Abstract
4-Methylimidazole (4-MeI) is a nitrogen-containing heterocyclic compound that is used in the manufacture of chemicals, dyes and pharmaceuticals and may be found in a variety of foods following formation during heating. The purpose of this study was to use two different in silico programs, CASE Ultra and Toxtree, to investigate potential structure-activity relationships in 4-MeI and its metabolites for mutagenicity and carcinogenicity, and combine that information with the available literature to draw conclusions regarding the strength of the predictions observed. Neither CASE Ultra nor Toxtree identified any structural alerts that were associated with mutagenic activity. Data for 4-MeI from a single study were used in the development of the CASE Ultra mouse and rat carcinogenicity models, but no additional similar structures were identified in the carcinogenicity model training set. One metabolite, 5-methylhydantoin, was predicted to be positive in the CASE Ultra carcinogenicity male and female mouse models; positive predictivity percentages of 60.9% and 73.7%, respectively. However, low structural similarity between 5-methylhydantoin and the compounds identified in the training set (<25%) decreases confidence in the positive prediction. Three metabolites were predicted to be positive in the CASE Ultra mouse micronucleus model, but again suffered from low structural similarity. Both limited structural similarity and inconsistent responses among the other clastogenicity models suggest that additional structurally similar compounds are needed to assess the predictive capacity of these alerts for biological activity of these compounds.
1. Introduction
4-Methylimidazole (4-MeI) is a nitrogen-containing heterocyclic that may be formed by reaction between ammonia and reducing sugars (Vollmuth Citation2018). 4-MeI has been used in the manufacture of chemicals, dyes, and pharmaceuticals. Additionally, it may be formed via cooking a variety of foods, including coffee and soy sauce. It also is present in two types of caramel colors (class III and IV) that are used in several products including dark beer, baked goods, soft drinks, and cereals (Folmer et al. Citation2018; Vollmuth Citation2018).
Based on the potential for widespread exposure to 4-MeI, and limited chronic toxicity data, the National Cancer Institute (NCI) nominated 4-MeI to the National Toxicology Program (NTP) for toxicity and carcinogenicity studies. The NTP conducted a long-term carcinogenicity study in B6C3F1 mice and F344/N rats; animals were fed diets containing high concentrations of 4-MeI (0, 312, 625 or 1250 ppm in mice and 0, 625 (males only), 1250, 2500 or 5000 (females only) ppm in rats) for 106 weeks. A statistically significant increase in mouse alveolar/bronchial neoplasms was reported, which led to the NTP classification of ‘clear evidence of carcinogenic activity’ in male and female B6C3F1 mice. This classification was considered not to be consistent with NTP’s classification of other mouse lung carcinogens where a similar strength of lung tumor response was classified as ‘some evidence of activity’ (Haseman Citation2013). In female F344/N rats, NTP determined there was ‘equivocal evidence of carcinogenic activity’ based on an increased incidence of mononuclear cell leukemia. No evidence of carcinogenic activity was noted in male rats (National Toxicology Program Citation2007).
Genetic toxicity studies were conducted in Salmonella typhimurium, rat and mouse bone marrow, and mouse peripheral blood. No evidence of genotoxic effects was reported in S. typhimurium strains TA97, TA98, TA100, or TA1535 with liver S-9 from Aroclor-induced male Sprague-Dawley rats and Syrian hamsters, the rat and mouse bone marrow micronucleus assay, and the mouse peripheral blood micronucleus assay (Chan et al. Citation2004; National Toxicology Program Citation2007).
