ABSTRACT
We studied abundance, diversity, and composition of soil invertebrates along snowmelt gradients to generally understand how soil animal communities are responding to life conditions across snowbeds along a west–east transect of the European Alps and to create a reference inventory for future investigations of climate change effects on snowbed habitats. We extracted microarthropods (collembolans, oribatid mites) and macroinvertebrates (spiders, beetles, insect larvae) from soil cores taken from three sections along the snowmelt gradient: high (early snowmelt), middle, and low (late snowmelt) sections. Linear models showed no correlations between either soil conditions or time of snowmelt and densities of soil animals. A small, though statistically significant, variation in the generally high soil organic matter and sand contents and high porosity of snowbed soils seems to have no effect on soil invertebrates. Species found along the snowmelt gradient were in similar shares generalist and specialist species. Microarthropod community composition in general was driven by soil porosity and soil organic matter content; for macroinvertebrate community composition we found no specific driver. We conclude that invertebrate species assemblages in snowbeds are rather similar in the European Alps.
Introduction
Snowbeds are depressions where snow accumulates and does not melt until late in the growing season, resulting in characteristic plant communities dominated by bryophytes, small perennial hemicryptophytes, and prostrate dwarf shrubs at the base of the snowbed, flowing into graminoid-dominated alpine grassland vegetation at the upper edge. Steep snowmelt gradients across snowbeds are commonly found above the alpine treeline and represent “experiments by nature” that permit testing hypotheses related to the effect of snowpack duration (season length) over very short spatial distances. Snowbeds are characterized by short growing seasons (Friedel Citation1961; Körner Citation2003; Matteodo et al. Citation2016), gradients in nutrient contents (Little et al. Citation2016), and stable soil temperatures during the snow cover season (Shimono and Kudo Citation2003; Körner et al. Citation2019), resulting in rather stable microbial activities (Schimel, Bilbrough, and Welker Citation2004) and rates of litter decomposition (Carbognani, Petraglia, and Tomaselli Citation2014) throughout the cold months. Communities inhabiting snowbeds are an important component of alpine biodiversity because they consist of species specifically adapted or restricted to these habitats (Björk and Molau Citation2007), even comprising endemic species (Dullinger, Dirnböck, and Grabherr Citation2000), but mostly species with a broad ecological amplitude. Until now, most snowbed studies focused on plant species richness (Domènech et al. Citation2016; Gritsch, Dirnböck, and Dullinger Citation2016), plant phenology after release from snow (e.g., Galen and Stanton Citation1995; Huelber et al. Citation2006; Sedlacek et al. Citation2015; Carbognani et al. Citation2016), the biology of snowbed specialist plant species (Lluent et al. Citation2013; Petraglia et al. Citation2014; Körner et al. Citation2019), or the impact of snow(melt) on plant community composition (e.g., Fowler and Overby Citation2016; Tonin et al. Citation2019).
However, even though the importance of the linkage between above- and belowground communities has been emphasized many times (e.g., de Deyn and van der Putten Citation2005; Kardol and Wardle Citation2010), the role of alpine soil invertebrates inhabiting snowbeds still awaits exploration, despite their being central for ecosystem functioning (Eisenhauer Citation2012; Römbke, Bernard, and Martin-Laurent Citation2018; Eisenhauer, Bonn, and Guerra Citation2019). Except for two very early studies on snowbed invertebrates in Norway (Hagvar, Melaen, and Ostbye Citation1974) and New Zealand (Dickinson et al. Citation1998) and two more recent studies from the Snowy Mountains of Australia (Green and Slatyer Citation2019) and from alpine habitats in New Zealand (Minor, Babenko, and Ermilov Citation2017), we found no other study on soil invertebrates permanently populating snowbeds. More local studies investigated community succession along glacier melt gradients rather than in snowbeds (Janetschek Citation1993; Kaufmann Citation2001) or community composition in alpine habitats in general (Haybach Citation1992; Steinwandter et al. Citation2018; Winkler et al. Citation2018).
As the climate will continue to warm in the European Alps at the current higher than average rate (Beniston et al. Citation2003; Beniston Citation2006; Hock et al. Citation2019), snowmelt regimes will change and so will the life conditions across snowmelt gradients (Vorkauf et al. Citation2021). Due to their very nature (i.e., their dependence on snow), snowbed communities are potentially more affected by climatic warming than other alpine biota. Changes in snowpack duration affect plant growth via time availability for resource assimilation (Galen and Stanton Citation1995), which may in turn affect resource availability for invertebrate species. Also, shorter periods of snow cover may change the microclimate (Matteodo et al. Citation2016) and impact life cycles (Søvik et al. Citation2003). Some of these changes may already be under way (Carbognani, Petraglia, and Tomaselli Citation2012; Gritsch, Dirnböck, and Dullinger Citation2016; Matteodo et al. Citation2016), causing plant productivity and the amount and quality of litter input and seasonal decomposition to change (Carbognani, Petraglia, and Tomaselli Citation2014), which may incur changes of functional species richness or even the local loss of specialist species (Komac et al. Citation2015). Here, we present one of very few studies on soil invertebrate abundances and diversity, their community composition, and their distribution along snowmelt gradients in the European Alps. We expect the snowmelt gradients across the snowbeds to represent a short distance gradient otherwise laid out for several hundred meters, with early snowmelt on the upper edge and late snowmelt at the bottom of the snowbed. The purpose of the study is, first, to generally understand which soil invertebrates are adapted to life conditions across snowbeds and, second, to create a reference inventory for future investigations of climate change effects on snowbed habitats. We hypothesize that invertebrate species composition will change along the snowmelt gradient due to the concomitant change in vegetation, as snowbeds host plant communities with a close to maximum productivity at the edge and a regional minimum productivity at the bottom of the snowbeds. For plants it has already been shown that snowbed communities comprise many specialist species, which will very likely also hold true for soil invertebrates. We therefore hypothesize that the soil community will comprise a high proportion (up to 50 percent) of specialist species that are adapted to snowbed conditions (e.g., hygrophilous, muscicolous, or lichenicolous species). Monitoring especially these specialists will help us in future to predict effects of climatic warming, because they will very likely be the first species to be affected by changing snowmelt regimes.
