1.0, 2.0, 4.0, 8.0 and 16.0 mM) used as a PTP1B substrate in the absence or presence of compounds 1 and 2. The Michaelis-Menten constant (Km) and maximum velocity (Vmax) of PTP1B were determined by Eadie-Hofstee plots using a GraphPad Prism® 4 program (GraphPad Software Inc., USA).
Results and discussion
Recent evidence suggests a key role for PTPs in the control of insulin signaling and metabolism [Citation1,Citation2]. Of the PTPs, PTP1B appears to be an attractive therapeutic target because the PTP1B levels in muscles and adipose tissues correlate with the degree of insulin resistance in subjects with diabetes and obesity [Citation1,Citation2]. Furthermore, recent genetic evidence has supported the view that PTP1B inhibitors could be potential agents for the treatment of type 2 diabetes and obesity [Citation5,Citation7].
As part of our ongoing study to search for natural PTP1B inhibitors from plants, the aerial part of S. glabrescens were chosen for detailed investigation, since the methanol extract was found to inhibit PTP1B at 30 μg/mL [Citation11]. Bioassay-guided fractionation on the MeOH extract led to the isolation of three diterpenes 1, 2 and 3 (). The structures of the isolated compounds were identified as ent-16βH,17-isobutyryloxy-kauran-19-oic acid (1), ent-16βH,17-acetoxy-18-isobutyryloxy-kauran-19-oic acid (2) and ent-16βH,17-hydroxy-kauran-19-oic acid (3) by analyses of MS and NMR data, and comparison with those reported in the literature Citation14Citation20-23. Although compound 1 was previously reported by Murakami et al. for the first time [Citation20], only the chemical structure of its alkaline hydrolysate was confirmed by the analysis of 1H-NMR data. In this study, the structure was more precisely elucidated by 13C-NMR, DEPT and 2D-NMR as indicated in Materials and Methods.
The three isolates were tested for their inhibitory activity against PTP1B, and the results are shown in . Of the isolates, ent-16βH,17-isobutyryloxy-kauran-19-oic acid (1) which has an isobutyryloxy moiety at C-17 of the kaurane-type structure exhibited the strongest inhibitory activity (IC50 = 8.7 ± 0.9 μM). However, compound 3 (IC50 > 200 μM) with a hydrolyzed isobutyryloxy group at C-17 of 1 exhibited significantly lower activity than 1. This suggests that an isobutyryloxy moiety at C-17 of the kaurane diterpene is essential for activity. Compound 2 substituted with an acetoxy and an isobutyryloxy group at C-17 and C-18, respectively, inhibited PTP1B activity in a dose-dependent manner with an IC50 value of 30.6 ± 2.1 μM, which was less effective than 1. A known phosphatase inhibitor, RK-682 (IC50 = 3.5 ± 0.4 μM) [Citation24], was used as a positive control in this assay. In addition, the isolates were assayed for their inhibitory effects on other types of protein phosphatases, and it was shown that the compounds had no inhibitory effects toward VHR and PP1 at levels up to 200 μM. To elucidate the inhibition mode of 1 and 2 on the activity of PTP1B, kinetic analyses were performed with different concentrations of substrate. As shown in , the mechanisms of inhibition by the two compounds were determined using an Eadie-Hofstee plot. When pNPP was used as substrate, both 1 and 2 decreased the Vmax values, but did not alter the Km values of PTP1B (). Accordingly, 1 and 2 were found to be non-competitive inhibitors with Ki values of 9.1 and 31.8 μM, respectively. This indicates that they may bind to the enzyme-substrate complex or interact with an allosteric site distinct from the active site of the enzyme [Citation25]. Kaurane diterpenes have been reported to inhibit TNF-α secretion in trypsin-stimulated HMC-1 cells [Citation22], to reduce nitric oxide and prostaglandin E2 production in LPS-induced RAW264.7 cells [Citation26], and to induce caspase-dependent apoptotic or necrotic cell death [Citation27]. Besides, this type diterpenes have been described to have anti-HIV, anti-platelet aggregation and cytotoxic activities Citation28-30. However, to our knowledge, PTP1B inhibitory activity of these diterpenes is being reported here for the first time in this study.
Table I. Comparison of the inhibitory activity of compounds 1–3 isolated from S. glabrescens against PTP1B, VHR and PP1.