Based on these NTP studies, the current no significant risk level for 4-MeI under the California law Proposition 65 is 29 µg/day. The European Food Safety Authority (EFSA) identified the intermediate dose (625 mg/kg diet) in the NTP carcinogenicity study as the no adverse effect level; this dose was equivalent to 80 mg/kg body weight/day (Morita and Uneyama Citation2016). 4-MeI concentrations in food and beverages were reported to range from not detected to 28 mg/kg (International Agency for Research on Cancer Citation2013). Smith and colleagues (Citation2015) reported that the mean concentration of 4-MeI in soft drink samples ranged from less than the limit of quantitation to 916 µg/L. Folmer and colleagues reported that cumulative exposure to 4-MeI in the U.S. population at least 2 years of age, ranged from 0.06 to 0.09 mg/person/day when using 2-day dietary intake survey data from the 2007 to 2010 National Health and Nutrition Examination Survey (NHANES). When exposure estimates from 10- to 14-day food diaries from the NPD Group, Inc. National Eating Trends-Nutrient Intake Database (NPD NET-NID) source were used, the calculated cumulative exposure intake ranged from 0.06 to 0.08 mg/person/day for the same population (Folmer et al. Citation2014). A more recent analysis, using 2009–2012 NHANES and NPD NET-IND sources, reported mean combined 4-MeI exposure levels of 0.027–0.077 and 0.025–0.070 mg/person/day, respectively (Folmer et al. Citation2018).
Fennell and colleagues (2019) investigated potential species differences associated with 4-MeI metabolism in vitro and in vivo. In vitro studies were conducted using F344/N rat and B6C3F1 mouse lung and liver microsomes and lung S-9 fractions. In vivo studies were conducted in the same strain of rats and mice, which were administered 50 or 150 mg/kg-bw [14C]-4-MeI via oral gavage. 4-MeI was not oxidized in vitro in the subcellular lung and liver preparations. Elimination of [14C]-4-MeI in vivo was mainly via the urine, with 79–89% of the radioactivity recovered in the urine of rats and 41–70% recovered in the urine of mice, within 48 h of dosing. Total recovery of radioactivity derived from [14C]-4-MeI (the sum of excreta, tissues, carcass and cage rinse) was 95–98% of administered 4-MeI dose in rats and 92–96% of administered 4-MeI dose in mice. Most of this radioactivity was identified as unchanged 4-MeI; 73–78% in rats and 60–77% in mice. High resolution liquid chromatography-mass spectrometry (LC-MS) was used to identify the metabolites of 4-MeI in rat urine: 4-hydroxymethylimidazole (1.5–1.9%), a mono-glucuronide conjugate, 4-hydroxymethylimidazole glucuronide (0.5–0.7%), 2-methylhydantoic acid (values not provided), and 5-methylhydantoinFootnote1 (8.1–13.7%) at the 50 mg/kg-bw dose. Similar metabolites were detected by LC-MS in mouse urine: 4-hydroxymethylimidazole (2.4–4.8%), a mono-glucuronide conjugate, 4-hydroxymethylimidazole glucuronide (3.8–6.6%) and 5-methylhydantoin (16.7–20.9%) at the 50 mg/kg-bw dose. Thus, 4-MeI metabolism appeared to occur through similar pathways in rats and mice (Fennell et al. Citation2019).
Structure-activity relationship (SAR) studies have been increasingly used to identify molecular features that are associated with a variety of toxic endpoints, including carcinogenicity and genotoxicity. To further understand the mode of action associated with induction of mouse lung tumor formation following chronic exposure to high concentrations of 4-MeI in the diet, two SAR programs were utilized to identify potential carcinogenic and/or genotoxic structural alerts and features present in 4-MeI and metabolites identified by Fennell et al. (Citation2019). Structural features combined with the available literature for the compounds of interest, were used to draw conclusions regarding the strength of the predictions observed using carcinogenicity and genotoxicity SAR models.
2. Materials and methods
2.1. Chemicals and structures
Chemical abstract services registry numbers (CASRNs), chemical names, simplified molecular-input line-entry system (SMILES) strings and chemical structures, obtained from the study of Fennell et al. (Citation2019) and confirmed in ChemIDPlus, of the compounds of interest are shown in .
Table 1. Chemical information on 4-methylimidazole and metabolites.
2.2. Computational software
2.2.1. CASE Ultra
The carcinogenicity and genotoxicity modules in CASE Ultra software (v. 1.6.2.3; MultiCASE Inc.) were used to predict the carcinogenic and genotoxic potential of 4-MeI and the metabolites listed in . Names and descriptions of the 14 carcinogenicity and genotoxicity models used for this evaluation are provided in . The SMILES string for each chemical was entered into the CASE Ultra software to conduct the predictions. Predictions from all 14 models for each compound were retrieved for analysis.
