Abstract
A new series of bis-triazole 19a-l was synthesised for the purpose of being hybrid molecules with both anti-inflammatory and anti-cancer activities and assessed for cell cycle arrest, NO release. Compounds 19c, 19f, 19h, 19 l exhibited COX-2 selectivity indexes in the range of 18.48 to 49.38 compared to celecoxib S.I. = 21.10), inhibit MCF-7 with IC50 = 9–16 μM compared to tamoxifen (IC50 = 27.9 μM). and showed good inhibitory activity against HEP-3B with IC50 = 4.5–14 μM compared to sorafenib (IC50 = 3.5 μM) (HEP-3B). Moreover, derivatives 19e, 19j, 19k, 19 l inhibit HCT-116 with IC50 = 5.3–13.7 μM compared to 5-FU with IC50 = 4.8 μM (HCT-116). Compounds 19c, 19f, 19h, 19 l showed excellent inhibitory activity against A549 with IC50 = 3–4.5 μM compared to 5-FU with IC50 = 6 μM (A549). Compounds 19c, 19f, 19h, 19 l inhibit aromatase (IC50 of 22.40, 23.20, 22.70, 30.30 μM), EGFR (IC50 of 0.112, 0.205, 0.169 and 0.066 μM) and B-RAFV600E (IC50 of 0.09, 0.06, 0.07 and 0.05 μM).
Introduction
Breast cancer (BC) represents the highest incidence rate responsible for mortality and morbidity in women all over the world especially metastatic breast cancerCitation1. About 80% of all breast cancers are oestrogen dependent “ER-positive” that means cancer cells grow in response to the hormone estrogenCitation2. The therapeutic strategies designed for treatment of breast cancer include the use of drugs which either act as antiestrogens, that block oestrogen receptors and preventing oestrogens from binding to their active site and performing their action, these are known as competitive inhibitors as tamoxifen (1), a selective oestrogen receptor modulator (SERM)Citation3,Citation4.
Another principle of endocrine therapy for treatment of breast cancer is the use of aromatase inhibitors (AIs), which inhibit the conversion of androgens such as androstenedione (2) to oestrogens by inhibition of aromatase activityCitation5. Aromatase is a member of the cytochrome P450 enzyme that possesses an iron-porphyrin group (Fe2+-containing heme) at its active siteCitation6. Aromatase inhibitors therefore constitute a frontline therapy for oestrogen-dependent breast cancer that are sub-classified into steroidal aromatase inhibitors which decrease the oestrogen biosynthesis by competing with the substrate androstenedione for binding to the active site of the enzymeCitation7. Exemestane (3) is a hormone therapy drug used to treat breast cancer in women who have gone through menopause and is the most representative member of this class, with an irreversible mechanism of inhibitionCitation8. Non-steroidal aromatase inhibitors like letrozole (4) and anastrozole (5) act by a reversible mechanism of inhibition by formation of coordinating bond with heme-iron in the catalytic site of aromataseCitation9. Nonsteroidal aromatase inhibitors have two primary elements in their structure, azole part containing nitrogen atom that interacts with heme-iron atom of aromatase, while the bulky aryl part resembles the substrate’s steroid ringCitation10. These triazole derivatives are very potent, specific and selective aromatase inhibitors that allow almost complete oestrogen suppressionCitation11,Citation12. However, unwanted severe side effects due to complete inhibition of oestrogen synthesis by their prolonged use like increased risk of heart disease and osteoporosis restrict their domain of clinical applicationCitation13, leading to continue exploration of new strategies for treatment.
Receptor tyrosine kinase (RTK) or HER receptor family (also called ErbB or epidermal growth factor receptor EGFR family) consists of four structurally close receptor: EGFR (HER1/ErbB1), HER2 (ErbB2), HER3 (ErbB3), and HER4 (ErbB4)Citation14. Their structure shows three domains responsible for their activity and subsequent phosphorylation of the key tyrosine residues namely, an extracellular ligand binding domain, a transmembrane domain and an intracellular tyrosine kinase domainCitation15–19.
HER2 (human epidermal growth factor receptor 2) participates in 20% of breast cancer cases called HER2-positive breast cancer, is unique within the EGFR family in that it does not have known ligands and cannot form ligand-dependent homodimersCitation20. Thus, to initiate downstream signalling, it must form heterodimers with other EGFR proteins once their specific ligands have boundCitation19. HER dimerisation inhibitors are one of chemotherapeutic management that blocks the ability of HER2 receptor to bind to other EGFR receptor family membersCitation21. Another chemotherapeutic tool in treatment of breast cancer is the utilisation of EGFR tyrosine kinase inhibitorsCitation22. Many compounds possessing sulphonamide moiety have been reported as highly effective kinase inhibitors such as vemurafenib (6), the first kinase inhibitor for treatment of late-stage melanoma by inhibition of EGFR signalling through selectively blocking mutant B-RAFV600E associated proliferative disordersCitation23. However, evident reports supported EGFR overexpression in numerous triple negative breast cancer (TNBC) patients, identified by lacking of oestrogen receptor (ER), progesterone receptor (PR) and HER-2 receptors, suggested that inhibition of EGFR is beneficial in these patientsCitation24,Citation25. Encorafenib (7) is another kinase inhibitors carrying both sulphonamide and pyrazole backbone which has been recently approved by the FDA to be used as combined kinases inhibitor cancer treatmentCitation26. Currently, medications with a “single-target single drug” are becoming less effective in treating cancer as it is heterogeneous, complicated and multi-genicCitation27,Citation28. Multi-target cancer chemotherapy as an alternative to combination therapy not only provides greater therapeutic anticancer benefits but it also reduces the risk of drug resistanceCitation29. It was noticed that, following EGFR inhibitor treatment acquired BRAFV600E mutation was suggested as a mechanism of resistanceCitation30–32. B-RAFV600E inhibition can also activate EGFR, resulting in recurring tumour proliferation, so in order to resolve this problem; a B-RAFV600E/EGFR combination inhibitor was utilizedCitation33.
Most of the clinically employed non-steroidal anti-inflammatory drugs (NSAIDs) are known to inhibit the two isoforms of cyclooxygenase (COX) enzyme: COX-1 (constitutive form) and COX-2 (inducible form)Citation34–38. Concomitant use of non-selective NSAIDs such as indomethacin (8) which act on both COX isoforms results in an increased risk of gastrointestinal complicationsCitation39–41. In contrast, selective COX-2 inhibitors such as celecoxib (9); a pyrazole-based containing sulphonamide moiety, act as anti-inflammatory without unwanted side effects on G.I. and renal systemsCitation42. There is an evidence to suggest the role of COX-2 in carcinogenesis, as the resulted prostaglandins due to activation of COX-2 are involved in key processes of cancer development including proliferation, mutagenesis, resistance to apoptosis, angiogenesis, immune suppression and invasionCitation43,Citation44. It has been proposed that COX-2 expression in ductal carcinoma in situ (DCIS) has a critical role in the development of breast cancer, as it plays a role in the destruction of the basal membrane and in formation of new blood vessels allowing tumour growth so the use of COX-2 inhibitors has been associated with reduced breast cancer riskCitation45–49.
In addition, non-steroidal anti-inflammatory drugs (NSAIDs) are used as auxiliary therapy with chemotherapeutics in order to cure pain caused by cancer or its treatmentCitation50–52. Nitric oxide (NO) contributes to the modulation of several key physiological functionsCitation53. One of the most important strategies used to overcome (GI-NSAIDs) side effects is designing nitric oxide-donating NSAIDs (NO-NSAIDs), which are capable of generating the radical bio-mediator and gastro protective NOCitation54. Moreover, NO can prevent tumour cells from metastasising and assists macrophage to kill tumour cellsCitation55. Furthermore, evidences showed that low levels (nanomolar) of NO promote tumour cell proliferation, angiogenesis and metastasis, while higher concentrations sensitivity of tumours to chemotherapy and overcome drug resistanceCitation56. Therefore combination of high concentrations NO donors and cancer therapy increases its efficiencyCitation57.
Our previously synthesised compound (10) have been reported as anti-inflammatory/anti-cancer agent having 1,2,4 triazole core linked to nitric oxide donating tail and to pyrazole scaffold as celecoxib analogue and it was the most active derivative against EGFR having sulphonamide groupCitation58. Recently, we also reported aldoxime derivative (11) with oxime as nitric oxide donor moiety acting as COX-2/aromatase inhibitor with both anti-inflammatory/anticancer activitiesCitation59. In addition, many triazoles and bistriazoles possess clinical importance (e.g. antibacterial, antifungal, anti-inflammatory, and anticancer)Citation60–62. Derivatives (12a,b) were reported as 1,2,4-triazoles bearing oxime as nitric oxide donor moiety and having B-RAFV600E inhibitory activity as anti-cancer agentsCitation63. Beside the exploration of new targets, there is another approach of combining two or more pharmacophores in a single moleculeCitation64,Citation65.
Based on the aforementioned data and as a continuation of our research interest in the scope of synthesis and biological evaluation of new anti-inflammatory and anti-cancer hybrid agents here we describe, synthesis, in vitro evaluation as COX-1/COX-2 inhibitors, in vitro anti-cancer activity on MCF7, Hep3B, HCT116, A549, normal fibroblasts F180 cell lines, cell cycle analysis, apoptosis, mechanistic study on aromatase, and EGFR & BRAFV600E enzymes inhibition and NO release for new series of bis-triazole scaffold as celecoxib analogue with nitric oxide donor activity 19a-l. The target derivatives 19a-l are hybrid molecules, their design was coming from lead compounds celecoxib (9) and previously reported compounds 10,11,12 in which some modifications in the structure of these compounds were carried out to trigger COX-2/Aromatase/BRAF/EGFR selective inhibition as: (1) Y-shape structure of celecoxib was maintained while pyrazole ring is replaced by 1,2,3-triazole moiety linked to sulphonamide moiety which is important pharmacophore for COX-2 inhibition (2) the presence of oxime tail as nitric oxide donor moiety linked to 1,2,4-triazole linker was incorporated in our designed compounds 19a-l to maintain the aromatase/EGFR/COX-2 inhibition as in previously reported derivatives 10 & 11 and to maintain the selective B-RAFV600E inhibition as in previously reported derivatives 12a,b ( and ).
Figure 1. Structure of selective oestrogen receptor blocker Tamoxifen (1), Steroidal aromatase inhibitors Exmestane (2), nonsteroidal aromatase inhibitor letrozole (4), anastrozole (5) Kinase inhibitors, Vemurafenib (6), Encorafenib (7), Non selective NSAIDs, Indomethacin (8), selective COX-2 inhibitors celecoxib (9), and 1,2,4 triazoles with anti-cancer activities (10) and anti-cancer 1,2,4 triazoles (10), (11), and B-RAFV600E inhibitor (12).
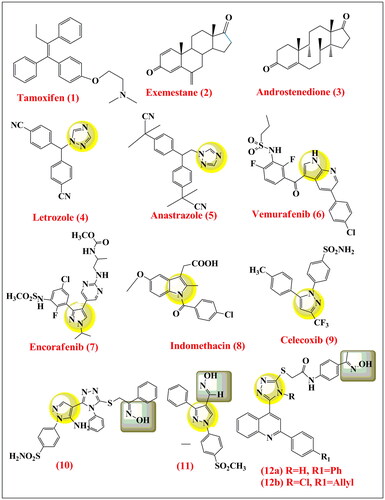
Experimental
Chemistry
General: Melting points were determined on a Thomas-Hoover capillary apparatus and are uncorrected. Infra-red (IR) spectra were recorded as films on KBr plates using a Nicolet 550 Series II Magna FT-IR spectrometer.1H NMR and 13C NMR spectra were measured on a Bruker 400 MHz NMR Spectrophotometer, Faculty of Pharmacy, Cairo University, Egypt in dimethyl sulfoxide (DMSO)-d6 with TMS as the internal standard, where J (coupling constant) values are estimated in Hertz (Hz). Mass spectra (MS) were recorded on a Water’s Micromass ZQ 4000 mass spectrometer using the electro-spray (ES) ionisation mode. Microanalyses were performed for C, H and N were carried out on Perkin-Elmer 2400 analyser (Perkin-Elmer, Norwalk, CT, USA) at the microanalytical unit of EL-Azhar University, Egypt. All compounds were within ± 0.4% of the theoretical values. Compounds 13, 15aCitation66 and 17a-fCitation67,Citation68 were prepared according to previously reported procedures.
Experimental procedures and spectral data for compound 15a,b (supplementary data S3)
A mixture of acid hydrazide (13) (0.01 mole) and phenyl isothiocyanate or 4-methoxy phenyl isothiocyanate (14a,b) (0.01 mole) in absolute ethanol (60 ml) was heated under reflux for 4h. The formed precipitate on cooling was filtered, dried and crystallised from ethanol to give compounds 15a,b. Physical and spectral data for 15b is listed below:
N-(4-methoxyphenyl)-2-(5-methyl-1-(4-sulfamoylphenyl)-1H-1,2,3-triazole-4-carbonyl) hydrazinecarbothioamide (15b): Yield 80%; white solid; m.p. 218–220 °C; IR (KBr): 3282, 3224 (NH2), 3147 (NH), 3043 (C–H aromatic), 2974 (C–H aliphatic), 1681 (C = O), 1550 (C = N), 1334, 1166 (SO2NH2), 1276 (C = S) cm−1; 1H NMR (DMSO-d6, 400 MHz, δ ppm): 2.61(s, 3H, CH3), 3.34 (s, 3H, OCH3) 7.11 (s, 1H, NH, CSNHNH-, D2O exchangeable D2O exchangeable), 7.30 (d, 2H, J = 8.0 Hz, 4-methoxyphenyl H-3, H-5), 7.52 (d, 2H, J = 8.0 Hz, 4-methoxyphenyl H-2, H-6), 7.64 (s, 2H, SO2NH2, D2O exchangeable), 7.88 (d, 2H, J = 8.0 Hz, 4-aminosulfonylphenyl H-3, H-5), 7.96 (d, 2H, J = 8.2 Hz 4-aminosulfonylphenyl H-2, H-6), 9.79 (s, 1H, CSNH-phenyl, D2O exchangeable), 10.62 (s, 1H, -CSNHNH-, D2O exchangeable); MS m/z (ES+) 461.09 (M+) (100%). Anal. Calcd. for C18H19N7O4S2: C, 46.84; H, 4.15; N, 21.24; Found; C, 46.62; H, 4.28; N, 21.46.