Figure 2 Inhibition kinetics of compounds 1 and 2. Panel a shows an Eadie-Hofstee plot of the inhibitory effect of compound 1 on PTP1B-catalyzed hydrolysis of pNPP. Data are expressed as mean initial velocity for n = 3 replicates at each substrate concentration. Symbols: (*) 0 μM, (▪) 3 μM, (▾) 7 μM, (▪) 15 μM, (•) 30 μM compound 1. Panel b shows an Eadie-Hofstee plot of the inhibitory effect of compound 2 on PTP1B. Data are expressed as mean initial velocity for n = 3 replicates at each substrate concentration. Symbols: (*) 0 μM, (▴) 12 μM, (○) 21 μM, (♦) 35 μM, (▽) 60 μM compound 2.
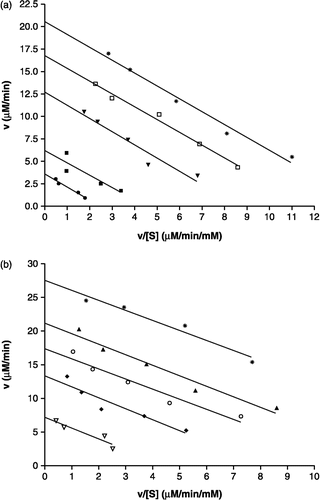
In conclusion, bioassay-guided investigation of the methanol extract of the aerial part of S. glabrescens afforded two active kaurane diterpenes, ent-16βH, 17-isobutyryloxy-kauran-19-oic acid (1) and ent-16βH,17-acetoxy-18-isobutyryloxy-kauran-19-oic acid (2), along with ent-16βH,17-hydroxy-kauran-19-oic acid (3). The present study indicates that the isolates 1 and 2 are non-competitive inhibitors of PTP1B. Because PTP1B is an intracellular enzyme, a further study to confirm their cellular effects is under progress.
Acknowledgements
This research was supported in part by the grants from the Plant Diversity Research Center of 21st Frontier Research Program (PF0320903-00), the Molecular and Cellular BioDiscovery Research Program (M1-0311-00-0023) of the Ministry of Science and Technology of Korea, and from KRIBB Research Initiative Program.
References
- Johnson TO, Ermolieff J, Jirousek MR. Protein tyrosine phosphatase 1B inhibitors for diabetes. Nat Rev Drug Discov 2002; 1: 696–709
- Asante-Appiah E, Kennedy BP. Protein tyrosine phosphatases: The quest for negative regulators of insulin action. Am J Physiol Endocrinol Metab 2003; 284: E663–E670
- Ahmad F, Azevedo JJ, Cortright R, Dohm G, Goldstein B. Alterations in skeletal muscle protein-tyrosine phosphatase activity and expression in insulin-resistant human obesity and diabetes. J Clin Invest 1997; 100: 449–458
- Byon JCH, Kusari AB, Kusari J. Protein-tyrosine phosphatase-1B acts as a negative regulator of insulin signal transduction. Mol Cell Biochem 1998; 182: 101–108
- Elchebly M, Payette P, Michaliszyn E, Cromlish W, Collins S, Loy AL, Normandin D, Cheng A, Himms-Hagen J, Chan CC, Ramachandran C, Gresser MJ, Tremblay ML, Kennedy BP. Increased insulin sensitivity and obesity resistance in mice lacking the protein tyrosine phosphatase-1B gene. Science 1999; 283: 1544–1548
- Ukkola O, Santaniemi M. Protein tyrosine phosphatase 1B: A new target for the treatment of obesity and associated co-morbidities. J Intern Med 2002; 251: 467–475
- Cook WS, Unger RH. Protein tyrosine phosphatase 1B: A potential leptin resistance factor of obesity. Dev Cell 2002; 2: 385–387
- Taylor SD, Hill B. Recent advances in protein tyrosine phosphatase 1B inhibitors. Expert Opin Invest Drugs 2004; 13: 199–214
- Chen RM, Hu LH, An TY, Li J, Shen Q. Natural PTP1B inhibitors from Broussonetia papyrifera. Bioorg Med Chem Lett 2002; 12: 3387–3390
- Kim YC, Oh H, Kim BS, Kang TH, Ko EK, Han YM, Kim BY, Ahn JS. In vitro protein tyrosine phosphatase 1B inhibitory phenols from the seeds of Psoralea corylifolia. Planta Med 2005; 71: 87–89
- Hong JH, Lee MS, Bae EY, Kim YH, Oh H, Oh WK, Kim BY, Ahn JS. Screening of the inhibitory activity of medicinal plants against protein tyrosine phosphatase 1B. Kor J Pharmacogn 2004; 35: 16–21
- Bae K. The medicinal plants of Korea. Seoul: Kyo-Hak Publishing Co.; 2000. p 512.