Table 2. CASE Ultra model descriptions and inactive and active thresholds.
The predictions identify structural alerts that are associated with ‘positive’ or ‘negative’ activity that is represented by the model. CASE Ultra implements the Computer Automated Structure Evaluation (CASE) approach, which is based on the use of molecular skeletal fragments as the molecular descriptor. The structural alerts are based on the chemicals within the training set of each model. Each alert is assigned a contribution coefficient that is obtained during model training and development and may be modified based on the presence of positive and/or negative modulators. In cases where there is more than one alert, the individual models are combined to produce an overall probability of a compound being positive in the evaluated model (e.g. positive in the female mouse rodent carcinogenicity model).
The calculated probability is compared to the inactive and active threshold values, predefined by the CASE Ultra program, for each model to classify the chemical as ‘positive,’ ‘known positive,’ ‘negative,’ ‘known negative,’ ‘inconclusive,’ or ‘out of domain’. A chemical is defined as a ‘known positive’ or ‘known negative’ when data from the chemical has been used in model development. If experimental data are available, predicted results are superseded by the available data. A chemical is identified as ‘positive’ or ‘negative’ when the calculated probability is greater than the active threshold or below the inactive threshold, respectively (). If the calculated probability falls between the inactive and active thresholds, the chemical is identified as ‘inconclusive’. This indicates that prediction probabilities within this range are marginal and model results are not reliable for identification of a positive or negative response. A chemical identified as ‘out of domain’ indicates that the chemical structure does not fall within the domain of applicability of the model. The criteria used for each classification is provided in .
Table 3. CASE Ultra criteria for classification.
2.2.2. Toxtree
Toxtree (v. 3.1.0) is an open-source tool that was commissioned by the European Commission’s Joint Research Center (JRC) and developed in conjunction with Ideaconsult Ltd. The program uses a decision tree model to predict a variety of toxic endpoints. Toxtree was used to identify structural alerts in the five compounds of interest using several rulebases in the software. The models used for this evaluation, along with a brief description, are provided in . Output from Toxtree includes the alert that is flagged by a module or ‘none’ if no alerts are flagged.
Table 4. Toxtree modules.
3. Results
3.1. CASE ultra model predictions
CASE Ultra (v. 1.6.2.3) was used to predict the potential carcinogenicity and genotoxicity of 4-MeI, and four putative metabolites: 4-hydroxymethylimidazole, a mono-glucuronide conjugate of 4-hydroxymethylimidazole, 2-methylhydantoic acid, and 5-methylhydantoin. A summary of the SAR results for potential carcinogenicity, mutagenicity, and clastogenicity are provided in , respectively, and the structural alerts identified by CASE Ultra are presented in .
Figure 1. Structural alerts from CASE Ultra for 4-methylimidazole and metabolites. ‘Identified structural alert’ column shows the structure of the positive alert identified for the noted chemical within the specified CASE Ultra Model. The ‘Identified structural alert ID’ column provides the ID number used by the model for the shown positive alert. Therefore, the same positive alert may have different ID numbers for different models (i.e. Alert 269 and Alert 252 represent the same structure in two different CASE Ultra models).
Table 5. CASE Ultra carcinogenicity SAR analysis.
Table 6. CASE Ultra mutagenicity SAR analysis.
Table 7. CASE Ultra clastogenicity SAR analysis.
3.1.1. Carcinogenicity predictions
4-MeI is contained within the training set that CASE Ultra used to develop the models utilized in this analysis, and, the available in vivo data from the NTP cancer bioassay were used to classify this compound in the models (National Toxicology Program Citation2007). The presence of in vivo data was used to override the calculated prediction probability (27.4%; which would otherwise classify the chemical as a ‘negative’) and the chemical was listed as ‘known positive’ in the male and female mouse carcinogenicity models (). A single structural alert was identified within 4-MeI, i.e. 4-MeI itself (Alert 269 in the female mouse carcinogenicity model and Alert 252 in the male mouse carcinogenicity model; see ). No other structurally similar compounds were identified from the male and female mouse carcinogenicity model training sets. Based on the same in vivo data, 4-MeI was identified as a ‘known negative’ in the male and female rat carcinogenicity models.
