ABSTRACT
Previous studies have indicated that miR-128 was downregulated in a variety of cancers including colorectal cancer (CRC). However, the role and the underlying molecular mechanisms of miR-128 in CRC still remain largely unknown. The aim of this study was to investigate the level of miR-128-1-5p in CRC patients and to explore both the effects and regulatory mechanisms of miR-128-1-5p in the malignancy of CRC. Real-time PCR and western blot were used to analyze the expression levels of miR-128-1-5p and the direct downstream target protein tyrosine kinase C theta isoform (PRKCQ). Cell Counting Kit-8, clone formation, TUNEL apoptosis assays, and subcutaneous tumor model were performed to investigate the malignant ability of colon cancer cells. A luciferase assay was performed to explore whether miR-128-1-5p could directly bind to 3’-UTR region of PRKCQ. In the present study, we detected the decreased expression and clinical significances of miR-128-1-5p in colorectal cancer tissues and cell lines. Functional experiments revealed that miR-128-1-5p inhibited cell proliferation and induced cell apoptosis and that PRKCQ was identified as a target of miR-128-1-5p and involved in miR-128-1-5p-mediated proliferation and apoptosis. In conclusion, our results showed that miR-128-1-5p reduced CRC growth by modulating PRKCQ expression and is a possible new therapeutic target for patients with CRC.
Introduction
Colorectal cancer (CRC) is one of the most frequently diagnosed malignancies in the worldCitation1. The incidence of this disease is increasing, with about 1.8 million new cases and more than 880,000 deaths from CRC in 2018Citation2. Despite advances have made in current therapies, including surgical resection and adjuvant chemotherapy, the recurrence and metastasis of CRC are still two of the obstacles affecting its therapeutic effectCitation3,Citation4. Therefore, it is urgent to explore the underlying molecular pathological mechanisms in CRC progression, and it may provide a new therapeutic strategy for improving the clinical outcome of patients with CRC.
MicroRNAs (miRNAs) are a type of highly conserved and endogenous noncoding RNA, with 17–25 nucleotides long in size. Growing evidences support that miRNAs have played crucial roles in progression of various cancersCitation5,Citation6. MiRNAs can regulate various cellular processes, including cell proliferation, differentiation, apoptosis, migration and metastasisCitation5,Citation7,Citation8. Aberrant expression of miRNAs is frequently found in human cancers and associated with uncontrolled proliferation and metastasisCitation9–11. It was reported that miR-128 exhibited both oncogenic and tumor-suppressive roles in different cancer types. For example, miR-128 expression was remarkably down-regulated in thyroid cancer and acted as a tumor suppressor to inhibit the growth of thyroid cancer by negatively regulating sphingosine kinase-1 (SPHK1)Citation12. Low expression of miR-128 was correlated with shorter overall and disease-free survival in triple-negative breast cancer (TBNC) and miR-128 could inhibit glucose metabolism, mitochondrial respiration and proliferation of TNBC cells by targeting glucose metabolism, mitochondrial respiration and proliferation of TNBC cellsCitation13. However, high level of microRNA-128-3p (miR-128-3p) was found in non-small cell lung cancerCitation14. Mechanistically investigations suggested that miR-128 directed cancer stem cells (CSCs) and epithelial mesenchymal transition (EMT) programming by activating β-catenin and TGF-β signalingCitation14. Recently, miR-128-3p was reported to increase the sensitivity of CRC cells to oxaliplatin and could act as a clinical biomarker for oxaliplatin responseCitation15. Therefore, it is of great significance to explore what exact role of miR-128-1-5p plays and the relative mechanisms in CRC.
In this study, we explored the clinical significance and biological functions of miR-128-1-5p in CRC. Further experiments demonstrated that overexpression of miR-128-1-5p could inhibit CRC cell proliferation and facilitate CRC cell apoptosis by targeting PRKCQ. PRKCQ is a novel family of serine/threonine kinases, that is involved in regulating multiple essential cellular processes such as survival, proliferation, and differentiationCitation16. In addition, PRKCQ inhibition was proved enhancing chemosensitivity by regulating the expression of a pro-apoptotic Bcl2 family member BimCitation17. This finding might provide potential candidate for CRC therapies and highlight potential clinical applications of miR-128-1-5p in CRC.
