ABSTRACT
An experimental campaign was carried out to study the passivity of steel embedded in blended cement paste. Mockup samples made of steel coupons (milled or pre-corroded) embedded in cement paste were tested under controlled relative humidity or in a solution simulating clay underground water and under aerated and de-aerated conditions, during up to 3 years. The results of gravimetric measurements show that the average corrosion rates determined on milled coupons are lower than 1 µm/year and vary from 0.2 to 2.7 µm/year for pre-corroded coupons. An apparent passive corrosion state is reached rapidly whatever the environmental conditions. Moreover, solid characterisations (Raman spectroscopy and SEM-EDS) point to a stable state in terms of the nature of the corrosion products and of the composition of the steel/cement paste interface. These results are similar to the ones previously obtained for steel coupons embedded in Portland cement mortar.
This paper is part of a supplement on the 6th International Workshop on Long-Term Prediction of Corrosion Damage in Nuclear Waste Systems.
1. Introduction
In the framework of the current French disposal concept for intermediate level radioactive waste, containers and engineered barriers are to be built with reinforced concrete [Citation1]. Blended cements may be used. Owing to the long-term implications of these applications (up to several hundreds of years), a specific interest in passive corrosion arises. In the high alkalinity of the interstitial solution of Portland concrete, the passive corrosion of carbon steel is promoted by the formation of a thin protective layer. The stability of this layer depends highly on the solution chemistry (pH, buffering effect, carbonate and sulfate content) [Citation2]. The passive film is often described as a double layer composed of a continuous inner layer and a more loosely bound outer layer [Citation3,Citation4]. The composition of the passive film has been experimentally characterised [Citation5Citation6 Citation7 Citation8 Citation9–Citation10]. Several authors reported the presence of Fe(II) in the inner layer [Citation5Citation6–Citation7]. The passive film has also been described as a magnetite like structure [Citation9] or composed of oxyhydroxyde and maghemite [Citation10]. The behaviour of steel embedded in blended cement is not so well known. The electrochemical study of corrosion resistance in ternary mixes (incorporating slag and fly ash) indicates that a passive state is reached and even suggests a lower corrosion rate after passivation compared to Ordinary Portland Cement mortar [Citation11]. In contrast, the electrochemical impedance spectrometry (EIS) response of steel electrodes immersed in synthetic pore solution of a blast furnace slag cement (CEM III type, according to European standards) presents an active behaviour at 5 days [Citation12]. The presence of sulfide in pore solution of slag cement could be responsible for this behaviour by inhibiting the formation of the passive oxide layer or modifying its composition [Citation13Citation14–Citation15]. The presence of sulfide and unburnt carbon in fly ash could also have a deleterious effect on corrosion resistance [Citation16,Citation17]. It seems that the passive corrosion state is reached depending on the activation process used for fly ash and/or the environmental conditions [Citation18].
This corrosion study focusses on a ternary mix with slag and fly ash (CEM V cement type). It follows previous work on the passive corrosion of steel embedded in Portland cement (CEM I) [Citation19,Citation20]. The present paper sums up the analytical results (gravimetric measurements, Raman spectroscopy and SEM-EDS) obtained on steel coupons embedded in blended cement paste and aged in various environmental conditions (clay rock environment, water saturated or partially saturated and aerated or de-aerated conditions) during up to 3 years.
2. Materials and methods
2.1. Materials
The mockups consist of a steel coupon (16 mm diameter and 5 mm height) embedded in the centre of a cement paste cylinder (50 mm diameter and 39 mm height or 40 mm diameter and 29 mm height). The coupons are milled from mild steel rebar. These samples are used as sliced (rough material) or thermally pre-corroded (400°C for 64 h). The thermal pre-corrosion allow us to obtain a thin homogeneous oxide layer representative of the mill scale composition (mainly magnetite). Mill scale is an oxide layer that is formed on the steel reinforcement during its manufacturing process at high temperature.
The cement paste is prepared with a blended cement type CEM V/A (S-V) from Calcia Company (Airvault factory), mixed with distilled water (w/c = 0.318). The composition of CEM V is presented in . The samples were cured for 28 days in their mould in a room at 20°C and high relative humidity (about 90%). For de-aerated conditions, the mockups are cured at 100% of relative humidity in a closed reactor initially filled with nitrogen gas.
Table 1. Chemical composition of the CEM V (all values are in mass %).
