Abstract
Natural compound schweinfurthins are of considerable interest for novel therapy development because of their selective anti-proliferative activity against human cancer cells. We previously reported the isolation of highly active schweinfurthins E-H, and in the present study, mechanisms of the potent and selective anti-proliferation were investigated. We found that schweinfurthins preferentially inhibited the proliferation of PTEN deficient cancer cells by indirect inhibition of AKT phosphorylation. Mechanistically, schweinfurthins and their analogs arrested trans-Golgi-network trafficking, an intracellular vesicular trafficking system, resulting in the induction of endoplasmic reticulum stress and the suppression of both lipid raft-mediated PI3K activation and mTOR/RheB complex formation, which collectively led to an effective inhibition of mTOR/AKT signaling. The trans-Golgi-network traffic arresting effect of schweinfurthins was associated with their in vitro binding activity to oxysterol-binding proteins that are known to regulate intracellular vesicular trafficking. Moreover, schweinfurthins were found to be highly toxic toward PTEN-deficient B cell lymphoma cells, and displayed 2 orders of magnitude lower activity toward normal human peripheral blood mononuclear cells and primary fibroblasts in vitro. These results revealed a previously unrecognized role of schweinfurthins in regulating trans-Golgi-network trafficking, and linked mechanistically this cellular effect with mTOR/AKT signaling and with cancer cell survival and growth. Our findings suggest the schweinfurthin class of compounds as a novel approach to modulate oncogenic mTOR/AKT signaling for cancer treatment.
Abbreviations
SA-J | = | schweinfurthin A-J |
TGN | = | trans-Golgi-network |
PTEN | = | phosphatase and tensin homolog |
DLBCL | = | diffuse large B cell lymphoma |
mTOR | = | mammalian target of rapamycin |
PDK1 | = | phosphoinositide-dependent kinase 1 |
PIP3 | = | phosphatidylinositol (3,4,5)-triphosphate |
WGA | = | wheat germ agglutinin |
ConA | = | concanavalin |
MAA | = | Maackia amurensis agglutinin |
PNA | = | peanut agglutinin |
ORPs | = | oxysterol-binding protein related family proteins |
Introduction
Natural compounds with unique biological activities are valuable tools for delineating key molecules and pathways involved in biological processes.Citation1 Schweinfurthins were originally isolated from the leaves of the African plant Macaranga schweinfurthii and from the Malagasy plant Macaranga alnifolia, and were reported to have selective anti-proliferative activity in certain glioblastoma, renal, melanoma and leukemia cells.Citation2,3 Because of the unique selectivity profile, there has been considerable interest and effort in developing schweinfurthin analogs for novel anti-cancer therapy.Citation4–6 However, this effort has been hampered by a lack of understanding of mechanism. Recently, schweinfurthin A (SA) was shown to interfere with phosphorylation of Rho in glioma cellsCitation5 and to induce expression of glucose-related protein 78 and phosphorylation of eukaryotic initiation factor 2a,Citation7 suggesting regulatory roles in signaling and endoplasmic reticulum (ER) induction by schweinfurthins. Interestingly, OSBP and ORP4L, 2 members of the oxysterol-binding protein related family proteins (ORPs) were reported as potential targets for SA and for a few other natural compounds that showed a similar anti-proliferative pattern in the NCI-60 cancer cell panel.Citation8 ORP family proteins have over 15 members and are conserved from C. elegans to humans, but have poorly defined functions in cancer cell survival and growth.Citation9 These studies have provided valuable insights about mechanism of the compounds, but have not yet explained the selective anti-proliferative activity of schweinfurthins. Thus, the mechanism remains to be investigated further in order to appropriately evaluate schweinfurthins for development as novel therapies.
Growth of cancer cells is driven by enhanced oncogenic signaling through either genetic or epigenetic dysregulation.Citation10 PI3K-AKT and MAPK-ERK are the 2 dominant oncogenic signaling pathways that are upregulated in a large proportion of human cancers and thus are key targets for anti-cancer therapies.Citation11,12 Appropriate activation of both pathways requires targeted delivery of both signaling molecules and their accessory molecules to the right cellular organelles, a process that is complex and poorly understood. Increasing evidence indicates the involvement of vesicles and soluble carriers in certain oncogenic signaling.Citation13,14 In this regard, certain RTK signaling molecules are known to assemble preferentially on the plasma membrane in lipid rafts, microdomains enriched with complex lipids including ceramide, sphingomyelin, glycolipid GM1 and cholesterol, to activate the PI3K-AKT pathway, but not the MAPK-ERK pathway.Citation15,16 Therefore, understanding the intracellular trafficking process in oncogenic signaling may offer novel opportunities beyond kinase inhibitors to modulate the proliferation and survival of oncogene-addicted cancer cells.
In this study, the mechanism of selective anti-proliferative activity of schweinfurthins was systematically characterized through combined approaches of chemical biology and bioinformatics. We found potent inhibitory activity of schweinfurthins on AKT phosphorylation but not ERK phosphorylation, effected through interfering with trans-Golgi-network (TGN) trafficking, an intracellular vesicular system circulating among the trans-Golgi apparatus, plasma membrane, and endosome/lysosomes.Citation17 Arrest of TGN trafficking by schweinfurthins resulted in an effective inhibition of mTOR/AKT signaling through inducing endoplasmic reticulum stress and suppressing both lipid raft-mediated PI3K activation and mTOR/RheB complex formation. Guided by this mechanism, we observed that schweinfurthins efficiently killed PTEN deficient B lymphoma cells in vitro, with low nM IC50 values, while IC50 values toward normal human peripheral blood mononuclear cells (PBMC) and human fibroblasts were 2 orders of magnitude higher. Biochemical analysis demonstrated that the binding capability of schweinfurthin derivatives to ORPs was required for both TGN trafficking arrest activity and anti-proliferative activity. Thus, our results reveal a previously unrecognized role of schweinfurthins in TGN trafficking and mechanistically link this cellular effect with mTOR-AKT signaling inhibition and with cancer cell survival and growth.
Results
Schweinfurthins induce an indirect and non-enzymatic inhibition of AKT phosphorylation for differential anti-proliferative activity
Among the 10 reported schweinfurthins A-J (SA to SJ), active schweinfurthins are highly similar in structure, containing a stilbene in the middle portion of the molecule (). We first confirmed the selective anti-proliferative activity of schweinfurthins by profiling SE in a custom panel of 60 human cell lines with known genetic defects, in a 4 day cell proliferation assay (). Using an IC50 value of 100 nM as a cut-off, approximately 40% of the tested cancer cell lines were sensitive to SE treatment. Importantly, serum-starved, quiescent IMR-90 fibroblasts were among the most resistant cells (, the eighth from right). Isolated preparations of SE, SF and SG showed almost identical selectivity and potency in a smaller cancer cell panel (Fig. S1A), and SG was thus synthesized for detailed investigation in this study because of its simpler structure.
Figure 1. Differential anti-proliferative activity and selective inhibition of AKT phosphorylation by schweinfurthins. (A) Structures of schweinfurthin A (SA), E (SE), F (SF) and G (SG). (B) IC50 values of 60 human cancer cell lines upon treatment with SE in a 4 day cell proliferation assay. (C) Correlation of IC50 values from the cell proliferation assay for SE with the cellular PTEN protein expression levels. (D) Selective inhibitory effects of SG in phosphorylation of AKT at Ser 473 and ERK in human melanoma cell lines. wt, wild type; mut, mutant. (E) Cell cycle effect of SG (100 nM) in melanoma A2058 cells in comparison with PI3K inhibitor (BEZ-235) and microtubule agent (paclitaxel) after a 24 h treatment. (F) Time-and dose-dependent reduction of p-AKT at Ser 473 by SG. (G) Time-dependent cell proliferation inhibition of SG in 3 PTEN deficient cell lines: WSU-DLCL2 (B lymphoma), DMS-273 (small cell lung cancer), and SF-539 (glioblastoma).
