Abstract
Castration resistance is a major issue during castration therapy for prostate cancer and thus more effective treatment are needed for castration-resistant prostate cancer (CRPC). NDRG2 (N-Myc downstream regulated gene 2), a recently identified tumor suppressor, was previously shown to inhibit the proliferation and invasion of prostate cancer, but whether NDRG2 is involved in CRPC remains to be known. Because androgen receptor (AR) axis plays an important role in castration resistance, we evaluate the role of NDRG2 in AR signaling and CRPC. Immunohistochemistry examination of prostate cancer tissues demonstrated that the expression of NDRG2 is negatively correlated with that of AR and c-Myc. Furthermore, AR negatively regulates NDRG2, as well as alters levels of c-Myc and prostate specific antigen (PSA). Forced expression of NDRG2 significantly inhibits the in vitro growth of androgen-dependent and castration-resistant prostate cancer cells; this was accompanied by alterations in PSA, but not by those of AR and c-Myc. Finally, by mimicking castration therapy in a xenograft mouse model, we showed that lentivirus-mediated NDRG2 overexpression efficiently overcomes castration resistance. Thus, by acting as a negative regulator downstream of AR, NDRG2 may emerge as a potential therapy molecule for CRPC.
Abbreviations
AR | = | Androgen Receptor |
CRPC | = | Castration-Resistant Prostate Cancer |
DHT | = | Dihydrotestosterone |
NDRG2 | = | N-Myc Downstream Regulated Gene 2 |
PC | = | Prostate Cancer |
Introduction
Prostate cancer (PC) is the most frequently diagnosed cancer among men. With the development of diagnostic techniques, the detection rate of PC has increased significantly in recent years.Citation1,2 If the cancer is detected early and is confined within the prostatic capsule, it can be cured by surgery or radiotherapy (including brachytherapy).Citation3 But the prognosis is often poor if metastasis has already occurred at the time of diagnosis. Currently, the mainstay of therapy for metastatic prostate cancer is castration, which can be accomplished by either orchectomy or androgen-antagonistic agents.Citation4 However, although castration is initially effective and prolongs the period free of disease progression, PC eventually becomes castration resistant, resulting in disease relapse and less than 25 months of median survival.Citation5
Androgen receptor (AR) is a ligand-dependent transcription factor that plays important roles in both normal prostate development and prostate cancer. When activated by endogenous androgen, testosterone and dihydrotestosterone (DHT), AR regulates the growth, survival and differentiation of prostate cells by transactivation of a subset of target genes.Citation6 Several mechanisms underlying castration resistance have been documented, including (a) AR mutations or amplification, (b) alterations in the balance between AR and its transcriptional co-regulators and (c) growth factors/kinases signal pathways that activate AR activity at post-castration androgen levels.Citation7-11 It is noteworthy that CRPC cells retain AR and AR-regulated gene expression in the absence of or with low levels of circulating androgens.Citation12 In addition, tumors that are refractory to conventional androgen deprivation therapy are susceptible to more potent androgen pathway inhibitors.Citation13,14 These studies strongly indicate that AR signaling continues to play a significant role in CRPC. Thus, more comprehensive understanding of the regulation of the AR axis and the development of novel AR signaling-targeting therapies is imperative in this field.
The human N-Myc downstream regulated gene 2 (NDRG2), which was first cloned in our laboratory in 1999 (GenBank accession no.AF 159092),Citation15 belongs to the NDRG gene family, consisting of NDRG1–4.Citation16 The NDRGs share roughly 60% amino acid identity and are highly conserved among species.Citation15 Previous studies from our group and others have demonstrated that NDRG2 is involved in cell differentiation,Citation17 proliferation,Citation18 apoptosisCitation19,20 and the stress response.Citation21 NDRG2 in particular has been proposed as a tumor suppressorCitation21 because mounting evidence demonstrates that NDRG2 is deregulated in various carcinomas, including glioblastoma,Citation15 breast cancer,Citation22 hepatocellular cancerCitation23 and colorectal cancer,Citation24 while upregulating NDRG2 could reverse the malignant phenotype of cancer cells.Citation21,25,26 Our most recent studies showed that NDRG2 expression levels were lower in PC tissues than in their benign counterparts, and forced expression of NDRG2 inhibited the proliferation and invasion of PC cells.Citation27,28 Particularly, NDRG2 inhibited proliferation of PC cell line PC3 by affecting several cell cycle regulators (cyclin-D1, CDK4 and p21),Citation27 supporting that NDRG2 plays a tumor suppressive role in PC. We previously confirmed that NDRG2 is repressed by c-Myc.Citation17 Of note, it was also shown that c-Myc is a known downstream target of AR and confers androgen-independent PC cell growth.Citation29,30 These findings prompt us to question whether NDRG2, as a newly identified c-Myc target gene, is also involved in AR-mediated castration resistance.
Results
The expression of NDRG2, c-Myc and AR are correlated in PC tissues
We previously showed that the expression level of NDRG2 is negatively correlated with those of c-Myc in prostate cancer tissues,Citation27 consistent with our previous finding that NDRG2 is repressed by c-Myc in transcriptional level.Citation17 Considering that c-Myc is a known target gene of AR,Citation29,30 we hypothesized that NDRG2 may be involved in AR signaling. Therefore, we first examined PC samples to see if there is any correlation among the expression of NDRG2, c-Myc and AR. For this purpose, 107 PC tissue sections from PC patients were analyzed with immunohistochemistry. The results showed that the rates of positive NDRG2, c-Myc and AR expression in PC specimens were 29.91% (32/107), 33.64% (36/107) and 71.96% (77/107), respectively. All three proteins were expressed both in the cytoplasm and in the nuclei of PC cells. NDRG2 protein was mainly expressed in the cytoplasm, but the c-Myc and AR proteins were expressed mainly in the nuclei (). Moreover, the expression levels of NDRG2 were negatively correlated with those of c-Myc and those of AR (), while the expression levels of c-Myc and AR were positively correlated (). These data suggest that there might be some regulatory relationship between NDRG2, c-Myc and AR.