Results
MiR-128-1-5p levels are downregulated in CRC with poor survival
Using CancerMIRNome database for the identification of prognostic miRNA biomarkers, we found that expression of miR-128-1-5p was investigated to be strongly associated with optimal survival in colon adenocarcinoma based on Hazard Ratio (HR = 0.39, p<0.001; ). Then, the expression of miR-128-1-5p in serum was found to be significantly downregulated in most human cancers, including CRC, compared with the healthy subjects (). The comparison of miR-128-1-5p expression between cancer and adjacent normal tissue in CancerMIRNome database revealed significantly lower miR-128-1-5p expression in colon adenocarcinoma (p<0.001; ) and rectum adenocarcinoma (p<0.001; ). To evaluate the association between miR-128-1-5p and prognosis, the Kaplan – Meier survival analysis was performed by CancerMIRNome. Patients with high miR-128-1-5p expression levels had significantly higher overall survival in colon adenocarcinoma (HR = 0.39, p<0.001; ). Similar trend was also found in rectum adenocarcinoma, although there was no significant difference (HR = 0.39, p = .17; ). Further, The ROC curve showed that miR-128-1-5p has better diagnostic ability in colon adenocarcinoma (AUC = 0.91; ) and rectum adenocarcinoma (AUC = 0.97, ).
Figure 1. The expression of MiR-128-1-5p and its correlation with prognosis in colon adenocarcinoma.
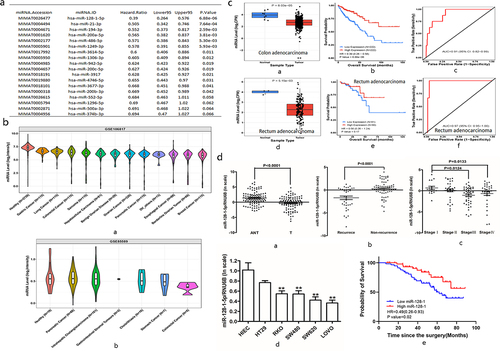
To better understand the role of miR-128-1-5p in CRC, we collected 107 pairs of CRC tissues and their respective adjacent normal tissues. Our results showed that the expression of miR-128-1-5p in CRC tissues was significantly down-regulated compared with their corresponding normal tissues (p<0.001; ). The expression levels of miR-128-1-5p were associated with recurrence (p<0.001; ) and AJCC stage (). Similarly, the expression of miR-128-1-5p in CRC cell lines (HT29, RKO, SW480, SW620 and LOVO) was significantly lower compared with the immortalized colon epithelial cell line HIEC (). To evaluate whether miR-128-1-5p expression levels are correlated with CRC progression, the relationship between miR-128-1-5p levels and clinicopathological parameters of CRC patients was assessed. As shown in , miR-128-1-5p levels were correlated with AJCC (p = .0333), histopathological grade (p = .0111), and distant metastasis (p = .0271). Survival analysis using the Kaplan-Meier method revealed that higher miR-128-1-5p levels were associated with longer overall survival in CRC patients (HR = 0.49, p = .02, ). These results suggested that miR-128-1-5p might play a critical role in the development and progression of colorectal cancer.
Table 1. Relationship between clinicopathological factors and miR-128-1-5p expression in CRC tissues.
MiR-128-1-5p inhibits CRC cell proliferation and induces cell apoptosis
To confirm the biological effects of miR-128-1-5p on CRC development, we overexpressed miR-128-1-5p in SW620 and LOVO CRC cell lines which had lower endogenous miR-128-1-5p among aforementioned cell lines. As shown in , overexpression of miR-128-1-5p led to a significant increase of miR-128-1-5p level in SW620 and LOVO cells, as determined by qRT-PCR. Overexpression of miR-128-1-5p decreased the proliferation rate of SW620 () and LOVO cells (. Colony formation assay also indicated that upregulation of miR-128-1-5p resulted in a significant decrease of colony number (). To explore the possible mechanism underlying the inhibitory effect on cell growth by miR-128-1-5p, cell apoptosis was performed. Flow cytometry showed that the apoptosis rate of CRC cells in the miR-128-1-5p overexpression cells was significantly higher than that in the vector (). Western blot also showed that overexpression of miR-128-1-5p increased apoptosis-related proteins total caspase-3 and cleaved caspase-3, and Bcl2-associated x protein (Bax), and reduced apoptosis inhibitory protein B-cell lymphoma 2(Bcl-2) (). These data implicated that miR-128-1-5p acted as a suppressor gene by regulating cell growth.
Figure 2. The biological effects of miR-128-1-5p in CRC.
