ABSRTRACT
Signaling plays an important role in regulating all cellular pathways. Altered signaling is one of the hallmarks of cancers. Phosphoproteomics enables interrogation of kinase mediated signaling pathways in biological systems. In cancers, this approach can be utilized to identify aberrantly activated pathways that potentially drive proliferation and tumorigenesis. To identify signaling alterations in head and neck squamous cell carcinoma (HNSCC), we carried out proteomic and phosphoproteomic analysis of HNSCC cell lines using a combination of tandem mass tag (TMT) labeling approach and titanium dioxide-based enrichment. We identified 4,920 phosphosites corresponding to 2,212 proteins in six HNSCC cell lines compared to a normal oral cell line. Our data indicated significant enrichment of proteins associated with splicing. We observed hyperphosphorylation of SRSF protein kinase 2 (SRPK2) and its downstream substrates in HNSCC cell lines. SRPK2 is a splicing kinase, known to phosphorylate serine/arginine (SR) rich domain proteins and regulate splicing process in eukaryotes. Although genome-wide studies have reported the contribution of alternative splicing events of several genes in the progression of cancer, the involvement of splicing kinases in HNSCC is not known. In this study, we studied the role of SRPK2 in HNSCC. Inhibition of SRPK2 resulted in significant decrease in colony forming and invasive ability in a panel of HNSCC cell lines. Our results indicate that phosphorylation of SRPK2 plays a crucial role in the regulation of splicing process in HNSCC and that splicing kinases can be developed as a new class of therapeutic target in HNSCC.
Abbreviations
HNSCC | = | Head and neck squamous cell carcinoma |
TiO2 | = | Titanium dioxide |
TMT | = | Tandem mass tag |
Introduction
Head and neck squamous cell carcinoma (HNSCC) is a heterogeneous group of cancers with high mortality.Citation1 Although targeted therapy for EGFR has been used in the treatment of HNSCC; the development of resistance is common.Citation2,3 Studies have shown that aberrant activation of kinase signaling pathways contribute to abnormality in cellular processes which results in tumorigenesis.Citation4,5 Oncogenic activation of PI3K, Notch and hedgehog signaling pathways have been reported in HNSCC and they are being tested in clinical studies as potential targets.Citation6-8
Mass spectrometry-based proteome/phosphoproteome profiling has become a reliable method for studying the signaling pathways/events that are dysregulated in any given system. In this study, we carried out LC-MS/MS analysis of TMT-labeled, titanium dioxide (TiO2) enriched phosphopeptides to study the signaling pathways that are altered in HNSCC cells compared to a normal oral cell line OKF6/TERT1. Mass spectrometry-based analysis led to the identification of 4,920 phosphosites in 2,212 proteins. Among the phosphorylated proteins, we observed hyperphosphorylation (fold change ≥ 1.5 in at least four HNSCC cell lines compared to normal cell line) of various serine/threonine kinases including cyclin dependent kinase 1 (CDK1), epidermal growth factor receptor (EGFR), protein kinase D2 (PRKD2), MAP/microtubule affinity-regulating kinase 2 (MARK2), maternal embryonic leucine zipper kinase (MELK), protein kinase N2 (PKN2), ATR serine/threonine kinase (ATR), SRSF protein kinase 2 (SRPK2) and ribosomal protein S6 kinase (RPS6KB2). Bioinformatics analysis of the data revealed significant enrichment of proteins involved in splicing.
Gene expression in eukaryotes is mainly regulated by alternative splicing, where the pre-mRNA is modified to mature mRNA through post-transcriptional modifications. Genome-wide sequencing studies have revealed extensive changes in splicing patterns in more than 90% of human protein coding genes.Citation9,10 The complex of proteins that catalyze splicing mechanism known as ‘spliceosome’ is a macromolecular complex containing five small nuclear ribonuclear proteins and ˜200 accessory proteins including various kinases and signaling molecules.Citation11,12 Aberrant activation or expression of splice factors and kinases has been shown to influence cellular transformation and other oncogenic processes.Citation13,14 Among the proteins involved in splicing, we identified hyperphosphorylation of SRPK2, a splicing kinase known to affect subcellular localization and activity of splice factors.Citation15 We also identified several substrates of SRPK2 to be aberrantly expressed or phosphorylated in HNSCC cells. Though studies have shown the significance of splicing proteins in multiple diseases including cancer, the key role of SRPK2 in HNSCC is not known. We studied the role of SRPK2 and its potential as a therapeutic target in head and neck squamous cell carcinoma.
Results
Quantitative proteomic profile of HNSCC
To study the dysregulated proteome of HNSCC cells, we employed TMT labeling approach. The experimental workflow employed for the study is depicted in . LC-MS/MS analysis of 12 bRPLC fractions was carried out using LTQ-Orbitrap Velos mass spectrometer. MS/MS data was searched against human protein database (RefSeq 65) using SEQUEST and Mascot search algorithms through Proteome Discoverer platform suite (version 1.4.1.14, Thermo Scientific). We identified a total of 3,255 proteins. Among these, 538 proteins were found to be upregulated (fold change ≥1.5) and 590 proteins were found to be downregulated (fold change ≤ 0.67) in at least four HNSCC cell lines used in this study compared to the normal cell line. A partial list of molecules differentially expressed in HNSCC cell lines compared to OKF6/TERT1 cell line is provided in . Among the differentially expressed proteins, proteins such as BAG family molecular chaperone regulator 2 (BAG2), actin-binding protein anillin (ANLN), Caprin 1(CAPRIN1), Lymphokine-activated killer T-cell-originated protein kinase (PBK) and WD repeat and HMG-box DNA-binding protein 1 (WDHD1) are some of the molecules which are overexpressed in all the HNSCC cell lines studied. The complete list of identified proteins and their corresponding peptides is provided in Tables S1 and S2, respectively.
Table 1. A partial list of molecules overexpressed in HNSCC cell lines.
Table 2. A partial list of molecules hyperphosphorylated in HNSCC cell lines.