Of the remaining chemicals, 5-methylhydantoin was predicted to be ‘positive’ in the male (60.9%) and female (73.7%) mouse carcinogenicity models, ‘negative’ in the male rat carcinogenicity model, and ‘inconclusive’ in the female rat carcinogenicity model. The other three metabolites: 4- hydroxymethylimidazole, the mono-glucuronide conjugate of 4-hydroxymethylimidazole and 2-methylhydantoic acid, were all predicted to be negative in the male and female mouse and rat carcinogenicity models ().
For 5-methylhydantion, structural alerts 124 and 179 were identified for the CARC MOUSE F model and structural alert 235 was identified for the CARC MOUSE M model. All the noted structural alerts represent varying fragments located within the hydantoin ring (). No additional structural alerts or features associated with carcinogenic activity were present in 5-methylhydantoin. presents the six model training set chemicals with the highest structural similarity to 5-methylhydantoin, for each identified structural alert. The training set chemicals had a structural similarity (as calculated using the Tanimoto coefficient) of ≤22% to 5-methylhydantoin.
Figure 2. Structurally similar chemicals from the model training sets for Alerts 124 (A), 179 (B) and 235 (C). The green highlight in chemical structures indicates the identified structural alert. The purple highlight in chemical structures indicates the presence of additional structural alerts that are associated with activity, but are not present in the query chemical.
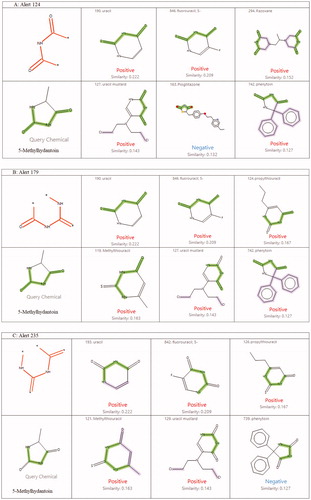
3.1.2. Mutagenicity and clastogenicity predictions
4-MeI was predicted to be ‘negative’ or identified as a ‘known negative’ in the expert rule-based and statistical-based mutagenicity models (see ). All four metabolites were also predicted to be ‘negative’ in the CASE Ultra expert rule-based and statistical-based mutagenicity models.
Mixed results were obtained from the four CASE Ultra models that represent a clastogenic mode of action (). 4-Hydroxymethylimidazole, 2-methylhydantoic acid and 5-methylhydantoin were predicted to be ‘positive’ only in the GT3 MNT MOUSE model, with positive predictive values of 58.8%, 61.1% and 73.9%, respectively. The parent compound, 4-MeI, is contained in the training set for the model and the available data were used to classify this compound as ‘known negative’ in this model. The remaining chemicals were either predicted as ‘negative’ or ‘out of domain’ for this and the remaining models ().
Structural alert 252, a fragment of the imidazole ring, was identified in 4-hydroxymethylimidazole. Structural alert 1, a primary carboxylic acid amine fragment, was identified in 2-methylhydantoic acid. Structural alert 93, a hydantoin ring fragment, and structural alert 153, an isopropyl methyl ketone fragment, were identified in 5-methylhydantoin. The structural similarities of 4-hydroxymethylimidazole, 2-methylhydantoic acid or 5-methylhydantoin to training set chemicals that contained the identified structural alerts were low (i.e. ≤21% structural similarity). Additionally, several of the compounds identified as structurally similar have additional alerts that are not shared with the compounds of interest (data not shown).
3.2. Toxtree model predictions
3.2.1. Benigni/bossa rulebase
The Benigni/Bossa rulebase for mutagenicity and carcinogenicity identifies a list of structural alerts for carcinogenicity. These structural alerts identify molecular functional groups or structures that are linked to carcinogenic activity. The list of structural alerts includes genotoxic and non-genotoxic modes of action (Benigni et al. Citation2008). A structural alert for imidazole and benzimidazole compounds was identified for the parent compound and two metabolites: 4-hydroxymethylimidazole and its glucuronide conjugate.