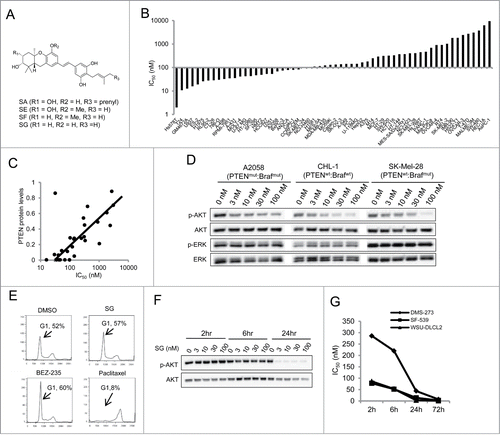
To investigate the mechanism of the selective activity profile, we examined the relationship between cell line genomics and cell growth IC50 values. Bioinformatic analysis showed that among 15 cancer genes that were found to be mutated in at least 3 cell lines in our panel, phosphatase and tensin homolog (PTEN) was the only gene whose mutation inversely correlated with IC50 values using both t-test and Wilcoxon test (Fig. S1B). A similar analysis was then run for SA in the NCI-60 panel profiling data, and among the 17 genes identified, the PTEN mutation was again found to correlate positively with sensitivity (Fig. S1C). Mutation of phosphatidylinositol 3-kinase regulatory subunit α (PI3KR1) was also found to be positively correlated with the sensitivity in the same data set. Both PTEN and PIK3R1 are known negative regulators for PI3K signaling and are frequently mutated in many human cancers and cancer cell lines with a corresponding characteristic high level of AKT phosphosylation (p-AKT).Citation11,18 Furthermore, PTEN protein expression levels in tested cancer cell lines inversely correlated with sensitivity (), and PTEN mutation status was associated with both low protein expression and a high extent of p-AKT (Fig. S1D). Notably, mutation of neurofibromin 2 (NF2), a negative regulator of mammalian target of rapamycin (mTOR) that is a major process downstream of PI3K-AKT signaling,Citation19 correlated positively with resistance to SA (Fig. S1C), further supporting an important role of PI3K-AKT signaling status for anti-proliferative schweinfurthins. The other gene whose mutation was found to be associated with cell line sensitivity to SA was cyclin-dependent kinase inhibitor 2A, but this association barely met the statistical power in the data set for SE, and thus was not further studied here.
The correlative bioinformatic results suggested a specific interference in PI3K-AKT signaling by schweinfurthins. Indeed, SG potently inhibited AKT phosphorylation at Ser 473 in a dose-dependent manner, with IC50 at nM concentrations in both PTEN wild type and mutant adherent cancer cells after a 24 h treatment (). This inhibitory activity appeared to be specific because ERK phosphorylation did not decrease by the same treatment. Induction of caspase-3/7 in sensitive A2058 melanoma cells was only detected after a 48 h treatment but not after a 24 h treatment with SG (Fig. S2A), indicating that inhibition of AKT phosphorylation preceded the induction of cell apoptosis. Moreover, treatment with SG resulted in G1 cell cycle arrest in the A2058 cells, which was similar to that of BEZ-235, a PI3K inhibitor, but differed from that of paclitaxel, a microtubule inhibitor (). These data showed for the first time that schweinfurthins possess potent inhibitory activity in AKT activation, linked to PTEN status, for cell proliferation and survival.
To explore the mechanism of p-AKT reduction, SG at 1 μM was assayed for enzymatic inhibitory activity against 293 kinases and 20 phosphatases in a cell-free Millipore® kinase/phosphatase system. SG showed neither inhibitory nor stimulatory activity against any of the kinases and phosphatases tested, including 15 kinases known to be mainly involved in PI3K-AKT-mTOR phosphorylation (Figs. S2B,C). Treatment with SG for 24 h in 2 lung cancer cell lines, A549 and HCC827, did not impact the phosphorylation status of EGFR, MET, IGF1R or other major RTKs (Fig. S2D). Importantly, wash-out experiments revealed that significant inhibition of AKT phosphorylation in cells required continuous treatment for 6–24 h regardless of SG concentration (), which differs dramatically from the effect of PI3K kinase inhibitors.Citation20 In line with the delayed inhibition of AKT phosphorylation, continuous exposure to SG for 24 h or more was required to elicit strong anti-proliferative activity in sensitive cancer cell lines (). Taken together, these results indicated that schweinfurthins inhibited AKT phosphorylation in an indirect and non-enzymatic manner, resulting in selective growth inhibition of AKT signaling-dependent cancer cells.
Schweinfurthins inhibit terminal sialylation of glycoconjugates prior to AKT phosphorylation inhibition
While examining the effect of SG in cellular RTK activation, we noted that there was a molecular weight decrease of a few kDa for both RTKs (EGFR, ErbB2 and IGFR1) and their phosphorylated forms (p-EGFR, p-ErbB2 and p-IGFR1) upon treatment (). We hypothesized that the size decrease was due to an impairment of glycosylation of the RTKs. To test the hypothesis, EGFR and MET were immunoprecipitated from cells treated with or without SG for 24 h, and blotted with 2 lectins, wheat germ agglutinin (WGA) and concanavalin (ConA), which preferentially recognize sialylated glycans and core mannose N-glycans, respectively ().Citation21 As shown in , treatment with SG resulted in reduced WGA staining and simultaneously increased ConA staining of tested RTKs, suggesting loss of terminal sialic acid residues in the glycans that were attached to core protein of the RTKs. Flow cytometric characterization further showed a diminished binding of Maackia amurensis agglutinin (MAA) lectin, which specifically recognizes (2-3)-linked terminally sialylated glycans,Citation22 to the cell surface of 2 different cell lines after SG treatments for 6 or 24 h ( and Fig. S2E). Consistently, cell surface binding of peanut agglutinin (PNA) lectin, which recognizes asialylated glycans, increased upon SG treatment (Fig. S2E). Notably, the cell surface desialylation was obvious after a 6 h treatment and thereby preceded the p-AKT decrease and the inhibition of cell proliferation (). Terminal sialylation is known to occur in late and/or post Golgi structures where synthesized molecules are packaged and transported to other cellular organelles.Citation23 The results collectively revealed an unexpected function of schweinfurthins in cell surface sialylation within hours of exposure, and suggested a role of schweinfurthins in post-Golgi intracellular trafficking.
Figure 2. Schweinfurthins inhibited sialylation of glycosylated membrane receptor proteins. (A) Western blots of major receptor tyrosine kinases (EGFR, ErbB2, MET and IGF1R) and their phosphorylated forms (p-EGFR, p-ErbB2, p-MET, and p-IGFR1) from cells treated with or without schweinfurthin G (SG) at 100 nM for 24h. Arrows denote the different sizes of detected proteins on SDS-PAGE. (B) Illustration of the glycan-binding specificity of lectins. MAA, Maackia amurensis agglutinin; ConA, concanavalin; WGA, wheat germ agglutinin; PNA, peanut agglutinin. (C) Lectin blots of immunoprecipitates of EGFR and MET from 2 cell lines using WGA and ConA. Immunoblots of EGFR and MET are included as loading controls. (D) Flow cytometric analysis of cell surface glycan terminals using fluorescein-conjugated ConA and MAA, respectively, for cells following 24 h treatment with SG at 100 nM.