2.2. Experimental conditions
Mockups containing milled or pre-corroded steel coupons were tested under nine different environmental conditions, which are detailed in . Saturated salt solutions (KBr, KNO3 and K2SO4) were used to control the relative humidity (80, 90 and 95%, respectively). Experiments in controlled relative humidity were performed in plastic desiccator cabinets with no measurement of oxygen concentration in the gas phase. The composition of the solution simulating the clay underground water is based on the calculated formulation presented by Gaucher et al. [Citation21]. Experiments in an de-aerated environment were carried out in glove boxes, where the oxygen concentration was lower than 10 ppm in the gas phase of gloves box. One exception was the experiment in a synthetic clay pore water at 50°C, which was performed in a specific device which is similar to the one described by Martin et al. [Citation22,Citation23]. This device is composed of four stainless steel cells containing the mockups. At the beginning of the experiment, these cells are filled with synthetic Bure water at 50°C. The temperature is regulated through immersion of the cells in an oil bath at 50°C.
Table 2. Test conditions in terms of aerated (AE) or de-aerated (DE) environment, temperature, relative humidity (RH) and the salt used (to fix the RH) or synthetic clay pore water (W).
2.3. Analytical methods
Characterisations were performed after few months to 3 years of simulated aging. Mockups dedicated to surface analysis (Raman spectroscopy and SEM-EDS) were cut and coated in epoxy resin in order to prepare cross-sections showing the steel/cement paste interface.
2.3.1. Gravimetric measurements
Corrosion products were removed from the steel surface by chemical attack. The solution used was a mix of hydrochloric acid, antimony trioxide and tin(II) chloride [Citation24]. The difference between the initial mass (weight before pre-corrosion) and the final one (weight after experiment and cleaning) gives the total steel mass loss. In the case of pre-corroded steel coupons, this mass loss must be corrected to take into account the mass loss associated to the corrosion products produced during the pre-corrosion treatment. The latter is considered to be 5.2 mg with a standard deviation of 0.5 (value deduced from gravimetric measurements performed on 10 pre-corroded samples) for a steel coupon of 6.9019 ± 0.0202 g. The balance precision is 10−4 g. On the basis of these measurements, the average corrosion rate is estimated considering a uniform corrosion phenomenon.
2.3.2. Micro-Raman spectroscopy
The structural identification of the corrosion products was performed by means of micro-Raman spectroscopy. The spectrometer is a Horiba-Jobin Yvon X’Plora, and the frequency of the laser used is 532 nm. Microanalyses were performed with a 100× Olympus objective, giving an analysed area of about 2 µm in diameter. The laser beam is filtered at 1% or 10% of the nominal power in order to prevent potential thermal transformation of sensitive iron phases [Citation25]. Spectra identification is achieved by comparison with reference spectra given by earlier publications [Citation26Citation27–Citation28].
2.3.3. Scanning electron microscopy and energy dispersive spectroscopy
Chemical compositions of corrosion products and hydrated cement were characterised with three different scanning electron microscopes. Two SEMs with a tungsten filament are used: a LEO 120, from Cambridge Instruments, associated with SAMx Max View software (version 4.1.3) and an EVO MA15, from Zeiss, with a Brucker detector and ESPRIT software. The accelerating voltage used is 15 kV. The last one is a field emission gun equipped with a hot cathode (MEB-FEG 7000F, JEOL). The acquisition system is a Bruker SDD detector (silicon dry diode) associated with ESPRIT software. Oxygen quantification is made by the use of Si(Li) detectors equipped with a thin beryllium window (approximately 2 wt-% relative error on iron oxides standards). The lower detection limit is of 0.5 wt-% for elements heavier than aluminium and several per cent for oxygen.
3. Results
3.1. Average corrosion rates
Average corrosion rates were estimated from the gravimetric measurement performed on steel coupons embedded in blended cement paste and tested under different conditions (). For milled coupons, the average corrosion rates decreased from a maximum value of 0.7 to a value lower than 0.1 µm/year, whatever the experimental conditions and duration ((a) and (b)). This decrease is artificial as the mass loss varies erratically from 0.1 to 0.6 mg for all conditions and durations.
Figure 1. The average corrosion rate for sample containing a milled (a and b) or pre-corroded (c and d) steel coupon embedded in CEM V cement paste and tested under different environmental conditions (). (a) and (c) Results from mockups tested under aerated conditions and relative humidity of 80% (AE-RH 80), 90% (AE-RH 90) and 95% (AE-RH 95) or in de-aerated conditions and a relative humidity of 80% (DE-RH 80) and 95% (DE-RH 95). (b) and (d) Results from mockups tested in Bure site water and aerated conditions at 25°C (AE-W 25) and 50°C (AE-W 50) or deaerated conditions at 25°C (DE-W 25) and 50°C (DE-W 50).