Figure 1. The expression levels of NDRG2 were negatively correlated with those of c-Myc and AR in prostate cancer tissues. (A) Immunohistochemical staining of the sections from human prostate cancer tissues with anti-NDRG2 (a, d), anti-AR (b, e) and anti-c-Myc (c, f) antibodies (Scale bar: 20 μm). The sections in a, b, c and those in d, e, f originated from the same paraffin blocks, respectively. Gleason score: a, b, c: 2 + 2 = 4; d, e, f: 3 + 4 = 7. (B) Statistical analysis of the expression levels among NDRG2, c-Myc and AR. The number of specimens is shown using ‘scale’. Disparities across groups were analyzed using the Pearson correlation coefficient, and the graph was generated using SPSS13.0 software (a: r = -0.252, P < 0.01; b: r = -0.277, P < 0.01; c: r = 0.610, P < 0.01).
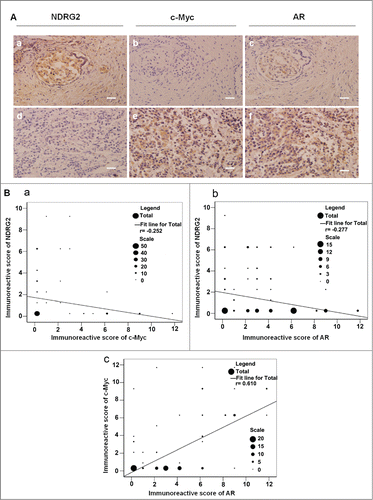
NDRG2 is regulated by AR and is involved in the AR signaling pathway
To explore the involvement of NDRG2 in AR signaling and AR-mediated castration resistance, LNCaP and 22Rv1 cell lines were chosen in this study. LNCaP is an androgen-dependent prostate cancer cell line that express mutant full-length AR; while 22Rv1 is a model of CRPC that expresses full-length mutated AR (110KDa) and constitutively active AR splice variants (80KDa).Citation31–34 We first asked whether NDRG2 can be regulated by AR signaling. DHT or bicalutamide were applied to stimulate or inhibit AR signaling. As expected, cell growth curves, EdU proliferation assay and FCM analysis confirmed that DHT stimulated, but bicalutamide inhibited, the cell growth of LNCaP and 22Rv1 ( and Supplementary Fig. 1). The levels of AR, c-Myc, NDRG2 and PSA were assessed by Western blotting. As shown in , DHT upregulated, while bicalutamide downregulated, the expression of AR compared with the corresponding controls in both LNCaP and 22Rv1 cells. Moreover, the immunofluorescence assay showed that DHT stimulation led to an increase in AR nucleic localization, while bicalutamide exerted an opposite effect (Supplementary Fig. 2). As expected, the upregulation of AR expression and its nuclear localization caused by DHT was accompanied by an increase in its known downstream axis, c-Myc and PSA. Meanwhile, a decrease in NDRG2 was observed in both cell lines upon AR activation by DHT (). In contrast, bicalutamide-mediated downregulation of AR was accompanied by an increase in NDRG2 as well as the opposite changes in AR axis, c-Myc and PSA (), with the alteration of secreted PSA being confirmed by the ELISA results (). Taken together with the published evidences showing that c-Myc is a known downstream target of AR and confers androgen-independent PC cell growthCitation29,30 while NDRG2 can be repressed by c-Myc,Citation17 these data suggest that NDRG2 may be regulated by AR through c-Myc and involved in the AR signaling pathway.
Figure 2. AR activation or inhibition leads to alterations in AR, NDRG2, c-Myc and PSA in LNCaP and 22Rv1 cells. LNCaP and 22Rv1 cells were treated with vehicle controls (Ethanol or DMSO), DHT (10 nM), or bicalutamide (10 μM). (A) Cell growth curves were drawn based on the MTT assay (n = 6). (B) Western blot analysis of AR, c-Myc, NDRG2 and PSA protein levels with the corresponding antibodies. α-Tubulin protein levels were used as a loading control. Representative blots are shown, and the relative expression levels of the proteins normalized to α-tubulin were derived from 4 independent experiments (n = 4). (C) ELISA analysis of PSA secretion. The histograms were drawn based on the average values (n = 3). In A, B, and D, data indicate the mean ± SD *: P < 0.05; **: P < 0.01.
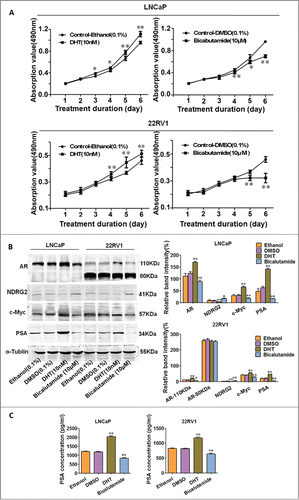
Forced expression of NDRG2 inhibits downstream AR signaling but not affects AR abundance and activity
Next, we asked whether NDRG2 affects AR and its downstream signaling. To this end, we utilized lentivirus system to overexpress NDRG2 in LNCaP and 22Rv1 and then examined the changes in AR's expression and activity as well as its downstream axis. A high efficiency of lentivirus infection in LNCaP and 22Rv1 cells was demonstrated by the fact that almost 100% of Lenti-Cherry-infected cells express cherry proteins (Supplementary Fig. 3). The Western blot results showed that Lenti-NDRG2-infected LNCaP and 22Rv1 cells express higher levels of NDRG2 protein than the controls (). Of note, NDRG2 overexpression neither has any influence on the expression levels of AR and c-Myc () nor changes the nuclear localization pattern of AR () in both LNCaP and 22Rv1 cells. This indicates that NDRG2 does not affect the quantity and nuclear translocation of AR. However, Western blot showed that NDRG2 overexpression led to a downregulation of PSA expression in both LNCaP and 22Rv1 cells (). This was further confirmed by ELISA, showing that the overexpression of NDRG2 decreased the levels of secreted PSA (). Taken together, these results imply that NDRG2 can be repressed by AR/ c-Myc as a target of c-Myc, and importantly, NDRG2 negatively regulates AR downstream signaling pathway without affecting the quantity or activity of AR itself.