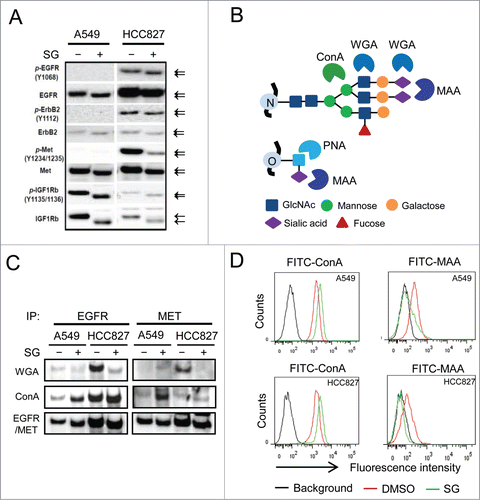
Schweinfurthins interfere with TGN trafficking for anti-proliferative activity
To further explore the mechanism for schweinfurthin alteration of sialylation of cell surface proteins, a biologically active fluorescent probe SG-Fluor and an inactive tetra-methylated derivative (SG-Methyl) of SG were generated (Fig. S3A).Citation24 After 2 h exposure, SG-Fluor was found mainly in perinuclear punctuated structures, which largely overlap with LAMP1, a cellular marker for endosomes and/or lysosomes (Fig. S3B). No significant co-location was observed for SG-Fluor with calreticulin, Mito-2 or Golgin-97, which are specific markers for ER, mitochondria and Golgi apparatus, respectively. The co-localization results suggested that schweinfurthins interfered with a pathway or process associated with endosomes/lysosomes. Endosomes/lysosomes are dynamic cellular organelles involved in cellular protein and membrane sorting through endocytosis, TGN and other mechanisms.Citation25 We first tested the effect of SG in endocytosis, and found that treatment with SG for 24 h had little effect on the endocytosis of fluorescent transferrin (Fig. S3C). In contrast, SG treatment resulted in a dose-dependent disruption of cellular TGN46 staining, a well-recognized marker for TGN, a post-Golgi vesicular trafficking system connecting trans-Golgi, plasma membrane and endosomes (), and thus indicative of a TGN trafficking arrest before cell apoptosis.Citation17,25 The arresting effect in TGN trafficking was also observed for anti-proliferative SE and SF, but not for the inactive schweinfurthin derivative SG-Methyl, even at a 10-fold higher concentration (Fig. S3D), linking TGN trafficking blockade with anti-proliferative activity. Furthermore, consistent with the above described desialylation of glycoconjugates, SG specifically interfered with TGN trafficking without significant impact to the cis or trans-Golgi apparatus, evidenced by unchanged staining patterns of GM-130 and Golgin-97 upon SG treatment ().
Figure 3. Schweinfurthins interfered with intracellular trans-Golgi-network. (A) Dose-dependent blockade activity of schweinfurthin G (SG) in TGN trafficking in HCC827 lung cancer cells. Representative immunofluorescence images of TGN46 expression are shown after a 24 h compound exposure. Hoechst stain was used to identify the nuclei. (B) Illustration of TGN trafficking system and its associated Golgi matrix proteins. (C) Co-immunostaining of TGN46 and trans-Golgi marker, Golgi-97, and cis-Golgi marker, GM-130, with or without SG treatment, in HCC827 cells. (D) Summary of required concentrations of SG to arrest TGN trafficking and IC50 values of SG in the proliferation of indicated cancer cells.
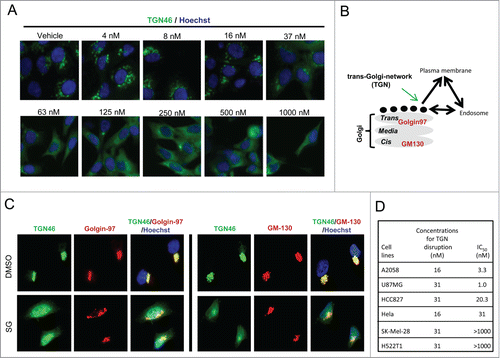
A time course experiment found that arrest of TGN trafficking required less than 3 h of SG exposure (Fig. S4A), which paralleled the timing of desialylation but preceded AKT phosphorylation inhibition and cell apoptosis induction ( and Fig. S2A). Dose response assays at 6 h and 24 h revealed a similar potency, showing significant TGN46 staining disruption at 32–64 nM at both time points without noticeable cell loss (Fig. S4B and ). The trafficking inhibitory effect by schweinfurthins was also reversible, as shown by reclustering of the TGN46 positive staining after withdrawal of compound from the culture (Fig. S4A). Using an In Cell Analyzer, an automated high resolution imaging instrument, we determined the concentrations that induced significant disruption of TGN46 staining in both sensitive and resistant cell lines after a 24 h treatment. In sensitive cell lines, the concentrations of SG required to induce TGN arrest were 8–32 nM, which corresponded well with the IC50 values for growth inhibition (). Importantly, TGN trafficking in resistant cells was equally affected by SG treatment (), which was consistent with the observation that SG decreased p-AKT in both sensitive and resistant cells upon treatment (). Together, these data disclosed for the first time a fast and reversible ‘arresting’ effect of schweinfurthins on TGN trafficking and an association between TGN trafficking arrest and inhibition of cell proliferation in schweinfurthin-responsive cells.
TGN trafficking arrest by schweinfurthins leads to lipid raft disorganization and inhibition of AKT phosphorylation
The above described sequential effects in TGN trafficking arrest and p-AKT reduction by schweinfurthins suggested a functional connection between TGN trafficking and AKT activation. To gain mechanistic insight, we characterized key molecules in the PI3K-mTOR-AKT pathway using the adherent glioblastoma cell line U87MG and the suspension lymphoma cell line WSU-DLCL2, both of which were deficient in PTEN and sensitive to schweinfurthin treatment. Treatment with SG for 16 h or longer inhibited phosphorylation at both sites Ser-473 and Tyr-308 of AKT without a significant effect on total AKT expression (), suggesting that both phosphoinositide-dependent kinase-1 (PDK1) and mTOR signaling were impacted. Indeed, phosphorylation of PDK1 and phosphorylation of p70S6K, which are upstream of PI3K activation and immediately downstream of mTORC1, respectively, were inhibited by SG in a time-dependent manner in both tested cell lines (). Because SG did not affect the kinase activity of PDK1 or any known PI3Kinases in cell-free biochemical assays (Fig. S2B), we reasoned that PDK1 phosphorylation inhibition came from lipid raft disorganization due to TGN trafficking arrest by schweinfurthins.Citation26 To test this hypothesis, expression of major lipid raft components including ceramide, GM1 and sphingomyelin and other common membrane glycoproteins were examined after a 24 h treatment of U87MG cells with SG. Cell surface expression of GM1 and sphingomyelin were significantly downregulated by SG treatment, while expression of EGFR or CD98hc was not affected by the same treatment (). Immunofluorescence images showed that ceramide was largely retained inside the cells rather than forming clusters at the cell surface after treatment with SG (), suggesting a transportation blockade for certain complex lipids by schweinfurthins. This is supported by a more quantitative flow cytometric analysis of permeabilized and non-permeabilized cells with or without SG treatment, in which a near complete loss of cell surface ceramide but only a minimal reduction in total ceramide was observed following SG treatment ().