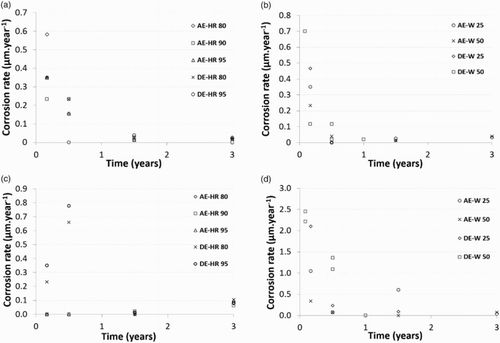
Table 3. Mass loss in milligrams deduced for gravimetric measurement for all tested conditions at different durations.
For pre-corroded steel coupons, the determination of corrosion rate is more difficult as the pre-corrosion treatment has to be taken into account. For mockups tested under humid atmospheres, the mass loss measurements show ambiguous evolution with time, and the mass losses measured (5.7 ± 0.9 mg) are close to the one associated to pre-corrosion treatment. After three years of aging, the average corrosion rates are close to 0.1 µm/year ((c) and (d)). For mockups immersed in synthetic clay pore water, the mass losses are globally higher (6.5 ± 1.4 mg). This led to the deduction of average corrosion rates higher than 1 µm/year during the first months of aging in de-aerated conditions. Afterwards, the average corrosion rates rapidly decreased below 1 µm/year.
Whatever the environmental conditions, a corrosion state typical of passive corrosion, but without any clear evidence of the existence of a passive corrosion layer, is reached. The similar behaviour observed for different aeration conditions (aerated/deaerated) could suggest an influence of the dissolved gaseous oxygen initially introduced in the mockups during manufacturing (about 4.7 × 10−6 mol per mockup). The curing conditions in a nitrogen atmosphere associated with the presence of sulfide, coming from CEM V, should ensure a significant consumption of this oxygen entrapped in the pore volume. The remaining oxygen is consumed by corrosion, as well as diffused out of the sample. In synthetic clay pore water at 25°C, the diffusion of oxygen in a saturated porosity (about 20%) is very slow for such a CEM V cement paste (the diffusion coefficient is about 2–5 × 10−13 m2/s) [Citation29], and the transport of oxygen could be a concern. In unsaturated medium, the transport of oxygen will rapidly increase with the decrease in relative humidity (or water saturation degree). In the range of 1 to 0.9 in water saturation degree, the hydrogen diffusion coefficient increases by one order of magnitude compared to the diffusion coefficient in solution [Citation30,Citation31]. On the basis of all these observations, an anoxic environment is expected to be promoted in mockups tested in deaerated conditions at more or less long term.
Comparable conclusions were drawn during a previous study relative to mockups made with Portland mortar [Citation20]. For comparison, the corrosion rate associated to archaeological analogues tested in deuterated iron hydrocarbonate solution under anoxic conditions is lower than 2 µm/year [Citation32,Citation33].
3.2. Nature of corrosion products
Raman analyses were performed on mockups containing a pre-corroded steel coupon to identify the type of corrosion products. The corrosion products remain almost the same as the initial corrosion products (mainly magnetite with a little hematite), whatever the aging conditions and duration ((a)). Hematite is always observed with magnetite. The identification of maghemite in a humid atmosphere and in aerated conditions at 6 months of aging would suggest a slow conversion of magnetite into maghemite ((b)). This phenomenon could be induced by oxidation of Fe2+ into Fe3+ by oxygen. It has not been observed for the longest test durations (18 months or 3 years) and has to be confirmed.