Figure 3. Overexpression of NDRG2 causes downregulation of PSA but not affects AR quantity and activity in LNCaP and 22Rv1 cells. (A) Expression pattern of NDRG2 and the designated proteins upon NDRG2 overexpression. Cells infected with lentivirus encoding NDRG2 and LacZ were lysed, and Western blots were performed using the designated antibodies. α-Tubulin protein levels were used as a loading control. Representative blots are shown, and the relative expression levels of the different proteins normalized to α-Tubulin were derived from 4 independent experiments (n = 4). (B) Immunofluorescence detection of AR subcellular localization pattern with anti-AR antibody in Lenti-NDRG2-infected and control cells (Scale bar: 20 μm). (C) ELISA analysis of PSA secretion in Lenti-NDRG2-infected and control cells. The histograms were drawn based on the average values (n = 3). In A and C, data indicate the mean ± SD *: P < 0.05; **: P < 0.01; ***: P < 0.001.
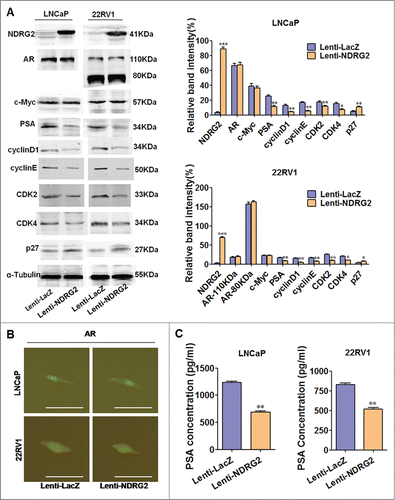
Forced expression of NDRG2 inhibits growth of CRPC cell lines
To further evaluate the role of NDRG2 in CRPC, we examined the effect of Lenti-NDRG2 on the malignant behavior as well as molecular changes in LNCaP and 22Rv1 cells. Androgen-independent growth and castration resistance in LNCaP cells were also mimicked by IL-4 (50 ng/mL) treatment of these cells because IL-4 has been reported to sensitize the AR and stimulate cell growth through the activation of pro-survival signaling pathways.Citation35
As shown in , Lenti-NDRG2 infection significantly inhibits the growth of LNCaP cells when cultured either in routine medium or in the presence of IL-4 ( left), as well as the growth of 22Rv1 cells ( right). EdU staining showed that Lenti-NDRG2 significantly promotes proliferation when compared with Lenti-LacZ (). This is further confirmed by FCM analysis, showing that Lenti-NDRG2 infection leads to more G0/G1 accumulation (Supplementary Fig. 4). Consistently, Western blot demonstrated that Lenti-NDRG2 infection can downregulate the expression of G1 phase regulators (cyclinD1, cyclinE, CDK2, CDK4) and upregulate the G1 phase inhibitor p27 in both cell lines (). In addition, NDRG2 overexpression causes cell apoptosis relative to Lenti-LacZ (). Together, these data indicated that NDRG2 overexpression retards cell growth of not only androgen-dependent prostate cancer but also CRPC by inhibiting cell proliferation and promoting cell apoptosis.
Figure 4. Lentivirus-mediated overexpression of NDRG2 inhibited the proliferation and induced apoptosis of LNCaP and 22Rv1 cells in vitro. LNCaP and 22Rv1 cells were infected with lentivirus encoding NDRG2 and LacZ and cultured in the presence or absence of IL-4 (50 ng/mL). (A) The growth curves were drawn based on the MTT assay (n = 6). (B) Cell proliferation was measured by EdU staining, with the average percentage of proliferative cells shown in the histograms (n = 3). (C) Cell apoptosis detection with FCM, with the average percentage of apoptotic cells shown on the histograms (n = 3). The EdU stain and FCM assays were performed 5 d after culture. Data indicate the mean ± SD *: P < 0.05; **: P < 0.01.
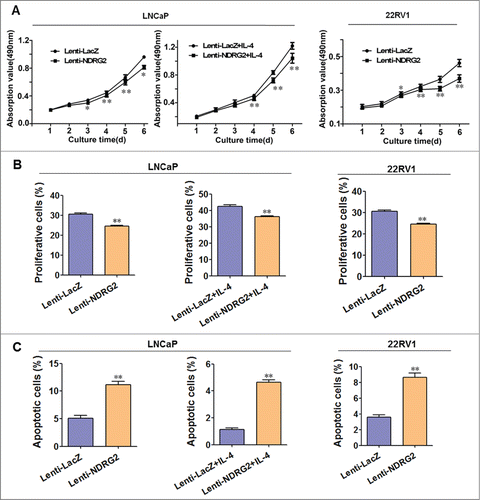
NDRG2 retards the in vivo growth of CRPC
Finally, we wanted to know whether ectopically overexpressed NDRG2 could inhibit the in vivo tumor growth of CRPC and thus potentially overcome castration resistance. To this end, we injected Lenti-LacZ- or Lenti-NDRG2-infected 22Rv1 cells into nude mice to establish a xenograft model. The mice were then given castration therapy by orchectomy, and tumor growth was monitored. Encouragingly, the mice transplanted with 22Rv1 cells developed xenograft outgrowth and tumor growth curves showed that castration alone had no effect on tumor growth (Lenti-LacZ + Castration vs. Lenti-LacZ, F ≤ 0.658, P > 0.05) (), reinforcing the castration resistance of 22Rv1 cells. However, Lenti-NDRG2 significantly suppressed the growth of 22Rv1-inoculated tumors following castration (). As a result, the weights of the tumors in the Lenti-NDRG2 + Castration group were significantly lighter than those of the Lenti-LacZ + Castration group (). The lower tumor/body mass ratio in the Lenti-NDRG2 + Castration group indicated that the physical condition of the mice was much better than that of the mice in the other 2 groups (). Taken together, forced overexpression of NDRG2 can strikingly inhibit the in vivo tumor growth of CRPC, suggesting its potential usage as a novel therapeutic agent.