Material and methods
Study areas
The study was conducted as part of the Long-term Monitoring of Ecosystem Processes Programme of the Austrian National Park Hohe Tauern (Körner et al. Citation2019) and comprised three sites within the National Park (Innergschlöss [IN], Untersulzbachtal [UN], Seebachtal [SE]), as well as two LTER/LTSER (Long-term (Socio-)Ecological Research) sites (LTER-Switzerland, Alpine Research Station Furka, ALPFOR, Switzerland [FU]; LTSER IT25, Val Mazia/Matschertal, Italy [OB]; Figure S1a). In total, twenty-two transects (each 3 m wide, 7–10 m long) representing snowmelt gradients were established at elevations between 2,300 and 2,700 m.a.s.l.; that is, 200 to 450 m above the local climatic treeline (for details on the individual sites see ). Each transect was divided into three subtransects named A, B, and C, with the central B transect spared for nondestructive observations (Figure S1b). Vertically, each subtransect was separated in 1-m quadrats. The highest two to three quadrats (H, shortest snow duration) represent local close to maximum plant productivity, and the plant community is a typical alpine grassland dominated by graminoids such as Carex curvula. The middle part (M) is the transition zone, and the lowest two to three quadrats (L, longest snow duration) represent lowest plant productivity, with plant communities comprising bryophytes, small perennial herbs (e.g., Soldanella pusilla), and prostrate dwarf shrubs (e.g., Salix herbacea).
Table 1. Description of the five alpine study sites
Soil invertebrates—Microarthropods
We used vertically stratified samples to evaluate the abundance and diversity of soil microarthropods (collembolans, oribatid mites). First, a 10 × 10 cm frame was fixed to the ground in either the A or C subtransect to vacuum the 100 cm2 area for all epigeic animals using a small customized, battery-driven hand vacuum cleaner (Figure S2). Next, the vegetation was cut at soil surface (the material was used for a different research module), followed by coring the center of the harvested area using an O’Connor splitcore soil sampler containing PVC tubes (Figure S1; inner diameter 4.8 cm, each tube 5 cm high). Depending on the profile depth (e.g., stones), the soil core was divided into two or three layers (0–5 cm, 5–10 cm, and 10–15 cm, if available), including the litter and raw humus layer in the top core. Each core was closed with lids at the top and bottom, and samples were transported to the soil laboratory at the University of Innsbruck in a cool box within less than three days. For each of the five sites, thirty sample locations were selected (ten for each transect section H, M, L), evenly distributed among the available number of transects. This resulted in a total of 507 mesofauna samples (i.e., 5 sites × 30 sample locations × available number of strata).
Soil microarthropods were extracted by heat with a Macfadyen High Gradient apparatus for eight to ten days (Macfadyen Citation1953); the collection fluid was saturated saltwater. After extraction, all animals were transferred into a sieve (mesh size 500 µm) and rinsed in tap water, fixed in 75 percent ethanol, and identified to species level (adult specimens) using a stereomicroscope following the identification keys of Weigmann (Citation2006) for oribatid mites and Bretfeld (Citation1999), Fjellberg (Citation1998, Citation2007), Potapov (Citation2001), and Thibaud, Schulz, and Da Gama Assalino (Citation2004) for collembolans. For more details on methods, see Meyer (Citation2019).
Abundances of species per sample plot were extrapolated to individuals per square meter to account for different sample areas of litter (100 cm2) and soil samples (18.1 cm2). Because there were only very few soil invertebrates at soil depths below 5 cm, these few individuals were added to the 0–5 cm sample for further statistical analyses.
Soil invertebrates—Macrofauna
At three out of the five study sites (Furka, Oberettes, Innergschlöss) additional soil samples were taken as larger soil blocks to obtain soil macroinvertebrates (invertebrates > 2 mm; e.g., spiders, beetles, insect larvae). Due to the destructiveness of sampling such soil blocks, these were taken from outside the transects at the three sites (but close to and vertically aligned to the H, M, L sections).
At each of the three sites, eighteen samples (20 × 20 cm, 10–15 cm deep depending on soil depth) were taken from three selected snowbeds (six samples in each transect section H, M, and L), put into linen bags, and transported to the soil lab. From these fifty-four macrofauna samples, soil animals were heat-extracted for twelve days using a modified Kempson apparatus (Kempson, Lloyd, and Ghelardi Citation1963), with propylene glycol as collection fluid. After extraction, soil animals were transferred to 75 percent ethanol and identified using a dissection stereomicroscope. Taxonomic identification followed Schaefer (Citation2009). The physical and chemical soil characteristics of these soil blocks were assumed to match those obtained nearby by soil cores. As with mesofauna species, abundances were extrapolated to individuals per square meter.
Soil parameters
At each site two types of soil samples were taken for physical and chemical analysis and root sampling: undisturbed cylinder samples and disturbed dug-out samples of similar volume. The cylinder samples are required for the analysis of the soil water balance (pF curve) and for the determination of the root biomass. The disturbed samples were needed for the analysis of soil texture and soil chemical parameters. The samples were taken from the top 6 cm (excluding the litter and top raw humus layer). The cylinders had a volume of 100 cm3 (diameter of 5 cm and a height of 5 m), and the disturbed sample had at least 150 g dry weight. Cylinder samples were completely saturated in a water bath before further analyses; the disturbed soil samples were air-dried and then sieved to 2 mm. Altogether, 116 soil samples were taken (FU: 29, OB: 15, UN: 30, IN: 25, SE: 17).
To obtain soil organic matter content (SOM), 5 g of air-dried soil was incinerated at 550°C for 3 hours in a muffle furnace (all sites had silicate bed rock; hence, there was no need for a carbonate treatment). The pF curve was measured using an automated analyzing system (HYPROP, Meter Group, Munich, Germany) following the manufacturer’s guidelines. Soil texture was assessed by means of an automated measuring device (PARIO, Meter Group) following the manufacturer’s guidelines with slight modifications in the sample preparation, because the content of hardly decomposable organic matter in the samples was very high. Therefore, the time for the destruction of the organic matter was extended and the addition of H2O2 increased. Soil chemical analyses were carried out at the Research Center Laimburg (Bozen/Bolzano, Italy) following standard protocols (Table S1). For more details on methods, see Newesely, Tappeiner, and Körner (Citation2019).