- Xiang Y, Fan C-Q, Yue J-M. Novel sesquiterpenoids from Siegesbeckia orientalis. Helv Chim Acta 2005; 88: 160–170
- Jiang X, Yunbao M, Yunlong X. Diterpenoids from Siegesbeckia pubescens. Phytochemistry 1992; 31: 917–921
- Xiang Y, Zhang H, Fan C-Q, Yue J-M. Novel diterpenoids and diterpenoid glycosides from Siegesbeckia orientalis. J Nat Prod 2004; 67: 1517–1521
- Kang BK, Lee EH, Kim HM. Inhibitory effects of Korean folk medicine ‘Hi-Chum’ on histamine release from mast cells in vivo and vitro. J Ethnopharmacol 1997; 57: 73–79
- Kim HM, Lee JH, Won JH, Park EJ, Chae HJ, Kim HR, Kim CH, Baek SH. Inhibitory effect on immunoglobulin E production in vivo and in vitro by Siegesbeckia glabrescens. Phytother Res 2001; 15: 572–576
- Oh H, Kim BS, Bae EY, Kim MS, Kim BY, Lee HB, Kim CJ, Ahn JS. Inhibition of PTP1B by metabolites from Micromucor ramannianus var. angulisporus CRM000232. J Antibiot 2004; 57: 528–531
- Lee MS, Oh WK, Kim BY, Ahn SC, Kang DO, Sohn CB, Osada H, Ahn JS. Inhibition of VHR dual-specificity protein tyrosine phosphatase activity by flavonoids isolated from Scutellaria baicalensis: Structure-activity relationships. Planta Med 2002; 68: 1063–1065
- Murakami T, Isa T, Satake T. Eine neuuntersuchung der inhaltsstoffe von Siegesbeckia pubescens Makino. Tetrahedron Lett 1973; 50: 4991–4994
- Guo DA, Zhang ZG, Ye GQ, Lou ZC. Studies on liposoluble constituents from the aerial parts of Siegesbeckia orientalis L. Acta Pharm Sin 1997; 32: 282–285
- Cai XF, Shen G, Dat NT, Kang OH, Lee YM, Lee JJ, Kim YH. Inhibitory effect of kaurane type diterpenoids from Acanthopanax koreanum on TNF-α secretion from trypsin-stimulated HMC-1 cells. Arch Pharm Res 2003; 26: 731–734
- Etse JT, Gray AI, Waterman PG. Chemistry in the Annonaceae, XXIV. Kaurane and kaur-16-ene diterpenes from the stem bark of Annona reticulata. J Nat Prod 1987; 50: 979–983
- Hamaguchi T, Sudo T, Osada H. RK-682, a potent inhibitor of tyrosine phosphatase, arrested the mammalian cell cycle progression at G1phase. FEBS Lett 1995; 372: 54–58
- Wiesmann C, Barr KJ, Kung J, Zhu J, Erlanson DA, Shen W, Fahr BJ, Zhong M, Taylor L, Randal M, McDowell RS, Hansen SK. Allosteric inhibition of protein tyrosine phosphatase 1B. Nat Struc Mol Biol 2004; 11: 730–737
- Hwang BY, Lee JH, Koo TH, Kim HS, Hong YS, Ro JS, Lee KS, Lee JJ. Kaurane diterpenes from Isodon japonicus inhibit nitric oxide and prostaglandin E2 production and NF-B activation in LPS-stimulated macrophage RAW264.7 cells. Planta Med 2001; 67: 406–410
- Suzuki I, Kondoh M, Nagashima F, Fujii M, Asakawa Y, Watanabe Y. A comparison of apoptosis and necrosis induced by ent-kaurane-type diterpenoids in HL-60 cells. Planta Med 2004; 70: 401–406
- Wu Y-C, Hung Y-C, Chang F-R, Cosentino M, Wang H-K, Lee K-H. Identification of ent-16,17-dihydroxykauran-19-oic acid as an anti-HIV principle and isolation of the new diterpenoids annosquamosins A and B from Annona squamosa. J Nat Prod 1996; 59: 635–637
- Yang Y-L, Chang F-R, Wu C-C, Wang W-Y, Wu Y-C. New ent-kaurane diterpenoids with anti-platelet aggregation activity from Annona squamosa. J Nat Prod 2002; 65: 1462–1467
- Xiang W, Na Z, Li S-H, Li M-L, Li R-T, Tian Q-E, Sun H-D. Cytotoxic diterpenoids from Isodon enanderianus. Planta Med 2003; 69: 1031–1035