Figure 5. Lentivirus-mediated overexpression of NDRG2 suppressed the in vivo growth of 22Rv1 xenografts following castration. Nude mice were injected subcutaneously with 22Rv1 cells stably expressing LacZ or NDRG2 and underwent castration therapy by orchectomy. (A) Tumor growth was monitored by assessing tumor volume (mm3) every week. (B) The tumor nodules were collected at the end of the experiment. (C) The tumor weight (g) was recorded. (D) Ratios of tumor weight/mouse weight were recorded. The curves and histograms were drawn based on the average values (n = 6). Data indicate the mean ± SD *: P < 0.05; **: P < 0.01.
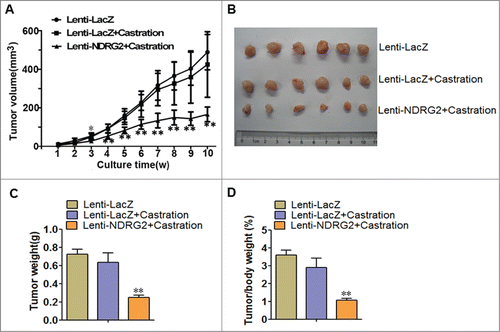
Discussion
It has been well established that AR is a key molecule for castration resistance.Citation7–11 AR can promote ligand-independent prostate cancer progression by directly regulating the transcription of c-Myc,Citation30 which confers androgen independence.Citation29 As a target gene of c-Myc,Citation17 NDRG2 has been widely accepted as a tumor suppressor in many types of cancers,Citation15,21,25-27 and our previous study reported a negative correlation betw-een the expression of NDRG2 and that of c-Myc in prostate cancer.Citation27 These findings prompted us to investigate whether NDRG2 is involved in AR signaling and plays an important role in CRPC. Initial immunohistochemical staining of PC patient samples indicated that the expression of the NDRG2 protein was negatively correlated with 2 molcules known to be intimately involved in CRPC, AR and c-Myc. Thus, we sought to determine the potential regulatory relationship between them.
We first examined whether NDRG2 is regulated by AR signaling. By utilizing an AR activator (DHT) and an AR inhibitor (bicalutamide), we confirmed that the abundance and activity of AR, as well as PSA production, was oppositely regulated by DHT and bicalutamide, which is consistent with the previous results.Citation36,37 Accordingly, we demonstrated that the expression level of c-Myc was positively regulated, while that of NDRG2 was negatively regulated, by alterations in AR. A previous studyCitation38 in rats showed that androgen deprivation by castration plus adrenalectomy significantly increased NDRG2 expression, but DHT decreased its expression in the kidney cortical collecting duct. Together, these data clearly revealed that NDRG2 is a downstream molecule of AR. As for the mechanism, our results and current knowledge favor the notion that c-Myc acts as an intermediate between AR and NDRG2, as c-Myc is a known upstream regulator of NDRG2Citation17 and a downstream target of AR. However, we cannot exclude the possibility that AR may regulate NDRG2 directly or independently of c-Myc, given that AR is a well-characterized nuclear transcription factor. Additionally, we explored the possibility that NDRG2 may affect AR/AR signaling. By modulating NDRG2 with a lentivirus expression system, we found that overexpression of NDRG2 did not influence the expression level and subcellular localization of AR, but could downregulate the expression and production of PSA, a well-known downstream target of AR. We thus assume that, as a target of AR/c-Myc, NDRG2 plays an important inhibitory role in AR(/c-Myc)/NDRG2/PSA signaling pathway, but the exact mechanism still awaits further investigation.
PSA is a widely accepted marker for the diagnosis and prognosis of prostate cancer.Citation39 Our finding that NDRG2 attenuates the AR-PSA axis strongly implies that overexpression of NDRG2 may reverse the malignant phenotype of prostate cancer. Indeed, this has been confirmed by our in vitro data, showing that ectopically overexpressed NDRG2 inhibited the growth of both androgen-dependent prostate cancer cell line LNCaP and CRPC cell line 22Rv1. Importantly, we demonstrated that NDRG2 can also inhibit the IL-4-induced, androgen-independent growth of LNCaP cells through G1 phase arrest and induction of apoptosis. IL-4 has been shown to be significantly elevated and directly correlated with elevated PSA in CRPC.Citation35 By activating AR signaling through Akt/NF-kBCitation35,40 and CBP/p300,Citation33 IL-4 can not only increase the sensitivity of AR-positive cancer cells to androgen but also promote androgen-independent growth.Citation41 Thus, by acting as an intermediate negative regulator between AR and PSA, NDRG2 is able to exert a broad inhibitory role on androgen-dependent prostate cancer and CRPC.