Experimental procedures and spectral data for compounds 16a,b (supplementary data S3)
A mixture of hydrazinecarbothioamide (15a,b) (0.01 mole) in an aqueous solution of sodium hydroxide (0.01 mole) was heated under reflux for 4h. After cooling the solution was acidified with hydrochloric acid and the formed precipitate was filtered, dried and crystallised from ethanol to give compounds 16a,b. Physical and spectral data are listed below:
4-(4-(5-mercapto-4-phenyl-4H-1,2,4-triazol-3-yl)-5-methyl-1H-1,2,3-triazol-1-yl)benzenesulfonamide (16a): Yield 65%; white solid; m.p. 244–246 °C; IR (KBr): 3360, 3278 (NH2), 3249 (NH), 3070 (C–H aromatic), 2966 (C–H aliphatic), 1593 (C = N), 1280 (C = S), 1330, 1168 (SO2NH2) cm−1; 1H NMR (DMSO-d6, 400 MHz, δ ppm): 2.61 (s, 3H, CH3), 7.41 (d, 2H, J = 8.0 Hz, phenyl H-2, H-6), 7.49 (m, 3H, phenyl H-3, H-4, H-5), 7.60 (s, 2H, SO2NH2, D2O exchangeable), 7.83 (d, 2H, J = 7.8 Hz, 4-aminosulfonylphenyl H-2, H-6), 8.02 (d, 2H, J = 7.8 Hz, 4-aminosulfonylphenyl H-3, H-5), 14.31 (s, 1H, NH-, D2O exchangeable); MS m/z (ES+) 413.07 (M+) (100%). Anal. Calcd. For C17H15N7O2S2; C, 49.38; H, 3.66; N, 23.71; Found; C, 49.56; H, 3.84; N, 23.90.
4-(4-(5-mercapto-4-(4-methoxyphenyl)-4H-1,2,4-triazol-3-yl)-5-methyl-1H-1,2,3-triazol-1-yl)benzenesulfonamide (16b): Yield 68%; white solid; m.p. 268–270 °C; IR (KBr): 3360, 3275 (NH2), 3251 (NH), 3066 (C–H aromatic), 2970 (C–H aliphatic), 1593 (C = N), 1331, 1168, (SO2NH2), 1281 (C = S), cm−1; 1H NMR (DMSO-d6, 400 MHz, δ ppm): 2.60 (s, 3H, CH3), 3.34 (s, 3H, OCH3), 7.40 (d, 2H, J = 8.0 Hz, 4-methoxyphenyl H-3, H-5), 7.49 (d, 2H, J = 8.0 Hz, 4-methoxyphenyl H-2, H-6), 7.60 (s, 2H, SO2NH2, D2O exchangeable), 7.80 (d, 2H, J = 7.8 Hz, 4-aminosulfonylphenyl H-2, H-6), 8.04(d, 2H, J = 7.8 Hz, 4-aminosulfonylphenyl H-3, H-5), 14.30 (s, 1H, NH-, D2O exchangeable); MS m/z (ES+) 443.08 (M+) (100%). Anal. Calcd. For C17H15N7O2S2; C, 48.75; H, 3.86; N, 22.11; Found; C, 48.88; H, 3.72; N, 22.18.
Experimental procedures and spectral data for compounds 18a-l (Supplementary data S3)
An equimolecular mixture of 16a,b, phenacyl bromide derivatives (17a-f) (0.01 mole) and triethyl amine (0.12 g, 0.012 mole) in acetonitrile (50 ml) was heated under reflux for 10 h. The reaction mixture was evaporated to dryness and the residue was crystallised from ethanol to give compounds 17a-f. Physical and spectral data are listed below:
4-(5-methyl-4-(5-((2-oxo-2-phenylethyl)thio)-4-phenyl-4H-1,2,4-triazol-3-yl)-1H-1,2,3-triazol-1-yl)benzenesulfonamide (18a): Yield 61%; white solid; m.p. 288 - 290 °C; IR (KBr): 3370, 3206 (NH2), 3070 (C–H aromatic), 2981 (C–H aliphatic), 1674 (C = O), 1581 (C = N), 1323, 1161 (SO2NH2) cm1; 1H NMR (DMSO-d6, 400 MHz, δ ppm): 2.57 (s, 3H, CH3), 4.99 (s, 2H, CH2), 7.49 (d, 2H, J = 8.0 Hz, phenyl H-2, H-6), 6.58 (m, 7H, 4-phenyltriazole H-2, H-6, H-3, H-4, H-5, NH2, D2O exchangeable), 7.69 (m, 1H, phenyl H-4), 7.85 (d, 2H, J = 8.0 Hz phenyl H-3, H-5), 8.04 (m, 4H, 4-aminosulfonylphenyl H-2, H-6, H-3, H-5); 13C NMR (DMSO-d6 100 MHz, δ ppm): 10.09, 126.00, 127.64, 128.13, 128.89, 129.33, 129.99, 130.38, 133.71, 134.20, 134.27, 135.79, 138.17, 145.51, 147.91, 152.06, 193.51; MS m/z (ES+) 531.11 (M+) (100%). Anal. Calcd. For C25H21N7O3S2: C, 56.48; H, 3.98; N, 18.44; Found; C, 56.78; H, 4.12; N, 18.10.
4-(5-methyl-4-(5-((2-oxo-2-(p-tolyl)ethyl)thio)-4-phenyl-4H-1,2,4-triazol-3-yl)-1H-1,2,3-triazol-1-yl)benzenesulfonamide (18b): Yield 61%; white solid; m.p. 236- 238 °C; IR (KBr): 3367, 3267 (NH2), 3066 (C–H aromatic), 2920 (C–H aliphatic), 1674 (C = O), 1597 (C = N), 1346, 1161 (SO2NH2) cm1; 1H NMR (DMSO-d6, 400 MHz, δ ppm): 2.41 (s, 3H, phenyl-CH3), 2.57 (s, 3H, triazole-CH3), 4.96 (s, 2H, CH2), 7.38 (d, 2H, J = 8.0 Hz, 4-methylphenyl H-3, H-5), 7.48 (d, 2H, J = 8.0 Hz, 4-methylphenyl H-2, H-6), 7.57 (s, 2H, SO2NH2, D2O exchangeable), 7.58 (m, 3H, phenyl H-3, H-4, H-5), 7.86 (d, 2H, J = 8.0 Hz phenyl H-2, H-6), 7.94 (d, 2H, J = 8.0 Hz 4-aminosulfonylphenyl H-2, H-6), 8.03 (d, 2H, J = 8.0 Hz 4-aminosulfonylphenyl H-3, H-5); 13C NMR (DMSO-d6 100 MHz, δ ppm): 10.08, 21.69, 46.21, 126.00, 127.65, 128.12, 129.00, 129.87, 129.99, 130.39, 133.26, 133.70, 134.18, 135.81, 138.17, 144.85, 145.47, 147.89, 152.12, 193.01; MS m/z (ES+) 545.13 (M+) (100%). Anal. Calcd. For C26H23N7O3S2: C, 57.23; H, 4.25; N, 17.97; Found; C, 57.54; H, 4.06; N, 18.14.
4-(4-(5-((2-(4-methoxyphenyl)-2-oxoethyl)thio)-4-phenyl-4H-1,2,4-triazol-3-yl)-5-methyl-1H-1,2,3-triazol-1-yl)benzenesulfonamide (18c): Yield 71%; white solid; m.p. 296 - 298 °C; IR (KBr): 3347, 3209 (NH2), 3070 (C–H aromatic), 2939 (C–H aliphatic), 1666 (C = O), 1597 (C = N), 1327, 1165 (SO2NH2) cm1; 1H NMR (DMSO-d6, 400 MHz, δ ppm): 2.57 (s, 3H, triazole-CH3), 3.87 (s, 3H, phenyl-OCH3), 4.93 (s, 2H, CH2), 7.08 (d, 2H, J = 8.2 Hz, 4-methoxyphenyl H-3, H-5), 7.48 (d, 2H, J = 8.2 Hz, 4-methoxyphenyl H-2, H-6), 7.57 (s, 2H, SO2NH2, D2O exchangeable), 7.58 (m, 3H, phenyl H-3, H-4, H-5), 7.86 (d, 2H, J = 8.0 Hz phenyl H-2, H-6), 8.01 (d, 2H, J = 8.0 Hz 4-aminosulfonylphenyl H-2, H-6), 8.03 (d, 2H, J = 8.0 Hz 4-aminosulfonylphenyl H-3, H-5); 13C NMR (DMSO-d6 100 MHz, δ ppm): 10.08, 46.20, 56.12, 114.55, 126.00, 127.65, 128.13, 128.61, 129.98, 130.37, 131.30, 133.71, 134.20, 135.81, 138.17, 145.48, 147.88, 152.17, 164.09, 191.84; MS m/z (ES+) 561.13 (M+) (100%). Anal. Calcd. For C26H23N7O4S2: C, 55.60; H, 4.13; N, 17.46; Found; C, 55.88; H, 4.34; N, 17.22.
4-(4-(5-((2-(4-chlorophenyl)-2-oxoethyl)thio)-4-phenyl-4H-1,2,4-triazol-3-yl)-5-methyl-1H-1,2,3-triazol-1-yl)benzenesulfonamide (18d): Yield 67%; white solid; m.p. 236 - 238 °C; IR (KBr): 3348, 3251 (NH2), 3066 (C–H aromatic), 2997 (C–H aliphatic), 1681 (C = O), 1589 (C = N), 1338, 1180 (SO2NH2) cm1; 1H NMR (DMSO-d6, 400 MHz, δ ppm): 2.57 (s, 3H, triazole-CH3), 4.97 (s, 2H, CH2), 7.48 (d, 2H, J = 8.0 Hz phenyl H-2, H-6), 7.57(s, 2H, SO2NH2, D2O exchangeable), 7.58 (m, 3H, phenyl H-3, H-4, H-5), 7.65 (d, 2H, J = 8.2 Hz, 4-chlorophenyl H-2, H-6), 7.86 (d, 2H, J = 8.2 Hz, 4-chlorophenyl H-3, H-5), 8.03 (d, 2H, J = 8.0 Hz 4-aminosulfonylphenyl H-2, H-6), 8.06 (d, 2H, J = 8.0 Hz 4-aminosulfonylphenyl H-3, H-5); 13C NMR (DMSO-d6 100 MHz, δ ppm): 10.10, 25.95, 62.49, 126.00, 127.64, 128.12, 129.45, 129.99, 130.39, 130.82, 133.70, 134.17, 134.50, 135.81, 138.17, 139.18, 145.50, 147.94, 151.95, 192.67; MS m/z (ES+) 565.08 (M+) (100%). Anal. Calcd. For C25H20ClN7O3S2: C, 53.05; H, 3.56; N, 17.32; Found; C, 53.22; H, 3.72; N, 17.56.
4-(5-methyl-4-(5-((2-(4-nitrophenyl)-2-oxoethyl)thio)-4-phenyl-4H-1,2,4-triazol-3-yl)-1H-1,2,3-triazol-1-yl)benzenesulfonamide (18e): Yield 84%; white solid; m.p. 298 − 300 °C; IR (KBr): 3332, 3251 (NH2), 3059 (C–H aromatic), 2994 (C–H aliphatic), 1685 (C = O), 1597 (C = N), 1338, 1172 (SO2NH2) cm1; 1H NMR (DMSO-d6, 400 MHz, δ ppm): 2.57 (s, 3H, triazole-CH3), 5.03 (s, 2H, CH2), 7.48 (d, 2H, J = 7.8 Hz phenyl H-2, H-6), 7.57 (s, 2H, SO2NH2, D2O exchangeable), 7.58 (m, 3H, phenyl H-3, H-4, H-5), 7.86 (d, 2H, J = 8.2 Hz, 4-nitrophenyl H-2, H-6), 8.03(d, 2H, J = 8.2 Hz, 4-nitrophenyl H-3, H-5), 8.27 (d, 2H, J = 8.0 Hz 4-aminosulfonylphenyl H-2, H-6), 8.38 (d, 2H, J = 8.0 Hz 4-aminosulfonylphenyl H-3, H-5); 13C NMR (DMSO-d6 100 MHz, δ ppm): 10.08, 25.90, 62.52, 124.39, 126.00, 127.65, 128.08, 130.02, 130.34, 130.44, 133.64, 134.11, 135.85, 138.15, 140.49, 145.47, 147.99, 150.64, 151.83, 193.01; MS m/z (ES+) 576.10 (M+) (100%). Anal. Calcd. For C25H20N8O5S2: C, 52.07; H, 3.50; N, 19.43; Found; C, 57.18; H, 3.34; N, 19.58.
4-(4-(5-((2-(4-bromophenyl)-2-oxoethyl)thio)-4-phenyl-4H-1,2,4-triazol-3-yl)-5-methyl-1H-1,2,3-triazol-1-yl)benzenesulfonamide (18f): Yield 79%; white solid; m.p. 274 - 276 °C; IR (KBr): 3344, 3248 (NH2), 3066 (C–H aromatic), 2951 (C–H aliphatic), 1681 (C = O), 1585 (C = N), 1338, 1171 (SO2NH2) cm1; 1H NMR (DMSO-d6, 400 MHz, δ ppm): 2.57 (s, 3H, triazole-CH3), 4.96 (s, 2H, CH2), 7.47 (d, 2H, J = 7.8 Hz phenyl H-2, H-6), 7.57 (s, 2H, SO2NH2, D2O exchangeable), 7.58 (m, 3H, phenyl H-3, H-4, H-5), 7.79 (d, 2H, J = 8.2 Hz, 4-bromophenyl H-2, H-6), 7.85 (d, 2H, J = 8.2 Hz, 4-bromophenyl H-3, H-5), 8.02 (d, 2H, J = 8.0 Hz 4-aminosulfonylphenyl H-2, H-6), 8.05 (d, 2H, J = 8.0 Hz 4-aminosulfonylphenyl H-3, H-5); 13C NMR (DMSO-d6 100 MHz, δ ppm): 10.07, 25.91, 46.03, 126.00, 127.65, 128.09, 128.41, 130.01, 130.41, 130.89, 132.40, 133.66, 134.13, 134.79, 135.83, 138.15, 145.47, 147.93, 151.96, 192.90; MS m/z (ES+) 609.03 (M+) (100%). Anal. Calcd. For C25H20BrN7O3S2: C, 49.18; H, 3.30; N, 16.06; Found; C, 49.44; H, 3.52; N, 16.28.
4-(4-(4-(4-methoxyphenyl)-5-((2-oxo-2-phenylethyl)thio)-4H-1,2,4-triazol-3-yl)-5-methyl-1H-1,2,3-triazol-1-yl)benzenesulfonamide (18 g): Yield 75%; white solid; m.p. 294 - 296 °C; IR (KBr): 3340, 3224 (NH2), 3062 (C–H aromatic), 2966 (C–H aliphatic), 1681 (C = O), 1597 (C = N), 1300, 1168 (SO2NH2) cm1; 1H NMR (DMSO-d6, 400 MHz, δ ppm): 2.57 (s, 3H, CH3), 3.34 (s, 3H, OCH3) 4.98 (s, 2H, CH2), 7.08 (d, 2H, J = 8.4 Hz, 4-methoxyphenyl H-2, H-6), 7.40 (d, 2H, J = 8.4 Hz, 4-methoxyphenyl H-3, H-5), 6.56 (m, 4H, 4-phenyltriazole H-3, H-5, NH2, D2O exchangeable), 7.69 (m, 1H, phenyl H-4), 7.86 (d, 2H, J = 7.6 Hz phenyl H-2, H-6), 8.03 (d, 2H, J = 8.4 Hz, 4-aminosulfonylphenyl H-2, H-6), 8.05 (d, 2H, J = 8.4 Hz, 4-aminosulfonylphenyl H-3, H-5); 13C NMR (DMSO-d6 100 MHz, δ ppm): 10.07, 55.96, 115.03, 125.99, 126.60, 127.65, 128.88, 129.34, 129.47, 133.78, 134.29, 135.76, 138.18, 145.45, 148.15, 152.50, 160.46, 193.58; MS m/z (ES+) 561.13 (M+) (100%). Anal. Calcd. For C26H23N7O4S2: C, 55.60; H, 4.13; N, 17.46; Found; C, 55.76; H, 4.32; N, 17.12.