Figure 4. Schweinfurthins inhibited mTOR-AKT activation through modulation of lipid raft integrity, mTOR intracellular location and ER stress. Schweinfurthin G (SG) modulated the substrates of both mTOR and PDK1 in adherent U87MG glioblastoma (A) and suspension WSU-DLCL2 B cell lymphoma (B) cell lines in a time-dependent manner. (C) Altered cell surface expression of major lipid raft components including ceramide, GM1 and sphingomyelin detected by fluorescence microscopy in U87MG cells. (D) Decreased expression and transport of GM1 and ceramide but not EGFR or CD98hc to plasma membrane by flow cytometry in U87MG cells. (E) Physical dissociation of mTOR with RheB by fluorescent microscopy in U87MG cells. (F) Reduced molecular association of mTOR with RheB in U87MG cells by immunoprecipitation of mTOR followed by protein gel blotting. (G) Dose-dependent upregulation of p-eIF2a by SG treatment in U87MG and WSU-DLCL2 cells. H) A scheme of the proposed mechanism by which SG inhibits mTOR-AKT signaling and thereby cell survival and proliferation in AKT-mTOR-dependent cancer cells through blocking the trans-Golgi-network trafficking. SG treatment condition: 100 nM for 24 h.
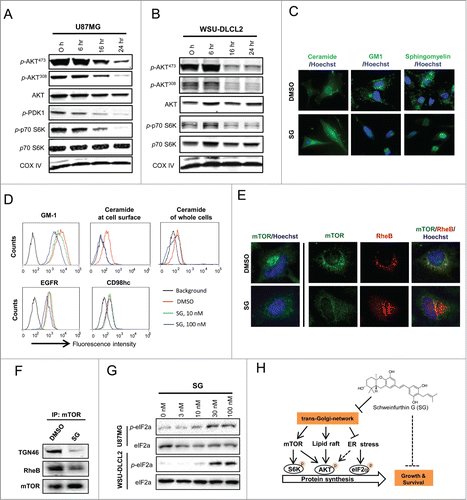
Biochemical characterization further revealed a time-dependent reduction of ceramide derivatives including sphingosine and sphingomyelin, but not the ceramide precursor, sphinganine, in cells that had received SG treatment for 6 or 24 h (Fig. S5A). Cholesterol and its derivative 7-dehydrocholesterol were also reduced, likely by a non-enzymatic mechanism.Citation27 Extraction of cholesterol from cultured cells by methyl-cyclo-dextran resulted in a specific inhibition of AKT phosphorylation, but not of ERK phosphorylation (Fig. S5B), an effect that was comparable to the signaling inhibitory activity of schweinfurthins (). Consistent with the lipid raft disrupting effect, SG treatment decreased the cellular quantity of phosphatidylinositol (3,4,5)-triphosphate (PIP3) (Fig. S5C) that is preferentially formed in lipid raft domains of plasma membraneCitation15,16. On the other hand, inhibition of PI3K by pharmacological agents such as wortmannin, BEZ235 and temsirolin had no effect on TGN trafficking (Fig. S5D). These results demonstrated a disruptive role of schweinfurthins in lipid raft organization, which led to inhibition of PIP3 formation and AKT activation, by inhibiting the targeted delivery and/or synthesis of several major lipid components, through blocking of TGN trafficking.
TGN trafficking arrest by schweinfurthins dislocates mTOR to inhibit formation of functional mTORC1 complex and induce ER stress
P70S6K is a direct effector for the mTORC1 complex, and its phosphorylation plays a key role in regulating protein synthesis.Citation28 Inhibition of p70S6K phosphorylation by SG () suggested a regulatory role of schweinfurthins in mTOR function. We then explored the mechanism by which disruption in TGN trafficking might affect mTOR function. In normal growth conditions, mTOR was significantly co-localized with the endosomal/lysosomal marker LAMP1 (Fig. S6), which was consistent with a recent observation that functional mTORC1 forms a complex with RheB in the late endosomes/lysosomes.Citation29 Treatment with SG was found to dissociate mTOR from RheB by dislocation of mTOR from endosome/lysosome organelles in U87MG cells (). In support of this physical dissociation, association between mTOR with TGN46 and RheB decreased in mTOR immunoprecipitates from cells that had received SG treatment compared with those treated with vehicle (). These results indicated that TGN trafficking blockade by schweinfurthins inhibited the formation of functional mTOR-RheB complexes by dissociation of mTOR from endosomes/lysosomes.
Disruption of TGN trafficking induces ER stress,Citation30 and ER stress induction has been shown to decrease p-AKT, especially for Thr-308.Citation31 In this context, treatments with SG in both U87MG and WSU-DLCL2 cells up-regulated phosphorylation of eukaryotic initiation factor 2a (p-EIF2a) in a dose-dependent manner (), confirming an ER stress induction effect by SB after a 9 h treatment in cells.Citation7 Notably, a higher drug concentration was required to induce significant p-EIF2a up-regulation than to inhibit AKT phosphorylation in the same WSU-DLCL2 cells (), suggesting that induction of ER stress contributed to inhibition of AKT activation by schweinfurthins. In summary, a schematic presentation of the mechanism of anti-proliferative schweinfurthins is shown in , in which an arrest of TGN trafficking by schweinfurthins leads to lipid raft disorganization, mTOR dislocation, and ER stress induction that cooperatively decrease AKT activation and eventually, result in cell cycle arrest and apoptosis in mTOR/AKT signaling-dependent cells.
Schweinfurthins potently kill PTEN-deficient B lymphoma cells
PI3K-PTEN-AKT signaling plays a central role in regulating the proliferation and survival of haematopoietic cancer cells.Citation32,33 Genetic mutation and/or epigenetic silencing of PTEN have been observed in a large proportion of human B lymphomas, particularly in the diffuse large B cell lymphoma (DLBCL) subtype.Citation34 A potent inhibitory activity of schweinfurthins in mTOR-AKT activation suggested a potential high sensitivity of PTEN deficient DLBCL cells to schweinfurthins. Indeed, all 10 DLBCL cell lines that we tested showed great sensitivity, represented by IC50 values (single digit nM) for SG after a 4 day treatment (). These values were 2–10 times lower than those of BEZ-235, a dual mTOR/PI3K inhibitor. Importantly, normal human PBMC and fibroblasts showed much lower sensitivity, with IC50 values 10 and 450 times higher respectively, than the DLBCL cell lines tested (). These results indicate a superior activity of schweinfurthins compared to PI3K inhibitors in B lymphoma cells, with a good potential therapeutic window with respect to normal cells.
Figure 5. PTEN-deficient DLBCL cells were preferentially killed by schweinfurthin G (SG). (A) Plots of IC50 values of 10 DLBCL cell lines, normal human PBMC and fibroblasts IMR-90 upon SG treatment for 4 days. A PI3K inhibitor, BEZ-235, was included as a comparison. Cell lines with known PTEN mutation are indicated by asterisks. (B) Differential anti-proliferative effects of SG in DLBCL cells with wild type (wt) or mutant (mut) PTEN status. Protein expression of PTEN by the cell lines is shown.
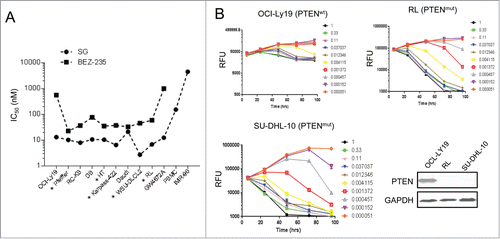
A 4 day cell proliferation assay showed that among adherent cells, PTEN deficient cancer cells were more sensitive than PTEN wild type cells to schweinfurthin treatment (). In contrast, among DLBCL cells, no significant difference was observed in IC50 values regardless of PTEN status (). We reasoned that it might be because of the higher sensitivity of DLBCL cells vs adherent cells toward schweinfurthins and thus a shortened treatment time might be needed to detect the difference. Indeed, in a time-dependent cell proliferation assay, SG inhibited the proliferation but not the survival of PTEN wild type OCI-Ly-19 cells over a period up to 4 d even at the highest dose tested (1 μM), which is roughly 100 times the IC50 for inhibition of proliferation (). A similar growth arrest effect was seen for PTEN wild type DB and Daudi cells (data not shown). In contrast, PTEN deficient RL, SU-DHL-10 and WSU-DLCL2 cells were completely eliminated by a 2 day treatment with SG (). These results thus suggested a rationale to develop schweinfurthin analogs for treating patients with DLBCL, especially those with PTEN deficiency.