3.2.2. Structure alerts for the in vivo micronucleus assay in rodents
The module for evaluating the in vivo micronucleus assay in rodents identifies structural alerts associated with chemicals identified as positive in the in vivo micronucleus assay. The same structural alert was identified in all five chemicals: ‘SA34 H-acceptor path3 H acceptor’. This structural alert (see ) is composed of two hydrogen bond acceptors that are linked by any two atoms (except hydrogen). This was the only structural alert identified in this module.
3.2.3. Protein binding alerts
The protein-binding alert module identifies structural alerts associated with covalent protein binding. The nucleophilic substitution type 2 (SN2) reaction alert was flagged in the rulebase for two of the metabolites: 4-hydroxymethylimidazole and its mono-glucuronide conjugate.
3.2.4. In vitro mutagenicity (ames test) alerts
No structural alerts were identified for any of the five compounds in the in vitro mutagenicity module. This finding is consistent with predictions from CASE Ultra, which predicts that these compounds are not mutagenic.
3.2.5. Structural alerts for identification of Michael acceptors
Michael addition is one specific type of chemical reaction that can produce a covalent bond between a chemical and a protein. The structural alerts for Michael acceptors have been separated out from the protein binding module (discussed in 3.2.3), into a separate module in Toxtree. No structural alerts were identified for any of the five compounds in the Michael acceptors module.
3.2.6. DNA binding alerts
No structural alerts were identified for any of the five compounds in the DNA binding module. This prediction is consistent with the outcomes from CASE Ultra, which predicts that these compounds are not mutagenic.
4. Discussion
SAR evaluations have been increasingly used to assess and identify structural features associated with a variety of chemical toxicities, including genotoxicity and carcinogenicity. Commercial and open source software packages are available to assess toxicity based on structural alerts and physico-chemical properties. The model results, however, cannot be used in isolation and must be connected to biological knowledgebases to fully understand the potential chemical toxicity. To this end, we evaluated the presence of structural alerts in 4-MeI and four identified rodent metabolites and connected those results to known biological responses. CASE Ultra and Toxtree models were utilized to evaluate the potential carcinogenicity and presence of structural fragments of 4-MeI and four known metabolites.
The calculated probabilities of 4-MeI being a carcinogen in the CASE Ultra male and female mouse models were 26.2% and 27.4%, respectively. In the male and female rat models, the calculated prediction probabilities were 29.2% and 25.3%, respectively. All probabilities were below the inactive threshold (30%) for the models, which would have led to the chemical being predicted to be ‘negative’ in all the CASE Ultra carcinogenicity models. However, CASE Ultra identified 4-MeI as a ‘known positive’ or ‘known negative’ in mice and rats, respectively. Inclusion of the NTP study that identified 4-MeI as a carcinogen in model development led to the overriding of the predicted classification.
The structural alert identified for 4-MeI in the male and female mouse carcinogenicity models was a methylated imidazole ring (alerts 252 and 269, respectively). Other than 4-MeI, no additional compounds in the training sets of either mouse model contained the noted structural alerts. Furthermore, the only data used for this carcinogenclassification was that of the NTP study (2007) previously discussed. Therefore, the outcome reported by CASE Ultra is driven by the limited in vivo data available and is not supported by additional chemicals, with the same or similar structural alerts, in the models’ training sets.
In 2014, Krishna and colleagues reported SAR results for 4-MeI using computational models Osiris, Toxtree, and DEREK to predict genotoxic and tumorigenic potential. For all three programs, 4-MeI was predicted to be ‘negative’ in the genotoxicity models. Comparatively, 4-MeI was predicted to be ‘positive’ in the Osiris tumorigenicity model but ‘negative’ in the Toxtree and DEREK models. Similar to the reported CASE Ultra results, it appears that 4-MeI was predicted to be tumorigenic in the Osiris model because the chemical itself was used in model development (Krishna et al. Citation2014).