3.3. Steel/mortar interface
SEM-EDS analyses of the steel/cement paste interface highlighted two different kinds of corrosion patterns. The first typical corrosion pattern is observed on pre-corroded coupons. The interface is characterised by a succession of two layers: a corrosion product layer – more or less dense – at the surface of the coupon followed by an iron-enriched layer (transition zone between the corrosion product layer and the sound cementitious material) and finally the unaltered part of the cement paste ((b)). The thickness of the corrosion product layer varies from 1.5 to 6 µm. These values are in the same range as those determined for the initial corrosion products formed during the pre-corrosion treatment. The second typical corrosion pattern is found in mockups containing milled steel coupons ((a)). This pattern is characterised by an iron-enriched layer directly at the surface of the milled steel coupons. In the two different kinds of corrosion patterns, an iron-enriched layer is detected. This iron enrichment is associated to a relative depletion of calcium and silicon. Whatever the environmental conditions and durations, the thickness of the iron-enriched layer remains lower than some tens of micrometres. This thickness does not increase with aging time. It must be noted that the limit between the iron-enriched layer and the unaltered cement paste can be difficult to determine due to the heterogeneous distributions of the iron in the unaltered part of the cement paste (according to the different compositions of slag, fly ash and clinker). This kind of corrosion pattern has also been identified in mockups made with Portland cement [Citation20].
Figure 3. Elementary composition (wt-%), in a function of the distance from the surface of the steel coupon, of the principal elements: oxygen (O), silicon (Si), calcium (Ca) and iron (Fe). The SEM-EDS analyses are performed (a) on sample containing a milled steel coupon tested under de-aerated conditions and immersed in Bure site water at 50°C for an aging time of 1 year as well as (b) on a pre-corroded steel coupon embedded in cement and tested under aerated conditions and a relative humidity of 95% for an aging time of 18 months.
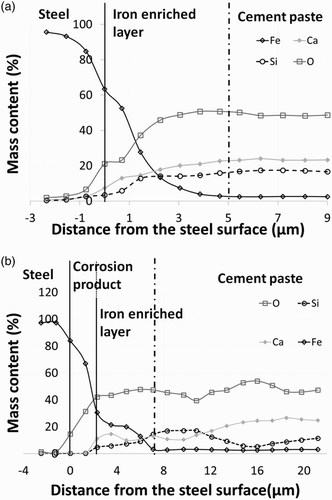
4. Discussion: influence of binder
The micro-Raman and SEM analyses show that the corrosion product layer is stable in terms of the nature of corrosion products (magnetite and more rarely hematite) and thickness (some of micrometres). The same conclusion can be drawn for the thickness of the iron-enriched layer. These observations are in accordance with the passive corrosion state defined by low corrosion rates (≤0.1 µm/years after 3 years). These results are similar to the ones deduced from experiments carried out on mockups of steel coupons embedded in a Portland mortar [Citation20]. This observation seems to indicate that, within a short-term observation (up to 3 years), the use of cement CEM V instead of cement CEM I has no significant influence on the corrosion rate reached. The later could be referred as an apparent passive state. Indeed, the formation of a passive film on the surface of the steel is not evidenced in this study but all the corrosion rates estimated are typical of passive corrosion behaviour.
These conclusions are in accordance with the electrochemical study of the resistance of corrosion of ternary mixes (Portland cement, slags and fly ash) [Citation11]. The presence of sulfide in slag and fly ash would have suggested another behaviour [Citation13,Citation14,Citation16]. The deleterious effect of sulfide on corrosion has to be explained by two different mechanisms. The release of reduced species, coming from slag, would consume oxidant so that the formation of the passive oxide layer would be inhibited [Citation14]. This effect is expected to be maintained until sulfide is entirely oxidised. On the other hand, Tromans suggests that the incorporation of sulfide in the passive oxide layer would yield a non-protective layer [Citation15].
In slag cement (up to 75 wt-% of slag), the corrosion current measured just after the curing period is 10 times higher than the one for pure Portland mortar [Citation13]. But this strong influence disappeared with time, after which a passivation trend is observed. The sulfide present in fly ash could have a deleterious effect or not, depending on the method of activation and possibly under environmental conditions [Citation17,Citation18].
In this study, the amount of sulfide in CEM V is about 0.17 wt-% (). This amount could have been too low to have a significant impact on passive corrosion state. The reducing properties of CEM V are potentially not high enough to significantly counteract the oxidative protection of steel.
5. Conclusion
Corrosion studies were performed on steel coupons embedded in blended cement paste (CEM V) aged under different environmental conditions (under a relative humidity of 80, 90 and 95% or immersed in a solution synthetic clay pore water solution (at 25 and 50°C)), in both cases under aerated and de-aerated conditions. Tests were carried out for a duration of 1 month to 3 years. It was found that:
Whatever the environmental conditions, the average corrosion rates are typical of an apparent passive corrosion state after 1 year. A decrease of one order of magnitude of the corrosion rates during the first few months was observed.