Root mass
For the determination of the belowground plant biomass (root biomass), sample cylinders were taken in addition to the soil samples at the same plots and soil depths. The roots were washed out in the laboratory under running water in three rinsing cycles (with sieves of 2 mm, 1 mm, and 0.5 mm mesh width, respectively) and then dried at 80°C and weighted. For more details on methods, see Newesely, Tappeiner, and Körner (Citation2019).
Data on snow cover
In each section (H, M, L) of each transect a soil temperature logger was installed at 3- to 4-cm depth in subtransect B (iButton DS 1922L, with measuring interval of 3 hours). The time point of snowmelt can be clearly identified by the sudden temperature jump from near 0°C to positive temperatures. The opposite pattern applies to the time point of snowing in early autumn. Loggers have been continuously measuring soil temperature since the beginning of the project, and we used data from four years (2017–2020) to evaluate mean snowmelt patterns for each site ().
Table 2. Mean day of year of snowmelt and mean duration of snow cover (number of days), comprising data from years 2017–2020. FU = Furka, OB = Oberettes, UN = Untersulzbachtal, IN = Innergschlöss, S = Seebachtal
Statistical analyses
Differences in soil parameters, in total abundances (individuals per square meter per sampling plot) of microarthropods and macroinvertebrates, and in species richness (number of species per sampling plot) between sites were analyzed by linear models (LMs) with site as factor, followed by Tukey’s post hoc tests at a significance level of p <.05 using the R package multcomp (Hothorn et al. Citation2019). Differences in abundances between generalist and specialist species for each site were also analyzed by LMs with factors ecological type (generalist/specialist) and site. To analyze differences in soil parameters and snow cover (day of year of snowmelt, duration of snow cover) between transect sections (H, M, L), we used linear mixed effect models (LMEs) with site as a random effect to account for site-specific differences using R package lme4 (Bates et al. Citation2015). The same procedure was used to test for the effect of day of year of snowmelt and duration of snow cover on abundances of invertebrates. Generalized linear models with a Gamma distribution (due to positively skewed abundance data) were used to test for correlations between soil parameters and abundances of invertebrates. Before doing so, we calculated intracorrelation coefficients to test whether adding site as random effect is necessary here as well; however, intracorrelation coefficients revealed no dependencies. For each model, specifications and residual diagnostics were performed with R package dharma (Hartig Citation2020) and model quality and goodness-of-fit were evaluated with R package performance (Lüdecke, Makowski, and Waggoner Citation2020).
For each site we calculated both the Shannon-Weaver diversity and Simpson diversity using R package vegan (Oksanen et al. Citation2019), and to test for differences in species diversity among sites, we employed LMs.
Community compositions of microarthropods and macroinvertebrates were analyzed via nonmetric multidimensional scaling (NMDS) using Bray-Curtis distance with function metaMDS in R package vegan. To test for significant differences in community composition between sites and transect sections, we employed permutational multivariate analyses of variance (PERMANOVAs) with function adonis in R package vegan. To evaluate the effect of soil parameters on community composition, we calculated constrained analyses of principal coordinates using Bray-Curtis distance with function capscale in R package vegan. To obtain the best-fitting model, a combination of an a priori selection of predictors based on expertise and a forward selection approach was employed. We first selected soil parameters with low correlation coefficients to other parameters to avoid multicollinearity but chose at least one parameter out of the following groups: soil texture (amount of sand, silt, and clay), soil nutrients (soil organic matter content, total nitrogen, cation exchange capacity, saturation), and soil physical parameters (porosity, bulk density, pF values). Starting from the full model, we then continuously dropped nonsignificant terms until only significant ones were left. All analyses were computed using R (v4.0.2; R Core Team Citation2020) in R Studio (v1.3.959; R Studio Team Citation2020).
Results
Soil properties
Except for root mass and clay content, soil parameters differed significantly among sites (), even though within-site variation was mostly rather high. Noteworthy is the high variation in SOM and total nitrogen (Ntot) in the Seebachtal transects. Significant differences along the snowmelt gradient were found only for porosity and bulk density. Soil porosity increased significantly from L to H (LME, Tukey contrasts: p = .005), whereas bulk density significantly decreased (LME, Tukey contrasts: p = .006).
Table 3. Descriptive means (SDs) of soil parameters for each site and section
Snow cover
We evaluated data on snow cover (day of year of end of snowmelt, duration of snow cover) for the year 2017 (sampling year of soil fauna) as well as for all four years for which data were available (2017–2020). Day of year of end of snowmelt and duration of snow cover differed significantly between sections L and H when considering data for 2017 only (LME, Tukey contrasts: p = .002 for day of year of end of snowmelt; LME, Tukey contrasts: p = .012 for duration of snow cover) as well as when including data from all four years (LME, Tukey contrasts: p = .001 for day of snowmelt; LME, Tukey contrasts: p = .002 for duration of snow cover). Estimated mean difference in end of snowmelt between sections L and H was eighteen days.
Snowmelt finished earliest in 2017 in the Seebachtal transects () and latest at the Furka in section H (the highest point of the snowmelt gradient). The lowest point of the snowmelt gradient (section L) was covered with snow the longest in the Innergschlöss, which was derived from the latest mean day of year of end of snowmelt as well as from the longest mean duration of snow cover.
Soil invertebrate abundance
In total, we obtained 8,678 adult collembolans belonging to twenty-five species in ten families, 5,216 adult oribatid mites belonging to forty-nine species in twenty-four families, as well as 1,639 macroinvertebrates mostly belonging to spiders, beetles, and insect larvae (Tables S2, S3, S5).
The significantly highest mean abundance of collembolans was found at the Furka, followed by the Seebachtal, and the lowest mean abundance was obtained from Oberettes (LM: F4,142 = 6.51, p <.001; ). No significant difference in Collembola abundance was found among transect sections L, M, and H along the snowmelt gradient, but the highest abundances were still recorded from the bottom of the gradient (section L). No correlation between the total abundance of collembolans and any soil parameters was detected. In addition, no effect of day of snowmelt or of duration of snow cover could be statistically detected.
Figure 1. Mean abundances (individuals per square meter, in log scale) of (a) collembolans and (b) oribatid mites within each research site. Different letters above box plots indicate significant differences in abundances between sites. FU = Furka, OB = Oberettes, UN = Untersulzbachtal, IN = Innergschlöss, S = Seebachtal.