One inconsistency in Toxtree results was noted between Krishna et al. (Citation2014) and the results described in this paper. In the present analysis, a structural alert for imidazole and benzimidazole compounds was identified in 4-MeI with the Benigni/Bossa rulebase for mutagenicity and carcinogenicity. Comparatively, ‘no alerts for carcinogenic activity’ were noted when the same model was evaluated by Krishna et al. The inconsistency is likely associated with the versions of the Toxtree program that were used in the two analyses. The identified structural alert (‘SA49 nongen.imidazole and benzimidazole’) in this study was described in a paper that was published after the release date of version 2.5.0 (Benigni et al. Citation2013), which was used by Krishna et al., and may explain the difference in alerts presented in the two evaluations.
For all but one of the metabolites, the positive predictive potential for each of the evaluated CASE Ultra carcinogenicity models was below the inactive threshold (30%). 5-Methylhydantoin was predicted to be positive in the male and female mouse carcinogenicity models with predicted probabilities of 60.9% and 73.7%, respectively. While the predicted probability of being positive is greater than the active threshold for the models (50%), there are some factors that decrease confidence in the predictions. Low structural similarity of 5-methylhydantoin to the chemicals within the model training sets is noted (≤22%). Additionally, several of the chemicals that were identified as containing the same structural feature as observed in 5-methylhydantoin contained additional structural alerts (e.g. alkyl chloro groups, furan rings and phenyl rings) that were not present in 5-methylhydantoin. While structural alerts can be associated with specific types of toxicity, structural fragments should not be seen as toxic effects. Structural fragments can serve as indicators of potential hazards, but the reactivity of the fragment, and ultimately the compound as a whole, is known to be dependent on the presence and positioning of other substituents (Alves et al. Citation2016). The low degree of structural similarity of 5-methylhydantoin to chemicals used in model development, combined with the limited structural alerts present in the chemical, decreases the confidence in the reported positive prediction for 5-methylhydantoin.
Neither the CASE Ultra models nor the Toxtree mutagenicity model (AMES assay) identified structural alerts that were associated with mutagenic activity. All the CASE Ultra models (expert rule-based and statistical-based) identified the compounds of interest as either ‘known negative’ or ‘negative’. Several in vitro studies are consistent with the prediction that 4-MeI was not a mutagen or genotoxicant. For example, studies by Beevers and Adamson (Citation2016) and Chan et al. (2004; NTP 2007) showed that 4-MeI was not mutagenic in S. typhimurium TA97, TA98, TA100, TA102, TA1535 or TA1537 in the absence or presence of S9. 4-MeI also failed to induce formation of micronucleated erythrocytes after intraperitoneal injection or oral exposure (Chan et al. 2004; National Toxicology Program Citation2007). Voogd and colleagues (Citation1979) evaluated a series of nitroimidazoles and imidazoles to assess mutagenic potential. Results suggested that the imidazole feature itself does not induce direct mutagenic activity. Thus, the models’ predictions are consistent with the in vitro and in vivo studies available in the literature.
The outcomes from the four CASE Ultra models that represent a clastogenic mode of action were mixed, with all but one model returning predictions of ‘negative’, ‘out of domain’ or ‘inconclusive.’ Only the mouse micronucleus assay model (GT3 MNT MOUSE) predicted any of the compounds of interest to be positive. 4-MeI was listed as a ‘known negative’ in this model because the experimental data were used in the development of the model. While three of the four metabolites (4-hydroxymethylimidazole, 2-methylhydantoic acid, and 5-methylhydantoin) were predicted to be positive in the GT3 MNT MOUSE model, there are limitations with these predictions. The structural similarity of these three metabolites to the chemicals within the mouse micronucleus model training set were ≤21% (as determined by the Tanimoto coefficient). Similar to what was observed with 5-methylhydantoin in the carcinogenicity models, several of the chemicals that were identified as containing the same structural alerts observed in the metabolites of interest, contained additional structural alerts that were not present in these three chemicals. These factors, combined with the lack of concordance with the CASE Ultra clastogenicity models (i.e. GT2 CHROM CHL, GT2 CHROM CHO, and GT4 L5178Y), decreases the confidence in the positive prediction in the GT3 MNT MOUSE model.