Identification of dysregulated phosphoproteins in HNSCC cell lines
Since we observed widespread dysregulation of protein expression in HNSCC cell lines compared to the normal oral cell line, we sought to study the altered signaling events in these cells using phosphoproteomic approach. Protein phosphorylation plays a crucial role in intracellular signaling. Identification of post-translational modifications in cancer cells will not only help in elucidating the signaling mechanisms but also help in the identification of potential novel therapeutic candidates. We performed TMT-based quantitative phosphoproteomic analysis to identify dysregulated signaling in HNSCC cell lines compared to a normal oral cell line OKF6/TERT1 (). The enriched (pSer/Thr) peptides were analyzed on LTQ-Orbitrap Velos mass spectrometer. With a false discovery rate (FDR) cutoff of 1% and PhosphoRS probability cutoff of 75%, we identified a total of 4,920 phosphosites from 2,212 proteins (Table S3). The peptides with a fold change ±1.5 were considered as hyperphosphorylated or hypophosphorylated. Based on these, we identified hyperphosphorylation of 1,299 sites and hypophosphorylation of 639 sites in at least four HNSCC cell lines. These corresponded to 878 hyperphosphorylated proteins and 402 hypophosphorylated proteins respectively. Among the proteins identified, 23 kinases were found to be hyperphosphorylated in all HNSCC cell lines. The distribution of phosphosites identified in all cells is shown in . Among the phosphosites identified, 88% of phosphorylation sites accounted for serine phosphorylation, 11% of phosphorylation sites for threonine residues and 1% of phosphorylation sites for tyrosine residues. A heat map representing the phosphorylation changes of a subset of kinases identified in HNSCC cell lines compared to OKF6/TERT1 is depicted in . To identify consensus motifs enriched in the datasets, we further carried out motif analysis using motif-x algorithm. From the hyperphosphorylated phosphopeptides, we identified 4 distinct phosphorylation motifs including pSPxK, pSPxR, pSPxRK and pSPxxxK. These are proline directed motifs where serine is followed by proline at +1 position ().
Figure 2. Summary statistics of the analysis. (A) Distribution of phosphoserine, phosphothreonine and phosphotyrosine sites (B) Heat map of the phosphorylation changes in a subset of kinases identified in HNSCC cell lines compared to OKF6/TERT1. (C) Motifs that were found to be enriched in the hyperphosphorylated kinase sites in the data set.
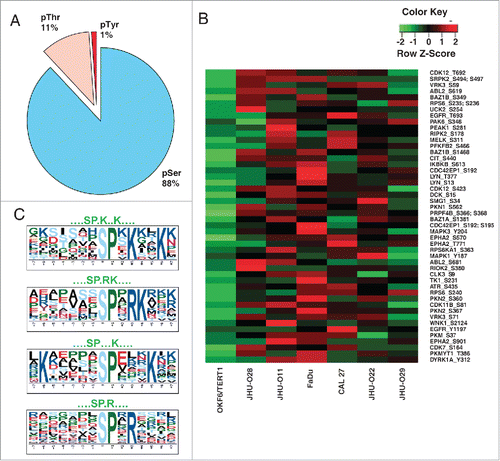
We performed bioinformatics analysis of differentially phosphorylated proteins and categorized them based on the cellular localization and biological function (Fig. S1). The classifications were based on annotations in HPRD.Citation16,17 Our analysis revealed that 21% of the proteins were involved in regulation of nucleic acid metabolism; 18% in signal transduction, 15% in cell communication and 11% in cellular growth and maintenance. Classification of the proteins based on subcellular localization revealed 40% of the hyperphosphorylated proteins localized to the nucleus and 25% localized to the cytoplasm. Among the other predominant protein groups, 7% of the proteins were localized to plasma membrane.
Pathway analysis revealed the dysregulation of proteins associated with splicing in HNSCC
To gain insights into the altered signaling pathways in HNSCC, we used GeneSpring GX v12.6.1 (Agilent Technologies) for analysis. The list of overexpressed proteins identified from proteomic data (538) and hyperphosphorylated proteins from phosphoproteomic (878) data were included for pathway analysis. The analysis was carried out using Pathway Architect module of GeneSpring wherein both data sets were mapped on to pathways. Among these, mRNA processing pathway was found to be significantly enriched in HNSCC cells compared to the normal cell line. Out of 127 proteins depicted in the pathway diagram (), 32 proteins from our phosphoproteomic data and 16 proteins from proteomic data were found to map on to this pathway. These include splicing kinase such as SRSF protein kinase 2 (SRPK2), Serine/threonine-protein kinase PRP4 homolog (PRPF4B) and various other splicing factors including, serine/arginine-rich splicing factor 2 (SRSF2), serine/arginine-rich splicing factor 9 (SRSF9), members of heterogeneous ribonuclear protein family (hnRNPs), RNA binding motif protein 17 (RBM17), splicing factor 3b (SF3B1) and DEAH (Asp-Glu-Ala-His) box polypeptide 16 (DHX16) (). MS/MS spectra for representative peptides from SRSF2 (T29) and SRPK2 (S494 and S497) are shown in . In agreement with the mass spectrometry data, we observed hyperphosphorylation of SRPK2 (Ser 494) in HNSCC cells using western blot analysis (). Further we analyzed the expression of SRPK2 in a panel of HNSCC cell lines. Western blot analysis of SRPK2 revealed an increase in the expression of the protein in HNSCC cells compared to the non-neoplastic oral cells ().
Figure 3. Pathway analysis revealed dysregulation of proteins associated with splicing machinery in HNSCC. Gene spring version 12.6.1 was used for pathway analysis. Molecules that mapped to the mRNA processing pathway from the phosphoproteomic data are highlighted in green, molecules that mapped from the proteomic data are highlighted in orange and molecules that are common in both data sets are highlighted in yellow. The fold changes are represented using heat strips.
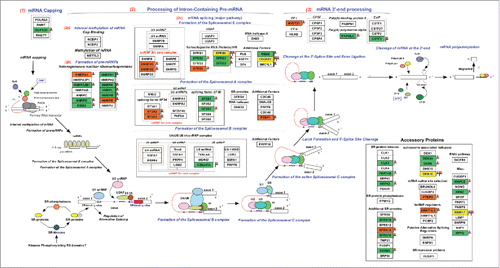
Figure 4. Representative MS and MS/MS spectra of phosphopeptides identified in HNSCC cells (A) serine/arginine-rich splicing factor 2 (SRSF2) (B) SRSF protein kinase 2 (SRPK2) (C) Western blot analysis shows overexpression and hyperphosphorylation of SRPK2 in a panel of HNSCC cell lines compared to OKF6/TERT1. (D) Western blot analysis shows a decrease in SRPK2 expression in HNSCC cell lines transfected with SRPK2 siRNA. β-actin was used as a loading control.