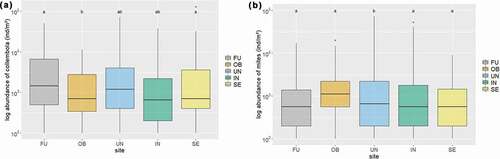
Oribatid mites were most abundant in the Untersulzbachtal, followed by Oberettes, and the lowest abundance of mites was recorded from the Seebachtal (LM: F4,141 = 4.55, p = .002; ). Again, no significant difference was found in mean oribatid mite abundance among transect sections, but in contrast to the collembolans, the highest abundance was found at the top of the snowmelt gradient (transect section H). Again, no effect of soil parameters, day of snowmelt, or duration of snow cover could be statistically detected.
Surprisingly, no significant differences were found for macroinvertebrate abundances, either among sites or among the H, M, L sections of the snow duration gradients along the transects. Again, we found no correlations between abundances of macroinvertebrates and any of the soil or snow parameters.
Species/taxon richness and community composition
Altogether, we found seventy-four soil microarthropod species; the most species-rich site was the Seebachtal (seventeen collembolan and twenty-five oribatid mite species), followed by the Furka (thirteen collembolan and twenty-two oribatid mite species), and the least species were found at Oberettes (ten collembolan and eighteen oribatid mite species; LM: F4,148 = 2.73, p = .031). Eleven species (five collembolan and six oribatid mite species; that is, 15 percent of all microarthropod species) were found at all five sites, and the most common species (found at all sites with high abundances) was Pseudisotoma sensibilis Tullberg, 1876 (Collembola). On the other hand, thirty-six species (nine collembolan and twenty-seven oribatid mite species; that is, 49 percent of all species) were unique to one site, and most were recorded from the Seebachtal (five collembolan and eleven oribatid mite species). These unique species were mostly found in very low abundances, whereas the more common species (occurring in at least three sites) showed high abundances (Tables S2, S3). Shannon-Weaver diversity () and Simpson diversity differed significantly among sites (LM: F4,148 = 4.21, p = .003; LM: F4,148 = 7.73, p <.001, respectively), and the highest diversity was recorded for the Seebachtal transects (Shannon-Weaver 1.57, Simpson 0.72) and Oberettes (1.52, 0.78). Most species found along the snowmelt gradient were in similar shares generalist or specialist species (i.e., species adapted to conditions specifically associated with alpine snowbeds such as alpine, hygrophilous, lichenicolous, or muscicolous species; see Table S4). For oribatid mites the proportions of generalists and specialists were balanced; we found no significant differences in abundances of both ecological groups within each site. For collembolans we found a significantly higher number of specialists in snowbeds of the Seebachtal (); in all other sites the proportions were again statistically balanced.
Figure 2. Shannon-Weaver index for microarthropod diversity within each research site. Different letters above boxplots indicate significant differences in microarthropod diversity between sites. FU = Furka, OB = Oberettes, UN = Untersulzbachtal, IN = Innergschlöss, S = Seebachtal.
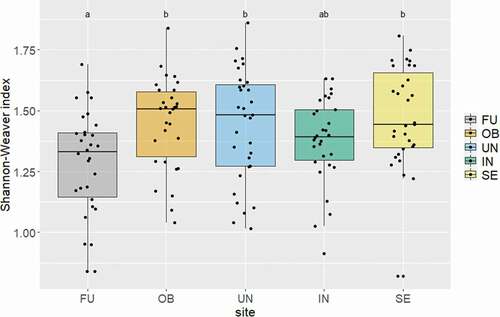
Figure 3. Mean abundances of generalist and specialist collembolans within each site. Asterisks indicate significant differences in abundances of generalists and specialists within a site. FU = Furka, OB = Oberettes, UN = Untersulzbachtal, IN = Innergschlöss, S = Seebachtal.
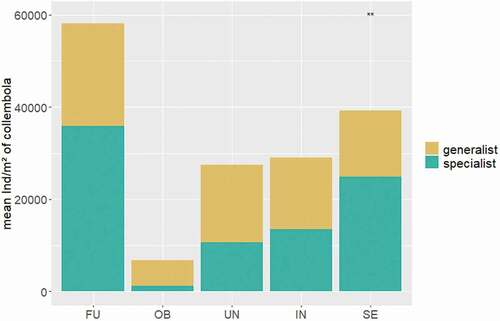
Furka also harbored the most macroinvertebrates (820 individuals), followed by the Innergschlöss (445) and Oberettes (374). Most individuals were larvae of true flies (Brachycera, 164 individuals) or beetles (adults and larvae, 109 individuals; Table S3).
NMDS analysis of microarthropods showed that community composition was quite similar at all five sites, with big overlaps between sites (), except for Oberettes, which did not overlap with Innergschlöss and Untersulzbachtal. Nevertheless, PERMANOVA revealed significant differences in community composition among sites (PERMANOVA: p = .001). The same is true for collembolans (not shown), oribatid mites (not shown), and macroinvertebrates (): we found large overlaps between sites but still significant differences in community composition (p = .001 for all three PERMANOVAs). We found no differences in species assemblages between transect sections H, M, and L.
Drivers of snowbed community composition
Constrained MDS revealed that microarthropod community composition in general was mainly driven by soil porosity (p = .001) and soil organic matter content (p = .001). The same parameters were responsible for community composition of collembolans (p = .001 and p = .002, respectively), whereas oribatid mite communities were driven by soil porosity (p = .001) and silt (p = .011). No parameters were found that significantly affected community composition of macroinvertebrates.
Discussion
Our analysis of diversity and abundance of soil invertebrates together with soil properties across steep snowmelt gradients at five alpine sites, widely spaced over the European Alps, revealed a surprisingly small variation in soil characteristics, associated with a similarly surprising small variation in invertebrate species distribution. Contrary to our expectations, data on snowmelt patterns revealed only a small difference between high (early snowmelt) and low (late snowmelt) sections of less than three weeks.