4-(4-(4-(4-methoxyphenyl)-5-((2-oxo-2-(p-tolyl)ethyl)thio)-4H-1,2,4-triazol-3-yl)-5-methyl-1H-1,2,3-triazol-1-yl)benzenesulfonamide (18h): Yield 80%; white solid; m.p. 286- 288 °C; IR (KBr): 3390, 3116 (NH2), 3016 (C–H aromatic), 2920 (C–H aliphatic), 1681 (C = O), 1597 (C = N), 1361, 1145 (SO2NH2) cm1; 1H NMR (DMSO-d6, 400 MHz, δ ppm): 2.41 (s, 3H, phenyl-CH3), 2.56 (s, 3H, triazole-CH3), 3.84 (s, 3H, phenyl-OCH3) 4.94 (s, 2H, CH2), 7.08 (d, 2H, J = 8.4 Hz, 4-methoxyphenyl H-3, H-5), 7.37 (d, 2H, J = 8.4 Hz, 4-methoxphenyl H-2, H-6), 7.41 (d, 2H, J = 8.2 Hz, 4-methylphenyl H-2, H-6), 7.58 (s, 2H, SO2NH2, D2O exchangeable), 7.86 (d, 2H, J = 8.2 Hz 4-methylphenyl H-2, H-6), 7.93 (d, 2H, J = 8.2 Hz 4-aminosulfonylphenyl H-2, H-6), 8.03 (d, 2H, J = 8.2 Hz 4-aminosulfonylphenyl H-3, H-5); 13C NMR (DMSO-d6 100 MHz, δ ppm): 10.08, 21.69, 55.95, 115.02, 125.98, 126.62, 127.66, 129.00, 129.47, 129.87, 133.26, 133.80, 135.76, 138.20, 144.84, 145.46, 148.14, 152.54, 160.45, 193.07; MS m/z (ES+) 575.14 (M+) (100%). Anal. Calcd. For C27H25N7O4S2: C, 56.33; H, 4.38; N, 17.03; Found; C, 56.58; H, 4.16; N, 16.86.
4-(4-(4-(4-methoxyphenyl)-5-((2-(4-methoxyphenyl)-2-oxoethyl)thio)-4H-1,2,4-triazol-3-yl)-5-methyl-1H-1,2,3-triazol-1-yl)benzenesulfonamide (18i): Yield 70%; white solid; m.p. 242 - 244 °C; IR (KBr): 3425, 3223 (NH2), 3116 (C–H aromatic), 2962 (C–H aliphatic), 1743 (C = O), 1593 (C = N), 1369, 1145 (SO2NH2) cm1; 1H NMR (DMSO-d6, 400 MHz, δ ppm): 2.57 (s, 3H, triazole-CH3), 3.84 (s, 3H, phenyl-OCH3), 3.87 (s, 3H, 4-phenyl-triazole-OCH3), 4.92 (s, 2H, CH2), 7.08 (d, 2H, J = 8.2 Hz, 4-methoxyphenyl H-3, H-5), 7.10 (d, 2H, J = 8.2 Hz, 4-methoxyphenyl H-2, H-6), 7.86 (d, 2H, J = 8.2 Hz, 4-(4-methoxyphenyl)triazole H-2, H-6), 7.59 (s, 2H, SO2NH2, D2O exchangeable), 7.86 (d, 2H, J = 8.2 Hz, 4-(4-methoxyphenyl)triazole H-3, H-5), 8.01 (d, 2H, J = 8.0 Hz 4-aminosulfonylphenyl H-2, H-6), 8.03 (d, 2H, J = 8.0 Hz 4-aminosulfonylphenyl H-3, H-5); 13C NMR (DMSO-d6 100 MHz, δ ppm): 10.07, 25.89, 55.95, 56.11, 114.55, 115.02, 125.99, 126.61, 127.66, 128.59, 129.48, 131.31, 133.79, 135.77, 138.19, 145.44, 148.13, 152.60, 160.45, 164.08, 191.90; MS m/z (ES+) 591.14 (M+) (100%). Anal. Calcd. For C27H25N7O5S2: C, 54.81; H, 4.26; N, 16.57; Found; C, 54.62; H, 4.12; N, 16.84.
4-(4-(5-((2-(4-chlorophenyl)-2-oxoethyl)thio)-4-(4-methoxyphenyl)-4H-1,2,4-triazol-3-yl)-5-methyl-1H-1,2,3-triazol-1-yl)benzenesulfonamide (18j): Yield 77%; white solid; m.p. 214 - 216 °C; IR (KBr): 3325, 3174 (NH2), 3066 (C–H aromatic), 2962 (C–H aliphatic), 1674 (C = O), 1589 (C = N), 1346, 1165 (SO2NH2) cm1; 1H NMR (DMSO-d6, 400 MHz, δ ppm): 2.56 (s, 3H, triazole-CH3), 3.84 (s, 3H, OCH3), 4.95 (s, 2H, CH2), 7.08 (d, 2H, J = 8.0 Hz 4-methoxyphenyl H-3, H-5), 7.39 (d, 2H, J = 8.0 Hz 4-methoxyphenyl H-2, H-6), 7.58 (s, 2H, SO2NH2, D2O exchangeable), 7.64 (d, 2H, J = 8.0 Hz 4-chlorophenyl H-2, H-6), 7.86 (d, 2H, J = 8.2 Hz, 4-chlorophenyl H-3, H-5), 8.03 (d, 2H, J = 8.0 Hz 4-aminosulfonylphenyl H-2, H-6), 8.05 (d, 2H, J = 8.0 Hz 4-aminosulfonylphenyl H-3, H-5); 13C NMR (DMSO-d6 100 MHz, δ ppm): 10.07, 25.90, 55.95, 115.03, 125.99, 126.57, 127.66, 129.44, 130.82, 133.76, 134.46, 135.78, 138.18, 139.19, 145.45, 148.18, 152.38, 160.46, 192.73; MS m/z (ES+) 595.09 (M+) (100%). Anal. Calcd. For C26H22ClN7O4S2: C, 52.39; H, 3.72; N, 16.45; Found; C, 52.12; H, 3.54; N, 16.20.
4-(4-(4-(4-methoxyphenyl)-5-((2-(4-nitrophenyl)-2-oxoethyl)thio)-4H-1,2,4-triazol-3-yl)-5-methyl-1H-1,2,3-triazol-1-yl)benzenesulfonamide (18k): Yield 89%; white solid; m.p. 310 – 312 °C; IR (KBr): 3344, 3248 (NH2), 3088 (C–H aromatic), 2997 (C–H aliphatic), 1681 (C = O), 1585 (C = N), 1338, 1172 (SO2NH2) cm1; 1H NMR (DMSO-d6, 400 MHz, δ ppm): 2.56 (s, 3H, triazole-CH3), 3.84 (s, 3H, OCH3), 5.01 (s, 2H, CH2), 7.08 (d, 2H, J = 7.8 Hz 4-methoxyphenyl H-3, H-5), 7.40 (d, 2H, J = 7.8 Hz 4-methoxyphenyl H-2, H-6), 7.58 (s, 2H, SO2NH2, D2O exchangeable), 7.86 (d, 2H, J = 8.2 Hz, 4-nitrophenyl H-2, H-6), 8.02(d, 2H, J = 8.2 Hz, 4-nitrophenyl H-3, H-5), 8.27 (d, 2H, J = 8.0 Hz 4-aminosulfonylphenyl H-2, H-6), 8.38 (d, 2H, J = 8.0 Hz 4-aminosulfonylphenyl H-3, H-5); 13C NMR (DMSO-d6 100 MHz, δ ppm): 10.07, 46.26, 55.96, 115.04, 124.38, 125.99, 126.53, 127.66, 129.45, 130.35, 133.72, 135.80, 138.17, 140.47, 145.45, 148.23, 150.63, 152.23, 160.47, 193.06; MS m/z (ES+) 576.10 (M+) (100%). Anal. Calcd. For C26H22N8O6S2: C, 51.48; H, 3.66; N, 18.47; Found; C, 51.26; H, 3.56; N, 18.72.
4-(4-(5-((2-(4-bromophenyl)-2-oxoethyl)thio)-4-(4-methoxyphenyl)-4H-1,2,4-triazol-3-yl)-5-methyl-1H-1,2,3-triazol-1-yl)benzenesulfonamide (18 l): Yield 86%; white solid; m.p. 230 - 232 °C; IR (KBr): 3367, 3263 (NH2), 3012 (C–H aromatic), 2974 (C–H aliphatic), 1674 (C = O), 1585 (C = N), 1338, 1166 (SO2NH2) cm1; 1H NMR (DMSO-d6, 400 MHz, δ ppm): 2.56 (s, 3H, triazole-CH3), 2.84 (s, 3H, OCH3), 4.94 (s, 2H, CH2), 7.07 (d, 2H, J = 7.8 Hz 4-methoxyphenyl H-3, H-5), 7.39 (d, 2H, J = 7.8 Hz 4-methoxyphenyl H-2, H-6), 7.58 (s, 2H, SO2NH2, D2O exchangeable), 7.79 (d, 2H, J = 8.2 Hz, 4-bromophenyl H-2, H-6), 7.39 (d, 2H, J = 8.2 Hz, 4-bromophenyl H-3, H-5), 7.86 (d, 2H, J = 8.2 Hz, 4-bromophenyl H-3, H-5), 7.97 (d, 2H, J = 8.4 Hz 4-aminosulfonylphenyl H-2, H-6), 8.02 (d, 2H, J = 8.4 Hz 4-aminosulfonylphenyl H-3, H-5); 13C NMR (DMSO-d6 100 MHz, δ ppm): 10.09, 55.96, 115.02, 125.99, 126.60, 127.64, 128.38, 129.47, 130.91, 132.39, 133.78, 134.81, 135.75, 138.19, 145.49, 148.18, 152.34, 160.46, 192.96; MS m/z (ES+) 639.04 (M+) (100%). Anal. Calcd. For C26H22BrN7O4S2: C, 48.75; H, 3.46; N, 15.31; Found; C, 48.52; H, 3.50; N, 15.22.
Experimental procedures and spectral data for compounds 19a-l (supplementary data S3)
A mixture of the appropriate ketones 18a-l (0.01 mole), hydroxylamine hydrochloride (0.01 mole) and sodium acetate (0.01 mole) in acetonitrile (30 ml) was heated under reflux for 10h. The formed precipitate upon cooling was filtered off, washed with sodium carbonate solution (10%) and distilled water, dried and recrystallized from ethanol to give compounds 19a-l. Physical and spectral data are listed below:
(E)-4-(4-(5-((2-(hydroxyimino)-2-phenylethyl)thio)-4-phenyl-4H-1,2,4-triazol-3-yl)-5-methyl-1H-1,2,3-triazol-1-yl)benzenesulfonamide (19a): Yield 54%; white solid; m.p. 308 - 310 °C; IR (KBr): 3618 (OH), 3330, 3240 (NH2), 3062 (C–H aromatic), 2920 (C–H aliphatic), 1597 (C = N), 1342, 1161 (SO2NH2) cm1; 1H NMR (DMSO-d6, 400 MHz, δ ppm): 2.60 (s, 3H, CH3), 4.46 (s, 2H, CH2), 7.40 (m, 5H, phenyl H-2, H-3, H-4, H-5, H-6), 7.51 (m, 3H, 4-phenyltriazole H-3, H-4, H-5), 7.59 (s, 2 H, NH2, D2O exchangeable), 7.68 (d, 2H, J = 8.0 Hz phenyl H-2, H-6), 7.86 (d, 2H, J = 8.0 Hz 4-aminosulfonylphenyl H-2, H-6), 8.03 (d, 2H, J = 8.0 Hz 4-aminosulfonylphenyl H-3, H-5), 11.83 (s, 1H, OH, D2O exchangeable); 13C NMR (DMSO-d6 100 MHz, δ ppm): 10.13, 26.02, 126.01, 126.37, 127.67, 128.25, 129.02, 129.67, 129.81, 130.28, 133.75, 134.22, 134.74, 135.89, 138.17, 145.49, 148.28, 151.80, 152.40; MS m/z (ES+) 546.13 (M+) (100%). Anal. Calcd. For C25H22N8O3S2: C, 54.93; H, 4.06; N, 20.50; Found; C, 54.78; H, 4.22; N, 20.68.
(E)-4-(4-(5-((2-(hydroxyimino)-2-(p-tolyl)ethyl)thio)-4-phenyl-4H-1,2,4-triazol-3-yl)-5-methyl-1H-1,2,3-triazol-1-yl)benzenesulfonamide (19b): Yield 67%; white solid; m.p. 302- 304 °C; IR (KBr): 3607 (OH), 3347, 3224 (NH2), 3066 (C–H aromatic), 2958 (C–H aliphatic), 1597 (C = N), 1323, 1165 (SO2NH2) cm1; 1H NMR (DMSO-d6, 400 MHz, δ ppm): 2.60 (s, 3H, phenyl-CH3), 3.78 (s, 3H, triazole-CH3), 4.44 (s, 2H, CH2), 6.95 (d, 2H, J = 8.0 Hz, 4-methylphenyl H-3, H-5), 7.40 (d, 2H, J = 8.0 Hz, 4-methylphenyl H-2, H-6), 7.51 (m, 3H, phenyl H-3, H-4, H-5), 7.53 (s, 2H, SO2NH2, D2O exchangeable), 7.61 (d, 2H, J = 8.0 Hz phenyl H-2, H-6), 7.86 (d, 2H, J = 8.2 Hz 4-aminosulfonylphenyl H-2, H-6), 8.03 (d, 2H, J = 8.2 Hz 4-aminosulfonylphenyl H-3, H-5), 11.60 (s, 1H, OH, D2O exchangeable); 13C NMR (DMSO-d6 100 MHz, δ ppm): 10.12, 25.89, 55.68, 114.44, 126.00, 127.10, 127.66, 127.78, 128.24, 129.82, 130.28, 133.76, 134.21, 135.87, 138.17, 145.59, 148.25, 151.95, 160.54; MS m/z (ES+) 560.14 (M+) (100%). Anal. Calcd. For C26H24N8O3S2: C, 55.70; H, 4.31; N, 19.99; Found; C, 55.92; H, 4.52; N, 20.16.