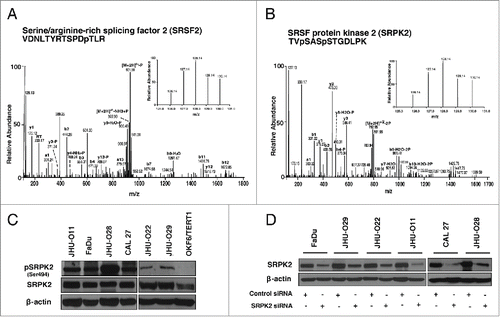
Invasive and colony forming properties of HNSCC cells
To understand if SRPK2 had any association with features of transformed cells such as loss of contact inhibition and increased cell migration, we examined the colony formation and invasive ability of the HNSCC cells. Except JHU-O11 and JHU-O28, all HNSCC cells were capable of forming well defined colonies. The colonies of JHU-O11 and JHU-O28 were less clearly defined than those of other HNSCC cells, indicating cell scattering as evidenced by the invasion data. Our data also revealed that all HNSCC cells except JHU-O29 have a high invasive ability. Overall, our data indicate that three of the HNSCC cell lines (FaDu, CAL 27 and JHU-O22) had both high colony forming and invasive ability, whereas the other cells exhibited only a high colony forming ability (JHU-O29) or only a high invasive ability (JHU-O11 and JHU-O28) (Fig. S2).
Inhibition of SRPK2 reduced the colony forming ability of HNSCC cells
Silencing of SRPK2 using its specific siRNA was confirmed by Western blot analysis (). siRNA mediated silencing of SRPK2 resulted in a decrease in the colony forming ability of HNSCC cells () compared to control siRNA transfected cells. Using an alternative approach, we studied the colony forming ability of HNSCC cells by inhibiting the activity of SRPK2 using SRPIN340, a small molecule inhibitor of SRPK2.Citation18 In agreement with the results observed using siRNA-mediated silencing, inhibition of SRPK2 by SRPIN340 led to a significant decrease in the colony formation ability of HNSCC cell lines ().
Figure 5. Inhibition of SRPK2 reduces the colony forming ability of HNSCC cells. Colony formation assay following (A) siRNA mediated knockdown of SRPK2 or control siRNA in a panel of HNSCC cell lines, as indicated. Colonies formed were visualized after staining with methylene blue. (B) A graphical representation of the colony forming ability of the HNSCC cells upon SRPK2 silencing *p < 0.05. (C) Colony forming ability of the HNSCC cells upon inhibition of SRPK2 using SRPIN340 or control (DMSO), in the indicated panel of HNSCC cells (D) A graphical representation of the colony forming ability of HNSCC cells upon SRPK2 inhibition *p < 0.05.
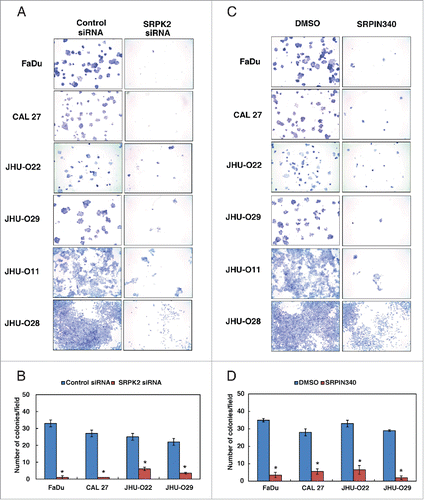
Inhibition of SRPK2 decreases the invasive ability of HNSCC cells
Our data showed that SRPK2 plays a significant role in the proliferative ability of HNSCC cells. siRNA mediated silencing of SRPK2 led to a significant reduction in the invasive property of all HNSCC cells (). In agreement with the siRNA results, inhibition of SRPK2 with SRPIN340 resulted in significant decrease in the invasive property of all HNSCC cells (). Taken together, our results indicate that SRPK2 plays an important role in regulating the oncogenic potential of HNSCC cells.
Figure 6. Inhibition of SRPK2 reduces the invasive ability of HNSCC cells. Invasion assays were carried out using in a transwell system using Matrigel-coated filters and the number of cells that migrated to the lower chamber was counted. Cells that migrated are visualized following methylene blue staining in a panel of HNSCC cell lines as indicated. (A) HNSCC cell lines were transfected with either control (Scrambled) or SRPK2 siRNA and invaded cells were photographed (B) A graphical representation of the invasive ability of the HNSCC cells upon SRPK2 silencing *p < 0.05. (C) HNSCC cells were treated with a small molecule inhibitor of SRPK2 (SRPIN340) or vehicle control (DMSO) and invaded cells were photographed. (D) A graphical representation of the invasive ability of the HNSCC cells upon SRPK2 inhibition *p < 0.05.
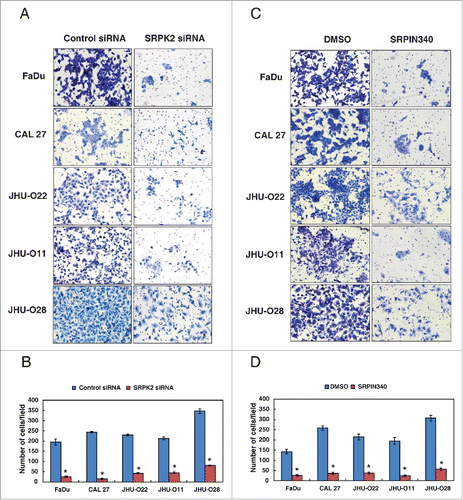
Discussion
Activation of multiple signaling pathways can be directly assessed by studying the global phosphorylation events. This is because a large majority of activated kinases can be identified due to an increase in their phosphorylation status and the corresponding pathways can be determined by their substrates that are phosphorylated. In recent years, kinases have become one of the most intensively studied groups of proteins as drug targets. In this study, we used mass spectrometry-based quantitative proteomic and phosphoproteomic analysis to identify altered signaling pathways in HNSCC. Our analysis revealed significant enrichment of molecules involved in mRNA processing / splicing. Among the splicing proteins, serine arginine (SR) proteins are a group of RNA binding proteins, which possess a unique serine/arginine (SR) domain. These proteins are important for constitutive as well as alternative pre-mRNA splicing. The splicing function of SR proteins is modulated by kinases which results in altered localization of these proteins in the cell.