To pursue the potential use of NDRG2 as a novel therapeutic candidate, we established 22Rv1 xenograft nude mouse models and then evaluated the in vivo effect of NDRG2 on castration resistance. The results clearly demonstrated that lentivirus-mediated overexpression of NDRG2 could inhibit the growth of 22Rv1 xenografts following castration therapy, whereas single orchectomy did not achieve any therapeutic effect. Thus, with improvements in delivery techniques, NDRG2 may serve as a new promising therapeutic agent or at least a hypurgia for castration therapy in CRPC. As for whether NDRG2 can broadly cooperate or synergize with other AR-signaling-targeted therapies, it still awaits systematic investigation in the future.
In summary, integrating the PC patient tissue examination and in vitro and in vivo experiments, we demonstrate that NDRG2, by acting downstream of AR and inhibiting the AR signal pathway, is able to efficiently inhibit CRPC cell growth in vitro and in vivo. These studies not only offer new insight into the mechanism of castration resistance in prostate cancer but also provide a potential and powerful candidate for the treatment of CRPC in the future.
Materials and Methods
Collection of clinical prostate cancer samples
A total of 107 PC samples were collected between 2008 and 2010 from patients with prostatic tumors. The donor age range was 50–89 years, with a mean age of 65.7 ± 8.9 y Before treatment, the tissues were gathered by transurethral resection or needle biopsy in the Department of Urologic Surgery, Xijing Hospital, FMMU (Xi'an, CHN).
The collection and use of human prostatic tissues were approved by the Fourth Military Medical University medical ethics committee. All patients provided written informed consent. Each tumor was scored for pathological stage based on the Gleason system, which was introduced in 1984 by Gleason. All of the samples had been reviewed and given a final diagnosis by 3 different clinical pathologists.
Immunohistochemistry detection
Immunohistochemistry staining was performed with a mouse anti-human NDRG2 antibody (Santa Cruz Biotechnology, CA), rabbit anti-human c-Myc and rabbit anti-human AR antibodies (Cell Signaling, MA), using an immunohistochemistry kit (Boster Company, Wuhan, CHN) according to the manufacturer's instructions. The intensity were quantified using a scoring system from 0 to 12 (0 = no signal, 1–4 = weak signal, 5–8 = intermediate signal, and 9–12 = strong signal).Citation27 Each sample was examined separately and scored by 2 pathologists. The photos were collected through light microscopy and using SPOT Advanced Software (Olympus, Nagano, JPN).
Cell lines and reagents
LNCaP and 22Rv1 cells (from ATCC) were maintained in RPMI 1640 (Gibco BRL, NY, USA) containing 10% fetal bovine serum (Gibco BRL, NY, USA) at 37°C in a humidified atmosphere with 5% CO2. When reaching approximately 80% confluence, the cells were collected or split through trypsinization.
DHT (Sigma-Aldrich, MO, USA) was dissolved in ethanol (ready-to-use concentration: 10 nM). Bicalutamide (Sigma-Aldrich, MO, USA) was dissolved in DMSO (ready-to-use concentration: 10 μM). IL-4 (Sigma-Aldrich, MO, USA) was dissolved in PBS (ready-to-use concentration: 50 ng/mL). All the dissolved regents were stored at −20°C.
Lentivirus generation and infection
Recombinant lentiviral vectors were constructed with the ViraPower™ Lentiviral System (Invitrogen, NY, USA) as described previously.Citation42 Lenti-LacZ was used as a negative control, while Lenti-Cherry was used to optimize the infection efficiency. For cell infection, LNCaP and 22Rv1 cells were seeded into 25 cm2 plastic culture flasks and grown to approximately 40% confluence, following which the lentivirus-containing supernatants were added into the cell culture medium in the presence of 1 μg/ml Polybrene (Sigma-Aldrich, USA). Twenty-four hours after infection, the cells were selected with blasticidin (LNCaP, 4 μg/mL; 22Rv1, 6 μg/mL) for approximately 3 weeks, so that the uninfected cells were eliminated entirely.
Western blot analysis
Western blotting was performed as described previously.Citation27 The primary antibodies are as following: mouse anti-human NDRG2 (1:500 dilution, Santa Cruz Biotechnology, CA, USA), rabbit anti-human PSA, c-Myc and AR (1:1000 dilution, Cell Signaling Technology, MA, USA), rabbit anti-human cyclinD1, cyclinE, CDK2, CDK4, and p27 (1:1000 dilution, Santa Cruz Biotechnology, CA, USA). Detection of α–tubulin served as a loading control. The images were scanned and quantified using Kodak Digital Science ID software (Kodak, NY, USA). The experiments were repeated 4 times.
MTT assay
Cells were seeded in 96-well plates (2000 cells/well for LNCaP, 1000 cells/well for 22Rv1) in sextuplicate and cultured the medium with or without the indicated reagents for up to 6 d The viable cells were determined using the 3-(4,5-dimethylthiazol-2-yl)-2,5-diphenyltetrazolium bromide (MTT) assay and reading the absorbance at 490 nm. Cell growth curves were drawn based on the average absorption values.
EdU staining
Cells were seeded in 96-well plates in triplicate and cultured in reagent-free medium or medium containing the indicated reagents for 5 d EdU staining was performed according to the manufacturer's instruction (Ribo Company, Guangzhou, CHN). Briefly, EdU was added into the culture media and the cells were fixed and examined via fluorescence microscope. Six visual fields (400×) were chosen randomly to count and calculate the proportion of proliferative cells, whose nuclei were stained red.
Flow cytometry assays (FCM)
Cells were plated in 6-well culture dishes (1 × 105 cells/well) in triplicate and cultured as in EdU staining. Five days later, cells were harvested, fixed and stained with 50 μg/ml propidium iodide in the presence of RNAseA (10 μg/ml) and Triton X-100 (0.1%). The cell cycle distribution was measured with a flow cytometer (BD Company, NJ, USA). Data are presented as the mean ± SD. The experiments were repeated at least 3 times.