(E)-4-(4-(5-((2-(hydroxyimino)-2-(4-methoxyphenyl)ethyl)thio)-4-phenyl-4H-1,2,4-triazol-3-yl)-5-methyl-1H-1,2,3-triazol-1-yl)benzenesulfonamide (19c): Yield 69%; white solid; m.p. 300 - 302 °C; IR (KBr): 3609 (OH), 3340, 3244 (NH2), 3062 (C–H aromatic), 2990 (C–H aliphatic), 1597 (C = N), 1346, 1165 (SO2NH2) cm1; 1H NMR (DMSO-d6, 400 MHz, δ ppm): 2.32 (s, 3H, triazole-CH3), 2.60 (s, 3H, phenyl-OCH3), 4.44 (s, 2H, CH2), 7.20 (d, 2H, J = 8.0 Hz, 4-methoxyphenyl H-3, H-5), 7.39 (d, 2H, J = 8.0 Hz, 4-methoxyphenyl H-2, H-6), 7.41 (m, 3H, phenyl H-3, H-4, H-5), 7.53 (s, 2H, SO2NH2, D2O exchangeable), 7.55 (d, 2H, J = 8.0 Hz phenyl H-2, H-6), 7.86 (d, 2H, J = 8.2 Hz 4-aminosulfonylphenyl H-2, H-6), 8.03 (d, 2H, J = 8.2 Hz 4-aminosulfonylphenyl H-3, H-5), 11.71 (s, 1H, OH, D2O exchangeable); 13C NMR (DMSO-d6 100 MHz, δ ppm): 10.12, 21.28, 25.93, 126.00, 126.26, 127.67, 128.24, 129.60, 129.82, 130.28, 131.93, 133.76, 134.21, 135.88, 138.17, 139.26, 148.26, 151.87, 152.24; MS m/z (ES+) 576.14 (M+) (100%). Anal. Calcd. For C26H24N8O4S2: C, 54.15; H, 4.20; N, 19.43; Found; C, 54.38; H, 4.42; N, 19.54.
(E)-4-(4-(5-((2-(4-chlorophenyl)-2-(hydroxyimino)ethyl)thio)-4-phenyl-4H-1,2,4-triazol-3-yl)-5-methyl-1H-1,2,3-triazol-1-yl)benzenesulfonamide (19d): Yield 72%; white solid; m.p. 292 - 294 °C; IR (KBr): 3611 (OH), 3332, 3244 (NH2), 3070(C–H aromatic), 2990 (C–H aliphatic), 1583 (C = N), 1342, 1165 (SO2NH2) cm1; 1H NMR (DMSO-d6, 400 MHz, δ ppm): 2.60 (s, 3H, triazole-CH3), 4.43 (s, 2H, CH2), 7.40 (d, 2H, J = 8.2 Hz phenyl H-2, H-6), 7.42 (d, 2H, J = 8.0 Hz, 4-chlorophenyl H-2, H-6), 7.49 (m, 3H, phenyl H-3, H-4, H-5), 7.53 (s, 2H, SO2NH2, D2O exchangeable), 7.69 (d, 2H, J = 8.0 Hz, 4-chlorophenyl H-3, H-5), 7.86 (d, 2H, J = 8.2 Hz 4-aminosulfonylphenyl H-2, H-6), 8.03 (d, 2H, J = 8.2 Hz 4-aminosulfonylphenyl H-3, H-5), 11.93 (s, 1H, OH, D2O exchangeable); 13C NMR (DMSO-d6 100 MHz, δ ppm): 10.13, 25.94, 62.49, 126.00, 127.65, 128.17, 128.26, 129.03, 129.80, 130.27, 133.63, 134.23, 134.32, 135.88, 138.16, 145.51, 148.31, 151.51, 151.55; MS m/z (ES+) 580.09 (M+) (100%). Anal. Calcd. For C25H21ClN8O3S2: C, 51.68; H, 3.64; N, 19.28; Found; C, 51.78; H, 3.86; N, 19.52.
(E)-4-(4-(5-((2-(hydroxyimino)-2-(4-nitrophenyl)ethyl)thio)-4-phenyl-4H-1,2,4-triazol-3-yl)-5-methyl-1H-1,2,3-triazol-1-yl)benzenesulfonamide (19e): Yield 73%; white solid; m.p. 274 – 276 °C; IR (KBr): 3618 (OH), 3330, 3244 (NH2), 3070 (C–H aromatic), 2908 (C–H aliphatic), 1597 (C = N), 1342, 1165 (SO2NH2) cm1; 1H NMR (DMSO-d6, 400 MHz, δ ppm): 2.59 (s, 3H, triazole-CH3), 4.47 (s, 2H, CH2), 7.40 (d, 2H, J = 8.2 Hz phenyl H-2, H-6), 7.42 (m, 3H, phenyl H-3, H-4, H-5), 7.58 (s, 2H, SO2NH2, D2O exchangeable), 7.85 (d, 2H, J = 8.2 Hz, 4-nitrophenyl H-2, H-6), 7.94 (d, 2H, J = 8.2 Hz, 4-nitrophenyl H-3, H-5), 8.03 (d, 2H, J = 8.0 Hz 4-aminosulfonylphenyl H-2, H-6), 8.25 (d, 2H, J = 8.0 Hz 4-aminosulfonylphenyl H-3, H-5), 12.35 (s, 1H, OH, D2O exchangeable); 13C NMR (DMSO-d6 100 MHz, δ ppm): 10.11, 25.95, 124.15, 126.00, 127.58, 127.67, 128.26, 129.80, 130.28, 133.72, 134.21, 135.93, 138.15, 141.01, 145.49, 148.02, 148.38, 151.19, 151.38; MS m/z (ES+) 591.11 (M+) (100%). Anal. Calcd. For C25H21N9O5S2: C, 50.75; H, 3.58; N, 21.31; Found; C, 50.58; H, 3.74; N, 21.08.
(E)-4-(4-(5-((2-(4-bromophenyl)-2-(hydroxyimino)ethyl)thio)-4-phenyl-4H-1,2,4-triazol-3-yl)-5-methyl-1H-1,2,3-triazol-1-yl)benzenesulfonamide (19f): Yield 80%; white solid; m.p. 290 - 292 °C; IR (KBr): 3602 (OH), 3344, 3251 (NH2), 3097 (C–H aromatic), 2927 (C–H aliphatic), 1583 (C = N), 1338, 1165 (SO2NH2) cm1; 1H NMR (DMSO-d6, 400 MHz, δ ppm): 2.60 (s, 3H, triazole-CH3), 4.42 (s, 2H, CH2), 7.40 (d, 2H, J = 7.8 Hz phenyl H-2, H-6), 7.42 (m, 3H, phenyl H-3, H-4, H-5), 7.51 (d, 2H, J = 8.2 Hz, 4-bromophenyl H-2, H-6), 7.53 (s, 2H, SO2NH2, D2O exchangeable), 7.59 (d, 2H, J = 8.2 Hz, 4-bromophenyl H-3, H-5), 7.86 (d, 2H, J = 8.0 Hz 4-aminosulfonylphenyl H-2, H-6), 8.03 (d, 2H, J = 8.0 Hz 4-aminosulfonylphenyl H-3, H-5), 11.94 (s, 1H, OH, D2O exchangeable); 13C NMR (DMSO-d6 100 MHz, δ ppm): 10.14, 25.87, 123.04, 126.00, 127.66, 128.26, 128.44, 129.80, 130.27, 130.90, 131.95, 132.40, 133.75, 133.99, 134.23, 135.88, 138.16, 145.51, 148.31, 151.50, 151.; MS m/z (ES+) 624.04 (M+) (100%). Anal. Calcd. For C25H21BrN8O3S2: C, 48.00; H, 3.38; N, 17.91 Found; C, 47.82; H, 3.12; N, 18.08.
(E)-4-(4-(5-((2-(hydroxyimino)-2-phenylethyl)thio)-4-(4-methoxyphenyl)-4H-1,2,4-triazol-3-yl)-5-methyl-1H-1,2,3-triazol-1-yl)benzenesulfonamide (19 g): Yield 82%; white solid; m.p. 240 - 242 °C; IR (KBr): 3600 (OH), 3336, 3232 (NH2), 3070 (C–H aromatic), 2974 (C–H aliphatic), 1597 (C = N), 1346, 1168 (SO2NH2) cm1; 1H NMR (DMSO-d6, 400 MHz, δ ppm): 2.59 (s, 3H, CH3), 3.82 (s, 3H, OCH3) 4.46 (s, 2H, CH2), 7.02 (d, 2H, J = 8.0 Hz, 4-methoxyphenyl H-2, H-6), 7.31 (d, 2H, J = 8.0 Hz, 4-methoxyphenyl H-3, H-5), 7.41 (m, 3H, 4-phenyltriazole H-3, H-4, H-5), 7.58 (s, 2H, SO2NH2, D2O exchangeable), 7.68 (d, 2H, J = 7.6 Hz phenyl H-2, H-6), 7.87 (d, 2H, J = 8.4 Hz, 4-aminosulfonylphenyl H-2, H-6), 8.03 (d, 2H, J = 8.4 Hz, 4-aminosulfonylphenyl H-3, H-5), 11.83 (s, 1H, OH, D2O exchangeable); 13C NMR (DMSO-d6 100 MHz, δ ppm): 10.14, 55.90, 114.86, 125.99, 126.38, 126.67, 127.65, 129.00, 129.57, 129.65, 133.86, 134.78, 135.80, 138.20, 145.49, 148.51, 152.22, 152.41, 160.37; MS m/z (ES+) 576.14 (M+) (100%). Anal. Calcd. For C26H24N8O4S2: C, 54.15; H, 4.20; N, 19.43; Found; C, 54.34; H, 4.48; N, 19.70.
(E)-4-(4-(5-((2-(hydroxyimino)-2-(p-tolyl)ethyl)thio)-4-(4-methoxyphenyl)-4H-1,2,4-triazol-3-yl)-5-methyl-1H-1,2,3-triazol-1-yl)benzenesulfonamide (19h): Yield 81%; white solid; m.p. 212- 214 °C; IR (KBr): 3599 (OH), 3340, 3217 (NH2), 3020 (C–H aromatic), 2915 (C–H aliphatic), 1512 (C = N), 1342, 1168 (SO2NH2) cm1; 1H NMR (DMSO-d6, 400 MHz, δ ppm): 2.32 (s, 3H, phenyl-CH3), 2.59 (s, 3H, triazole-CH3), 3.81 (s, 3H, phenyl-OCH3) 4.44 (s, 2H, CH2), 7.02 (d, 2H, J = 8.4 Hz, 4-methoxyphenyl H-3, H-5), 7.20 (d, 2H, J = 8.4 Hz, 4-methoxphenyl H-2, H-6), 7.31 (d, 2H, J = 8.2 Hz, 4-methylphenyl H-2, H-6), 7.56 (s, 2H, SO2NH2, D2O exchangeable), 7.86 (d, 2H, J = 8.2 Hz 4-aminosulfonylphenyl H-2, H-6), 8.03 (d, 2H, J = 8.2 Hz 4-aminosulfonylphenyl H-3, H-5), 11.71 (s, 1H, OH, D2O exchangeable); 13C NMR (DMSO-d6 100 MHz, δ ppm): 10.11, 21.29, 55.90, 114.86, 125.99, 126.29, 126.66, 127.64, 128.55, 129.58, 129.87, 132.14, 133.26, 133.80, 135.76, 139.22, 144.84, 145.46, 148.14, 152.28, 160.36; MS m/z (ES+) 590.15 (M+) (100%). Anal. Calcd. For C27H26N8O4S2: C, 54.90; H, 4.44; N, 18.97; Found; C, 55.26; H, 4.68; N, 19.30.
(E)-4-(4-(5-((2-(hydroxyimino)-2-(4-methoxyphenyl)ethyl)thio)-4-(4-methoxyphenyl)-4H-1,2,4-triazol-3-yl)-5-methyl-1H-1,2,3-triazol-1-yl)benzenesulfonamide (19i): Yield 75%; white solid; m.p. 270 - 272 °C; IR (KBr): 3610 (OH), 3371, 3262 (NH2), 3059 (C–H aromatic), 2966 (C–H aliphatic), 1516 (C = N), 1300, 1165 (SO2NH2) cm1; 1H NMR (DMSO-d6, 400 MHz, δ ppm): 2.59 (s, 3H, triazole-CH3), 3.78 (s, 3H, phenyl-OCH3), 3.82 (s, 3H, 4-phenyl-triazole-OCH3), 4.43 (s, 2H, CH2), 6.95 (d, 2H, J = 8.2 Hz, 4-methoxyphenyl H-3, H-5), 7.02 (d, 2H, J = 8.2 Hz, 4-methoxyphenyl H-2, H-6), 7.32 (d, 2H, J = 8.2 Hz, 4-(4-methoxyphenyl) triazole H-2, H-6), 7.58 (s, 2H, SO2NH2, D2O exchangeable), 7.61 (d, 2H, J = 8.2 Hz, 4-(4-methoxyphenyl)triazole H-3, H-5), 7.86 (d, 2H, J = 8.0 Hz 4-aminosulfonylphenyl H-2, H-6), 8.03 (d, 2H, J = 8.0 Hz 4-aminosulfonylphenyl H-3, H-5), 11.60 (s, 1H, OH, D2O exchangeable); 13C NMR (DMSO-d6 100 MHz, δ ppm): 10.11, 25.76, 55.68, 55.70 114.42, 114.86, 125.99, 126.64, 127.11, 127.65, 127.78, 129.56, 133.84, 135.80, 138.19, 145.47, 148.47, 151.96, 152.36, 160.36, 160.53; MS m/z (ES+) 606.15 (M+) (100%). Anal. Calcd. For C27H26N8O5S2: C, 53.45; H, 4.32; N, 18.47; Found; C, 53.26; H, 4.64; N, 18.66.
(E)-4-(4-(5-((2-(4-chlorophenyl)-2-(hydroxyimino)ethyl)thio)-4-(4-methoxyphenyl)-4H-1,2,4-triazol-3-yl)-5-methyl-1H-1,2,3-triazol-1-yl)benzenesulfonamide (19j): Yield 86%; white solid; m.p. 233 - 235 °C; IR (KBr): 3607 (OH), 3340, 3217 (NH2), 3070 (C–H aromatic), 2978 (C–H aliphatic), 1512 (C = N), 1346, 1168 (SO2NH2) cm1; 1H NMR (DMSO-d6, 400 MHz, δ ppm): 2.59 (s, 3H, triazole-CH3), 3.82 (s, 3H, OCH3), 4.43 (s, 2H, CH2), 7.02 (d, 2H, J = 8.0 Hz 4-methoxyphenyl H-3, H-5), 7.32 (d, 2H, J = 8.0 Hz 4-methoxyphenyl H-2, H-6), 7.46 (d, 2H, J = 8.0 Hz 4-chlorophenyl H-2, H-6), 7.58 (s, 2H, SO2NH2, D2O exchangeable), 7.69 (d, 2H, J = 8.2 Hz, 4-chlorophenyl H-3, H-5), 7.86 (d, 2H, J = 8.2 Hz 4-aminosulfonylphenyl H-2, H-6), 8.03 (d, 2H, J = 8.2 Hz 4-aminosulfonylphenyl H-3, H-5), 11.92 (s, 1H, OH, D2O exchangeable); 13C NMR (DMSO-d6 100 MHz, δ ppm): 10.12, 25.79, 55.90, 114.84, 125.99, 126.66, 127.65, 128.18, 129.03, 129.57, 133.64, 133.84, 134.32, 135.82, 138.19, 145.50, 148.54, 151.59, 151.94, 160.36; MS m/z (ES+) 610.10 (M+) (100%). Anal. Calcd. For C26H23ClN8O4S2: C, 51.10; H, 3.79; N, 18.34; Found; C, 51.36; H, 3.98; N, 18.70.