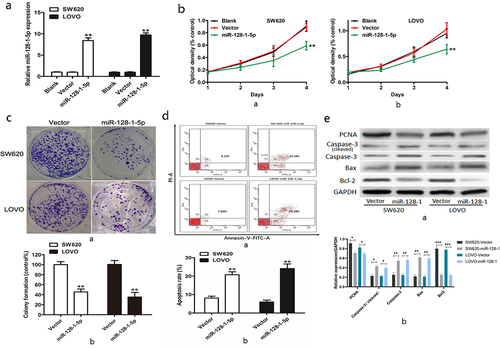
PRKCQ is a direct target of miR-128-1-5p
To investigate the mechanism of miR-128-1-5p in CRC, we used online algorithm to search potential targets of miR-128-1-5p, including TargetScan, DIANA, miRDB, and microRNA, and Venn diagrams was required to explore the common genes (). Specifically, we found the 3’-UTR of PRKCQ had binding site of miR-128-1-5p (). To confirm whether PRKCQ was a direct target of miR-128-1-5p in CRC cells, luciferase reporter assay was performed to assess luciferase activities and the results showed that the activity of PRKCQ 3’-UTR-WT was obviously reduced after transfection with miR-128-1-5p (). However, there was no significant difference in activity of PRKCQ 3’-UTR-MUT (). Furthermore, miR-128-1-5p overexpression markedly suppressed the mRNA and protein expression of PRKCQ in CRC cells (). Moreover, the qRT-PCR results showed that the level of PRKCQ was significantly upregulated in CRC cell lines than in the intestinal epithelial cell line HIEC (). The level of PRKCQ in CRC tissues was also higher compared with their respective adjacent normal tissues (). Pearson correlation assay suggested that miR-128-1-5p expression was negatively correlated with the mRNA level of PRKCQ (). As shown in , PRKCQ levels were correlated with AJCC (p = .006), distant metastasis (p = .0167), tumor size (p = .0187), vascular invasion (p = .0158), histopathological grade (p = .0158), and lymph metastasis (p = .0239). Survival analysis using the Kaplan-Meier also revealed that higher PRKCQ levels were associated with shorter overall survival in CRC patients (HR = 2.998, p = .0008, ). Taken together, these data demonstrated that PRKCQ was a direct target of miR-128-1-5p, acting as a tumor promoter in CRC.
Figure 3. The target of miR-128-1-5p in CRC.
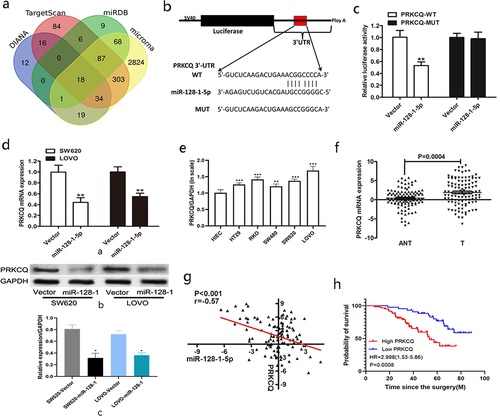
Table 2. Relationship between clinicopathological factors and PRKCQ expression in CRC tissues.
MiR-128-1-5p modulates CRC cells proliferation and apoptosis by targeting PRKCQ
To investigate whether miR-128-1-5p may inhibit cell growth by silencing the PRKCQ expression in CRC cells, we assessed the effect of PRKCQ on cell proliferation by transfecting PRKCQ in miR-128-1-5p overexpression SW620 and LOVO cells. Western blotting showed that the expression of PRKCQ was notably increased by transfecting PRKCQ in miR-128-1-5p overexpression SW620 and LOVO cells (). Interestingly, overexpression of PRKCQ significantly reversed the inhibition of cell growth induced by miR-128-1-5p (). The colony formation assays also demonstrated that upregulating PRKCQ in miR-128-1-5p overexpression cells increased colony numbers comparing control groups (). The results of the cell apoptosis assays suggested that overexpression of PRKCQ abolished the increased cell apoptosis resulting from miR-128-1-5p (). Furthermore, the levels of caspase-3 and Bax were upregulated together with Bcl-2 downregulated by PRKCQ overexpression (). Collectively these findings clearly described that PRKCQ is a mediator of miR-128-1-5p in progression of CRC.
Figure 4. PRKCQ is downstream of the miR-128-1-5p signaling.
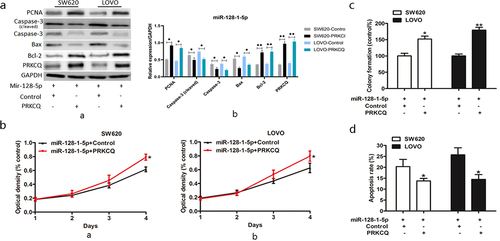
In vivo effect of miR-128-1-5p on CRC
To further investigate whether miR-128-1-5p affect CRC growth in vivo, LOVO cells stably overexpressing miR-128-1-5p were subcutaneously injected into nude mice to examine the effects of miR-128-1-5p on tumor growth in vivo. As shown in , overexpression of miR-128-1-5p could suppress the tumor growth, demonstrated by decreased mean tumor volume and weight (). Furthermore, the expression of miR-128-1-5p was significantly increased in the xenograft tumor tissues (), whereas the mRNA expression of PRKCQ was significantly decreased in the xenograft tumor tissues (). In addition, the immunohistochemical staining showed that the protein expression of Ki-67, Bcl-2 and PRKCQ was significantly downregulated together with caspase-3 upregulated in the tumor tissues of miR-128-1-5p overexpression group (). Taken together, our results supported the notion that miR-128-1-5p inhibited CRC cell growth via regulating PRKCQ.