In Toxtree, an alert for a nongenotoxic mechanism of action was flagged in the Benigni/Bossa rulebase for 4-hydroxymethylimidazole and the glucuronide conjugate. A proton acceptor alert was flagged in the in vivo micronucleus assay in the rodent module for all the tested chemicals and a nucleophilic substitution type 2 (SN2) reaction alert was flagged in the protein binding alerts rulebase. No other structural alerts were identified in the remaining Toxtree modules.
The alert identified in the Benigni/Bossa rulebase is associated with a non-genotoxic mechanism of action. Benigni et al. (Citation2013) described this alert as being associated with a mechanism of action of hormonal imbalance and a pathway where secretion of trophic hormones is increased, which in turn leads to increased cell proliferation. This may lead to the development of tumors through the increased number of cell divisions and greater opportunity for introducing random genetic errors (Benigni et al. Citation2013). This process has been reported for agents that induce thyroid or testicular tumors. However, those tumor types were not associated with dietary exposure to 4-MeI in mice or rats (National Toxicology Program Citation2007). Furthermore, 4-MeI did not increase cell proliferation, as measured by bromodeoxyuridine (BrdU) incorporation, in mouse lung cells (Cruzan et al. Citation2015).
One structural alert (SA34 H-acceptor path3 H acceptor) was identified in one module of Toxtree (in vivo micronucleus assay in rodents) for all five compounds of interest. It is noted in the model documentation that the true positive rate of the individual structural alert is relatively low. For the structural alert identified in 4-MeI and the four metabolites, a true positive rate of 34% was noted (55/163). This suggests that the toxic potential of such alerts may not always be expressed in an experimental system (Benigni et al. Citation2009).
The nucleophilic substitution type 2 (SN2) reaction alert was identified in the protein-binding alerts rulebase for two of the metabolites: 4-hydroxymethylimidazole and its glucuronide conjugate. This alert is for a mechanism of protein binding that is a common feature of compounds that undergo chemical reactions and on its own, does not provide strong evidence that a compound will produce adverse effects (Enoch et al. Citation2011).
In conclusion, the predictions from the CASE Ultra and Toxtree models suggest that 4-MeI does not possess structural features that are associated with mutagenic effects in vitro and in vivo. These predictions are consistent with the available published literature on the lack of mutagenic effect of 4-MeI. Predicted carcinogenicity outcomes for 4-MeI were driven by inclusion of 4-MeI data in model development (i.e. single study that identified 4-MeI as a carcinogen). The combined lack of supporting carcinogenicity studies on 4-MeI and the lack of structures similar to 4-MeI in the training sets decreases confidence in the carcinogenicity predictions. Confidence in positive model predictions for metabolites also was decreased by the low structural similarity to training set compounds and the presence of one or two structural alerts that on their own, are not strong predictors of biological activity. Additional research using compounds with greater structural similarity to 4-MeI to understand how their structure translates to biological activity could be useful in providing additional context to the results obtained.
Acknowledgements
The authors wish to acknowledge Ms. Katie Roberts and Ms. Catherine Sprankle for their assistance with technical editing of the manuscript.
Disclosure statement
This work received financial support from the American Beverage Association, which was not involved in study design, data collection, data analysis, data interpretation, or writing of the manuscript. The funding source approved submission of the article for publication. The authors declare that they have no conflicting financial interest in the outcome of this study.
Notes
1 Compound was misidentified as 4-methylhydantoin in Fennell et al. Citation2018 (personal communication).
References
- Alves V, Muratov E, Capuzzi S, Politi R, Low Y, Braga R, Zakharov AV, Sedykh A, Mokshyna E, Farag S, et al. 2016. Alarms about structural alerts. Green Chem. 18(16):4348–4360.
- Beevers C, Adamson RH. 2016. Evaluation of 4-methylimidazole, in the Ames/Salmonella test using induced rodent liver and lung S9. Environ Mol Mutagen. 57(1):51–57.