Recent studies have shown an association between altered splicing machinery and cancers.Citation19,20 Dysregulation of splicing has been reported as an important factor in leukemia.Citation21-23 Alterations in spliceosome associated genes/proteins can significantly impact alternative splicing events like exon skipping or intron retention as reported in breast cancer, lung adenocarcinoma and chronic lymphocytic leukemia.Citation22,24,25 We identified dysregulation of multiple proteins encoded by genes involved in pre-mRNA splicing. Overexpression (≥ 1.5 fold) of splicing regulators including SRSF5, SRSF6, SF3A2, SF3A3 and RBM17 was observed in HNSCC cells compared to a normal oral cell line. Additionally, we observed significant dysregulation in the phosphorylation pattern of splicing regulators across HNSCC cell lines. Our phosphoproteome data indicates hyperphosphorylation (≥ 1.5 fold) of proteins including SRSF2, SRSF6, SRSF9, SRSF11, PRPF4B and SRPK2 in the HNSCC cell lines. We observed a 2-fold increase in phosphorylation of serine/arginine rich protein kinase 2 (SRPK2) in the panel of HNSCC cell lines. This is also evident from our Western blot data, where the expression of pSRPK2 was below detectable levels in the normal oral keratinocytes.
Though multiple studies have looked into the landscape of alternate splice forms of various proteins as biomarkers, there are limited studies on the role of splicing kinases in cancer progression.Citation26-28 Several studies have shown the involvement of various members of splicing kinases, especially SRPKs in multiple cellular processes including proliferation and angiogenesis.Citation29,30 Overexpression of splicing factors (SRSF1, SRSF2) has been reported in lung, colon and breast cancers.Citation13,31,32 SR protein specific kinases (SRPK1, 2 and 3), which is a highly conserved group of kinases, phosphorylates the SR domain and regulates the splicing activity of SR proteins.Citation14,33 Among the members of SRPKs, the role of SRPK1 in cancer has been established. Overexpression of SRPK1 has been reported in breast, colon and pancreatic cancers.Citation31 siRNA mediated silencing of SRPK1 in ovarian cancer cell lines resulted in reduced cell proliferation and increased sensitivity to cisplatin.Citation34 Inhibition of SRPK1 using siRNA or pharmacological inhibitor suppressed VEGF expression and decreased melanoma growth in vivo. Studies by Zhou et al., have shown that SRPKs constitute a major branch of Akt signaling pathway in EGF mediated alternate splicing.Citation35
SRPK2 is a serine arginine rich protein specific kinase that phosphorylates SR family of splicing factors at serine residue located in serine arginine rich regions.Citation29 It has been shown that phosphorylation of acinus by SRPK2 results in the expression of cyclin A1 and plays a key role in promoting leukemia cell proliferation.Citation29 SRPK2 phosphorylates SRSF2 in nuclear speckles and mediate the pre-mRNA splicing events.Citation33 Karni et al., have reported SRSF2 to be upregulated in multiple human tumors and its overexpression leads to alternative splicing of the tumor suppressor proteins bridging integrator 1(BIN1), mitogen-activated protein kinase-interacting serine/threonine kinase 2 (MNK2) and ribosomal S6 kinase (S6K1).Citation36 The alternative splice variant of BIN1 lacks tumor-suppressor activity whereas the MNK2 and S6K1 isoforms have oncogenic properties.Citation36 SRSF6, another target of SRPK2, is one of the most ubiquitous splicing factors that play an important role in constitutive splicing as well as in the selection of alternative splice sites.Citation37 Filippov et al., has shown that SRSF6 depletion leads to an increased resistance to DNA damage in p53 dependent way in U2OS cells.Citation37 Thus altered expression of SRSF6 upon DNA damage has been known to affect changes in the splicing activity/ pattern.Citation37 SRSF9, another target of SRPK2, has been reported to be overexpressed in different cancers including ovarian, prostate and bladder cancer.Citation38-40 Additionally, SRSF11 another regulator of alternative splicingCitation40 was hyperphosphorylated (>2 fold) in our data.Citation41 Studies have reported SRPK1 and SRPK2 to be overexpressed in non-small cell lung cancer and their overexpression correlated with hyperphosphorylation of SRSF1 and SRSF2.Citation13 In concurrence with these studies, our data reveals both hyperphosphorylation and overexpression of SRPK2 in HNSCC cell lines. Taken together, these studies highlight the crucial role of SRPKs and their substrates in the regulation of splicing in cancer. Our in vitro results demonstrate that inhibition of SRPK2 leads to reduction in growth, invasion and migration of HNSCC cells. Overall, our study indicates a widespread dysregulation in the genes/proteins involved in splicing machinery in the HNSCC cells. We hypothesize that the aberrant regulation of SRPK2 and other genes involved in splicing may lead to altered splicing in HNSCC cells, which needs to be systematically evaluated further. Overall, our study provides a scaffold for future studies to investigate the regulation of splicing and development of SR protein-specific kinases as a new class of therapeutic targets in HNSCC.
Materials and methods
Cell culture
FaDu and CAL 27 were purchased from American Type Culture Collection (ATCC, Manassas, VA). OKF6/TERT1, a normal oral keratinocyte cell line,Citation41 was a gift from Dr. James Rheinwald (Brigham and Women's Hospital in Boston, MA).Citation42 HNSCC cell lines, JHU-O11, JHU-O22, JHU-O28, JHU-O29 and FaDu were cultured in RPMI-1640 with 10% FBS. CAL 27 was cultured in DMEM with 10% FBS. All cell lines were grown in a humidified incubator with 5% CO2 at 37°C as described previously.Citation43 The cell lines used for the study were authenticated by short tandem repeat analysis.