Figure 5. The effect of miR-128-1-5p on CRC growth in vivo.
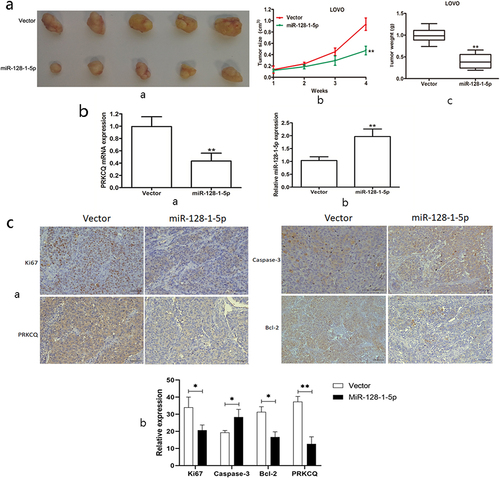
Discussion
CRC is one of the most aggressive malignancies with the third leading cause of cancer-related deaths in United StatesCitation18. Current management strategies for CRC treatment mainly include curative surgery and post-operative adjuvant chemotherapyCitation19–21. Further studies of the underlying mechanisms of CRC initiation and progression are required to reduce the mortality caused by this cancer. In the present study, we screened for miRNA associated with prognosis in CRC, and the expression of miR-128-1-5p was strongly associated with optimal survival. Therefore, we attempted to explore the expression and role of miR-128-1-5p in CRC.
Previous studies have shown that numerous miRNAs were deregulated in CRC, and correlate with CRC pathological stage and prognosisCitation22–24. MiR-128-1-5p has been identified as a tumor suppressor and found to be downregulated in certain malignancies. MiR-128 has been reported to be down-regulated in thyroid carcinoma and overexpression of miR-128 suppressed thyroid carcinoma cancer cell growth, migration and invasion by negatively regulating SPHK1 expressionCitation12. MiR-128 was also found to be downregulated in glioma tissues and cells and overexpression of miR-128 could inhibit LN229 and U251 cells proliferation and invasionCitation25. A recent study has shown that miR-128 might act as a tumor suppressor in CRCCitation26. Consisted with these results, our data showed that miR-128-1-5p was significantly downregulated in CRC tissues and cells and low miR-128 expression was associated with advance AJCC stage and distant metastasis and indicated a poorer prognosis. CCK-8 and colony formation assay showed that overexpression of miR-128-1-5p significantly inhibited the proliferation of CRC cells. To reveal the potential mechanisms of how miR-128-1-5p affected cell proliferation, cell apoptosis was detected and cytometry analysis showed that miR-128-1-5p induced cell apoptosis with significantly alteration of Bcl-2, Bax and Caspase-3.
MiRNAs regulate gene expression by binding to partially complementary sequences of 3’ untranslated region (3’UTR) of target genes to suppress mRNA translationCitation8. To identify potential miR-128-1-5p targets in CRC cells, we screened predicted targets by online target tools and forecasted as upregulated in CRC tissues according to literature. The potential candidate PRKCQ, a member of the PKC familyCitation27,Citation28, was regarded as a direct target of miR-128-1-5p. Luciferase reporter activity confirmed that PRKCQ was directly targeted by miR-128-1-5p. A negative correlation between miR-128-1-5p and PRKCQ mRNA levels was observed in CRC tissues. Interestingly, restoration of PRKCQ expression could significantly reverse the effects of miR-128-1-5p overexpression on cell viability and apoptosis, suggesting that miR-128-1-5p functions through lowering the expression of PRKCQ. PRKCQ was involved in the various diseases, especially autoimmune disorders and cancers, and the mechanisms were involving immune cells activation, and cancer cell proliferation, migration, and differentiationCitation16. Indeed, PRKCQ can activate transcription factors in both tumor cells and T cells, but with the implication of different AP-1 and NF-κB subunitsCitation29. ByerlyCitation30 et al reported that enhanced PRKCQ expression promoted growth-factor-independent growth, anoikis resistance, and migration, and inhibition of PRKCQ kinase activity may be an attractive therapeutic approach. In this study, we revealed significantly higher expression of PRKCQ in CRC, and proved higher level of PRKCQ closely correlated with malignant clinical phenotype and poor survival. Collectively, these data demonstrated that PRKCQ was a direct target of miR-128-1-5p, acting as a tumor promoter in CRC.