(E)-4-(4-(5-((2-(hydroxyimino)-2-(4-nitrophenyl)ethyl)thio)-4-(4-methoxyphenyl)-4H-1,2,4-triazol-3-yl)-5-methyl-1H-1,2,3-triazol-1-yl)benzenesulfonamide (19k): Yield 83%; white solid; m.p. 288 – 290 °C; IR (KBr): 3614 (OH), 3321, 3298 (NH2), 3066 (C–H aromatic), 2982 (C–H aliphatic), 1516 (C = N), 1342, 1172 (SO2NH2) cm1; 1H NMR (DMSO-d6, 400 MHz, δ ppm): 2.58 (s, 3H, triazole-CH3), 3.81 (s, 3H, OCH3), 4.46 (s, 2H, CH2), 7.01 (d, 2H, J = 8.2 Hz 4-methoxyphenyl H-3, H-5), 7.32 (d, 2H, J = 8.2 Hz 4-methoxyphenyl H-2, H-6), 7.58 (s, 2H, SO2NH2, D2O exchangeable), 7.86 (d, 2H, J = 8.2 Hz, 4-nitrophenyl H-2, H-6), 7.94 (d, 2H, J = 8.2 Hz, 4-nitrophenyl H-3, H-5), 8.03 (d, 2H, J = 8.2 Hz 4-aminosulfonylphenyl H-2, H-6), 8.24 (d, 2H, J = 8.2 Hz 4-aminosulfonylphenyl H-3, H-5), 12.34 (s, 1H, OH, D2O exchangeable); 13C NMR (DMSO-d6 100 MHz, δ ppm): 10.12, 25.85, 55.89, 114.82, 124.14, 125.98, 126.68, 127.57, 127.66, 129.59, 133.84, 135.84, 138.18, 141.02, 145.51, 148.01, 148.61, 151.37, 151.58, 160.34; MS m/z (ES+) 621.12 (M+) (100%). Anal. Calcd. For C26H23N9O6S2: C, 50.23; H, 3.73; N, 20.28; Found; C, 50.38; H, 3.54; N, 20.52.
(E)-4-(4-(5-((2-(4-bromophenyl)-2-(hydroxyimino)ethyl)thio)-4-(4-methoxyphenyl)-4H-1,2,4-triazol-3-yl)-5-methyl-1H-1,2,3-triazol-1-yl)benzenesulfonamide (19 l): Yield 83%; white solid; m.p. 243 - 245 °C; IR (KBr): 3613 (OH), 3367, 3244 (NH2), 3066 (C–H aromatic), 2935 (C–H aliphatic), 1512 (C = N), 1333, 1165 (SO2NH2) cm1; 1H NMR (DMSO-d6, 400 MHz, δ ppm): 2.59 (s, 3H, triazole-CH3), 2.82 (s, 3H, OCH3), 4.42 (s, 2H, CH2), 7.02 (d, 2H, J = 8.2 Hz 4-methoxyphenyl H-3, H-5), 7.32 (d, 2H, J = 8.2 Hz 4-methoxyphenyl H-2, H-6), 7.58 (d, 2H, J = 8.2 Hz, 4-bromophenyl H-2, H-6), 7.62 (s, 2H, SO2NH2, D2O exchangeable), 7.64 (d, 2H, J = 8.4 Hz, 4-bromophenyl H-3, H-5), 7.86 (d, 2H, J = 8.4 Hz 4-aminosulfonylphenyl H-2, H-6), 8.03 (d, 2H, J = 8.4 Hz 4-aminosulfonylphenyl H-3, H-5); 13C NMR (DMSO-d6 100 MHz, δ ppm): 10.12, 25.76, 55.90, 114.84, 123.04, 125.99, 126.64, 127.68, 128.45, 129.56, 131.12, 131.94, 133.83, 135.84, 138.18, 145.47, 148.53, 151.69, 151.93, 160.36; MS m/z (ES+) 654.05 (M+) (100%). Anal. Calcd. For C26H23BrN8O4S2: C, 47.64; H, 3.54; N, 17.09; Found; C, 47.88; H, 3.74; N, 17.40.
Biological evaluation
In-vitro anti-inflammatory activity
The in vitro COX-1/COX-2 inhibition was determined using COX-1 inhibitor screening Kit-K548 (Biovision, S. Milpitas Blvd., Milpitas, CA 95035 USA) and COX-2 inhibitor screening Kit-K547 (Biovision, S. Milpitas Blvd., Milpitas, CA 95035 USA). The potency of the tested compounds was determined as the concentration causing 50% enzyme inhibition (IC50). In addition, the COX-2 selectivity indexes (S.I values) which are calculated as IC50 (COX-1)/IC50 (COX-2) were carried out and compared with that of the standard drugs celecoxibCitation69.
In-vitro cytotoxicity screening and IC50 determination
3-(4, 5-dimethylthiazol-2-yl)-2,5-diphenyltetrazolium (MTT) cell viability assay was done in all the three cancer cell lines MCF7, HCT116, Hep3b, A549, and F180. In brief, cells were seeded at 1 × 104 density per well in 96-well plate, in the next day cell were treated with a single dose of 10 µM from each compound. The wells without treatment were used as a control. After 48 h, media was removed and fresh media which contains 0.5 mg/mL thiazolyl blue tetrazolium bromide reagent (Sigma-Aldrich- St. Louis, MO, USA, M2128) was added. The cells where incubated for 3 h at 37 °C and 5% CO2, then DMSO (Sigma-Aldrich- St. Louis, MO, USA) was added to solubilise the formed formazan crystals and was taken at 570 nm using Varioskan Flash (Thermo Fisher Scientific, Waltham, MA, USA) microplate spectrophotometer. The half-maximal inhibitory concentration (IC50) of the most potent compounds was assessed using dose-response curve (GraphPad Prism software, version 8.00, Graph Pad software, Inc)Citation70,Citation71.
Cell cycle analysis
The effect of compounds 19c, 19f, 19h, 19 l on cell growth was determined using flow cytometry according to reported procedure.Citation72 Compounds 19c, 19f, 19h, 19 l were added on breast MCF7 cells with (IC50 = 14, 16, 15, and 9 μM, respectively) for 24h. Rinsed breast cells with phosphate buffer saline (PBS), centrifuged, 70% ethanol was added, cells were washed with PBS and suspended with 0.1 mg/mL RNase. Moreover, cells were marked with 40 mg/mL propidium iodide (PI) and cell cycle distribution was determined using FACS Calibur flow cytometer (Becton Dickinson). ()
Table 1. Cell cycle analysis of compounds 19c, 19f, 19h, 19 l and control for breast MCF7 cells.
Determination of apoptosis using Annexin-V
The apoptosis of compounds 19c, 19f, 19h, 19 l was determined using Annexin-V-FITC technique.Citation73,Citation74 Where these final compounds were added on MCF7 breast cells at their IC50 = 14, 16, 15, 9 μM, respectively, cells were incubated for 24h., collected, rinsed twice with PBS and marked with mixture of fluorescein isothiocyanate (FITC), Annexin-V and PI (propidium iodide) are left for 30 min at room temperature in the dark. Analysis was carried out using FACS Calibur flow cytometer (BD Biosciences, San Jose, CA, USA) according to the following procedure:
(1) Transfer the previously prepared cells from 4 °C to the bench-top and equilibrate to room temperature. (2) Gently re-suspend cells by inverting the tube or by gentle up and down pipetting. (3) Pellet the cells at 500 × g for 5 min. Carefully aspirate the supernatant without disrupting the pellet. It is better to incompletely remove the supernatant than to accidently aspirate part of the pellet and lose cells. (4) Wash the cells by gently suspending in 1 ml 1X PBS. Again, pellet the cells at 500 x g for 5 min and carefully remove the supernatant. (5) Gently suspend the cell pellet in 200 µL 1X Propidium Iodide + RNase Staining Solution. (6) Incubate at 37 °C in the dark for 20 – 30 min. (7) Place tubes on ice (still in the dark). (8) Gently suspend cells that settled during the incubation by pipetting up and down, pass cells through an appropriate filter to remove cell aggregates. (9) Run samples on flow cytometer, set appropriate FSC vs. SSC gates to exclude debris and cell aggregates. Collect Propidium iodide fluorescence intensity on FL2 of a flow cytometer and 488 nM laser excitation ().
Table 2. Apoptosis of compounds 19c, 19f, 19h, 19 l and control against breast MCF7 cells.
Aromatase inhibitory activity
Aromatase inhibitory activity was determined using aromatase (CYP19A) inhibitor screening Kit-K984 (Biovision, S. Milpitas Blvd., Milpitas, CA 95035 USA). IC50 of compounds 19c, 19f, 19h, 19 l were determined in MCF7 cells according to the following procedureCitation59, to create stock solutions, dissolve test substances in the appropriate solvent. Prepare a 5X solution of each required test concentration for each test substance by dilution in aromatase assay buffer. Reconstitute the Recombinant Human Aromatase stock (2X) with 1 ml of aromatase assay buffer to prepare it. To create a uniform solution, thoroughly vortex the contents before transferring the mixture to a 15 ml conical tube. Aromatase assay buffer should be used to raise the volume to 24.50 l, then 50 l of the NADPH generating system (100X) should be added for a final volume of 2.5 ml. Prepare reaction wells with test substances, corresponding no inhibitor controls (which may also serve as solvent controls), a background control (containing no fluorogenic aromatase substrate), and (if desired) a positive inhibition control using 5 M letrozole (5X solution, 1 M final concentration). Let the test ligands to interact with the aromatase by incubating the plate at 37 °C for at least 10 min. With the exception of the background control, add 30 l of the Aromatase Substrate/NADP+ (3X) mixture to each well to begin the reaction. This will result in a final reaction volume of 100 l/well. Measure the fluorescence at Ex/Em = 488/527 nm in kinetic mode for 60 min right away (within 1 s).
EGFR Kinase inhibitory activity
EGFR (Catalog # 40321) Kinase Assay Kit was used to determine EGFR kinase inhibitory activity of compounds 19c, 19f, 19h, 19 l according to reported procedureCitation75: (1) We add ATP, Poly (Glu:Tyr 4:1) and PTK substrate (10 mg/ml) in 5x Kinase Buffer 1.0 to achieve a 10 mM concentration then adding DTT to 5x Kinase Buffer 1.0; (2) The master mixture (25 l per well) was prepared as follows, 6 l (5x Kinase Buffer 1), 1 l of ATP (500 M), 1 l of the PTK substrate Poly (Glu:Tyr 4:1) (10 mg/ml), and 17 l of water are divided among N wells then Pour 25 l into each well, finally, Add 5 l of the "Test Inhibitor" well’s inhibitor solution. (3) We add 5 l of the identical solution devoid of an inhibitor to the "Positive Control" and "Blank" samples (Inhibitor buffer). (4) By combining 2400 ml of water with 600 ml of 5x Kinase Buffer 1, we can make 3 ml of 1x Kinase Buffer 1. For 100 reactions, 3 ml of 1x Kinase Buffer 1 are sufficient. The "Blank" wells should have 20 l of 1x Kinase Buffer 1 added to them. (5) On ice, we thaw the EGFR enzyme, when the tube containing the enzyme initially defrosts, quickly spin it to recover its entire contents and determine how much EGFR is needed for the test, then we diluted the enzyme with 1x Kinase Buffer 1 to 1 ng/l. At −80 °C, aliquot the remaining undiluted enzyme. (6) We Add 20 l of diluted EGFR enzyme to the "Positive Control" and "Test Inhibitor Control" wells to start the reaction. (7) We incubate for 40 min at 30 °C. Defrost the Kinase-Glo max solution, 50 l of the Kinase-Glo Max reagent should be added to each well following the 40 min reaction. (8) We Covered the plate with aluminium foil and incubated for 15 min at room temperature. (9) Finally, we use the microplate reader to measure luminescence.
B-RAF V600E kinases inhibitory activity
BRAFV600E (Catalog # 40533) Kinase Assay Kit was used to assess BRAFV600E kinase inhibitory activity of compounds 19c, 19f, 19h, 19 l according to the reported procedureCitation76: Defrost the ATP, 5X Raf substrate, and 5X Kinase Buffer. (Optional) To achieve a 10 mM concentration, add DTT to 5x Kinase Buffer 1 (e.g. add 10 l of 1 M DTT to 1 ml of 5x Kinase Buffer 1). the master mixture (25 l per well) as follows: 6 l of 5x Kinase Buffer 1, 1 l of ATP (500 mM), 10 l of 5X Raf substrate, and 8 l of water are divided among N wells. Pour 25 l into each well. Add 5 l of the "Test Inhibitor" well’s inhibitor solution. Add 5 l of the identical solution devoid of an inhibitor to the "Positive Control" and "Blank" samples (Inhibitor buffer). By combining 2400 ml of water with 600 ml of 5x Kinase Buffer 1, you can make 3 ml of 1x Kinase Buffer 1. For 100 reactions, 3 ml of 1x Kinase Buffer 1 are sufficient. The "Blank" wells should have 20 l of 1x Kinase Buffer 1 added to them. On ice, defrost the B-Raf(V600E) or B-Raf(WT) enzyme. When the tube containing the enzyme initially defrosts, quickly spin it to recover its entire contents. Determine how much B-Raf is needed for the test, and then diluted the enzyme with 1x Kinase Buffer 1 to 2 ng/l for B-Raf V600E or 2.5 ng/l for B-Raf WT. At −80 °C, aliquot the remaining undiluted enzyme. The freeze/thaw cycle is sensitive to B-Raf enzymes. Reduce the number of freeze-thaw cycles. Reusing thawed aliquots or diluted enzyme is not advised. Add 20 l of diluted B-Raf V600E or B-Raf WT enzyme to the wells labelled "Positive Control" and "Test Inhibitor Control" to start the reaction. 45 min at 30 °C of incubation. Defrost the Kinase-Glo Max solution. Add 50 l of the Kinase-Glo Max reagent to each well once the 45-min reaction has finished. Plate should be covered with aluminium foil and incubated for 15 min at room temperature. Use the microplate reader to measure luminescence.