Immunofluorescence assay for AR
Cells were seeded in 48-well plates (1 × 104 cells per well) in triplicate and cultured as in EdU staining. After permeabilization (0.5% Triton X-100), fixation (4% formaldehyde) and blocking (10% normal goat serum and 0.5% Triton X-100), rabbit anti-human AR monoclonal antibody (Cell Signaling Technology, MA, USA) was added to the cells overnight at 4°C. Cells were then stained with FITC-conjugated goat-anti-rabbit polyclonal antibody at 37°C for 2 h in the dark. The fluorescence staining intensity was then examined using immunofluorescence microscopy and photographed. The experiments were repeated at least 3 times.
PSA ELISA
Cells were seeded in 6-well plates (5 × 105 cells per well) in triplicate and cultured as in EdU staining. The conditioned media in different groups was assayed for PSA levels using commercial sandwich ELISA kits (Cusabio, Wuhan, CHN) according to the manufacturer's instructions. Each sample was tested in duplicate and quantified using an Emax precision microplate reader (Molecular Devices Corp, CA, USA) at 450 nm. The levels of PSA in different groups were normalized relative to the corresponding control group. Data are presented as the mean ± SD. The experiments were repeated at least 3 times.
Tumor growth assays in vivo
Male BALB/c nude mice were purchased from Shanghai Experimental Animal Center (Shanghai, CHN). All of the protocols were approved by the Animal Care and Use Committee at the Fourth Military Medical University and were performed in accordance with the animal care rules set forth by the university (Permit number 10001). LNCaP and 22Rv1 cells that were infected with Lenti-LacZ or Lenti-NDRG2 were harvested, resuspended in sterile PBS and injected subcutaneously into the left flanks of the 6-week-old nude mice (1 × 107 cells/mouse; 6 mice/group). In the groups of Lenti-LacZ + castration and Lenti-NDRG2 + castration, the mice were given orchectomy surgery to mimic castration therapy just after cell injection. The mice were monitored every day; tumor volumes were calculated every week based on caliper measurements of the length and width of the lesions using the formula: V (mm3) = length × width2/2. After 10 weeks, the mice were sacrificed by cervical dislocation under anesthesia (130 mg ketamine/8.8 mg xylazine/kg body weight), and tumor specimens were taken, photographed, measured and weighed. Data are presented as the mean ± SD.
Statistical analysis
Statistical analyses were performed using SPSS 13.0 software. For immunohistochemistry results, disparities across groups were analyzed using the Pearson correlation coefficient. Analysis of variance (ANOVA) and Student-Newman-Keuls (SNK) tests were computed to determine whether there were differences among the different groups of in vitro and in vivo assays. A P value of less than 0.05 was considered statistically significant, and histograms were prepared using Origin 6.0 (Microcal Software, Inc., Northampton, MA).
Disclosure of Potential Conflicts of Interest
No potential conflicts of interest were disclosed.
Funding
This study was supported by grants from the Key Project of Chinese National Programs for Fundamental Research and Development (Project No. 2009CB521704) and the National Natural Science Foundation of China (Nos. 30800492, 30972723, 81230043 and C050205).
References
- Siegel R, Naishadham D, Jemal A. Cancer statistics, 2012. CA Cancer J Clin 2012; 62:10-29; PMID:22237781
- Sahu A, Iyer MK, Chinnaiyan AM. Insights into Chinese prostate cancer with RNA-seq. Cell Res 2012; 22:786-8; PMID:22453238; http://dx.doi.org/10.1038/cr.2012.50
- Wolf AM, Wender RC, Etzioni RB, Thompson IM, D'Amico AV, Volk RJ, Brooks DD, Dash C, Guessous I, Andrews K, et al. American cancer society guideline for the early detection of prostate cancer: update 2010. CA Cancer J Clin 2010; 60:70-98; PMID:20200110
- Heidenreich A, Bastian PJ, Bellmunt J, Bolla M, Joniau S, van der Kwast T, et al. EAU guidelines on prostate cancer. part II: treatment of advanced, relapsing, and castration-resistant prostate cancer. Eur Urol 2014; 65(2):467-79; PMID:24321502; http://dx.doi.org/10.1016/j.eururo.2013.11.002
- Smith MR, Cook R, Lee KA, Nelson JB. Disease and host characteristics as predictors of time to first bone metastasis and death in men with progressive castration-resistant nonmetastatic prostate cancer. Cancer 2011; 117:2077-85; PMID:21523719; http://dx.doi.org/10.1002/cncr.25762
- Shang Y, Myers M, Brown M. Formation of the androgen receptor transcription complex. Mol Cell 2002; 9:601-10; PMID:11931767; http://dx.doi.org/10.1016/S1097-2765(02)00471-9