In the present study, we identified that miR-128-1-5p was frequently down-regulated in human CRC tissues and cells and functioned as a suppressor gene to inhibit cell proliferation in vitro and in vivo. Mechanistically, miR-128-1-5p suppressed the cell proliferation by directly inhibiting PRKCQ expression. Our study might provide novel insights into understanding the molecular pathogenesis of CRC and offer a potential target for the diagnosis and therapy of patients with CRC.
Material and methods
Bioinformatic analysis
CancerMIRNome (http://bioinfo.jialab-ucr.org/CancerMIRNome/) is a comprehensive database for facilitating the use of publicly available cancer miRNome data to assist in miRNA research in 33 cancer types from The Cancer Genome Atlas (TCGA), and 40 public cancer circulating miRNome profiling datasets from NCBI Gene Expression Omnibus (GEO) and ArrayExpressCitation31.
CancerMIRNome provides a user-friendly interface and a suite of advanced functions, and we focused the different expression between tumor and normal samples, ROC analysis, and KM survival analysis for miR-128-1-5p in COAD and READ by this database. Expression of miR-128-1-5p in serum in both healthy and different cancer types also explored in CancerMIRNome. Online algorithms were used to search potential targets of miR-128-1-5p, including TargetScan (http://www.targetscan.org/), DIANA (https://dianalab.e-ce.uth.gr/mited/), miRDB (http://mirdb.org/), and microRNA (http://www.microrna.org/), and Venn diagrams was required to explore the common genes.
Human tissue samples
107 human CRC and their adjacent normal tissues were obtained from patients who received surgery in the Department of Colorectal Surgery between January 2006 and October 2012. All patients did not receive anticancer treatments before operation and written informed consent was obtained from all patients enrolled in this study. The study protocol was reviewed and approved by the Institutional Human Experiment and Ethic Committee of Fudan University Cancer Hospital. After collection, the fresh tissues were immediately frozen in liquid nitrogen for RNA isolation.
Cell lines and cell culture
The human CRC cell lines (HT29, RKO, SW480, SW620 and LOVO) and the normal human intestinal epithelial cell line (HIEC) were purchased from the Type Culture Collection of the Chinese Academy of Sciences (Shanghai, China) and cultured as described previouslyCitation32.
RNA extraction and real-time PCR analysis
Total RNA was extracted using Trizol reagent (Invitrogen, Thermo Fisher Scientific, Inc., Carlsbad, CA) according to the manufacturer’s instructions. All RNA was reverse-transcribed into cDNA according to the manufacturer’s instructions with the PrimScript RT reagent Kit (Takara Biotechnology Co. Ltd., Beijing, China). qRT-PCR was carried out as described previouslyCitation32. The primers were obtained from Sangon (Shanghai, China), the primers sequences were as follows: miR-128-1-5p (NR_029672.1): forward, 5ʹ-CCACAGAGAGGATTCCAGAA-3ʹ, reverse, 5ʹ-TCCAGGCTCTGTATAGAAGCT −3ʹ; U6 RNA (NR_004394.1): forward, 5ʹ-CTCGCTTCGGCAGCACA-3ʹ, reverse, 5ʹ-AACGCTTCACGAATTTGCGT-3ʹ; PRKCQ (NM_001242413.2): forward, 5ʹ-ATGTCGCCATTTCTTCGGATT-3ʹ, reverse, 5ʹ-ACATACTCTTTGACGAGCACAG −3ʹ; GAPDH (NM_001256799.3): forward, 5ʹ-AGCCTTCTCCATGGTGGTGAA-3ʹ, reverse, 5ʹ-ATCACCATCTTCCAGGAGCGA −3ʹ.
Lentivirus production and transduction
Lentivirus-medicated miR-128-1-5p overexpression vector was purchased from GenePharma (Shanghai, China). Lentivirus was produced by transfecting 293T cell linewith Lenti-Pac HIV Expression Packaging Mix and the lentiviral vector using Lipofectamine 2000 (Life Technologies Corporation, Carlsbad, CA, USA). Stably transfected cell lines were established by infecting SW620 and LOVO cells with lentiviruses containing 2 μg/mL puromycin. The full-length sequence of PRKCQ was cloned into the pcDNA3.1 vector and empty vector was used as negative control simultaneously.