Molecular interaction of schweinfurthins with ORPs
Recently, SA was shown to inhibit the binding of oxysterol to OSBP-or ORP4-overexpressing cellular membrane fractions,Citation8 and knockdown of ORP5 by siRNA in mammlian cells led to TGN trafficking disruption,Citation35 mimicking the cellular effect of schweinfurthins. Those results suggested ORPs as potential targets for schweinfurthins; however, a direct molecular interaction between ORPs and schweinfurthins had not been demonstrated. To explore the mechanism, we generated both biologically active (SG-Bio-Act) and inactive (SG-Bio-Inact) biotinylated SG derivatives whose cell growth inhibitory properties differed 200-fold () and 2 recombinant ORP proteins with partially overlapping structures, ORP1L and ORP1S, in addition to OSBP, from mammalian cells ( and Fig. S7A). Consistent with their different anti-proliferative activities, the active SG-Bio-Act impaired TGN trafficking, while the inactive SG-Bio-Inact did not (Fig. S7B). Using an ELISA method, a specific binding of SG-Bio-Act to both OSBP and ORP1S was detected, while no such interaction was observed for SG-Bio-Inact with either protein (Fig. S7C). Furthermore, in an SPR-based BIACore assay, robust dose-dependent interactions were detected for soluble ORP1L, ORP1S or OSBP to immobilized SG-Bio-Act, while no specific interaction was detected for all 3 proteins with immobilized SG-Bio-Inact ( and Fig. S7D). Binding kinetics of ORP1L to SG-Bio-Act was characterized and revealed faster association and faster dissociation compared with the binding of ORP1S with SG-Bio-Act. The overall binding affinities of ORP1L and ORP1S to SG-Bio-Act were similar, and were at sub-μM levels (Fig. S7E). These biochemical binding results demonstrated a direct molecular interaction between schweinfurthin derivatives and C-terminal sterol-binding domain (CRD) of ORPs, and correlated the binding capability of schweinfurthin derivatives to ORPs with their biological TGN trafficking blockade and cell growth inhibitory activities.
Figure 6. Schweinfurthins promoted the interaction between ORPs with PtdIns(4)P by binding to ORPs. (A) Structures of active (SG-Bio-Act) and inactive (SG-Bio-Inact) biotinylated derivatives of schweinfurthin G (SG). IC50 values of the compounds for the growth inhibition of melanoma A2058 cells are indicated. (B) Protein structures of recombinant ORPs used in this study. Proteins were expressed and isolated from HEK293 cells. (C, D) Sensorgrams of the interactions between active SG-Bio-Act and inactive SG-Bio-Inact with ORP1L (C) and ORP1S (D) from BIACore SPR analysis. For experimental details, see Methods. (E) Effects of SG in the interaction between recombinant OSBP with its lipid ligand PtdIns(4)P. 1, lysophosphatidic acid; 2, lysophosphocholine; 3, PtdIns; 4, PtdIns(3)P; 5, PtdIns(4)P; 6, PtdIns(5)P; 7, phosphatidylethanolamine; 8, phosphatidylcholine; 9, sphingosine-1-phosphate; 10, PtdIns(3,4)P2; 11, PtdIns(3,5)P2; 12, PtdIns(4,5)P2; 13, PtdIns(3,4,5)P3; 14, phosphatidic acid; 15, phosphatidylserine; 16, blank.
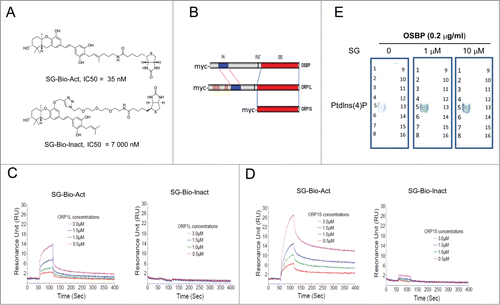
A major group of known ligands for ORPs are PIPs that bind to the pleckstrin homology (PH) domain at the N-terminal of long form ORPs () and are involved in vesicular trafficking,Citation36,37 and thus the effect of schweinfurthins on the interaction of OSBP with PIPs was further examined using a lipid overlay assay. Among the 14 PIPs tested, PI4P was identified as the major ligand for OSBP (). This is interesting because PI4P is primarily located in the Golgi apparatus and plays an important role in regulating TGN trafficking.Citation37 No binding of SG with any tested PIPs was detected (data not shown). Unexpectedly, incubation with SG enhanced the interaction between OSBP and PI4P (), suggesting that blockade of TGN trafficking by schweinfurthins may be due to a sequestration of PI4P by an enhanced interaction between certain ORPs and PI4P upon schweinfurthin binding.
Discussion
We report here a specific arresting effect on TGN trafficking as a key cellular mechanism, which resulted in potent inhibition of mTOR/AKT signaling and induction of ER stress, for the selective anti-proliferative schweinfurthin class of natural compounds. The TGN vesicular trafficking system is a complex cellular process involved in communication among multiple cellular organelles, for which there is only incomplete mechanistic understanding. mTOR/AKT signaling is the most frequently altered oncogenic pathway in human cancers, and has been a prime target for developing novel therapies for cells addicted to this signaling pathway for proliferation and/or survival. Our work thus identifies schweinfurthins as both a valuable tool for TGN biology research and a promising novel therapy lead for treating mTOR/AKT-dependent cancers such as PTEN-deficient DLBCL.
Using the combined approaches of pharmacology, bioinformatics and biochemistry, our data showed for the first time an indirect but potent inhibition of AKT phosphorylation by schweinfurthins. This effect appeared to be specific since ERK phosphorylation was not inhibited. Such a selective inhibition in mTOR/AKT signaling explains at least partially the observed selectivity of schweinfurthins toward those cancer cells having mutations in PTEN and PIK3R1, which are known to have characteristically high AKT phosphorylation. This conclusion was supported by the facts that inhibition of AKT phosphorylation paralleled cell growth inhibition but preceded apoptosis, as indicated by delayed induction of caspases 3/7. Notably, mutation status of PI3KCA, which catalyzes the formation of PIP3 from PIP2 and subsequently p-AKT, was not statistically associated with either sensitivity or resistance in our data sets, suggesting a non-completely overlapping function of PI3KCA active mutants and PTEN/PIK3R1 inactive mutants in mTOR/AKT signaling and cell proliferation. This notion seems to hold true since BEZ235, a dual PI3K/mTOR inhibitor, showed a better inhibition in growth of breast cancer cells with PTEN mutations than those with PI3KCA mutations.Citation20
Another major finding of this study is a fast and reversible “trafficking brake” of TGN circulation by schweinfurthins, which associates with inhibition of AKT phosphorylation. In order to delineate the mechanism of this association, we collected 5 lines of evidence. First, we measured the time sequence of these 2 biological effects, finding that impairment of TGN trafficking (<3 h treatment) occurred prior to inhibition of p-AKT (6–16 h treatment). Second, desialylation, another signature of defective TGN trafficking, was readily detected after 6 h treatment, a period of time that was not sufficient to inhibit p-AKT. Third, none of the tested mTOR, PI3K, or mTOR/PI3K dual inhibitors modulated TGN trafficking. Fourth, disruption of TGN trafficking impacted the transportation of complex lipids required for lipid raft integrity and PIP3 reduction, and disruption of lipid raft integrity by methyl-cyclo-dextran led to p-AKT decrease as was also seen for schweinfurthins. Finally, in schweinfurthin-treated cells, mTOR failed to form a complex with RheB due to an inappropriate cellular location after TGN trafficking blockade. The evidence collectively demonstrated that TGN trafficking arrest by schweinfurthins led to inhibition of mTOR/AKT activation through multiple mechanisms, and not the inverse. This finding, together with an earlier report that enhancement of TGN trafficking through amplification of GOLPH3 results into increased mTOR signaling and cell proliferation,Citation38 reveals an important role of TGN trafficking in mTOR/AKT signaling and cancer cell proliferation.