Cell lysis, protein digestion and TMT labeling
Cells were cultured till 70% confluency and serum starved for 12 h. After serum starvation, cells were washed three times with 1X phosphate buffer saline (PBS), harvested and lysed in lysis buffer (2% SDS, 5 mM sodium fluoride, 1 mM β-glycerophosphate, 1 mM sodium orthovanadate in 50 mM Triethyl ammonium bicarbonate (TEABC)). The cell lysates were sonicated and centrifuged at 12,000 rpm for 10 minutes. The protein concentration was estimated using bicinchoninic acid assay (BCA).Citation44 Equal amount of protein from each cell line was reduced and alkylated as described previously.Citation43 We employed filter aided sample preparation (FASP) protocol as described earlier to remove SDS.Citation45 Protein digestion was carried using TPCK treated trypsin (1:20) at 37°C for 12–16 h. Peptides from each cell line were labeled using TMT labeling method as per manufacturer's instruction (Catalog # 90110, Thermo Fisher Scientific). Peptides derived from OKF6/TERT1 were labeled with TMT tag 126, JHU-O28 with TMT tag 127N, JHU-O11 with TMT tag 127C, FaDu with TMT tag 128N, CAL 27 with TMT tag 128C, JHU-O22 with TMT tag 129N and JHU-O29 with TMT tag 130C.
Basic reversed-phase liquid chromatography (bRPLC) and phosphopeptides enrichment using TiO2
The labeled peptides were subjected to bRPLC fractionation generating 96 fractions, as described previously.Citation46 The fractions were concatenated into 12 fractions. From these pooled fractions 100 µg equivalent peptides were taken for total proteome analysis. All fractions were lyophilized and subjected to TiO2-based enrichment.Citation47,48 Briefly, the phosphopeptide enriched- TiO2 beads were washed two times with 80% ACN in 3% TFA, eluted using 4% ammonia solution and neutralized with 3% TFA. Eluted peptides were vacuum dried and desalted using C18 Stage Tips.
LC-MS/MS analysis
LC-MS/MS analysis of total proteome and enriched fractions of phosphopeptides was carried using an LTQ-Orbitrap Velos mass spectrometer interfaced with Proxeon Easy nanoLC II system (Thermo Scientific).Citation46 The settings used for phosphopeptide analysis were: a) MS1 scans were acquired from m/z: 350–1800 with resolution of 60,000 at 400 m/z, b) Ten most intense precursor ions were selected for MS2 analysis. Precursor ions were fragmented using HCD fragmentation at a resolution of 30,000 at m/z 400 (isolation width: 1.90 m/z; normalized collision energy: 43%; activation time: 0.1 ms.
Mass spectrometry data analysis
The mass spectrometry derived data was searched against Human RefSeq protein database (Version 65, containing 36211 protein entries with common contaminants added) using SEQUEST and MASCOT search algorithms through Proteome Discoverer platform (version 1.4.1.14, Thermo Scientific). For both the algorithms search parameters included maximum of two missed cleavages, carbamidomethylation at cysteine, TMT 6-plex (+229.163) modification at N-terminus of peptide and lysine were set as fixed modification while oxidation of methionine and phosphorylation at serine, threonine and tyrosine as variable modifications. For MS data, monoisotopic peptide mass tolerance was set to 20 ppm and MS/MS tolerance to 0.1 Da. PhosphoRS (version 3.1) was used to calculate the confident localization of phosphosite within the identified peptide (Phospho RS score ≥75).Citation49 False discovery rate of 1% was set at the PSM level. The complete mass spectrometry data generated from this study has been deposited to the ProteomeXchange Consortium (http://www.proteomexchange.org) via PRIDE partner repository with the dataset identifier PXD001862.
Bioinformatics analysis
Biological process and cellular localization information were obtained from Human Protein Reference Database (HPRD).Citation16,50 Pathway analysis was done using GeneSpring GX software (version 12.6.1). Using Pathway Architect module of GeneSpring GX, the proteins that were found to be overexpressed and hyperphosphorylated in at least four cell lines with a fold change of 1.5 was included for pathway analysis. For predicting consensus motifs based on the phosphosites identified in the study, we used motif-x algorithm.Citation51 The parameters used include (i) the flanking sequences around the phosphosites with a window size of 15 aminoacids long were extracted from RefSeq 65 database and used for extracting consensus motif. For phosphorylation sites that were localized at the region of the N- or C-termini, the surrounding sequence could not be extended and were excluded, (ii) the significance threshold was set to p < 0.01, (iii) the minimum occurrence of motifs was set to 10.
Colony formation assays
Colony formation assays were carried out as described previously.Citation52 Briefly, the cells were seeded at a density of 3×103, transfected with either SRPK2 siRNA or a scrambled siRNA as a control. The cells were allowed to grow for 10–12 days and the colonies were fixed with methanol, stained with 4% methylene blue and counted. Similarly the colony forming ability of the HNSCC cells were studied in presence of SRPK1/2 inhibitor (SRPIN340 - 10 µM, Catalog # sc-394310, Santa Cruz Biotechnology). The number of colonies formed was counted for ten randomly selected viewing fields and representative images were photographed at 2.5x magnification. All experiments were done in triplicate.
Cell invasion assays
Invasion assay was carried out in a transwell system (BD Biosciences) using Matrigel-coated filters as described.Citation52 Briefly, the invasive ability of the cells was assayed using polyethylene terephthalate (PET) membrane (8-μm pore size) (Catalog # 353097, BD Biosciences). The upper compartment of the culture insert was coated with Matrigel (Catalog # 354234, BD Biosciences). 2×104 cells were seeded into the transwell chambers in presence of serum-free medium. Complete media was added to the lower compartment and the cells were incubated at 37°C in 5% CO2 incubator for 48 h. After incubation, the non-migratory cells from the upper chamber were removed with a cotton-tip applicator. Cells that migrated to the lower side of membrane were fixed and stained using 4% methylene blue. The number of cells that penetrated through the matrigel and membrane was counted for ten randomly selected viewing fields at 10x magnification. All the experiments were done in triplicate.
Western blot analysis
Cells were cultured to 80% confluency and proteins were harvested in RIPA lysis buffer (10 mM Tris pH 7.4, 150 mM NaCl, 5 mM EDTA, 1% Triton-X-100, 0.1% SDS containing protease and phosphatase inhibitor cocktails) and sonicated. Mouse anti-SRPK2 antibody was obtained from BD transduction laboratories (Catalog # 611118). Phospho-SRPK2 (S494) antibody was obtained from Millipore (Catalog # 07–1817). Western blot analysis was carried out as described previously.Citation53
Disclosure of potential conflicts of interest
No potential conflicts of interest were disclosed.