The nature of the corrosion products and the thickness of the corrosion product layer present at the surface of the pre-corroded steel coupons do not seem to significantly evolve with time.
The steel coupon/cement paste interface shows iron enrichment. The thickness of the iron-enriched layer is about some tens of micrometres and does not seem to evolve significantly up to 3 years aging.
These results are similar to the ones previously obtained for steel coupons embedded in Portland cement mortar. This observation suggests that the use of blended cement such as CEMV, instead of Portland cement, does not affect significantly the apparent passive corrosion state at intermediate term (up to 3 years).
Disclosure statement
No potential conflict of interest was reported by the author.
Additional information
Funding
References
- Andra. ‘Dossier 2005, les recherches de l’Andra sur le stockage géologique des déchets radioactifs à haute activité et à vie longue: résultats et perspectives’, ISBN 2-9510108-7-7, published June 2005.
- Huet B, L’Hostis V, Miserque F, et al. Electrochemical behaviour of mild steel in concrete: influence of pH and carbonate content of concrete pore solution. Electrochim Acta. 2005;51:172–180. doi: 10.1016/j.electacta.2005.04.014
- Macdonald DD, Roberts B. A potentiostatic transient study of the passivation of carbon steel in 1 M NaOH. Electrochim Acta. 1978;23:557–564. doi: 10.1016/0013-4686(78)85036-1
- Huang ZC, Ord JL. An optical study of the iron electrode in alkaline electrolyte. J Electrochem Soc. 1985;132:24–28. doi: 10.1149/1.2113774
- Miserque F, Huet B, Bendjaballah D, et al. X-ray photoelectron spectroscopy and electrochemical studies of mild steel FeE500 passivation in concrete simulated water. Proceedings of the Eurocorr 2005 Conference; 4–8 September 2005; Lisboa, Portugal.
- Carnot A, Frateur I, Zanna S, et al. Corrosion mechanisms of steel concrete moulds in contact with a demoulding agent studied by EIS and XPS. Corros Sci. 2003;45:2513–2524. doi: 10.1016/S0010-938X(03)00076-3
- Ghods P, Isgor OB, Brown JR, et al. XPS depth profiling study on the passive oxide film of carbon steel in saturated calcium hydroxide solution and the effect of chloride on the film properties. Appl Surf Sci. 2011;257:4669–4677. doi: 10.1016/j.apsusc.2010.12.120
- Odziemkowski MS, Schuhmacher TT, Gillham RW, et al. Mechanism of oxide film formation on iron in simulating groundwater solutions: Raman spectroscopic studies. Corros Sci. 1998;40:371–389. doi: 10.1016/S0010-938X(97)00141-8
- Joiret S, Keddam M, Novoa XR, et al. Use of EIS, ring-disk electrode, EQCM and Raman spectroscopy to study the film of oxides formed on iron in 1 M NaOH. Cem Conc Res. 2002;24:7–15. doi: 10.1016/S0958-9465(01)00022-1
- Nieuwoudt MK, Comins JD, Cukrowski I. The growth of the passive film on iron in 0.05 M NaOH studied in situ by Raman microspectroscopy and electrochemical polarization. Part II: In situ Raman spectra of the passive film surface during growth by electrochemical polarization. J Raman Spectrosc. 2011;42:1353–1365. doi: 10.1002/jrs.2842
- Alonso MC, García Calvo JL, Sánchez M, et al. Ternary mixes with high mineral additions contents and corrosion related properties. Mater Corros. 2012;63(12):1078–1086. doi: 10.1002/maco.201206654
- Koleva DA, Boshkov N, van Breugel K, et al. Steel corrosion resistance in model solutions, containing waste materials. Electrochim Acta. 2011;58:628–646. doi: 10.1016/j.electacta.2011.10.010
- Valentini C, Berardo L, Alanis I. Influence of blast furnace slags on the corrosion rate of steel in concrete. In: NS Berke, V Chaker, D Whiting, editors. Corrosion rates of steel in concrete, ASTM STP 1065. Philadelphia (PA): American Society for Testing and Materials; 1990. p. 17–28.