At the molecular level, we demonstrated that a conserved sterol-binding domain in ORPs was sufficient to bind to SG in cell-free systems. Using active and inactive probes, we have shown for the first time that the capability of SG derivatives to bind to recombinant ORPs correlates with their activities in inhibiting TGN trafficking and cancer cell proliferation. Interestingly, the interaction of an active schweinfurthin derivative to the short form ORP (ORP1S) displayed 15 times longer residence time than long form ORP (ORP1L), indicating that the short ORPs could be even better binding partners. Study of C. elegans mutants has shown that loss of an individual ORP does not affect survival but loss of all 4 ORPs results in lethality in a portion of the animals.Citation39 Synthetic lethality of ORP loss was found in c. elegans when other vesicular trafficking molecules were mutated such as vps-28,-34.Citation39 Thus, it is predicted that the anti-proliferative activity of schweinfurthins involves their binding to more than one ORP. Importantly, our biochemical data showing that the presence of SG increased the interaction between Golgi-resident PI4P and recombinant OSBP suggests a conformational change in OSBP once it has bound to a schweinfurthin. Conceivably, binding of a schweinfurthin with ORPs traps PI4P, which results in an arrest of the PI4P-dependent TGN trafficking. In this context, knockdown of mammalian ORP5Citation35 or yeast Kes1Citation40 of the ORP family was shown to modulate vesicular trafficking involving PI4P signaling. A full understanding of the details of this mechanism will be highly interesting but requires further investigation.
Impairment of TGN trafficking by schweinfurthins likely had additional outcomes besides the inhibition of mTOR/AKT activation. This was evidenced by higher anti-proliferative potency of SG compared to BEZ-235 in the DLBCL cells tested. One of the mTOR/AKT-independent outcomes that could contribute to the anti-proliferative activity of schweinfurthins is the induction of ER stress. Sustained ER stress is known to cause cell growth inhibition and cell apoptosis though multiple mechanisms.Citation41 Interestingly, ER stress has recently been reported to induce AKT phosphorylation inhibition at Thr-308, but not Ser-473,Citation30 and thus the AKT phosphorylation inhibition by schweinfurthins could also be partly contributed by the induced ER stress. Another contributing outcome derived from the TGN trafficking brake likely involves an altered metabolism of ceramide and its derivatives, which is supported by a significant impact by SG on transport of ceramide and synthesis of its derivatives in post-Golgi organelles, whose intracellular accumulation induces apoptosis with multiple but poorly defined mechanisms.Citation41,42 Notably, expression of membrane glycoprotein receptors such as EGFR and CD98hc was not significantly altered by SG, indicating different post-Golgi routes or mechanisms involved in the transportation and trafficking of complex lipids and of membrane receptors. In addition, protein glycosylation plays important roles in the dimerization and activation of various receptors,Citation43 and desialylation by schweinfurthins could be also part of the anti-proliferative mechanism of a TGN trafficking brake. Therefore, there are probably multiple molecular pathways that cooperatively lead to the anti-proliferative activity after schweinfurthins inhibit the TGN.
In summary, we have identified an indirect but potent inhibitory activity of schweinfurthin natural compounds in mTOR/AKT signaling for their selective anti-proliferative activity by blocking TGN trafficking through multiple mechanisms. Our data suggest a novel approach to modulate oncogenic mTOR/AKT signaling and thus treating tumors using schweinfurthin-derived compounds.
Materials and Methods
Natural schweinfurthin compounds and their derivatives
Schweinfurthins E and F were isolated from a natural source as reported.Citation3 Schweinfurthin G and its fluorescent, biotinylated and methylated derivatives were synthesized at Eisai. The synthesis methods and the compound characterization can be found in Supplemental Materials and Methods.
In vitro cell proliferation assay
The assays for cancer cells and non-dividing immortalized normal human fibroblast were performed as reported using CellTiter-Glo (Promega, MI).Citation44 IC50 values were defined as the concentration which inhibited cell growth to 50% of control cell populations that received DMSO treatment only.
Bioinformatics
The mutation status of each gene analyzed in cancer cell lines was determined based on the “Catalog of somatic mutation in cancer” (COSMIC) database. For statistical analysis of each gene, cell lines were divided into mutant and wild type groups. Both Wilcoxon rank-sum test and the t-test were used on the IC50 and GI50 values of the cell lines from an in-house cancer cell panel and the reported NCI-60 cancer cell panel, respectively. All statistical tests were performed using the R statistical software.Citation45 For the correlation study between PTEN expression and IC50 values of schweinfurthins, we extracted the PTEN protein expression levels from the same NCI database of the cell lines that we used in this study.
Immunoprecipitation and western blot
Cells in the growth phase were treated with compounds for indicated time periods. Cell lysates were subjected to immunoprecipitation by incubation with antibody and protein A/G beads or were directly separated with SDS-PAGE followed by incubation of blotted membranes with primary antibody or biotinylated lectins. The bound antibody or lectin was visualized with fluorescent secondary antibody and quantified using an Odyssey imaging system. Equal protein amounts or housekeeping proteins such as β-actin, GAPDH or Collagen IX were run as loading controls.
Lipid overlay assay
Binding specificity of recombinant human ORPs to PIPs were determined using PIP MicroStrips (Echelon, UT) according to the manufacturer's manual. Briefly, the membranes with an array of 15 spotted PIPs were incubated with recombinant ORPs with or without SG or biotinylated SG at RT for 1 h, and the bound protein or biotinylated derivatives were detected by HRP-conjugated anti-myc antibody and HRP-conjugated streptavidin, respectively. The interaction was visualized by TMB.
Flow cytometry and immunofluorescence
For flow cytometric analyses of cell surface staining, cells were incubated with fluorescent antibody followed by detection using a Canto-1 6-color flow cytometry instrument (BD). To visualize intracellular molecules, cells were treated with fixation and permeabilization reagents before antibody staining. For cellular immunofluorescence, cells growing on glass coverslips were fixed and permeabilized with 0.1% Trixon X-100. After blocking with 2% normal goat serum, cells were incubated with primary antibody and then secondary antibody in the presence of blocking solution. Nuclei were stained with Hoechst 33342 (Sigma). Confocal images were acquired using a confocal microscope (Leica Microsystems), and regular immunofluorescence was imaged using an Olympus fluorescence microscopy equipped with FluorView software. Automated high resolution fluorescence imaging was conducted using an In Cell Analyzer 2000 (GE Health). The image photos were edited with Corel PaintShop for clarity.
In vitro binding assays
The interactions between recombinant ORPs and schweinfurthin derivatives were characterized by ELISA and surface plasmon resonance (SPR) using a BIACore T100 instrument. Details can be found in Supplemental Material and Methods.
Author Contributions
X.B., W.Z., Q.X., J.W., Y.T., T.U. and K.N. conceived and designed the study. X.B, W.Z., N.H.S., K.L.A., Q.X., K.T., Z.W., W.L., L.P., H.C., W.L., Y.S., N.T., Z.D., H.D., and Y.K. acquired and analyzed data. X.B., W.Z., N.Z., M.W.J., M.P., and K.N. wrote the paper. D.G.I.K. provided material support.