- Mahmoud AM, Zhu T, Parray A, Siddique HR, Yang W, Saleem M, Bosland MC. Differential effects of genistein on prostate cancer cells depend on mutational status of the androgen receptor. PloS One 2013; 8:e78479; PMID:24167630; http://dx.doi.org/10.1371/journal.pone.0078479
- Mazaris E, Tsiotras A. Molecular pathways in prostate cancer. Nephrourol Mon 2013; 5:792-800; PMID:24282788; http://dx.doi.org/10.5812/numonthly.9430
- Imberg-Kazdan K, Ha S, Greenfield A, Poultney CS, Bonneau R, Logan SK, Garabedian MJ. A genome-wide RNA interference screen identifies new regulators of androgen receptor function in prostate cancer cells. Genome Res 2013; 23:581-91; PMID:23403032; http://dx.doi.org/10.1101/gr.144774.112
- Baek KH, Hong ME, Jung YY, Lee CH, Lee TJ, Park ES, Kim MK, Yoo JH, Lee SW. Correlation of AR, EGFR, and HER2 expression levels in prostate cancer: immunohistochemical analysis and chromogenic in situ hybridization. Cancer Res Treat 2012; 44:50-6; PMID:22500161; http://dx.doi.org/10.4143/crt.2012.44.1.50
- Whitworth H, Bhadel S, Ivey M, Conaway M, Spencer A, Hernan R, Holemon H, Gioeli D. Identification of kinases regulating prostate cancer cell growth using an RNAi phenotypic screen. PloS One 2012; 7:e38950; PMID:22761715; http://dx.doi.org/10.1371/journal.pone.0038950
- Harris WP, Mostaghel EA, Nelson PS, Montgomery B. Androgen deprivation therapy: progress in understanding mechanisms of resistance and optimizing androgen depletion. Nat Clin Practice Urol 2009; 6:76-85; PMID:19198621; http://dx.doi.org/10.1038/ncpuro1296
- Andersen RJ, Mawji NR, Wang J, Wang G, Haile S, Myung JK, Watt K, Tam T, Yang YC, Bañuelos CA, et al. Regression of castrate-recurrent prostate cancer by a small-molecule inhibitor of the amino-terminus domain of the androgen receptor. Cancer Cell 2010; 17:535-46; PMID:20541699; http://dx.doi.org/10.1016/j.ccr.2010.04.027
- Scher HI, Beer TM, Higano CS, Anand A, Taplin ME, Efstathiou E, Rathkopf D, Shelkey J, Yu EY, Alumkal J, et al. Antitumour activity of MDV3100 in castration-resistant prostate cancer: a phase 1-2 study. Lancet 2010; 375:1437-46; PMID:20398925; http://dx.doi.org/10.1016/S0140-6736(10)60172-9
- Deng Y, Yao L, Chau L, Ng SS, Peng Y, Liu X, Au WS, Wang J, Li F, Ji S, et al. N-Myc downstream-regulated gene 2 (NDRG2) inhibits glioblastoma cell proliferation. Int J Cancer 2003; 106:342-7; PMID:12845671; http://dx.doi.org/10.1002/ijc.11228
- Zhou RH, Kokame K, Tsukamoto Y, Yutani C, Kato H, Miyata T. Characterization of the human NDRG gene family: a newly identified member, NDRG4, is specifically expressed in brain and heart. Genomics 2001; 73:86-97; PMID:11352569; http://dx.doi.org/10.1006/geno.2000.6496
- Zhang J, Li F, Liu X, Shen L, Liu J, Su J, Zhang W, Deng Y, Wang L, Liu N, et al. The repression of human differentiation-related gene NDRG2 expression by Myc via Miz-1-dependent interaction with the NDRG2 core promoter. J Biol Chem 2006; 281:39159-68; PMID:17050536; http://dx.doi.org/10.1074/jbc.M605820200
- Foletta VC, Prior MJ, Stupka N, Carey K, Segal DH, Jones S, Swinton C, Martin S, Cameron-Smith D, Walder KR. NDRG2, a novel regulator of myoblast proliferation, is regulated by anabolic and catabolic factors. J Physiol 2009; 587:1619-34; PMID:19204049; http://dx.doi.org/10.1113/jphysiol.2008.167882
- Li T, Hu J, He GH, Li Y, Zhu CC, Hou WG, Zhang S, Li W, Zhang JS, Wang Z, et al. Up-regulation of NDRG2 through nuclear factor-kappa B is required for Leydig cell apoptosis in both human and murine infertile testes. Biochim Biophys Acta 2012; 1822:301-13; PMID:22138128; http://dx.doi.org/10.1016/j.bbadis.2011.11.013
- Liu N, Wang L, Li X, Yang Q, Liu X, Zhang J, Wu Y, Ji S, Zhang Y, Yang A, et al. N-Myc downstream-regulated gene 2 is involved in p53-mediated apoptosis. Nucleic Acids Res 2008; 36:5335-49; PMID:18689861; http://dx.doi.org/10.1093/nar/gkn504
- Yao L, Zhang J, Liu X. NDRG2: a Myc-repressed gene involved in cancer and cell stress. Acta Biochim Biophys Sin (Shanghai) 2008; 40:625-35; PMID:18604454; http://dx.doi.org/10.1111/j.1745-7270.2008.00434.x
- Liu N, Wang L, Liu X, Yang Q, Zhang J, Zhang W, Wu Y, Shen L, Zhang Y, Yang A, et al. Promoter methylation, mutation, and genomic deletion are involved in the decreased NDRG2 expression levels in several cancer cell lines. Biochem Biophys Res Commun 2007; 358:164-9; PMID:17470364; http://dx.doi.org/10.1016/j.bbrc.2007.04.089
- Lee DC, Kang YK, Kim WH, Jang YJ, Kim DJ, Park IY, Sohn BH, Sohn HA, Lee HG, Lim JS, et al. Functional and clinical evidence for NDRG2 as a candidate suppressor of liver cancer metastasis. Cancer Res 2008; 68:4210-20; PMID:18519680; http://dx.doi.org/10.1158/0008-5472.CAN-07-5040