Supplemental_Datas.zip
Download Zip (5.7 MB)Acknowledgments
We thank the Department of Biotechnology (DBT), Government of India for research support to the Institute of Bioinformatics (IOB), Bangalore. We thank the “Infosys Foundation” for research support to IOB. AC was supported by Department of Science and Technology (DST) grants (SERC/LS-439/2011 and SR/SO/HS/0208/2013). IOB is supported by DBT Program Support on Neuroproteomics and infrastructure for proteomic data analysis (BT/01/COE/08/05). This work was supported by NCI's Clinical Proteomic Tumor Analysis Consortium initiative (U24CA160036) and FAMRI-funded 072017_YCSA. AR, GS and SC are recipients of Senior Research Fellowship from Council of Scientific and Industrial Research (CSIR), Government of India. RR is a recipient of research associateship from DBT. Harsha Gowda is a Wellcome Trust/DBT India Alliance Early Career Fellow. We thank Strand Life Sciences, Bangalore and Agilent Technologies for providing access to GeneSpring. We acknowledge Dr. Manoj Kumar Kashyap for critically reviewing the manuscript. We thank Dr. S. K. Shankar and Dr. Anita Mahadevan of National Institute of Mental Health and Neurological Sciences, for providing access to the microscopy imaging facility.
References
- Rothenberg SM, Ellisen LW. The molecular pathogenesis of head and neck squamous cell carcinoma. J Clin Invest 2012; 122:1951-7; PMID:22833868; http://dx.doi.org/10.1172/JCI59889
- Benavente S, Huang S, Armstrong EA, Chi A, Hsu KT, Wheeler DL, Harari PM. Establishment and characterization of a model of acquired resistance to epidermal growth factor receptor targeting agents in human cancer cells. Clin Cancer Res 2009; 15:1585-92; PMID:19190133; http://dx.doi.org/10.1158/1078-0432.CCR-08-2068
- Wang Z, Martin D, Molinolo AA, Patel V, Iglesias-Bartolome R, Degese MS, Vitale-Cross L, Chen Q, Gutkind JS. mTOR co-targeting in cetuximab resistance in head and neck cancers harboring PIK3CA and RAS mutations. J Natl Cancer Inst 2014; 106:dju215; http://dx.doi.org/10.1093/jnci/dju215
- Harsha HC, Pandey A. Phosphoproteomics in cancer. Mol Oncol 2010; 4:482-95; PMID:20937571; http://dx.doi.org/10.1016/j.molonc.2010.09.004
- Zhang X, Belkina N, Jacob HK, Maity T, Biswas R, Venugopalan A, Shaw PG, Kim MS, Chaerkady R, Pandey A, et al. Identifying novel targets of oncogenic EGF receptor signaling in lung cancer through global phosphoproteomics. Proteomics 2015; 15:340-55; PMID:25404012; http://dx.doi.org/10.1002/pmic.201400315
- Freudlsperger C, Burnett JR, Friedman JA, Kannabiran VR, Chen Z, Van Waes C. EGFR-PI3K-AKT-mTOR signaling in head and neck squamous cell carcinomas: attractive targets for molecular-oriented therapy. Expert Opin Ther Targets 2011; 15:63-74; PMID:21110697; http://dx.doi.org/10.1517/14728222.2011.541440
- Psyrri A, Seiwert TY, Jimeno A. Molecular pathways in head and neck cancer: EGFR, PI3K, and more. Am Soc Clin Oncol Educ Book 2013:246-55; PMID:23714515; http://dx.doi.org/10.1200/EdBook_AM.2013.33.246
- Bowles DW, McDermott JD, Jimeno A. Novel treatments for head and neck squamous cell carcinoma: preclinical identification and clinical investigation. Future Oncol 2014; 10:1065-80; PMID:24941990; http://dx.doi.org/10.2217/fon.14.18
- Pan Q, Shai O, Lee LJ, Frey BJ, Blencowe BJ. Deep surveying of alternative splicing complexity in the human transcriptome by high-throughput sequencing. Nat Genet 2008; 40:1413-5; PMID:18978789; http://dx.doi.org/10.1038/ng.259
- Wang ET, Sandberg R, Luo S, Khrebtukova I, Zhang L, Mayr C, Kingsmore SF, Schroth GP, Burge CB. Alternative isoform regulation in human tissue transcriptomes. Nature 2008; 456:470-6; PMID:18978772; http://dx.doi.org/10.1038/nature07509
- Naro C, Sette C. Phosphorylation-mediated regulation of alternative splicing in cancer. Int J Cell Biol 2013; 2013:151839; PMID:24069033; http://dx.doi.org/10.1155/2013/151839
- Will CL, Luhrmann R. Spliceosome structure and function. Cold Spring Harb Perspect Biol 2011; 3:a003707; PMID:21441581; http://dx.doi.org/10.1101/cshperspect.a003707
- Gout S, Brambilla E, Boudria A, Drissi R, Lantuejoul S, Gazzeri S, Eymin B. Abnormal expression of the pre-mRNA splicing regulators SRSF1, SRSF2, SRPK1 and SRPK2 in non small cell lung carcinoma. PLoS One 2012; 7:e46539; PMID:23071587; http://dx.doi.org/10.1371/journal.pone.0046539
- Zhou Z, Fu XD. Regulation of splicing by SR proteins and SR protein-specific kinases. Chromosoma 2013; 122:191-207; PMID:23525660; http://dx.doi.org/10.1007/s00412-013-0407-z
- Koizumi J, Okamoto Y, Onogi H, Mayeda A, Krainer AR, Hagiwara M. The subcellular localization of SF2/ASF is regulated by direct interaction with SR protein kinases (SRPKs). J Biol Chem 1999; 274:11125-31; PMID:10196197; http://dx.doi.org/10.1074/jbc.274.16.11125
- Goel R, Muthusamy B, Pandey A, Prasad TS. Human protein reference database and human proteinpedia as discovery resources for molecular biotechnology. Mol Biotechnol 2011; 48:87-95; PMID:20927658; http://dx.doi.org/10.1007/s12033-010-9336-8