Cell proliferation and colony formation assay
The CCK-8 assay was performed as our previous studyCitation32. In brief, 2 × 10Citation3 cells were cultured in a 96-well plate and cultured for 24-48-72-96 h. Then 10 μL CCK-8 solution was added to each well and incubated for 2 h at 37°C. Finally, the absorbance was measured at 450 nm. For colony formation assay, 8 × 10Citation2 cells were seeded in 6-well plates and cultured for 2 weeks at 37°C. Cell colonies were fixed with methanol and stained with 0.5% crystal violet. Colonies containing more than 50 cells were counted and photographed.
Flow cytometric analysis
Cells were dissociated with trypsin without EDTA, subsequently incubated with Annexin V- DY-634 PI Apoptosis Staining/Detection Kit (Abcam, Cambridge, United Kingdom). The ratio of apoptotic cells was detected with a flow cytometry (FACScan, BD Biosciences).
Vector constructs and luciferase reporter assay
The full-length of wild type 3’UTR of PRKCQ was cloned into the psi-CHECK2 vector (Promega, Madison, WI, USA) to form the reporter vector PRKCQ-wild-type (PRKCQ-WT). The corresponding mutant was created by mutating the miR-128-1-5p seed region binding site, which was named PRKCQ-MUT. All clones were verified by DNA sequencing. MiR-128-1-5p or vector was co-transfected with luciferase reporter vectors into 293T cells using LipofectamineTM 2000.Cells were harvested 36 h post-transfection and luciferase activities were analyzed by the dual-luciferase reporter assay system (Promega) in a luminescence reporter gene assay system (PerkinElmer, Norwalk, CT, USA) according to the manufacturer’s instructions.
Protein isolation and western blotting
Total protein was extracted using RIPA lysis buffer from Cell Signaling Technology (CST, Beverly, MA, USA) according to the manufacturer’s protocol. Western blotting was performed using standard protocols as described previouslyCitation32. The primary antibodies used in this study included: PRKCQ (Proteintech Group, Inc, IL, USA), Caspase-3 (CST), Bax (CST), Bcl-2 (CST), and GAPDH (Proteintech Group, Inc).
Xenograft model
4–6-week-old female immune-deficient nude mice (BALB/c null) were purchased from Slaccas (Slaccas Laboratory Animal, Shanghai, China). All animal procedures were performed in accordance with guidelines of the care and use of laboratory animals of Fudan University and approved by the Animal Ethics Committee of Zhongshan hospital. Stable miR-128-1-5p-overexpressing LOVO and control cells (1 × 10Citation7 cells) in 100 ul serum free medium were subcutaneously injected into the flank region of the mice (8 for one group). Tumor size was measured with a slide caliper every week. Mice were sacrificed 4 weeks later and the tumors were removed for further investigation.
Immunohistochemistry staining
Immunohistochemistry (IHC) staining was carried out as our previously described (23). Briefly, formalin-fixed tissues were embedded in paraffin and cut into 4-μm-thick sections. Subsequently, the specimens were dewaxed, hydrated, antigen retrieved by heat, blocked, and then incubated overnight at 4°C with primary antibody Ki67 (CST). Following, sections were incubated with HRP conjugated secondary antibodies. Finally, slides were treated with 3, 3’-diaminobenzidine (DAB) substrate and visualized under a Nikon Eclipse Ti inverted microscope (Nikon, Amstelveen, The Netherlands).
Statistical analysis
The results were presented as the mean ± standard error of the mean (SEM). Student’s t-test was used to assess differences between two groups. One-way analysis of variance (ANOVA) was used to compare differences among three groups or more. Association between miR-128-1-5p and PRKCQ expression was assessed using Spearman rank correlation test. The χ2 test was performed to analyze the relationship between miR-128-1-5p expression and the clinicopathological characteristics. Statistical analysis used SPSS 18.0 (SPSS, Chicago, USA) and a P < .05 was considered statistically significant.
Availability of data and materials
All the data generated or analyzed during this study are included in this published article.
Disclosure statement
No potential conflict of interest was reported by the author(s).
Additional information
Funding
Notes on contributors
Qingan Jia
Qingan Jia, obtained his PhD from Shanghai Medical College of Fudan University. Now he is an associate professor in Northwestern Polytechnical University. His research focuses on the mechanisms and treatment of cancer.
Xia Liao
Xia Liao, obtained her BS and Master degree from Xi’an Jiaotong University. Now she is an associate chief physician in The First Affiliated Hospital of Xi'an Jiao Tong University. Her research focuses on Nutritional intervention in cancer.