- Feng L, Xie Y, Zhang H, Wu Y. Down-regulation of NDRG2 gene expression in human colorectal cancer involves promoter methylation and microRNA-650. Biochem Biophys Res Commun 2011; 406:534-8; PMID:21352815; http://dx.doi.org/10.1016/j.bbrc.2011.02.081
- Lorentzen A, Lewinsky RH, Bornholdt J, Vogel LK, Mitchelmore C. Expression profile of the n-myc downstream regulated gene 2 (NDRG2) in human cancers with focus on breast cancer. BMC cancer 2011; 11:14; PMID:21226903; http://dx.doi.org/10.1186/1471-2407-11-14
- Zheng J, Li Y, Yang J, Liu Q, Shi M, Zhang R, Shi H, Ren Q, Ma J, Guo H, et al. NDRG2 inhibits hepatocellular carcinoma adhesion, migration and invasion by regulating CD24 expression. BMC cancer 2011; 11:251:1-9; PMID:21676268
- Yu C, Wu G, Dang N, Zhang W, Zhang R, Yan W, Zhao Y, Gao L, Wang Y, Beckwith N, et al. Inhibition of N-myc downstream-regulated gene 2 in prostatic carcinoma. Cancer Biol Ther 2011; 12:304-13; PMID:21623166; http://dx.doi.org/10.4161/cbt.12.4.16382
- Gao L, Wu GJ, Liu XW, Zhang R, Yu L, Zhang G, Liu F, Yu CG, Yuan JL, Wang H, et al. Suppression of invasion and metastasis of prostate cancer cells by overexpression of NDRG2 gene. Cancer Lett 2011; 310:94-100; PMID:21741166; http://dx.doi.org/10.1016/j.canlet.2011.06.015
- Bernard D, Pourtier-Manzanedo A, Gil J, Beach DH. Myc confers androgen-independent prostate cancer cell growth. J Clin Invest 2003; 112:1724-31; PMID:14660748; http://dx.doi.org/10.1172/JCI200319035
- Gao L, Schwartzman J, Gibbs A, Lisac R, Kleinschmidt R, Wilmot B, Bottomly D, Coleman I, Nelson P, McWeeney S, et al. Androgen receptor promotes ligand-independent prostate cancer progression through c-Myc upregulation. PloS One 2013; 8:e63563; PMID:23704919; http://dx.doi.org/10.1371/journal.pone.0063563
- Dozmorov MG, Hurst RE, Culkin DJ, Kropp BP, Frank MB, Osban J, Penning TM, Lin HK. Unique patterns of molecular profiling between human prostate cancer LNCaP and PC-3 cells. Prostate 2009; 69:1077-90; PMID:19343732; http://dx.doi.org/10.1002/pros.20960
- Sramkoski RM, Pretlow TG, 2nd, Giaconia JM, Pretlow TP, Schwartz S, Sy MS, Marengo SR, Rhim JS, Zhang D, Jacobberger JW. A new human prostate carcinoma cell line, 22Rv1. In Vitro Cell Dev Biol Anim 1999; 35:403-9; PMID:10462204; http://dx.doi.org/10.1007/s11626-999-0115-4
- Lee SO, Chun JY, Nadiminty N, Lou W, Feng S, Gao AC. Interleukin-4 activates androgen receptor through CBP/p300. Prostate 2009; 69:126-32; PMID:18819102; http://dx.doi.org/10.1002/pros.20865
- Fahrenholtz CD, Rick FG, Garcia MI, Zarandi M, Cai RZ, Block NL, Schally AV, Burnstein KL. Preclinical efficacy of growth hormone-releasing hormone antagonists for androgen-dependent and castration-resistant human prostate cancer. Proc Natl Acad Sci U S A 2014; 111:1084-9; PMID:24395797; http://dx.doi.org/10.1073/pnas.1323102111
- Lee SO, Lou W, Hou M, Onate SA, Gao AC. Interleukin-4 enhances prostate-specific antigen expression by activation of the androgen receptor and Akt pathway. Oncogene 2003; 22:7981-8; PMID:12970746; http://dx.doi.org/10.1038/sj.onc.1206735
- Lu S, Tan Z, Wortman M, Lu S, Dong Z. Regulation of heat shock protein 70-1 expression by androgen receptor and its signaling in human prostate cancer cells. Int J Oncol 2010; 36:459-67; PMID:20043082; http://dx.doi.org/10.3892/ijo_00000538
- Lu S, Wang A, Lu S, Dong Z. A novel synthetic compound that interrupts androgen receptor signaling in human prostate cancer cells. Mol Cancer Ther 2007; 6:2057-64; PMID:17620434; http://dx.doi.org/10.1158/1535-7163.MCT-06-0735
- Boulkroun S, Le Moellic C, Blot-Chabaud M, Farman N, Courtois-Coutry N. Expression of androgen receptor and androgen regulation of NDRG2 in the rat renal collecting duct. Pflugers Arch 2005; 451:388-94; PMID:16142456; http://dx.doi.org/10.1007/s00424-005-1410-x
- Dong Y, Lee SO, Zhang H, Marshall J, Gao AC, Ip C. Prostate specific antigen expression is down-regulated by selenium through disruption of androgen receptor signaling. Cancer Res 2004; 64:19-22; PMID:14729601; http://dx.doi.org/10.1158/0008-5472.CAN-03-2789
- Lee SO, Lou W, Nadiminty N, Lin X, Gao AC. Requirement for NF-(kappa)B in interleukin-4-induced androgen receptor activation in prostate cancer cells. Prostate 2005; 64:160-7; PMID:15678501; http://dx.doi.org/10.1002/pros.20218
- Lee SO, Pinder E, Chun JY, Lou W, Sun M, Gao AC. Interleukin-4 stimulates androgen-independent growth in LNCaP human prostate cancer cells. Prostate 2008; 68:85-91; PMID:18008330; http://dx.doi.org/10.1002/pros.20691
- Guo Y, Ma J, Wu L, Wang Q, Li X, Li X, Zhang Y, Zhang J, Yao L, Zhang J, et al. Hyperthermia-induced NDRG2 upregulation inhibits the invasion of human hepatocellular carcinoma via suppressing ERK1/2 signaling pathway. PloS One 2013; 8:e61079; PMID:23630579; http://dx.doi.org/10.1371/journal.pone.0061079