- Muthusamy B, Thomas JK, Prasad TS, Pandey A. Access guide to human proteinpedia. Curr Protoc Bioinformatics 2013; Chapter 1:Unit 1 21; 41:1.21:1.21.1-1.21.15; PMID:23504933
- Fukuhara T, Hosoya T, Shimizu S, Sumi K, Oshiro T, Yoshinaka Y, Suzuki M, Yamamoto N, Herzenberg LA, Hagiwara M. Utilization of host SR protein kinases and RNA-splicing machinery during viral replication. Proc Natl Acad Sci U S A 2006; 103:11329-33; PMID:16840555; http://dx.doi.org/10.1073/pnas.0604616103
- Grosso AR, Martins S, Carmo-Fonseca M. The emerging role of splicing factors in cancer. EMBO Rep 2008; 9:1087-93; PMID:18846105; http://dx.doi.org/10.1038/embor.2008.189
- Venables JP, Klinck R, Bramard A, Inkel L, Dufresne-Martin G, Koh C, Gervais-Bird J, Lapointe E, Froehlich U, Durand M, et al. Identification of alternative splicing markers for breast cancer. Cancer Res 2008; 68:9525-31; PMID:19010929; http://dx.doi.org/10.1158/0008-5472.CAN-08-1769
- Hsu TY, Simon LM, Neill NJ, Marcotte R, Sayad A, Bland CS, Echeverria GV, Sun T, Kurley SJ, Tyagi S, et al. The spliceosome is a therapeutic vulnerability in MYC-driven cancer. Nature 2015; 525:384-8; PMID:26331541; http://dx.doi.org/10.1038/nature14985
- Kashyap MK, Kumar D, Villa R, La Clair JJ, Benner C, Sasik R, Jones H, Ghia EM, Rassenti LZ, Kipps TJ, et al. Targeting the spliceosome in chronic lymphocytic leukemia with the macrolides FD-895 and pladienolide-B. Haematologica 2015; 100:945-54; PMID:25862704; http://dx.doi.org/10.3324/haematol.2014.122069
- Wang L, Lawrence MS, Wan Y, Stojanov P, Sougnez C, Stevenson K, Werner L, Sivachenko A, DeLuca DS, Zhang L, et al. SF3B1 and other novel cancer genes in chronic lymphocytic leukemia. N Engl J Med 2011; 365:2497-506; PMID:22150006; http://dx.doi.org/10.1056/NEJMoa1109016
- Eswaran J, Horvath A, Godbole S, Reddy SD, Mudvari P, Ohshiro K, Cyanam D, Nair S, Fuqua SA, Polyak K, et al. RNA sequencing of cancer reveals novel splicing alterations. Sci Rep 2013; 3:1689; PMID:23604310; http://dx.doi.org/10.1038/srep01689
- Zhang Q, Li H, Jin H, Tan H, Zhang J, Sheng S. The global landscape of intron retentions in lung adenocarcinoma. BMC Med Genomics 2014; 7:15; PMID:24646369; http://dx.doi.org/10.1186/1755-8794-7-15
- Brinkman BM. Splice variants as cancer biomarkers. Clin Biochem 2004; 37:584-94; PMID:15234240; http://dx.doi.org/10.1016/j.clinbiochem.2004.05.015
- Xu Y, Gao XD, Lee JH, Huang H, Tan H, Ahn J, Reinke LM, Peter ME, Feng Y, Gius D, et al. Cell type-restricted activity of hnRNPM promotes breast cancer metastasis via regulating alternative splicing. Genes Dev 2014; 28:1191-203; PMID:24840202; http://dx.doi.org/10.1101/gad.241968.114
- Zhang F, Wang M, Michael T, Drabier R. Novel alternative splicing isoform biomarkers identification from high-throughput plasma proteomics profiling of breast cancer. BMC Syst Biol 2013; 7 Suppl5:S8; PMID:24565027; http://dx.doi.org/10.1186/1752-0509-7-S5-S8
- Jang SW, Yang SJ, Ehlen A, Dong S, Khoury H, Chen J, Persson JL, Ye K. Serine/arginine protein-specific kinase 2 promotes leukemia cell proliferation by phosphorylating acinus and regulating cyclin A1. Cancer Res 2008; 68:4559-70; PMID:18559500; http://dx.doi.org/10.1158/0008-5472.CAN-08-0021
- Oltean S, Gammons M, Hulse R, Hamdollah-Zadeh M, Mavrou A, Donaldson L, Salmon AH, Harper SJ, Ladomery MR, Bates DO. SRPK1 inhibition in vivo: modulation of VEGF splicing and potential treatment for multiple diseases. Biochem Soc Trans 2012; 40:831-5; PMID:22817743; http://dx.doi.org/10.1042/BST20120051
- Hayes GM, Carrigan PE, Miller LJ. Serine-arginine protein kinase 1 overexpression is associated with tumorigenic imbalance in mitogen-activated protein kinase pathways in breast, colonic, and pancreatic carcinomas. Cancer Res 2007; 67:2072-80; PMID:17332336; http://dx.doi.org/10.1158/0008-5472.CAN-06-2969
- Kurokawa K, Akaike Y, Masuda K, Kuwano Y, Nishida K, Yamagishi N, Kajita K, Tanahashi T, Rokutan K. Downregulation of serine/arginine-rich splicing factor 3 induces G1 cell cycle arrest and apoptosis in colon cancer cells. Oncogene 2014; 33:1407-17; PMID:23503458; http://dx.doi.org/10.1038/onc.2013.86
- Wang HY, Lin W, Dyck JA, Yeakley JM, Songyang Z, Cantley LC, Fu XD. SRPK2: a differentially expressed SR protein-specific kinase involved in mediating the interaction and localization of pre-mRNA splicing factors in mammalian cells. J Cell Biol 1998; 140:737-50; PMID:9472028; http://dx.doi.org/10.1083/jcb.140.4.737
- Odunsi K, Mhawech-Fauceglia P, Andrews C, Beck A, Amuwo O, Lele S, Black JD, Huang RY. Elevated expression of the serine-arginine protein kinase 1 gene in ovarian cancer and its role in Cisplatin cytotoxicity in vitro. PLoS One 2012; 7:e51030; PMID:23236423; http://dx.doi.org/10.1371/journal.pone.0051030
- Zhou Z, Qiu J, Liu W, Zhou Y, Plocinik RM, Li H, Hu Q, Ghosh G, Adams JA, Rosenfeld MG, et al. The Akt-SRPK-SR axis constitutes a major pathway in transducing EGF signaling to regulate alternative splicing in the nucleus. Mol Cell 2012; 47:422-33; PMID:22727668; http://dx.doi.org/10.1016/j.molcel.2012.05.014