Binghui Xu
Binghui Xu, received his BS degree from Northwestern Polytechnical University in 2020. Now, he is a master student in Northwestern Polytechnical University. His research focuses on the pathology and treatment of cancer.
Yufang Li
Yufang Li, received her BS degree and MS degree from Air Force Medical University. Now, she is a PhD student in Northwestern Polytechnical University. Her research focuses on the pathology and treatment of cancer.
Lei Liang
Lei Liang, obtained his PhD from Shanghai Medical College of Fudan University. Now he is an associate chief physician in Shanghai Cancer Center. His research focus on surgery and comprehensive treatment of colon cancer.
References
- Kanth P, Inadomi JM. Screening and prevention of colorectal cancer. BMJ. 2021;374:n1855. doi:10.1136/bmj.n1855.
- Bray F, Ferlay J, Soerjomataram I, Siegel RL, Torre LA, Jemal A. Global cancer statistics 2018: gLOBOCAN estimates of incidence and mortality worldwide for 36 cancers in 185 countries. CA Cancer J Clin. 2018;68(6):394–10. doi:10.3322/caac.21492.
- Siegel RL, Miller KD, Goding Sauer A, Fedewa SA, Butterly LF, Anderson JC, Cercek A, Smith RA, Jemal A. Colorectal cancer statistics, 2020. CA Cancer J Clin. 2020;70(3):145–164. doi:10.3322/caac.21601.
- Rodel C, Liersch T, Becker H, Fietkau R, Hohenberger W, Hothorn T, Graeven U, Arnold D, Lang-Welzenbach M, Raab H-R, et al. Preoperative chemoradiotherapy and postoperative chemotherapy with fluorouracil and oxaliplatin versus fluorouracil alone in locally advanced rectal cancer: initial results of the German CAO/ARO/AIO-04 randomised phase 3 trial. Lancet Oncol. 2012;13(7):679–687. doi:10.1016/S1470-2045(12)70187-0.
- Wang Y, Wang L, Chen C, Chu X. New insights into the regulatory role of microRNA in tumor angiogenesis and clinical implications. Mol Cancer. 2018;17(1):22. doi:10.1186/s12943-018-0766-4.
- Jung G, Hernández-Illán E, Moreira L, Balaguer F, Goel A. Epigenetics of colorectal cancer: biomarker and therapeutic potential. Nat Rev Gastro Hepat. 2020;17(2):111–130. doi:10.1038/s41575-019-0230-y.
- Ali Syeda Z, Langden SSS, Munkhzul C, Lee M, Song SJ. Regulatory mechanism of MicroRNA expression in Cancer. IJMS. 2020;21(5):21. doi:10.3390/ijms21051723.
- Bartel DP. MicroRNAs: target recognition and regulatory functions. Cell. 2009;136(2):215–233. doi:10.1016/j.cell.2009.01.002.
- Guo Y, Guo Y, Chen C, Fan D, Wu X, Zhao L, Shao B, Sun Z, Ji Z. Circ3823 contributes to growth, metastasis and angiogenesis of colorectal cancer: involvement of miR-30c-5p/TCF7 axis. Mol Cancer. 2021;20(1):93. doi:10.1186/s12943-021-01372-0.
- Zhu Q, Zhang Q, Gu M, Zhang K, Xia T, Zhang S, Chen W, Yin H, Yao H, Fan Y, et al. Mir106A-5p upregulation suppresses autophagy and accelerates malignant phenotype in nasopharyngeal carcinoma. Autophagy. 2021;17(7):1667–1683. doi:10.1080/15548627.2020.1781368.
- Cao MQ, You AB, Zhu XD, Zhang W, Zhang YY, Zhang SZ, Zhang K-W, Cai H, Shi W-K, Li X-L, et al. MiR-182-5p promotes hepatocellular carcinoma progression by repressing FOXO3a. J Hematol Oncol. 2018;11(1):12. doi:10.1186/s13045-018-0555-y.
- Cao XZ, Bin H, Zang ZN. MiR-128 suppresses the growth of thyroid carcinoma by negatively regulating SPHK1. Biomed Pharmacother. 2019;109:1960–1966. doi:10.1016/j.biopha.2018.08.052.
- Xiao M, Lou C, Xiao H, Yang Y, Cai X, Li C, Jia S, Huang Y. MiR-128 regulation of glucose metabolism and cell proliferation in triple-negative breast cancer. Br J Surg. 2018;105(1):75–85. doi:10.1002/bjs.10646.
- Cai J, Fang L, Huang Y, Li R, Xu X, Hu Z, Zhang L, Yang Y, Zhu X, Zhang H, et al. Simultaneous overactivation of Wnt/β-catenin and TGFβ signalling by miR-128-3p confers chemoresistance-associated metastasis in NSCLC. Nat Commun. 2017;8(1):15870. doi:10.1038/ncomms15870.