All five study sites are located on silicate bedrock; therefore, pH values are low (all mean values are <4). The twenty-two snowbeds investigated in this study generally exhibit high organic matter contents; the Seebachtal transects with a mean SOM of 31 percent are particularly striking. Due to the high sand content and the high porosity, soils tend to warm up and dry out during the short snow-free period; thus, decomposer activity might be limited, thereby retarding litter decomposition (Carbognani, Petraglia, and Tomaselli Citation2012) and leading to an increase in organic matter content. Similar soil features (high organic matter content, high soil porosity, high sand content) have been reported for snowbeds in the Rhaetian Alps (Carbognani, Petraglia, and Tomaselli Citation2012) and the Upper Engadine (Hiller et al. Citation2005). Even though we found statistically significant differences in soil parameters among sites, contents of SOM and sand as well as soil porosity are already at such a high level that it was not surprising that these soil characteristics did not significantly correlate with abundances of soil invertebrates.
Soil invertebrate communities were dominated by microarthropods (approximately 26,000 ind/m2 on average per sample plot); far fewer macroinvertebrates were found (approximately 760 ind/m2 on average per sample plot). Temperature is a major regulator of metabolic activity of soil invertebrates, and collembolans and oribatid mites are better adapted to low temperatures during the snow cover season and short vegetation periods than macroinvertebrates (Lavelle and Spain Citation2001; Teets and Denlinger Citation2014). Especially for earthworms, the low temperatures restrict their ability to inhabit snowbed soils (Meshcheryakova and Berman Citation2014), and the poorly developed soils (all Leptosols) provide limited habitat for burrowing earthworms. We found mostly poorly mobile larvae of true flies as well as surface-active beetles (ground and rove beetles), which predate on microarthropods (König, Kaufmann, and Scheu Citation2011; Raso et al. Citation2014).
We found around 5,000 to 24,000 collembolans and 6,000 to 18,000 oribatid mites per square meter, but these numbers are much lower than those reported from snowbeds in Norway, where similar sampling methods have been used (Hagvar, Melaen, and Ostbye Citation1974), and also much lower compared to those of lowland habitats (Coleman, Callaham, and Crossley Citation2017), very likely owing to the particular soil and climatic conditions of snowbeds (e.g., the location in depressions and the resulting late snowmelt). We found no differences in abundance of microarthropods or species composition between bottom, middle, and top of the snowmelt gradient, very likely because the animals, in contrast to plants, are mobile and can move between these zones (Green and Slatyer Citation2019). Both collembolans and oribatid mites have been shown to inhabit the intranivean space, where they use the pores between snow crystals (Hågvar Citation2010) and can therefore move even during the season with snow cover. Other studies have shown that arthropod communities depend on snowmelt patterns and time of snowmelt (Leingärtner, Krauss, and Steffan-Dewenter Citation2014; Green and Slatyer Citation2019); however, contrary to our expectations, we could not verify this in our study, most likely due to the small difference in end of snowmelt between sections L and H. In general, collembolans are short-lived invertebrates, completing several generations per year, whereas oribatid mites have a life cycle of approximately two years. However, for both taxa studies in arctic and alpine regions have shown that they adapt to cold conditions by extending their life cycle to two years (collembolans) and even up to five years (oribatid mites; Søvik et al. [Citation2003] and citations therein). In our opinion the small difference in the duration of snow cover between L and H along the snowmelt gradient does not bias abundances of these animals due to different longevity, because they already extended their life cycle over a longer period.
Species composition was to similar shares (i.e., 50 percent each) made up of euryoecious species and species adapted to snowbed conditions. The species with the highest density by far, the collembolan Pseudisotoma sensibilis, a widespread palaearctic species living in the upper soil layer (Christian Citation1987), was found at all five sites. The most abundant oribatid mite that was found at all five sites, Tectocepheus velatus (Michael, 1880), is a euryoecious cosmopolitan species (Schatz Citation1983). In contrast, species with special habitat requirements such as lichens, mosses, or high moisture contents (e.g., the collembolans Sminthurides schoetti Axelson, 1903 and Tetracanthella afurcata Handschin, 1919 or the oribatid mites Melanozetes meridianus Sellnick, 1928 and Oribatula interrupta [Willmann, 1939]) were found in highly variable densities. We even found two oribatid species endemic to the Alps, Kunstidamaeus diversipilis (at Furka and Untersulzbachtal with mean abundances of approximately 670 ind/m2) and Trichoribates scilierensis (at Furka, Oberettes, and Seebachtal with mean abundances of approximately 1,730 ind/m2). Species diversity was highest at the Seebachtal and Oberettes (); the former harbored a range of species that were unique to this site but were found in very low densities (mostly just one individual in one sample). Due to some widespread species occurring in high densities in (almost) all sites, NMDS results exhibited large overlaps between sites, but species space was especially large for the Seebachtal (Figure S3a). Snowbeds of the Seebachtal differed from those of the other regions in that they offered little soil to sample and showed the least pronounced snowmelt gradients, and one of the three Seebachtal transects did not fully meet the requirements of a snowbed (being flatter, wind-exposed, and covered with dwarf shrubs). In addition to species diversity, species richness was highest in these transects. Aside from characteristic snowbed species such as T. afurcata and euryoecious species such as P. sensibilis, they harbored collembolan and mite species that are usually not found in snowbeds; for example, silvicolous species (Caleremaeus monilipes (Michael, 1882), Conoppia palmicincta (Michael, 1884), Phthiracarus globosus (Koch, 1841), Oribatida, probably brought there by grazing cattle. However, because these species were found in such low abundances, they did not affect the outcome of any linear models tested in this study (we ran each model with and without the Seebachtal transects).
Species assemblages of microarthropods and, within these, of collembolans, were mainly driven by soil porosity and organic matter content (). Collembolans are detritivores feeding on litter and fungi (Coleman, Callaham, and Crossley Citation2017) and are thus highly dependent on SOM. Also, species inhabiting the upper soil layer are highly mobile due to their jumping ability and thus rely on a porous soil structure. Oribatid mite communities were driven by soil porosity and silt content. Because they exhibit a large variety of feeding habits (Coleman, Callaham, and Crossley Citation2017), they are less dependent on organic matter as an entire group, but their dependence on soil porosity has been shown before (Ducarme et al. Citation2004), because they use the soil pores as living space.
To conclude, species assemblages of microarthropods in snowbeds are rather similar along our west–east transect of the European Alps, because most individuals belong to widespread species. Contrary to our hypotheses, they seem to depend neither on specific soil characteristics nor on the duration of snow cover and the timing of snowmelt. Monitoring these communities and especially the specialists within the communities over a longer period will be necessary to better understand how snowbed invertebrates will respond to a warmer future climate. However, as our data have shown, snowbeds are not suitable as models for larger snowpack duration gradients, as they are for plants, very likely due to the mobility of the animals. Contrary to plants, invertebrates, even (or especially) small ones such as collembolans and oribatid mites, are able to move under snow or away from it if conditions become too harsh.