Disclosure of Potential Conflicts of Interest
No potential conflicts of interest were disclosed.
KCBT 1019184 Supplemental Data.pdf
Download PDF (1.5 MB)Acknowledgments
The paper is dedicated to the memory of Jue-Lon Shie. We thank Drs. Hiroyuki Arai and Tomohiko Taguchi at University of Tokyo for providing the plasmids encoding human ORP family proteins. We gratefully acknowledge technical support, discussion and comments from Bruce Littlefield, Tina Watanabe, May Chin, Christine Kitsos, Guobing Miao, Dayong Qiu, Karen Ackermann, Kuan-chun Huang, Hiroyuki Katayama, Lei Wang, Jesse Chow, Yasuhiro Funahashi, Junji Matsui, Akihiko Tsuruoka, Yoshiya Oda, Takashi Owa and Kentaro Yoshimatsu of Eisai Product Creation Systems.
Funding
The isolation of natural schweinfurthins in Dr. Kingston's lab was supported by the NIH under Cooperative Agreement U01 TW000313 with the International Cooperative Biodiversity Groups.
Supplemental Material
Supplemental data for this article can be accessed on the publisher's website.
References
- Hung DT, Jamison TF, Schreiber SL. Understanding and controlling the cell cycle with natural products. Chem Biol 1996; 3:623–39; PMID:8807895; http://dx.doi.org/10.1016/S1074-5521(96)90129-5
- Beutler JA, Shoemaker RH, Johnson T, Boyd MR. Cytotoxic geranyl stilbenes from Macaranga schweinfurthii. J Nat Prod 1998; 61:1509–12; PMID:9868152; http://dx.doi.org/10.1021/np980208m
- Yoder BJ, Cao S, Norris A, Miller JS, Ratovoson F, Razafitsalama, J, Andriantsiferana, R, Rasamison, VE, Kingston, DGl. Antiproliferative prenylated stilbenes and flavonoids from Macaranga alnifolia from the Madagascar rainforest. J Nat Prod 2007; 70:342–6; PMID:17326683; http://dx.doi.org/10.1021/np060484y
- Neighbors JD, Salnikova MS, Beutler JA, Wiemer DF. Synthesis and structure-activity studies of schweinfurthin B analogs: Evidence for the importance of a D-ring hydrogen bond donor in expression of differential cytotoxicity. Bioorg Med Chem 2006; 14:1771–84; PMID:16290161; http://dx.doi.org/10.1016/j.bmc.2005.10.025
- Turbyville TJ, Gürsel DB, Tuskan RG, Walrath JC, Lipschultz CA, Lockett SJ, Wiemer DF, Beutler JA, Reilly KM. Schweinfurthin A selectively inhibits proliferation and Rho signaling in glioma and neurofibromatosis type 1 tumor cells in a NF1-GRD-dependent manner. Mol Cancer Ther 2010; 9:1234–43; PMID:20442305; http://dx.doi.org/10.1158/1535-7163.MCT-09-0834
- Kodet JG, Wiemer DF. Synthesis of indole analogues of the natural schweinfurthins. J Org Chem 2013; 78:9291–302; PMID:24004185; http://dx.doi.org/10.1021/jo4014244
- Kuder CH, Sheehy RM, Neighbors JD, Wiemer DF, Hohl RJ. Functional evaluation of a fluorescent schweinfurthin:mechanism of cytotoxicity and intracellular quantification. Mol Pharmacol 2012; 82:9–16; PMID:22461663; http://dx.doi.org/10.1124/mol.111.077107
- Burgett AW, Poulsen TB, Wangkanont K, Anderson DR, Kikuchi C, Shimada K, Okubo S, Fortner KC, Mimaki Y, Kuroda M, et al. Natural products reveal cancer cell dependence on oxysterol-binding proteins. Nat Chem Biol, 2011; 7:639–47; PMID:21822274; http://dx.doi.org/10.1038/nchembio.625
- Raychaudhuri S, Prinz WA. The diverse functions of oxysterol-binding proteins. Annu Rev Cell Dev Biol 2010; 26:157–77; PMID:19575662; http://dx.doi.org/10.1146/annurev.cellbio.042308.113334
- Hanahan D, Weinberg RA. Hallmarks of cancer: the next generation. Cell 2011; 144:646–74; PMID:21376230; http://dx.doi.org/10.1016/j.cell.2011.02.013
- Huse JT, Holland EC. Targeting brain cancer: advances in the molecular pathology of malignant glioma. Nat Rev Cancer 2010; 10:319–31; PMID:20414201; http://dx.doi.org/10.1038/nrc2818
- Hoeller D, Volarevic S, Dikic I. Compartmentalization of growth factor receptor signalling. Curr Opin Cell Biol 2005; 17:107–11; PMID:15780584; http://dx.doi.org/10.1016/j.ceb.2005.01.001
- Gonnord P, Blouin CM, Lamaze C. Membrane trafficking and signaling: two sides of the same coin. Semin Cell Dev Biol 2011; 23:154–64; PMID:22085846; http://dx.doi.org/10.1016/j.semcdb.2011.11.002
- Pfeffer SR. Rab GTPase regulation of membrane identity. Curr Opin Cell Biol 2013:25:414–9; PMID:23639309; http://dx.doi.org/10.1016/j.ceb.2013.04.002
- Nagy P, Vereb G, Sebestyén Z, Horváth G, Lockett SJ, Damjanovich S, Park JW, Jovin TM, Szollosi J. Lipid rafts and the local density of ErbB proteins influence the biological role of homo-and heteroassociations of ErbB2. J Cell Sci 2002; 115:4251–62; PMID:12376557; http://dx.doi.org/10.1242/jcs.00118
- Huo H, Guo X, Hong S, Jiang M, Liu X, Liao K. Lipid rafts/caveolae are essential for insulin-like growth factor-1 receptor signaling during 3T3-L1 preadipocyte differentiation induction. J Biol Chem 2003; 278:11561–9; PMID:12538586; http://dx.doi.org/10.1074/jbc.M211785200
- Santiago-Tirado FH, Bretscher A. Membrane-trafficking sorting hubs: cooperation between PI4P and small GTPases at the trans-Golgi network. Trends Cell Biol 2011; 21:515–25; PMID:21764313; http://dx.doi.org/10.1016/j.tcb.2011.05.005
- Cheung LW, Hennessy BT, Li J, Yu S, Myers AP, Djordjevic B, Lu Y, Stemki-Hale K, Dyer MD, Zhang F, et al. High frequency of PIK3R1 and PIK3R2 mutations in endometrial cancer elucidates a novel mechanism for regulation of PTEN protein stability. Cancer Discov 2011; 1:170–85; PMID:21984976; http://dx.doi.org/10.1158/2159-8290.CD-11-0039
- James MF, Han S, Polizzano C, Plotkin SR, Manning BD, Stemmer-Rachamimov AO, Gusella JF, Ramesh V. NF2/merlin is a novel negative regulator of mTOR complex 1, and activation of mTORC1 is associated with meningioma and schwannoma growth. Mol Cell Biol 2009; 29:4250–4261; PMID:19451225; http://dx.doi.org/10.1128/MCB.01581-08
- Maira SM, Stauffer F, Brueggen J, Furet P, Schnell C, Fritsch C, Brachmann S, Chene P, De Pover A, Schoemaker K, et al. Identification and characterization of NVP-BEZ235, a new orally available dual phosphatidylinositol 3-kinase/mammalian target of rapamycin inhibitor with potent in vivo antitumor activity. Mol Cancer Ther 2008; 7:1851–63; PMID:18606717; http://dx.doi.org/10.1158/1535-7163.MCT-08-0017