- Macphee DE, Cao HT. Theoretical description of impact of blast furnace slag (BFS) on steel passivation in concrete. Mag Concr Res. 1993;45(162):63–69. doi: 10.1680/macr.1993.45.162.63
- Tromans D. Anodic Polarization behaviour of mild steel in hot alkaline sulfide solutions. J Electrochem Soc. 1980;127(6):1253–1256. doi: 10.1149/1.2129865
- Muralidharan S, Saraswathy V, Thangavel K, et al. Competitive role of inhibitive and aggressive ions in the corrosion of steel in concrete. J Appl Electrochem. 2000;30:1255–1259. doi: 10.1023/A:1026570120698
- Saraswathy V, Muralidharan S, Thangavel K, et al. Influence of activated fly ash on corrosion-resistance and strength of concrete. Cem Concr Compos. 2003;25:673–680. doi: 10.1016/S0958-9465(02)00068-9
- Bastidas DM, Fernández-Jiménez A, Palomo A, et al. A study on the passive state stability of steel embedded in activated fly ash mortars. Corros Sci. 2008;50:1058–1065. doi: 10.1016/j.corsci.2007.11.016
- L’Hostis V, Amblard E, Blanc C, et al. Passive corrosion of steel in concrete in context of nuclear waste disposal. Corros Eng Sci Technol. 2011;46:177–181. doi: 10.1179/1743278210Y.0000000013
- Chomat L, L’Hostis V, Amblard E, et al. Long term study of passive corrosion of steel rebars in Portland mortar in context of nuclear waste disposal. Corros Eng Sci Technol. 2014;49:467–472. doi: 10.1179/1743278214Y.0000000201
- Gaucher EC, Tournassat C, Pearson FJ, et al. A robust model for pore-water chemistry of clayrock. Geochim Cosmochim Acta. 2009;73:6470–6487. doi: 10.1016/j.gca.2009.07.021
- Martin FA, Perrin S, Bataillon C. Evaluating the corrosion rate of low alloyed steel in Callovo-Oxfordian clay: towards a complementary EIS, gravimetric and structural study. Scientific Basis for Nuclear Waste Management XXXV, MRS Symposium Proceedings, Vol. 1475; 2012, p. 471–476.
- Martin F, Perrin S, Fenart M, et al. On corrosion of carbon steels in Callovo-Oxfordian clay: complementary EIS, gravimetric and structural study providing insights on long term behaviour in French geological disposal conditions. Corros Eng Sci Technol. 2014;49:460–466. doi: 10.1179/1743278214Y.0000000181
- Corrosion of metals and alloys – removal of corrosion products from corrosion test specimens, NF ISO 8407, ISO, Geneva, Switzerland; 2002.
- Neff D, Reguer S, Bellot-Gurlet L, et al. Structural characterization of corrosion products on archaeological iron: an integrated analytical approach to establish corrosion forms. J Raman Spectrosc. 2004;35:739–745. doi: 10.1002/jrs.1130
- Bellot-Gurlet L, Neff D, Reguer S, et al. Raman studies of corrosion layers formed on archaeological irons in various media. J Nano Res. 2009;8:147–156. doi: 10.4028/www.scientific.net/JNanoR.8.147
- L’Hostis V, Neff D, Bellot-Gurlet L, et al. Characterization of long-term corrosion of rebars embedded in concretes sampled on French historical buildings aged from 50 to 80 years. Mater Corros. 2009;60:93–98. doi: 10.1002/maco.200805019
- Monnier J, Neff D, Réguer S, et al. A corrosion study of the ferrous medieval reinforcement of the Amiens cathedral. Phase characterisation and localisation by various microprobes techniques. Corros Sci. 2010;52:695–710. doi: 10.1016/j.corsci.2009.10.028
- Richet C, Gallé C, Le Bescop P, et al. Synthèse des connaissances sur le comportement à long terme des bétons applications aux colis cimentés, French Report CEA-R-6050.
- Sercombe J, Vidal R, Gallé C, et al. Experimental study of gas diffusion in cement paste. Cem Conc Res. 2007;37:579–588. doi: 10.1016/j.cemconres.2006.12.003
- Frizon F, Gallé C. Experimental investigations of diffusive and convective transport of inert gas through cement pastes. J Porous Media. 2009;12(3):221–237. doi: 10.1615/JPorMedia.v12.i3.30
- Saheb M, Neff D, Bellot Gurlet L, et al. Raman study of a deuterated iron hydroxycarbonate to assess long-term corrosion mechanisms in anoxic soils. J Raman Spectrosc. 2011;42:1100–1108. doi: 10.1002/jrs.2828
- Saheb M, Berger P, Raimbault L, et al. Investigation of iron long-term corrosion mechanisms in anoxic media using deuterium tracing. J Nucl Mater. 2012;423:61–66. doi: 10.1016/j.jnucmat.2012.01.018