Supplemental Material
Download JPEG Image (1.1 MB)Supplemental Material
Download JPEG Image (375.3 KB)Supplemental Material
Download TIFF Image (98.5 KB)Supplementary material
Supplemental material for this article can be accessed on the publisher’s website
Disclosure statement
No potential conflict of interest was reported by the authors(s).
Additional information
Funding
References
- Bates, D., M. Mächler, B. Bolker, and S. Walker. 2015. Fitting linear mixed-effects models using Lme4. Journal of Statistical Software 67 (1):48. https://www.jstatsoft.org/index.php/jss/article/view/v067i01.
- Beniston, M., F. Keller, B. Koffi, and S. Goyette. 2003. Estimates of snow accumulation and volume in the Swiss Alps under changing climatic conditions. Theoretical and Applied Climatology 76 (3–4):125–40. doi:https://doi.org/10.1007/s00704-003-0016-5.
- Beniston, M. 2006. Mountain weather and climate: A general overview and a focus on climatic change in the Alps. Hydrobiologia 562 (1):3–16. doi:https://doi.org/10.1007/s10750-005-1802-0.
- Björk, R. G., and U. Molau. 2007. Ecology of Alpine snowbeds and the impact of global change. Arctic, Antarctic, and Alpine Research 39 (1):34–43. doi:https://doi.org/10.1657/1523-0430(2007)39[34:EOASAT]2.0.CO;2.
- Bretfeld, G. 1999. Synopses on Palaearctic Collembola Pt 2: Symphypleona. Goerlitz: Senckenberg Museum of Natural History.
- Carbognani, M., A. Petraglia, and M. Tomaselli. 2012. Influence of snowmelt time on species richness, density and production in a late snowbed community. Acta Oecologica 43:113–20. doi:https://doi.org/10.1016/j.actao.2012.06.003.
- Carbognani, M., A. Petraglia, and M. Tomaselli. 2014. Warming effects and plant trait control on the early-decomposition in alpine snowbeds. Plant and Soil 376 (1–2):277–90. doi:https://doi.org/10.1007/s11104-013-1982-8.
- Carbognani, M., G. Bernareggi, F. Perucco, M. Tomaselli, and A. Petraglia. 2016. Micro-climatic controls and warming effects on flowering time in alpine snowbeds. Oecologia 182: 573–585
- Christian, E. 1987. Catalogus Faunae Austriae, Part XII a: U.-Kl.: Collembola (Springschwänze). Vienna, Austria: Verlag der Österreichischen Akademie der Wissenschaften.
- Coleman, D. C., M. A. Callaham, and D. Crossley. 2017. Fundamentals of soil ecology. 3rd ed. San Diego: Academic Press.
- de Deyn, G. B., and W. H. van der Putten. 2005. Linking Aboveground-belowground diversity. Trends in Ecology & Evolution 20 (11): 625–33. doi:https://doi.org/10.1016/j.tree.2005.08.009.
- Dickinson, K. J. M., A. F. Mark, B. I. P. Barratt, and B. H. Patrick. 1998. Rapid ecological survey, inventory and implementation: A case study from Waikaia Ecological Region, New Zealand. Journal of the Royal Society of New Zealand 28 (1):83–156. doi:https://doi.org/10.1080/03014223.1998.9517556.
- Domènech, M., B. Komac, J. Peñuelas, and J. A. Conesa. 2016. Site-specific factors influence the richness and phenology of snowbed plants in the Pyrenees. Plant Biosystems 150 (4):741–49. doi:https://doi.org/10.1080/11263504.2014.990941.
- Ducarme, X., H. M. André, G. Wauthy, and P. Lebrun. 2004. Are there real endogeic species in temperate forest mites? Pedobiologia 48 (2):139–47. doi:https://doi.org/10.1016/j.pedobi.2003.10.002.
- Dullinger, S., T. Dirnböck, and G. Grabherr. 2000. Reconsidering endemism in the North-Eastern Limestone Alps. Acta Botanica Croatica 59 (1):55–82.
- Eisenhauer, N., A. Bonn, and C. A. Guerra. 2019. Recognizing the quiet extinction of invertebrates. Nature Communications 10 (1):1–3. doi:https://doi.org/10.1038/s41467-018-07916-1.
- Eisenhauer, N. 2012. Aboveground-Belowground interactions as a source of complementarity effects in biodiversity experiments. Plant and Soil 351 (1–2):1–22. doi:https://doi.org/10.1007/s11104-011-1027-0.
- Fjellberg, A. University of T. 1998. The Collembola of Fennoscandia and Denmark, part I: Poduromorpha. Fauna Ento. Leiden, The Netherlands: Brill.
- Fjellberg, A. 2007. The Collembola of Fennoscandia and Denmark, Part II: Entomobryomorpha and Symphypleona. Fauna Ento. Leiden, The Netherlands: Brill.
- Fowler, J. F., and S. Overby. 2016. Snow duration effects on density of the alpine endemic plant Packera franciscana. Western North American Naturalist 76 (3):383–87. doi:https://doi.org/10.3398/064.076.0301.
- Friedel, H. 1961. Schneedeckendauer Und Vegetationsverteilungen Im Gelände. In: Ökologische Untersuchungen in Der Subalpinen Stufe - Teil 1. Mitteilungen Der Forstlichen Bundes-Versuchsanstalt Mariabrunn: 317–369.
- Galen, C., and M. L. Stanton. 1995. Responses of snowbed plant species to changes in growing-season length. Ecology 76 (5):1546–57. doi:https://doi.org/10.2307/1938156.
- Green, K., and R. Slatyer. 2019. Arthropod community composition along snowmelt gradients in snowbeds in the snowy mountains of South-Eastern Australia. Australia Ecology 45: 144–157 .
- Gritsch, A., T. Dirnböck, and S. Dullinger. 2016. Recent changes in alpine vegetation differ among plant communities. Journal of Vegetation Science 27 (6):1177–86. doi:https://doi.org/10.1111/jvs.12447.