- Benigni R, Bossa C, Tcheremenskaia O. 2013. Nongenotoxic carcinogenicity of chemicals: mechanisms of action and early recognition through a new set of structural alerts. Chem Rev. 113(5):2940–2957.
- Benigni R, Bossa C, Tcheremenskaia O, Worth A. 2009. Development of structural alerts for the in vivo micronucleus assay in rodents. Luxembourg: Office for Official Publications of the European Communities.
- Benigni R, Bossa C, Jeliazkova N, Netzeva T, Worth A. 2008. The Benigni/Bossa rulebase for mutagenicity and carcinogenicity – a module of Toxtree. Luxembourg: Office for Official Publications of the European Communities.
- Cruzan G, Harkema JR, Hosako H, Wasil JM, Murray FJ. 2015. Evaluation of the mode of action of mouse lung tumors induced by 4-methylimidazole. Regul Toxicol Pharmacol. 73(2):501–508.
- Enoch SJ, Ellison CM, Schultz TW, Cronin MT. 2011. A review of the electrophilic reaction chemistry involved in covalent protein binding relevant to toxicity. Crit Rev Toxicol. 41(9):783–802.
- Fennell TR, Watson SL, Dhungana S, Snyder RW. 2019. Metabolism of 4-methylimidazole in Fischer 344 rats and B6C3F1 mice. Food Chem Toxicol. 123:181–194.
- Folmer DE, Doell DL, Lee HS, Noonan GO, Carberry SE. 2018. A U.S. population dietary exposure assessment for 4-methylimidazole (4-MeI) from foods containing caramel colour and from formation of 4-MeI through the thermal treatment of food. Food Addit Contam Part A Chem Anal Control Expo Risk Assess. 35(10):1890–1910.
- Folmer D, Doell D, Lee H, Noonan G, Carberry S. 2014. Refined exposure assessment for 4-MEI for the U.S. Population based on quantitative data from foods containing caramel color. Available from: https://iacmcolor.org/wp-content/uploads/2014/08/2014-ACS-4-MEI-Poster_Folmer_Final.pdf.
- Haseman JK. 2013. Evaluating consistency in the interpretation of NTP rodent cancer bioassays: an examination of mouse lung tumor effects in the 4-MEI study. Regul Toxicol Pharmacol. 66(1):109–115.
- International Agency for Research on Cancer. 2013. 4-Methylimidazole. Geneva, Switzerland: WHO Press.
- Krishna KA, Goel S, Krishna G. 2014. SAR genotoxicity and tumorigenicity predictions for 2-MI and 4-MI using multiple SAR software. Toxicol Mech Methods. 24(4):284–293.
- Morita T, Uneyama C. 2016. Genotoxicity assessment of 4-methylimidazole: regulatory perspectives. Genes Environ. 38:20.
- Chan PC; National Toxicology Program, US Department of Health and Human Services, Public Health Service, National Institutes of Health. 2004. NTP technical report on the toxicity studies of 2- and 4-methylimidazole (Cas No. 693-98-1 and 822-36-6) administered in feed to F344/N rats and B6C3F1 mice. Natl Toxicol Program Tech Rep Ser. (67):1–G12.
- National Toxicology Program. 2007. Toxicology and carcinogenesis studies of 4-methylimidazole (Cas No. 822-36-6) in F344/N rats and B6C3F1 mice (feed studies). Natl Toxicol Program Tech Rep Ser. (535):1–274.
- Smith TJ, Wolfson JA, Jiao D, Crupain MJ, Rangan U, Sapkota A, Bleich SN, Nachman KE. 2015. Caramel color in soft drinks and exposure to 4-methylimidazole: a quantitative risk assessment. PLoS One. 10(2):e0118138.
- Vollmuth TA. 2018. Caramel color safety – an update. Food Chem Toxicol. 111:578–596.
- Voogd CE, Van Der Stel JJ, Jacobs JA. 1979. Mutagenic action of nitroimidazoles. IV. Comparison of the mutagenic action of several nitroimidazoles and some imidazoles. Mutat Res. 66(3):207–221.