- Smith DF, Song X, Cummings RD. Use of glycan microarrays to explore specificity of glycan-binding proteins. Methods Enzymol 2010; 480:417–44; PMID:20816220; http://dx.doi.org/10.1016/S0076-6879(10)80033-3
- Bao X, Kobayashi M, Hatakeyama S, Angata K, Gullberg D, Nakayama J, Fukuda MN, Fukuda M. Tumor suppressor function of laminin-binding alpha-dystroglycan requires a distinct beta3-N-acetylglucosaminyltransferase. PNAS USA 2009; 106:12109–14; PMID:19587235; http://dx.doi.org/10.1073/pnas.0904515106
- El-Battari A, Prorok M, Angata K, Mathieu S, Zerfaoui M, Ong E, Suzuki M, Lombardo D, Fukuda M. Different glycosyltransferases are differentially processed for secretion, dimerization, and autoglycosylation. Glycobiology 2003; 13:941–53; PMID:14514709; http://dx.doi.org/10.1093/glycob/cwg117
- Topczewski JJ, Kuder CH, Neighbors JD, Hohl RJ, Wiemer DF. Fluorescent schweinfurthin B and F analogs with anti-proliferative activity. Bioorg Med Chem 2010; 18:6734–41; PMID:20724169; http://dx.doi.org/10.1016/j.bmc.2010.07.056
- Burd CG. Physiology and pathology of endosome-to-Golgi retrograde sorting. Traffic 2011; 12:956–62; PMID:21463456; http://dx.doi.org/10.1111/j.1600-0854.2011.01188.x
- Hill MM, Feng J, Hemmings BA. Identification of a plasma membrane Raft-associated PKB Ser473 kinase activity that is distinct from ILK and PDK1. Curr Biol 2002; 12:1251–5; PMID:12176337; http://dx.doi.org/10.1016/S0960-9822(02)00973-9
- Holstein SA, Kuder CH, Tong H, Hohl RJ. Pleiotropic effects of a schweinfurthin on isoprenoid homeostasis. Lipids 2011; 46:907–21; PMID:21633866; http://dx.doi.org/10.1007/s11745-011-3572-y
- Isotani S, Hara K, Tokunaga C, Inoue H, Avruch J, Yonezawa K. Immunopurified mammalian target of rapamycin phosphorylates and activates p70 S6 kinase alpha in vitro. J Biol Chem, 1999; 274:344933–8; PMID:10567431; http://dx.doi.org/10.1074/jbc.274.48.34493
- Sancak Y, Peterson TR, Shaul YD, Lindquist RA, Thoreen CC, Bar-Peled L, Sabatini DM. The Rag GTPases bind raptor and mediate amino acid signaling to mTORC1. Science 2008; 320:1496–501; PMID:18497260; http://dx.doi.org/10.1126/science.1157535
- Kim I, Xu W, Reed JC. Cell death and endoplasmic reticulum stress: disease relevance and therapeutic opportunities. Nat Rev Drug Disc 2008; 7:1013–30; PMID:19043451; http://dx.doi.org/10.1038/nrd2755
- Yung HW, Charnock-Jones DS, Burton GJ. Regulation of AKT phosphorylation at Ser473 and Thr308 by endoplasmic reticulum stress modulates substrate specificity in a severity dependent manner. PloS One 2011; 6:e17894; PMID:21445305; http://dx.doi.org/10.1371/journal.pone.0017894
- Küppers R. Mechanisms of B-cell lymphoma pathogenesis. Nat Rev Cancer 2005; 5:251–62; PMID:15803153; http://dx.doi.org/10.1038/nrc1589
- Kloo B, Nagel D, Pfeifer M, Grau M, Düwel M, Vincendeau M, Dorken B, Lenz P, Lenz G, Krappmann D. Critical role of PI3K signaling for NF-kappaB-dependent survival in a subset of activated B-cell-like diffuse large B-cell lymphoma cells. PNAS USA 2011; 108:272–7; PMID:21173233; http://dx.doi.org/10.1073/pnas.1008969108
- Pfeifer M, Grau M, Lenze D, Wenzel SS, Wolf A, Wollert-Wulf B, Dietze K, Nogai H, Storek B, Madle H, et al. PTEN loss defines a PI3K/AKT pathway-dependent germinal center subtype of diffuse large B-cell lymphoma. PNAS USA 2013; 110:12420–5; PMID:23840064; http://dx.doi.org/10.1073/pnas.1305656110
- Du X, Kumar J, Ferguson C, Schulz TA, Ong YS, Hong W, Prinz WA, Parton RG, Brown AJ, Yang H. A role for oxysterol-binding protein-related protein 5 in endosomal cholesterol trafficking. J Cell Biol 2011; 192:121–35; PMID:21220512; http://dx.doi.org/10.1083/jcb.201004142
- Raychaudhuri S, Im YJ, Hurley JH, Prinz WA. Nonvesicular sterol movement from plasma membrane to ER requires oxysterol-binding protein-related proteins and phosphoinositides. J Cell Biol 2006; 173:107–19; PMID:16585271; http://dx.doi.org/10.1083/jcb.200510084
- Wood CS, Schmitz KR, Bessman NJ, Setty TG, Ferguson KM, Burd CG. PtdIns4P recognition by Vps74/GOLPH3 links PtdIns 4-kinase signaling to retrograde Golgi trafficking. J Cell Biol 2009; 187:967–75; PMID:20026658; http://dx.doi.org/10.1083/jcb.200909063
- Scott KL, Kabbarah O, Liang MC, Ivanova E, Anagnostou V, Wu J, Dhakal S, Wu M, Chen S, Feinberg, T, et al. GOLPH3 modulates mTOR signalling and rapamycin sensitivity in cancer. Nature 2009; 459:1085–90; PMID:19553991; http://dx.doi.org/10.1038/nature08109
- Kobuna H, Inoue T, Shibata M, Gengyo-Ando K, Yamamoto A, Mitani S, Arai H. Multivesicular body formation requires OSBP-related proteins and cholesterol. PLoS Genet 2010; 6. Pii:e1001055; PMID:20700434; http://dx.doi.org/10.1371/journal.pgen.1001055
- Mousley CJ, Yuan P, Gaur NA, Trettin KD, Nile AH, Deminoff SJ, Dewar BJ, Wolpert M, Macdonald JM, Herman PK. A sterol-binding protein integrates endosomal lipid metabolism with TOR signaling and nitrogen sensing. Cell 2012; 148:702–15; PMID:22341443; http://dx.doi.org/10.1016/j.cell.2011.12.026
- Morad SA, Cabot MC. Ceramide-orchestrated signaling in cancer cells. Nat Rev Cancer 2013; 13:51–65; PMID:23235911; http://dx.doi.org/10.1038/nrc3398
- Ogretmen B, Hannun YA. Biologically active sphingolipids in cancer pathogenesis and treatment. Nat Rev Cancer 2004; 4:604–16; PMID:15286740; http://dx.doi.org/10.1038/nrc1411
- Ohtsubo K, Marth JD. Glycosylation in cellular mechanisms of health and disease. Cell 2006; 126:855–67; PMID:16959566; http://dx.doi.org/10.1016/j.cell.2006.08.019
- Xu Q, Huang KC, Tendyke K, Marsh J, Liu J, Qiu D, Littlefield BA, Nomoto K, Atasoylu O, Risatti CA, Sperry JB, Smith AB 3rd. In vitro and in vivo anticancer activity of (+)-spongistatin 1. Anticancer Res 2011; 31:2773–9; PMID:21868519
- R Development Core Team. R: A Language and Environment for Statistical Computing. 2010; Vienna, Austria: R Foundation for Statistical Computing.