- Hagvar, S., J. Melaen, and E. Ostbye. 1974. Quantitative studies of the invertebrate fauna in an alpine snow-bed community at Finse, South Norway. Norsk Entomologisk Tidsskrift 21:45–51.
- Hågvar, S. 2010. A review of Fennoscandian arthropods living on and in snow. European Journal of Entomology 107 (3):281–98. doi:https://doi.org/10.14411/eje.2010.037.
- Hartig, F. 2020. DHARMa: Residual diagnostics for hierarchical (multi-level/mixed) regression models. R Package Version 0.3.2.0.
- Haybach, G. 1992. Zur Collembolenfauna verschiedener Gebirgsstandorte in Österreich. Verhandlungen der Zoologisch-Botanischen Gesellschaft Österreichs 129:159–214.
- Hiller, B., A. Nuebel, G. Broll, and F. K. Holtmeier. 2005. Snowbeds on silicate rocks in the Upper Engadine (Central Alps, Switzerland) - Pedogenesis and interactions among soil, vegetation, and snow cover. Arctic, Antarctic, and Alpine Research 37 (4):465–76. doi:https://doi.org/10.1657/1523-0430(2005)037[0465:SOSRIT]2.0.CO;2.
- Hock, R., G. Rasul, C. Adler, B. Cáceres, S. Gruber, Y. Hirabayashi, M. Jackson, et al. 2019. High mountain areas. In The Ocean and Cryosphere in a Changing Climate., ed. H.-O. Pörtner, D. C. Roberts, V. Masson-Delmotte, P. Zhai, M. Tignor, E. Poloczanska, K. Mintenbeck, et al., 94. Intergovernmental Panel on Climate Change.
- Hothorn, B. F., P. Westfall, R. M. Heiberger, A. Schuetzenmeister, and S. Scheibe. 2019. Package “Multcomp”: Simultaneous inference in general parametric models. R Package Version 1.4–10. https://cran.r-project.org/package=multcomp.
- Huelber, K., M. Gottfried, H. Pauli, K. Reiter, M. Winkler, and G. Grabherr. 2006. Responses of snowbed species to snow removal dates in the Central Alps: Implications for climate warming. Arctic, Antarctic, and Alpine Research 38:99–103.
- Janetschek, H. 1993. Über Wirbellosen-Faunationen in Hochlagen der Zillertaler Alpen. Berichte des Naturwissenschaftlichen-Medizinischen Vereins Innsbsruck 80:121–65.
- Kardol, P., and D. A. Wardle. 2010. How understanding aboveground-belowground linkages can assist restoration ecology. Trends in Ecology & Evolution 25 (11):670–79. doi:https://doi.org/10.1016/j.tree.2010.09.001.
- Kaufmann, R. 2001. Invertebrate sucession on an Alpine glacier foreland. Ecology 82 (8):2261–78. doi:https://doi.org/10.1890/0012-9658(2001)082[2261:ISOAAG]2.0.CO;2.
- Kempson, D., M. Lloyd, and R. Ghelardi. 1963. A new extractor for woodland litter. Pedobiologia 3 (1):1–21 .
- Komac, B., C. Pladevall, J. Peñuelas, J. V. Conesa, and M. Domènech. 2015. Variations in functional diversity in snowbed plant communities determining snowbed continuity. Plant Ecology 216 (9):1257–74. doi:https://doi.org/10.1007/s11258-015-0506-4.
- König, T., R. Kaufmann, and S. Scheu. 2011. The formation of terrestrial food webs in glacier foreland: Evidence for the pivotal role of decomposer prey and intraguild predation. Pedobiologia 54 (2):147–52. doi:https://doi.org/10.1016/j.pedobi.2010.12.004.
- Körner, C., S. Riedl, T. Keplinger, A. Richter, J. Wiesenbauer, F. Schweingruber, and E. Hiltbrunner. 2019. Life at 0 °C: The biology of the alpine snowbed plant Soldanella pusilla. Alpine Botany 129 (2):63–80. doi:https://doi.org/10.1007/s00035-019-00220-8.
- Körner, C. 2003. Alpine plant life. Berlin, Heidelberg: Springer.
- Lavelle, P., and A. Spain. 2001. Soil ecology. Dordrecht, The Netherlands: Springer Science & Business Media.
- Leingärtner, A., J. Krauss, and I. Steffan-Dewenter. 2014. Elevation and experimental snowmelt manipulation affect emergence phenology and abundance of soil-hibernating arthropods. Ecological Entomology 39 (4):412–18. doi:https://doi.org/10.1111/een.12112.
- Little, C. J.,J. A. Wheeler, J. Sedlacek, A. J. Cortés, and C. Rixen. 2016. Small-scale drivers: The importance of nutrient availability and snowmelt timing on performance of the alpine shrub Salix herbacea. Oecologia 180 (4):1015–24. doi:https://doi.org/10.1007/s00442-015-3394-3.
- Lluent, A., A. Anadon-Rosell, J. M. Ninot, O. Grau, and E. Carrillo. 2013. Phenology and seed setting success of snowbed plant species in contrasting snowmelt regimes in the central Pyrenees. Flora: Morphology, Distribution, Functional Ecology of Plants 208 (3):220–31. doi:https://doi.org/10.1016/j.flora.2013.03.004.
- Lüdecke, D., D. Makowski, and P. Waggoner. 2020. Performance: Assessment of regression models performance. R Package Version 0.4.4.
- Macfadyen, A. 1953. Notes on methods for the extraction of small soil arthropods. Journal of Animal Ecology 22 (1):65–77. doi:https://doi.org/10.2307/1691.
- Matteodo, M., K. Ammann, E. P. Verrecchia, and P. Vittoz. 2016. Snowbeds are more affected than other subalpine–alpine plant communities by climate change in the Swiss Alps. Ecology and Evolution 6 (19):6969–82. doi:https://doi.org/10.1002/ece3.2354.
- Meshcheryakova, E. N., and D. I. Berman. 2014. Cold hardiness and geographic distribution of earthworms (Oligochaeta, Lumbricidae, Moniligastridae). Entomological Review 94 (4):486–97. doi:https://doi.org/10.1134/S0013873814040046.
- Minor, M. A., A. B. Babenko, and S. G. Ermilov. 2017. Oribatid mites (Acari: Oribatida) and Springtails (Collembola) in alpine habitats of Southern New Zealand. New Zealand Journal of Zoology 44 (1):65–85. doi:https://doi.org/10.1080/03014223.2016.1251950.