Nitric oxide release measurement
To 2 ml of a 1:1 v/v combination of 50 mM phosphate buffer (pH 7.4) with methanol containing 5 × 1 0 −4 M of N-acetyl-cysteine, compounds 19c, 19f, 19h, and 19 l were dissolved in DMSO (20 ml). The medication had a final concentration of 1 0 −4 M. The tested solutions were maintained in an incubator at 37 °C for 1 h, and then 1 ml of the reaction mixture was treated with 250 ml of Griess reagent, which is composed of sulphanilamide (2 g), N-naphthylethylenediamine dihydrochloride (0.2 g), and 85% phosphoric acid in distilled water (final volume: 100 ml). The absorbance at a maximum wavelength of 540 nm was measured after 10 min. at room temperature. To create the calibration curve, sodium nitrite standard solutions (10–80 nmol/mL) were employed. The target compounds 19c, 19f, 19h, and 19 l were reacted with in various solutions using the identical conditions, but with 0.1 N HCl of pH 1 instead of pH 7.4 phosphate buffer. The percentage of NO released in relation to a theoretical maximum release of 1 mol NO/mol of test chemical was used to express the resultsCitation77.
Molecular docking study
Docking study was out using Molecular Operating Environment (MOE) program (2014) and all docking studies were carried out using enzyme downloaded from Protein Data Bank (PDB). The enzymes included in study were COX-2, EGFR, aromatase, and B-RAFV600E enzymes. The crystal structure of the reference drug celecoxib bound at the COX-2 active site obtained from protein data bank at Research Collaboration for Structural Bioinformatics (RSCB) protein data bank [PDB] (entry 3LN1), EGFR enzyme (PDB, ID: 1M17). Aromatase enzyme (PDB, ID: 3EQM) and B-RAFV600E enzyme (PDB, ID: 1UWJ). The preparation of the target derivatives for docking was done via their 3D structure built by MOE. Before docking, 3D protonation of the structures, running conformational analysis using systemic search, selecting the least energetic conformer and applying the same docking protocol used with ligand were done.
Results and discussion
Chemistry
The general reactions used for the preparation of the final target 1,2,4-triazole-NO hybrids 19a-l are outlined in Scheme 1. The 1,2,3-triazole acid hydrazide 13 and 1,2,3-triazole-N-phenylhydrazinecarbothioamides derivative 15a were synthesised according to previously reported procedureCitation66. The addition reaction of 4-substituted phenylisothiocyanate 14b to acid hydrazide 13 in ethanol resulted in formation of 1,2,3-triazole-N-phenyl hydrazinecarbothioamides derivative 15b. The two 1,2,3-triazole-N-phenyl hydrazinecarbothioamides derivative 15a,b underwent cyclisation into the cornerstone intermediates 5-mercapto-1,2,4-triazole 16a,b through heating in alcoholic basic medium. The characteristic NH appeared as a sharp peak at 3249–3251 cm−1 in IR spectra, also characteristic acidic SH proton appeared at 14.30–14.31 ppm in 1H NMR. The target triazole-NO hybrids 19a-l were synthesised from the key intermediates 18a-l through 2 steps; the first one includes reaction of 5-mercapto-1,2,4-triazole 16a,b derivatives with 4-substituted phenacyl bromide 17a-l for preparation of benzene sulphonamide derivatives 18a-l. The second step was through heating of intermediates 18a-l with hydroxylamine hydrochloride in acetonitrile. The structures of the intermediates (18a-l) and the target hybrids (19a-l) were fully confirmed using IR,1H NMR, DEPT-13C NMR, EI-MS and elemental analyses. For intermediates 18a-l, the characteristic CH2 protons signal in 1H NMR spectra of both the intermediate derivatives 18a-l and the final compounds 19a-l appeared at δ 4.92–5.03 ppm, 4.42–4.47 ppm, respectively. While final compounds 19a-l showed characteristic D2O exchangeable OH proton of oxime at 11.60–12.35 ppm, also OH group appeared in IR spectrum of final compounds 19a-l at 3618–2599 cm−1 with the disappearance of carbonyl peak of the intermediates 18a-l. In addition, DEPT-13C NMR spectra, showed the disappearance of the deshielded quaternary carbon of the intermediates 18a-l carbonyl group at 191.84–193.58 ppm and appearance of more shielded signal at 151.44–160.54 ppm corresponding to C = N of oximes 19a-l.
Biological evaluation
In-vitro anti-inflammatory activity
COX-1 Inhibitor Screening Kit-K548 (Biovision, S. Milpitas Blvd., Milpitas, CA 95035 USA) and COX-2 Inhibitor Screening Kit-K547 (Biovision, S. Milpitas Blvd., Milpitas, CA 95035 USA) were used. The potency of the screened compounds was estimated as the concentration causing 50% enzyme inhibition (IC50). The results () displayed that compounds 19a, 19b, 19d, 19e, 19f, 19 g, 19h, 19i, 19j, 19k had good inhibitory activities against COX-1 isozyme (IC50 = 3.43–8.60 μM range). On the other hand, Compounds 19c, 19f, 19h, 19 l exhibited excellent COX-2 isozyme inhibitory activities (IC50 = 0.243–0.329 μM range). From the above results, it can be concluded that compounds 19c, 19f, 19h, 19 l have outstanding COX-2 selectivity indexes in the range of 18.48 to 49.38 when compared to celecoxib (the COX-2 selective reference drug) (COX-1 IC50 = 8.78 μM, COX-2 IC50 = 0.416 μM and S.I. = 21.10) (Supplementary data S1).
Table 3. In vitro COX-1 and COX-2 inhibitory activity of triazole derivatives 19a-l and reference drug celecoxib.
In-vitro cytotoxicity screening and IC50 determination
Cell culture methedology: One cell line from four different types of cancers were used as models, Hep3b hepatocellular carcinoma cell line and MCF7 human breast adenocarcinoma cell line, both were purchased from Sigma-Aldrich (Labco). While HCT116 colorectal cancer, A549 non-small cell lung cancer and normal fibroblast F180 (bought from American type culture collection (ATCC) cell line was bought from American type culture collection (ATCC). HCT116 and MCF7 cells were cultured in RPMI-1640 medium (Sigma-Aldrich- St. Louis, MO, USA) supplied with 10% foetal bovine serum (Sigma-Aldrich- St. Louis, MO, USA) and 1% penicillin/streptomycin (Sigma-Aldrich- St. Louis, MO, USA). Hep3b was maintained in DMEM medium (Sigma-Aldrich- St. Louis, MO, USA), in which 10% foetal bovine serum (Sigma-Aldrich- St. Louis, MO, USA) and 1% penicillin/streptomycin (Sigma-Aldrich- St. Louis, MO, USA) were added to the medium. All cells were incubated at 37 °C and 5% CO2. were used to evaluate the anti-cancer activity of the newly synthesised compounds 19a–l.
Cell viability assay: 3-(4,5-dimethylthiazol-2-yl)-2,5-diphenyltetrazolium (MTT) cell viability assay was done in all the four cancer cell lines MCF7, HCT116, A549 non-small cell lung cancer and Hep3b. in brief, cells were seeded at 1 X 104 density per well in 96-well plate, in the next day cell were treated with a single dose of 10 µM from each compound. The wells without treatment were used as a control. After 48 h, media was removed and fresh media which contains 0.5 mg/mL thiazolyl blue tetrazolium bromide reagent (Sigma-Aldrich- St. Louis, MO, USA, #M2128) was added. The cells where incubated for 3 h at 37 °C and 5% CO2, then DMSO (Sigma-Aldrich- St. Louis, MO, USA) was added to solubilise the formed formazan crystals and OD was taken at 570 nm using Varioskan Flash (Thermo Fisher Scientific, Waltham, MA, USA) microplate spectrophotometer. The half-maximal inhibitory concentration (IC50) of the most potent compounds was assessed using dose-response curve (GraphPad Prism software, version 8.00, GraphPad software, Inc). IC50 of Compounds 19a–l was determined using the MTT cell viability assay (). Compounds 19c, 19f, 19h, 19 l showed good inhibitory activity against MCF-7 with IC50 = 9–16 μM compared to tamoxifen (IC50 = 27.9 μM) (MCF-7). On the other hand, compounds 19f, 19j, 19k, 19 l showed inhibitory activity against HEP-3B with IC50 = 4.5–14 μM compared to sorafenib with IC50 = 3.5 μM (HEP-3B). Moreover, derivatives 19e, 19j, 19k, 19 l showed good inhibitory activity against HCT116 with IC50 = 5.3–13.7 μM compared to 5-FU with IC50 = 4.8 μM (HCT116). As working with EGFR, Non-small cell lung cancer (A549) was used. Compounds 19c, 19f, 19h, 19 l showed excellent inhibitory activity against A549 with IC50 = 3–4.5 μM compared to 5-FU with IC50 = 6 μM (A549) ( and ).
Figure 3. Cell viability % of compounds 19a-l and reference compound single dose (10 μM) against MCF-7, HEP-38, HCT-116 and A549 cancer cell line.
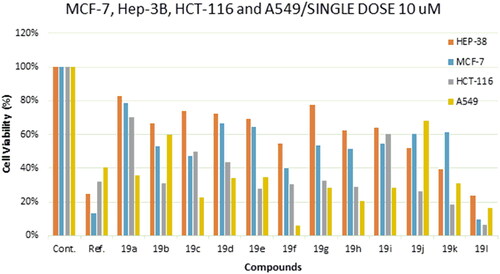
Figure 4. Compounds 19c, 19f, 19h, 19 l effect on DNA-ploidy flow cytometric analysis of breast MCF7 cells compared to negative control.
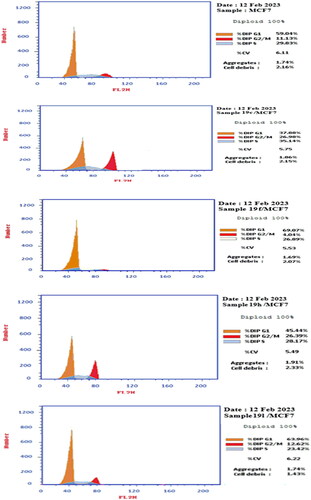
Figure 5. The percentage of Annexin-V-FITC-positive staining in breast MCF7 cells when treated with compounds 19c, 19f, 19h, 19 l compared to sorafenib and negative control.
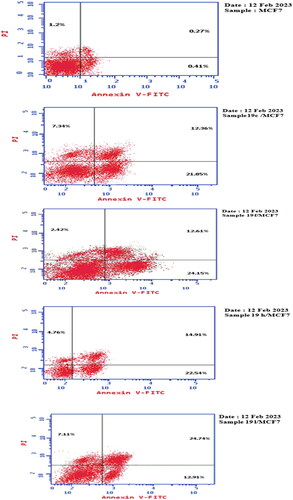
Figure 6. IC50% values of compounds 19c, 19f, 19h, 19 l compared to letrozole on aromatase against breast MCF7 cells.
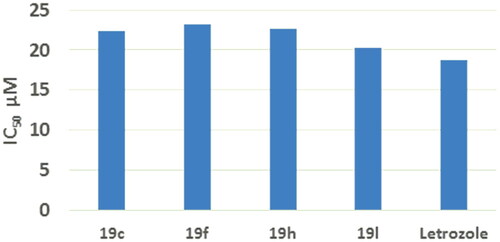
Table 4. In vitro MCF-7, HEP-3B, HCT-116, A549 and F180 inhibitory activity of triazole derivatives 19c,e,f,h,j-l and reference drugs doxorubicin, sorafenib, 5-F.U. and tamoxifen..
Cell cycle analysis
Cell cycle analysis of compounds 19c, 19f, 19h, 19 l were tested against breast cell line MCF7 at their IC50 concentrations, cell cycle phases alterations were noticed upon compounds 19c, 19f, 19h, 19 l addition on breast MCF7 cells (). It was noted that the percentage of cells upon adding compounds 19c, 19h at G0/G1 was decreased to 37.88% and 45.44%, respectively, in comparison to the control which showed 59.04%. While treating the same cells with 19f, 19 l caused in comparable increase in G0-G1 (69.07% and 63.69%, respectively). On the other hand, when treating breast cells MCF7 with compounds 19c, 19h, a dramatic increase in cells at G2/M phase was occurred to (26.98% and 26.39%, respectively) than control (11.13%). According to the displayed results, it can be concluded that compounds 19c, 19h showed cell cycle arrest at G2/M phase. On the other hand, compounds 19f, 19 l exerting their cell cycle arrest at G0-G1 phase ().
Determination of apoptosis using Annexin-V
Treating MCF7 breast cells with compounds 19c, 19f, 19h, 19 l resulted in an increment of annexin-V positive apoptotic cells at the early and late apoptotic stages with 21.85, 24.15, 22.54, 12.91% (early) (53.29, 58.90, 54.97, 31.48 fold) and 12.36, 12.61, 14.91, 24.74% (late) (45.77, 46.70, 55.22, 91.62 fold), respectively, compared to control (0.41% (early) and 0.27% (late), respectively). Moreover, compounds 19c, 19f, 19h, 19 l induced a percentage of necrosis 7.34%, 2.42%, 4.76%, and 7.11%, respectively, which were considerably higher than control that showed only 1.2% necrosis ().
Aromatase inhibitory activity
Aromatase (CYP19A) inhibitor screening Kit was used to determine aromatase inhibitory activity of compounds 19c, 19f, 19h, 19 l. The determination of IC50 was carried out using five concentrations and letrozole was used as reference. It was noticed that compounds 19c, 19f, 19h, 19 l induced strong inhibitory activity against aromatase with IC50 of 22.40, 23.20, 22.70 and 30.30 μM, respectively, which was comparable to that of letrozole (IC50 = 18.70 μM) () (Supplementary data S1).
EGFR kinase inhibitory activity
EGFR (Catalog # 40321) Kinase Assay Kit was used to evaluate EGFR kinase inhibitory activity of compounds 19c, 19f, 19h, 19 l. Five concentrations were used in this assay and encorafenib was used as reference for the determination of IC50. The results showed that compounds 19c, 19f, 19h, 19 l induced improved inhibitory activity against EGFR with IC50 of 0.112, 0.205, 0.169, and 0.066 μM, respectively, which was comparable to that of encorafenib (IC50 = 0.037 μM) () (Supplementary data S1).
B-RAF V600E kinases inhibitory activity
B-RAFV600E (Catalog # 40533) Kinase Assay Kit was used to assess B-RAFV600E kinase inhibitory activity of compounds 19c, 19f, 19h, 19 l. Five concentrations were used in this assay and encorafenib was used as reference for the determination of IC50. It was noted that compounds 19c, 19f, 19h, 19 l induced improved inhibitory activity against B-RAFV600E with IC50 of 0.09, 0.06, 0.07, and 0.05 μM, respectively, which was comparable to that of encorafenib (IC50 = 0.035 μM) () (Supplementary data S2).
Nitric oxide (NO) release
The percentage of NO released from the synthesised compounds 19a-l was determined using phosphate-buffered-saline (PBS at pH 7.4) through incubation (see data in ). All tested compounds 19a-l released NO in a slow rate (0.73–3.36%). Furthermore, derivatives 19c, 19f, 19h, 19 l were the highest NO donor (3.18%, 2.94%, 2.87%, and 3.36%, respectively).
Table 5. % of NO release of the synthesised compounds.