- Karni R, de Stanchina E, Lowe SW, Sinha R, Mu D, Krainer AR. The gene encoding the splicing factor SF2/ASF is a proto-oncogene. Nat Struct Mol Biol 2007; 14:185-93; PMID:17310252; http://dx.doi.org/10.1038/nsmb1209
- Filippov V, Schmidt EL, Filippova M, Duerksen-Hughes PJ. Splicing and splice factor SRp55 participate in the response to DNA damage by changing isoform ratios of target genes. Gene 2008; 420:34-41; PMID:18571879; http://dx.doi.org/10.1016/j.gene.2008.05.008
- Mukherji M, Brill LM, Ficarro SB, Hampton GM, Schultz PG. A phosphoproteomic analysis of the ErbB2 receptor tyrosine kinase signaling pathways. Biochemistry 2006; 45:15529-40; PMID:17176074; http://dx.doi.org/10.1021/bi060971c
- Yoshino H, Enokida H, Chiyomaru T, Tatarano S, Hidaka H, Yamasaki T, Gotannda T, Tachiwada T, Nohata N, Yamane T, et al. Tumor suppressive microRNA-1 mediated novel apoptosis pathways through direct inhibition of splicing factor serine/arginine-rich 9 (SRSF9/SRp30c) in bladder cancer. Biochem Biophys Res Commun 2012; 417:588-93; PMID:22178073; http://dx.doi.org/10.1016/j.bbrc.2011.12.011
- Zhu J, Gong JY, Goodman OB, Jr, Cartegni L, Nanus DM, Shen R. Bombesin attenuates pre-mRNA splicing of glucocorticoid receptor by regulating the expression of serine-arginine protein p30c (SRp30c) in prostate cancer cells. Biochim Biophys Acta 2007; 1773:1087-94; PMID:17540466; http://dx.doi.org/10.1016/j.bbamcr.2007.04.016
- Wu JY, Kar A, Kuo D, Yu B, Havlioglu N. SRp54 (SFRS11), a regulator for tau exon 10 alternative splicing identified by an expression cloning strategy. Mol Cell Biol 2006; 26:6739-47; PMID:16943417; http://dx.doi.org/10.1128/MCB.00739-06
- Dickson MA, Hahn WC, Ino Y, Ronfard V, Wu JY, Weinberg RA, Louis DN, Li FP, Rheinwald JG. Human keratinocytes that express hTERT and also bypass a p16(INK4a)-enforced mechanism that limits life span become immortal yet retain normal growth and differentiation characteristics. Mol Cell Biol 2000; 20:1436-47; PMID:10648628; http://dx.doi.org/10.1128/MCB.20.4.1436-1447.2000
- Syed N, Chavan S, Sahasrabuddhe NA, Renuse S, Sathe G, Nanjappa V, Radhakrishnan A, Raja R, Pinto SM, Srinivasan A, et al. Silencing of high-mobility group box 2 (HMGB2) modulates cisplatin and 5-fluorouracil sensitivity in head and neck squamous cell carcinoma. Proteomics 2014; 15(2–3):383-93
- Walker JM. The bicinchoninic acid (BCA) assay for protein quantitation. Methods Mol Biol 1994; 32:5-8; PMID:7951748; http://dx.doi.org/10.1385/0-89603-268-X:5
- Kim MS, Pinto SM, Getnet D, Nirujogi RS, Manda SS, Chaerkady R, Madugundu AK, Kelkar DS, Isserlin R, Jain S, et al. A draft map of the human proteome. Nature 2014; 509:575-81; PMID:24870542; http://dx.doi.org/10.1038/nature13302
- Selvan LD, Renuse S, Kaviyil JE, Sharma J, Pinto SM, Yelamanchi SD, Puttamallesh VN, Ravikumar R, Pandey A, Prasad TS, et al. Phosphoproteome of Cryptococcus neoformans. J Proteomics 2014; 97:287-95; PMID:23851311; http://dx.doi.org/10.1016/j.jprot.2013.06.029
- Larsen MR, Thingholm TE, Jensen ON, Roepstorff P, Jorgensen TJ. Highly selective enrichment of phosphorylated peptides from peptide mixtures using titanium dioxide microcolumns. Mol Cell Proteomics 2005; 4:873-86; PMID:15858219; http://dx.doi.org/10.1074/mcp.T500007-MCP200
- Pinto SM, Nirujogi RS, Rojas PL, Patil AH, Manda SS, Subbannayya Y, Roa JC, Chatterjee A, Prasad TS, Pandey A. Quantitative phosphoproteomic analysis of IL-33-mediated signaling. Proteomics 2015; 15:532-44; PMID:25367039; http://dx.doi.org/10.1002/pmic.201400303
- Taus T, Kocher T, Pichler P, Paschke C, Schmidt A, Henrich C, Mechtler K. Universal and confident phosphorylation site localization using phosphoRS. J Proteome Res 2011; 10:5354-62; PMID:22073976; http://dx.doi.org/10.1021/pr200611n
- Keshava Prasad TS, Goel R, Kandasamy K, Keerthikumar S, Kumar S, Mathivanan S, Telikicherla D, Raju R, Shafreen B, Venugopal A, et al. Human Protein Reference Database–2009 update. Nucleic Acids Res 2009; 37:D767-72; PMID:18988627; http://dx.doi.org/10.1093/nar/gkn892
- Schwartz D, Gygi SP. An iterative statistical approach to the identification of protein phosphorylation motifs from large-scale data sets. Nat Biotechnol 2005; 23:1391-8; PMID:16273072; http://dx.doi.org/10.1038/nbt1146
- Subbannayya Y, Syed N, Barbhuiya MA, Raja R, Marimuthu A, Sahasrabuddhe N, Pinto SM, Manda SS, Renuse S, Manju HC, et al. Calcium calmodulin dependent kinase kinase 2 - a novel therapeutic target for gastric adenocarcinoma. Cancer Biol Ther 2015; 16:336-45; PMID:25756516; http://dx.doi.org/10.4161/15384047.2014.972264
- Chang X, Ravi R, Pham V, Bedi A, Chatterjee A, Sidransky D. Adenylate kinase 3 sensitizes cells to cigarette smoke condensate vapor induced cisplatin resistance. PLoS One 2011; 6:e20806; PMID:21698293; http://dx.doi.org/10.1371/journal.pone.0020806