- Meyer, E. 2019. Langzeitmonitoring von Ökosystemprozessen Im Nationalpark Hohe Tauern. Modul 03: Bodenmesofauna. In Methoden-Handbuch. Verlag der Österreichischen Akademie der Wissenschaften, Vienna, Austria.
- Newesely, C., U. Tappeiner, and C. Körner 2019. Langzeitmonitoring von Ökosystemprozessen Im Nationalpark Hohe Tauern. Modul 01: Standortklima, Bodenphysik, Bodenchemie Und Pflanzliche Produktivität. In Methoden-Handbuch Verlag der Österreichischen Akademie der Wissenschaften, Vienna, Austria.
- Oksanen, J., F. G. Blanchet, M. Friendly, R. Kindt, P. Legendre, D. McGlinn, P. R. Minchin, R. B. O’Hara, G. L. Simpson, P. Solymos, et al. 2019. Vegan: Community ecology package. R Package Version 2.5–6.
- Petraglia, A., M. Tomaselli, A. Mondoni, L. Brancaleoni, and M. Carbognani. 2014. Effects of nitrogen and phosphorus supply on growth and flowering phenology of the snowbed forb Gnaphalium supinum L. Flora: Morphology, Distribution, Functional Ecology of Plants 209 (5–6):271–78. doi:https://doi.org/10.1016/j.flora.2014.03.005.
- Potapov, M. 2001. Synopses on palaearctic Collembola Pt 3: Lsotomidae. Goerlitz: Senckenberg Museum of Natural History.
- R Core Team. 2020. R: A language and environment for statistical computing. Vienna, Austria: R Foundation for Statistical Computing.
- R Studio Team. 2020. RStudio: Integrated development for R. Boston: RStudio, Inc.
- Raso, L., D. Sint, R. Mayer, S. Plangg, T. Recheis, S. Brunner, R. Kaufmann, and M. Traugott. 2014. Intraguild predation in pioneer predator communities of alpine glacier forelands. Molecular Ecology 23 (15):3744–54. doi:https://doi.org/10.1111/mec.12649.
- Römbke, J., J. Bernard, and F. Martin-Laurent. 2018. Standard methods for the assessment of structural and functional diversity of soil organisms: A review. Integrated Environmental Assessment and Management 14 (4):463–79. doi:https://doi.org/10.1002/ieam.4046.
- Schaefer, M. 2009. Brohmer - Fauna von Deutschland: Ein Bestimmungsbuch Unserer Heimischen Tierwelt. 23rd ed. Wiebelsheim: Quelle & Meyer Verlag.
- Schatz, H. 1983. Catalogus Faunae Austriae, Teil IXi: Unt.Ordn.: Oribatei, Hornmilben. Vienna, Austria: Verlag der Österreichischen Akademie der Wissenschaften.
- Schimel, J. P., C. Bilbrough, and J. M. Welker. 2004. Increased snow depth affects microbial activity and nitrogen mineralization in two Arctic tundra communities. Soil Biology & Biochemistry 36 (2):217–27. doi:https://doi.org/10.1016/j.soilbio.2003.09.008.
- Sedlacek, J., J.A. Wheeler, A.J. Cortés, O. Bossdorf, G. Hoch, C. Lexer, S. Wipf, S. Karrenberg, M. Van Kleunen, and C. Rixen. 2015. The response of the alpine dwarf shrub Salix herbacea to altered snowmelt timing: Lessons from a multi-site transplant experiment. PLoS ONE 10: 1–19.
- Shimono, Y., and G. Kudo. 2003. Intraspecific variations in seedling emergence and survival of Potentilla matsumurae (Rosaceae) between alpine fellfield and snowbed habitats. Annals of Botany 91 (1):21–29. doi:https://doi.org/10.1093/aob/mcg002.
- Søvik, G., H. P. Leinaas, R. A. Ims, and T. Solhøy. 2003. Population dynamics and life history of the oribatid mite Ameronothrus lineatus (Acari, Oribatida) on the high arctic archipelago of Svalbard. Pedobiologia 47(3): 257–71. doi:https://doi.org/10.1078/0031-4056-00189.
- Steinwandter, M., A. Rief, S. Scheu, M. Traugott, and J. Seeber. 2018. Structural and functional characteristics of high alpine soil macro-invertebrate communities. European Journal of Soil Biology 86 ( September 2017):72–80. doi:https://doi.org/10.1016/j.ejsobi.2018.03.006.
- Teets, N. M., and D. L. Denlinger. 2014. Surviving in a frozen desert: Environmental stress physiology of terrestrial Antarctic arthropods. Journal of Experimental Biology 217 (1):84–93. doi:https://doi.org/10.1242/jeb.089490.
- Thibaud, J.-M., H.-J. Schulz, and M. M. Da Gama Assalino. 2004. Synopses on Palaearctic Collembola Pt 4: Hypogastruridae. Goerlitz: Senckenberg Museum of Natural History.
- Tonin, R., R. Gerdol, M. Tomaselli, A. Petraglia, M. Carbognani, and C. Wellstein. 2019. Intraspecific functional trait response to advanced snowmelt suggests increase of growth potential but decrease of seed production in snowbed plant species. Frontiers in Plant Science 10 (March):1–12. doi:https://doi.org/10.3389/fpls.2019.00289.
- Vorkauf, M., A. Kahmen, C. Körner, and E. Hiltbrunner. 2021. Flowering phenology in alpine grassland strongly responds to shifts in snowmelt but weakly to summer drought. Alpine Botany 131 (1):73–88. doi:https://doi.org/10.1007/s00035-021-00252-z.
- Weigmann, G. Freie U.B. 2006. Hornmilben (Oribatida). Tierwelt Deutschlands. Keltern, Germany: Goecke & Evers.
- Winkler, M., P. Illmer, P. Querner, B. M. Fischer, K. Hofmann, A. Lamprecht, N. Praeg, J. Schied, K. Steinbauer, and H. Pauli. 2018. Side by side? Vascular plant, invertebrate, and microorganism distribution patterns along an alpine to nival elevation gradient. Arctic, Antarctic, and Alpine Research 50 (1):e1475951. doi:https://doi.org/10.1080/15230430.2018.1475951.