Molecular docking study
Molecular docking studies are used to predict protein-ligand interactions with different enzymes at the atomic levelCitation79. In this study, molecular docking studies of 1,2,3 triazole derivatives are docked with different enzymes downloaded from protein data bank (PDB) such as COX-2 enzyme (PDB, ID: 3LN1)Citation80, EGFR enzyme (PDB ID: 1M17)Citation81, aromatase enzyme (PDB, ID: 3EQM)Citation5 and B-RAF V600E enzyme (PDB ID: 1UWJ)Citation81.
Docking of triazole derivatives with COX-2 enzyme: The target triazole derivatives 19a-l were docked with COX-2 enzyme (PDB, ID; 3LN1). The native ligand Celecoxib showed an energy score − 9. 6 Kcal/Mol and the selected compounds 19c, 19f, 19h, 19l showed energy score of −8.1, −8.9, −8.5, and −8.2 Kcal/Mol, respectively. The higher selectivity index of such compounds may attributed to the presence of 1,2,3 triazole and 1,2,4 triazole which are hydrogen bond acceptor and sulphonamide moiety which is COX-2 selective moiety in addition these derivative contains NO2 and OCH3 moieties which can form hydrogen bond with amino acids of the target protein ( ).
Figure 9. (A) Visual representation (3D) of celecoxib docked with 3LN1 active site (B) Visual representation (3D) of compound 19c docked with 3LN1active site, (C) Visual representation (3D) of compound 19f docked with 3LN1 active site (D) Visual representation (3D) of compound 19h docked with 3LN1 active site (E) Visual representation (3D) of compound 19 l docked with 3LN1 active site.
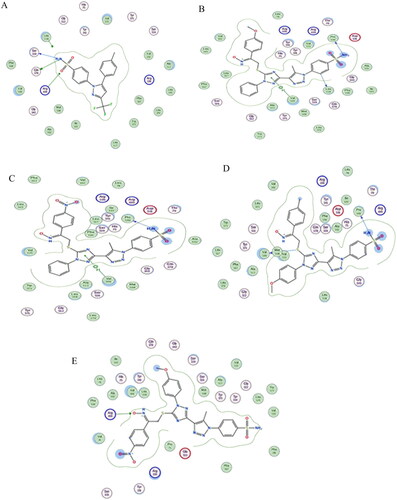
Table 6. Docking data of triazoles compounds 19a-l and celecoxib with COX-2 active site (3LN1).
Docking of triazole derivatives with EGFR enzyme: The 1,2,3 triazole 19a-l compounds and pyrazole EGFR inhibitor encorafenib were docked with EGFR enzyme (PDB, ID: 1M17). The ligand encorafenib showed an energy score − 7. 5 Kcal/Mol and the selected compounds 19c, 19f, 19h, 19l showed energy score of −8.7, −8.9, −8.8, and −8.8 Kcal/Mol respectively. The higher inhibitory activity of compounds 19c, 19f, 19h, 19 l to enzyme may attribute to the presence of higher number of nitrogen available for formation of hydrogen bond. They formed three or four H-bond interactions commonly with different amino acids (Lys721, Met769, Val702) amino acids residues through SO2NH2 or 1,2,3 triazole or 1,2,4 triazole or oxime nitrogen atoms. In addition to the formation of π … π stacking with aryl moieties or 1,2,3-triazole or 1,2,4-triazole rings of the synthesised compounds ( ).
Figure 10. (ِA) Visual representation (2D) of compound 19c docked with 1M17 active site, (B) Visual representation (2D) of compound 19f docked with 1M17 active site (C) Visual representation (2D) of compound 19h docked with 1M17 active site (D) Visual representation (2D) of compound 19 l docked with 1M17 active site (E)Visual representation (2D) of encorafenib docked with 1M17 active site (F) Visual representation (2D) of 4-anilinoquinazline docked with 1M17 active site.
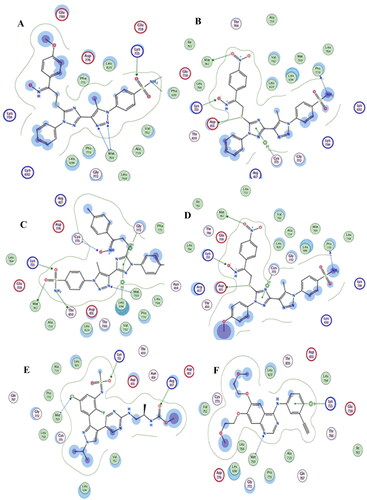
Table 7. Docking data of triazoles 19a-l and encorafenib with EGFR enzyme (1M17).
Docking of triazole derivatives with aromatase enzyme: The synthesised 1,2,3 triazole derivatives 19a-l and non-steroidal aromatase inhibitor letrozole were docked with aromatase enzyme (PDB, ID: 3EQM). The ligand letrozole showed an energy score − 7. 1 Kcal/Mol and the selected compounds 19c, 19f, 19h, 19l showed energy score of −11.3, −8.0, −10.2, and −8.8 Kcal/Mol, respectively. They formed three or four H bond interactions commonly with different amino acids (Met374, Leu372, Ala307) amino acids residues through SO2NH2 or 1,2,3 triazole or 1,2,4 triazole or oxime nitrogen atoms. In addition to the formation of π … π stacking with 1,2,3-triazole or 1,2,4- triazole rings of synthesised compounds with Hem 600 iron ( .
Figure 11. (A) Visual representation (3D & 2D) of letrozole docked with aromatase enzyme 3EQM active site (B) Visual representation (3D & 2D) of compound 19c docked with aromatase enzyme 3EQM active site, (C) Visual representation (3D & 2D) of compound 19f docked with aromatase enzyme 3EQM active site (D) Visual representation (3D & 2D) of compound 19h docked with aromatase enzyme 3EQM active site (E) Visual representation (3D & 2D) of compound 19 l docked with aromatase enzyme 3EQM active site.
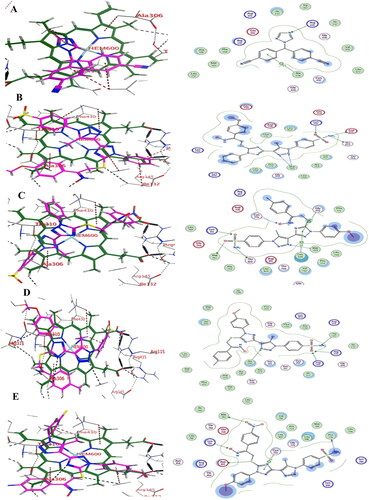
Table 8. Docking data of triazoles 19a-l and letrozole with aromatase enzyme (3EQM).
Docking of triazole derivatives with B-RAF V600E enzyme: The most active 1,2,3-triazole compounds 19c, 19f, 19h, 19 l were docked with kinase enzyme B-RAFV600E (PDB, ID: 1UWJ) active site. The selected compounds showed good energy score range (-7.4 to −8.3) incomparable with pyrazole B-Raf inhibitor encorafenib (-7.4) and sorafenib (-7.8). They formed three to four H-bonds and π … π stacking interaction with B-RafV600E enzyme through 1,2,3 triazole, 1,2,4 triazole, oxime moiety, SO2NH2 and phenyl moieties. They formed H-bond with amino acids like Asp593, Glu500, Val470 and Ser535 ( .
Figure 12. (A) Visual representation (2D) of compound 19c docked with enzyme B-RAFV600E (PDB entry 1UWJ) active site, (B) Visual representation (2D) of compound 19f docked with B-RAFV600E (C) Visual representation (2D) of compound 19h docked with B-RAFV600E (D) Visual representation (2D) of compound 19 l docked with B-RAFV600E (E)Visual representation (2D) of encorafenib docked with B-RAFV600E (F) Visual representation (2D) of Sorafenib docked with B-RAFV600E.
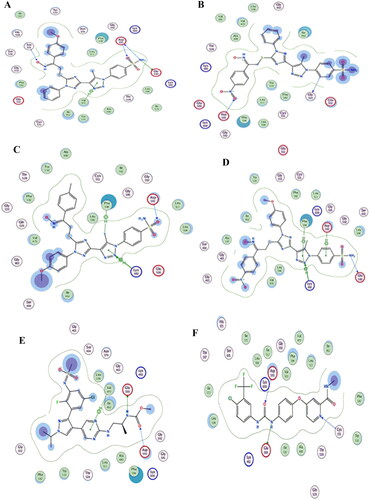
Table 9. Docking data of the most active triazoles 19c,f,h,l and encorafenib kinase enzyme B-RAFV600E (PDB, ID: 1UWJ) active site.
Statistical analysis
Differences between experimental groups were analysed using analysis of variance (ANOVA) followed by the Tukey’s test (p < 0.05) and all statistical procedures were done using Graph Pad Prism 7 for Mac OS X (Intuitive Software for Science, San Diego, CA, USA).
Authors’ contributions
Idea was created by Wael A. A. Fadaly. Synthesis of the compounds was carried out by Wael A. A. Fadaly and Mohamed T. M. Nemr. Discussion part was reedited by Fatma E. A. Mohamed and Marwa M. Abdelhakeem. Introduction part was reedited by Khaled R. A. Abdellatif. Docking studies were carried out by Taha H. Zidan. In vitro screening and IC50 determinations were performed by Nour N. Abu Jayab and Hany A. Omar. All the authors shared in writing, reviewing the manuscript in its final form.
Supplemental Material
Download PDF (863.9 KB)Acknowledgement
The authors thank Dr. Nada S. Abd El-Wahab, Pharmaceutical analytical Chemistry Department, Faculty of Pharmacy, Beni-Suef University, Egypt for carrying out nitric oxide release experiment.
Disclosure statement
The authors have declared no conflict of interest.
Additional information
Funding
References
- Othman EM, Fayed EA, Husseiny EM, et al. Apoptosis induction, PARP-1 inhibition, and cell cycle analysis of leukemia cancer cells treated with novel synthetic 1,2,3-triazole-chalcone conjugates. Bioorg Chem. 2022;123:1–15.
- Khalifa M, Allahloubi N, Salem S, Mahmoud M, Zekri A. Correlation between strength of estrogen receptor positivity and outcome of adjuvant tamoxifen therapy in breast cancer patients. Zagazig Univ Med J. 2020;0(0):0–0.
- Karn A, Jha AK, Shrestha S, Acharya B, Poudel S, Bhandari RB. Tamoxifen for breast cancer. J Nepal Med Assoc. 2010;49(177):62–67.
- Keely NO, Carr M, Yassin B, Ana G, Lloyd DG, Zisterer D, Meegan MJ. Design, synthesis and biochemical evaluation of novel selective estrogen receptor ligand conjugates incorporating an endoxifen-combretastatin hybrid scaffold. Biomedicines. 2016;4(3):15.
- Ghosh D, Griswold J, Erman M, Pangborn W. Structural basis for androgen specificity and oestrogen synthesis in human aromatase. Nature. 2009;457(7226):219–223.
- Leechaisit R, Pingaew R, Prachayasittikul V, et al. Synthesis, molecular docking, and QSAR study of bis-sulfonamide derivatives as potential aromatase inhibitors. Bioorganic Med Chem. 2019;27:1–10.
- Fantacuzzi M, De Filippis B, Gallorini M, et al. Synthesis, biological evaluation, and docking study of indole aryl sulfonamides as aromatase inhibitors. Eur J Med Chem. 2020;185:1–14.
- Lekgau K, Raphoko LA, Lebepe CM, et al. Design and synthesis of 6-amino-quinoxaline-alkynyl as potential aromatase (CYP19A1) inhibitors. J Mol Struct. 2022;1255:1–16.
- Geisler J. Differences between the non-steroidal aromatase inhibitors anastrozole and letrozole- of clinical importance. Br J Cancer. 2011;104(7):1059–1066.
- Giampietro L, Gallorini M, Gambacorta N, Ammazzalorso A, De Filippis B, Della Valle A, Fantacuzzi M, Maccallini C, Mollica A, Cataldi A, et al. Synthesis, structure-activity relationships and molecular docking studies of phenyldiazenyl sulfonamides as aromatase inhibitors. Eur J Med Chem. 2021;224:113737.
- Çetiner G, Acar U, Celik I, et al. New imidazole derivatives as aromatase inhibitor : Design, synthesis, biological activity, molecular docking, and computational ADME-Tox studies. 2023;1278.
- Zhou S, Liu Y, Jin L, Guo P, Liu Q, Shan J, Luo X, He H, Ma W, Zhang TCun, et al. Estrogen enhances the cytotoxicity of PARP inhibitors on breast cancer cells through stimulating nitric oxide production. J Steroid Biochem Mol Biol. 2021;209:105853.
- Sund M, Garcia-Argibay M, Garmo H, Ahlgren J, Wennstig A-K, Fredriksson I, Lindman H, Valachis A. Aromatase inhibitors use and risk for cardiovascular disease in breast cancer patients: A population-based cohort study. Breast. 2021;59:157–164.
- Karpov OA, Fearnley GW, Smith GA, et al. Receptor tyrosine kinase structure and function in health and disease. AIMS Biophys. 2015;2:476–502.
- Celik I, Ayhan‐Kılcıgil G, Karayel A, Guven B, Onay‐Besikci A. Synthesis, molecular docking, in silico ADME, and EGFR kinase inhibitor activity studies of some new benzimidazole derivatives bearing thiosemicarbazide, triazole, and thiadiazole. Journal of Heterocyclic Chem. 2022;59(2):371–387.
- Purba ER, Saita E-I, Maruyama IN. Mechanisms of activation of receptor tyrosine kinases: Monomers or dimers. Cells. 2014;6(2):304–330.
- Hubbard SR, Mohammadi M, Schlessinger J. Autoregulatory mechanisms in protein-tyrosine kinases. J Biol Chem. 1998;273(20):11987–11990.
- Butti R, Das S, Gunasekaran VP, Yadav AS, Kumar D, Kundu GC. Receptor tyrosine kinases (RTKs) in breast cancer: Signaling, therapeutic implications and challenges. Mol Cancer. 2018;17(1):34.
- Naishima NL, Faizan S, Raju RM. Design, synthesis, analysis, evaluation of cytotoxicity against MCF-7 breast cancer cells, 3D QSAR studies and EGFR, HER2 inhibition studies on novel Biginelli 1,4-Dihydropyrimidines. J Mol Struct. 2023;1277:1–23.
- Mitri Z, Constantine T, O'Regan R. The HER2 receptor in breast cancer: pathophysiology, clinical use, and new advances in therapy. Chemother Res Pract. 2012;2012:743193–743197.
- Mohmmed EA, Ramadan SS, EL-Saiid AS, Shousha WG. Frequency and clinical features of over-expressed her2 in Egyptian breast cancer women patients. Egypt J Hosp Med. 2021;85(1):3431–3435.
- Martínez-Sáez O, Prat A. Current and future management of HER2-Positive metastatic breast cancer. JCO Oncol Pract. 2021;17(10):594–604.
- Notarangelo T, Sisinni L, Condelli V, Landriscina M. Dual EGFR and BRAF blockade overcomes resistance to vemurafenib in BRAF mutated thyroid carcinoma cells. Cancer Cell Int. 2017;17(1):86.
- Tong CWS, Wu M, Cho WCS, To KKW. Recent advances in the treatment of breast cancer. Front Oncol. 2018;8:227.
- Pircher M, Winder T, Trojan A. Response to vemurafenib in metastatic triple-negative breast cancer harbouring a BRAF V600E mutation: a case report and electronically captured patient-reported outcome. Case Rep Oncol. 2021;14(1):616–621.
- Abdel-Maksoud MS, El-Gamal MI, Gamal El-Din MM, Oh CH. Design, synthesis, in vitro anticancer evaluation, kinase inhibitory effects, and pharmacokinetic profile of new 1,3,4-triarylpyrazole derivatives possessing terminal sulfonamide moiety. J Enzyme Inhib Med Chem. 2019;34(1):97–109.
- Halim PA, Sharkawi SMZ, Labib MB. Novel pyrazole-based COX-2 inhibitors as potential anticancer agents: design, synthesis, cytotoxic effect against resistant cancer cells, cell cycle arrest, apoptosis induction and dual EGFR/Topo-1 inhibition. Bioorg Chem. 2022;131:106273.
- Al-Wahaibi LH, Mohammed AF, Abdelrahman MH, Trembleau L, Youssif BGM. Citation and bgmy, trembleau. design, synthesis, and antiproliferative activity of new 5-chloro-indole-2-carboxylate and Pyrrolo[3,4-b]indol-3-one derivatives as potent inhibitors of EGFRT790M/BRAFV600E pathways lamya. Molecules. 2023;28(3):1269.
- Giordano S, Petrelli A. From single- to multi-target drugs in cancer therapy: when aspecificity becomes an advantage. Curr Med Chem. 2008;15(5):422–432.
- Katopodis P, Chudasama D, Wander G, Sales L, Kumar J, Pandhal M, Anikin V, Chatterjee J, Hall M, Karteris E. Kinase inhibitors and ovarian cancer. Cancers (Basel). 2019;11(9):1357.
- Vultur A, Villanueva J, Herlyn MM. BRAF inhibitor unveils its potential against advanced melanoma. Cancer Cell. 2010;18(4):301–302.
- Davies H, Bignell GR, Cox C, Stephens P, Edkins S, Clegg S, Teague J, Woffendin H, Garnett MJ, Bottomley W, et al. Mutations of the BRAF gene in human cancer. Nature. 2002;417(6892):949–954.,.
- Ho C-C, Liao W-Y, Lin C-A, Shih J-Y, Yu C-J, Yang JC-H. Acquired BRAF V600E mutation as resistant mechanism after treatment with osimertinib. J Thorac Oncol. 2017;12(3):567–572.
- Zhang J, Li Y, Chen S, Zhang L, Wang J, Yang Y, Zhang S, Pan Y, Wang Y, Yang Y. Systems pharmacology dissection of the anti-inflammatory mechanism for the medicinal herb folium eriobotryae. Int J Mol Sci. 2015;16(2):2913–2941.
- Abdellatif KRA, Eman KAÁ, Wael AA, Fadaly , Kamel GM. Synthesis, cyclooxygenase inhibition, anti- inflammatory evaluation and ulcerogenic chemistry. Med Chem Res. 2015;7
- Stankov SV. Definition of inflammation, causes of inflammation and possible anti-inflammatory strategies. Open Inflamm J. 2012;5:1–9.
- Abdellatif KRA, Elsaady MT, Amin NH. Design, synthesis and biological evaluation of some novel indole derivatives as selective COX-2 inhibitors. J Appl Pharm Sci. 2017;7:69–77.
- Dubios RN, Abrasmin SB, Crafford L, et al. Cyclooxygenase in biology and disease. Faseb J. 1998;12:1063–1073.
- Abdellatif KRA, Abdelall EKA, Labib MB, Fadaly WAA, Zidan TH. Design, synthesis of celecoxib-tolmetin drug hybrids as selective and potent COX-2 inhibitors. Bioorg Chem. 2019;90:103029.
- Abdellatif KRA, Fadaly WAA. Design, synthesis, cyclooxygenase inhibition and biological evaluation of new 1,3,5-triaryl-4,5-dihydro-1H-pyrazole derivatives possessing amino/methanesulfonyl pharmacophore. Bioorg Chem. 2017;70:57–66.
- Abdellatif KRA, Abdelgawad MA, Labib MB, Zidan TH. Synthesis, cyclooxygenase inhibition, anti-inflammatory evaluation and ulcerogenic liability of novel triarylpyrazoline derivatives as selective COX-2 inhibitors. Bioorg Med Chem Lett. 2015;25(24):5787–5791.
- Grosser T, Fries S, Fitzgerald GA. Biological basis for the cardiovascular consequences of COX-2 inhibition : therapeutic challenges and opportunities. J Clin Invest. 2006;116(1):4–15.
- Khaled RA, Abdellatif Eman KA, Abdelall MA, Abdelgawad MMA, Hany AO. Design and synthesis of certain novel arylidene thiazolidinone derivatives as anticancer agents. Derpharmachemica. 2015;7:149–161.
- Gupta V, Yadav S. Cyclooxygenase-2: pathway form anti-inflammatory to anti- cancer drugs. Int J Pharm Life Sci. 2011;2:571–582.
- Singh B, Lucci A. Role of cyclooxygenase-2 in breast cancer. J Surg Res. 2002;108(1):173–179.
- Koki AT, Masferrer JL. Celecoxib: a specific COX-2 inhibitor with anticancer properties. Cancer Control. 2002;9(2 Suppl):28–35.
- Harris RE. Cyclooxygenase-2 (cox-2) blockade in the chemoprevention of cancers of the colon, breast, prostate, and lung. Inflammopharmacology. 2009;17(2):55–67.
- Stolfi C, De Simone V, Pallone F, Monteleone G. Mechanisms of action of non-steroidal anti-inflammatory drugs (NSAIDs) and mesalazine in the chemoprevention of colorectal cancer. Int J Mol Sci. 2013;14(9):17972–17985.
- Mittal A, Dixit S, Rana S. Anticancer effects of chemotherapy and nature products. J Med Discov. 2017;2:1–9.
- Denkova AG, Liu H, Men Y, et al. Enhanced cancer therapy by combining radiation and chemical effects mediated by nanocarriers. Adv Ther. 2020;3:1–17.
- Fu S-L, Wu Y-L, Zhang Y-P, Qiao M-M, Chen Y. Anti-cancer effects of COX-2 inhibitors and their correlation with angiogenesis and invasion in gastric cancer. World J Gastroenterol. 2004;10(13):1971–1974.
- Abdelhaleem EF, Kassab AE, El-Nassan HB, Khalil OM. Design, synthesis, and biological evaluation of new celecoxib analogs as apoptosis inducers and cyclooxygenase-2 inhibitors. Arch Pharm (Weinheim)). 2022;355(11):e2200190.
- Abuo-Rahma GE-DAA, Abdel-Aziz M, Beshr EAM, Ali TFS. 1,2,4-Triazole/oxime hybrids as new strategy for nitric oxide donors: synthesis, anti-inflammatory, ulceroginicity and antiproliferative activities. Eur J Med Chem. 2014;71:185–198.
- Abuo-Rahma GE-DAA, Abdel-Aziz M, Mourad MAE, Farag HH. Synthesis, anti-inflammatory activity and ulcerogenic liability of novel nitric oxide donating/chalcone hybrids. Bioorg Med Chem. 2012;20(1):195–206.
- Zhang X, Ding J, Feng L, et al. Development of novel nitric oxide-releasing quinolinedione/furoxan hybrids as NQO1 inhibitors for intervention of drug-resistant hepatocellular cancer. Bioorg Chem. 2022;129:1–11.
- Shafran Y, Zurgil N, Ravid-Hermesh O, Sobolev M, Afrimzon E, Hakuk Y, Shainberg A, Deutsch M. Nitric oxide is cytoprotective to breast cancer spheroids vulnerable to estrogen-induced apoptosis. Oncotarget. 2017; 8(65):108890–108911.
- Eissa AG, Barrow D, Gee J, Powell LE, Foster PA, Simons C. 4th Generation nonsteroidal aromatase inhibitors: an iterative SAR-guided design, synthesis, and biological evaluation towards picomolar dual binding inhibitors. Eur J Med Chem. 2022;240:114569.
- Fadaly WAA, Elshaier YAMM, Hassanein EHM, et al. New 1,2,4-triazole/pyrazole hybrids linked to oxime moiety as nitric oxide donor celecoxib analogs: Synthesis, cyclooxygenase inhibition anti-inflammatory, ulcerogenicity, anti-proliferative activities, apoptosis, molecular modeling and nitric oxide release. Bioorg Chem. 2020;98:1–20.
- Fadaly WAA, Elshaier YAMM, Nemr MTM, et al. Bioorganic chemistry design, synthesis, modeling studies and biological evaluation of pyrazole derivatives linked to oxime and nitrate moieties as nitric oxide donor selective COX-2 and aromatase inhibitors with dual anti-inflammatory and anti-neoplasti. Bioorg Chem. 2023;134:106428.
- Duan Y-C, Ma Y-C, Zhang E, Shi X-J, Wang M-M, Ye X-W, Liu H-M. Design and synthesis of novel 1,2,3-triazole-dithiocarbamate hybrids as potential anticancer agents. Eur J Med Chem. 2013;62:11–19.
- Becerril JLM, Benítez JGS, Juárez JJT, Bañales JMG, Zerón HM, Navarro MDH. Evaluation of the effect of 1,3-Bis(4-Phenyl)-1H-1,2,3-Triazolyl-2-propanolol on gene expression levels of JAK2–STAT3, NF-κB, and SOCS3 in cells cultured from biopsies of mammary lesions. Biochem Genet. 2015;53(11-12):291–300.
- Hou Y-P, Sun J, Pang Z-H, Lv P-C, Li D-D, Yan L, Zhang H-J, Zheng EX, Zhao J, Zhu H-L, et al. Synthesis and antitumor activity of 1,2,4-triazoles having 1,4-benzodioxan fragment as a novel class of potent methionine aminopeptidase type II inhibitors. Bioorg Med Chem. 2011;19(20):5948–5954.
- Kaoud TS, Mohassab AM, Hassan HA, et al. NO-releasing STAT3 inhibitors suppress BRAF-mutant melanoma growth. Eur J Med Chem. 2020;186:1–65.
- AboulWafa OM, Daabees HMG, El-Said AH. Benzoxazole-appended piperidine derivatives as novel anticancer candidates against breast cancer. Bioorg Chem. 2023;134:106437.
- Kouznetsov VV, Gómez-Barrio A. Recent developments in the design and synthesis of hybrid molecules basedon aminoquinoline ring and their antiplasmodial evaluation. Eur J Med Chem. 2009;44(8):3091–3113.
- Bekheit MS, Mohamed HA, Abdel-Wahab BF, Fouad MA. Design and synthesis of new 1,4,5-trisubstituted triazole-bearing benzenesulphonamide moiety as selective COX-2 inhibitors. Med Chem Res. 2021;30(5):1125–1138.
- Abdelrazek FM, Hassaneen HM, Nassar EM. Phenacyl bromides revisited: facile synthesis of some new pyrazoles, pyridazines, and their fused derivatives. J Heterocycl Chem. 2011;46:475–481.
- Akbarzadeh A, Soleymani R, Taheri M, Rajabzadeh H, Daryani M. Synthesis and studies of potential antifungal and antibacterial agents new aryl thiazolyl mercury (II) derivatives compounds. J Chem. 2013;2013:1–6.
- Hussein AHM, Khames AA, El-Adasy A-BA, Atalla AA, Abdel-Rady M, Hassan MIA, Nemr MTM, Elshaier YAAM. Design, synthesis and biological evaluation of new 2-aminothiazole scaffolds as phosphodiesterase type 5 regulators and COX-1/COX-2 inhibitors. RSC Adv. 2020;10(50):29723–29736.
- Omar HA, Arafa E-SA, Salama SA, Arab HH, Wu C-H, Weng J-R. OSU-A9 inhibits angiogenesis in human umbilical vein endothelial cells via disrupting Akt-NF-κB and MAPK signaling pathways. Toxicol Appl Pharmacol. 2013;272(3):616–624.
- Nemr MTM, AboulMagd AM, Hassan HM, Hamed AA, Hamed MIA, Elsaadi MT. Design, synthesis and mechanistic study of new benzenesulfonamide derivatives as anticancer and antimicrobial agentsviacarbonic anhydrase IX inhibition. RSC Adv. 2021;11(42):26241–26257.
- Zaher AF, Abuel-Maaty SM, El-Nassan HB, Amer SAS, Abdelghany TM. Synthesis, antitumor screening and cell cycle analysis of novel benzothieno[3,2-b]pyran derivatives. J Enzyme Inhib Med Chem. 2016;31(suppl 4):145–153.
- Ibrahim TS, Hawwas MM, Malebari AM, Taher ES, Omar AM, Neamatallah T, Abdel-Samii ZK, Safo MK, Elshaier YAMM. Discovery of novel quinoline-based analogues of combretastatin A-4 as tubulin polymerisation inhibitors with apoptosis inducing activity and potent anticancer effect. J Enzyme Inhib Med Chem. 2021;36(1):802–818.
- Shawky AM, Ibrahim NA, Abdalla AN, Abourehab MAS, Gouda AM. Novel pyrrolizines bearing 3,4,5-trimethoxyphenyl moiety: design, synthesis, molecular docking, and biological evaluation as potential multi-target cytotoxic agents. J Enz Inhib. 2021;36(1):1312–1332.
- Nakamura JL. The epidermal growth factor receptor in malignant gliomas: pathogenesis and therapeutic implications. Expert Opin Ther Targets. 2007;11(4):463–472.
- Obaid NM, Bedard K, Huang WY. Strategies for overcoming resistance in tumours harboring BRAF mutations. Int J Mol Sci. 2017;18(3):585.
- Wink DA, Ridnour LA, Hussain SP, Harris CC. The reemergence of nitric oxide and cancer. Nitric Oxide. 2008;19(2):65–67.
- Abdellatif KRA, Belal A, Omar HA. Design, synthesis and biological evaluation of novel triaryl (Z)-olefins as tamoxifen analogues. Bioorg Med Chem Lett. 2013;23(17):4960–4963.
- Çetiner G, Acar Çevik U, Celik I. New imidazole derivatives as aromatase inhibitor: design, synthesis, biological activity, molecular docking, and computational ADME-Tox studies. J Mol Struct. 2023;1278:1–9.
- Wang JL, Limburg D, Graneto MJ, Springer J, Hamper JRB, Liao S, Pawlitz JL, Kurumbail RG, Maziasz T, Talley JJ, et al. The novel benzopyran class of selective cyclooxygenase-2 inhibitors. Part 2: The second clinical candidate having a shorter and favorable human half-life. Bioorg Med Chem Lett. 2010;20(23):7159–7163.
- Stamos J, Sliwkowski MX, Eigenbrot C. Structure of the epidermal growth factor receptor kinase domain alone and in complex with a 4-anilinoquinazoline inhibitor. J Biol Chem. 2002;277(48):46265–46272.