- Liu T, Zhang X, Du L, Wang Y, Liu X, Tian H, Wang L, Li P, Zhao Y, Duan W, et al. RETRACTED ARTICLE: exosome-transmitted miR-128-3p increase chemosensitivity of oxaliplatin-resistant colorectal cancer. Mol Cancer. 2019;18(1):43. doi:10.1186/s12943-019-0981-7.
- Nicolle A, Zhang Y, Belguise K. The emerging function of PKCtheta in Cancer. Biomolecules. 2021;11(2):11. doi:10.3390/biom11020221.
- Byerly JH, Port ER, Irie HY. PRKCQ inhibition enhances chemosensitivity of triple-negative breast cancer by regulating Bim. Breast Cancer Res. 2020;22(1):72. doi:10.1186/s13058-020-01302-w.
- Siegel RL, Miller KD, Fuchs HE, Jemal A. Cancer statistics, 2022. CA Cancer J Clin. 2022;72(1):7–33. doi:10.3322/caac.21708.
- Dekker E, Tanis PJ, Vleugels JLA, Kasi PM, Wallace MB. Colorectal cancer. Lancet. 2019;394(10207):1467–1480. doi:10.1016/S0140-6736(19)32319-0.
- Stein A, Atanackovic D, Bokemeyer C. Current standards and new trends in the primary treatment of colorectal cancer. Eur J Cancer. 2011;47(Suppl 3):S312–4. doi:10.1016/S0959-8049(11)70183-6.
- Loupakis F, Cremolini C, Masi G, Lonardi S, Zagonel V, Salvatore L, Cortesi E, Tomasello G, Ronzoni M, Spadi R, et al. Initial therapy with FOLFOXIRI and bevacizumab for metastatic colorectal cancer. N Engl J Med. 2014;371(17):1609–1618. doi:10.1056/NEJMoa1403108.
- Zhang N, Hu X, Du Y, Du J. The role of miRnas in colorectal cancer progression and chemoradiotherapy. Biomed Pharmacother. 2021;134:111099. doi:10.1016/j.biopha.2020.111099.
- Saberinia A, Alinezhad A, Jafari F, Soltany S, Akhavan Sigari R. Oncogenic miRnas and target therapies in colorectal cancer. Clin Chim Acta. 2020;508:77–91. doi:10.1016/j.cca.2020.05.012.
- Balacescu O, Sur D, Cainap C, Visan S, Cruceriu D, Manzat-Saplacan R, Muresan M-S, Balacescu L, Lisencu C, Irimie A, et al. The Impact of miRNA in colorectal cancer progression and its liver metastases. Int J Mol Sci. 2018;19(12):3711. doi:10.3390/ijms19123711.
- Lin Y, Wu Z. MicroRNA-128 inhibits proliferation and invasion of glioma cells by targeting COX-2. Gene. 2018;658:63–69. doi:10.1016/j.gene.2018.03.020.
- Chang A-C, Lien M-Y, Tsai M-H, Hua C-H, Tang C-H. WISP-1 Promotes epithelial-mesenchymal transition in oral squamous cell carcinoma cells via the miR-153-3p/Snail axis. Cancers. 2019;11(12):11. doi:10.3390/cancers11121903.
- Zafar A, Wu F, Hardy K, Li J, Tu WJ, McCuaig R, Harris J, Khanna KK, Attema J, Gregory PA, et al. Chromatinized protein kinase C-θ directly regulates inducible genes in epithelial to mesenchymal transition and breast cancer stem cells. Mol Cell Biol. 2014;34(16):2961–2980. doi:10.1128/MCB.01693-13.
- Belguise K, Milord S, Galtier F, Moquet-Torcy G, Piechaczyk M, Chalbos D. The PKCθ pathway participates in the aberrant accumulation of Fra-1 protein in invasive ER-negative breast cancer cells. Oncogene. 2012;31(47):4889–4897. doi:10.1038/onc.2011.659.
- Hage-Sleiman R, Hamze AB, Reslan L, Kobeissy H, Dbaibo G. The novel PKCtheta from Benchtop to clinic. J Immunol Res. 2015;2015:348798. doi:10.1155/2015/348798.
- Byerly J, Halstead-Nussloch G, Ito K, Katsyv I, Irie HY. PRKCQ promotes oncogenic growth and anoikis resistance of a subset of triple-negative breast cancer cells. Breast Cancer Res. 2016;18(1):95. doi:10.1186/s13058-016-0749-6.
- Li R, Qu H, Wang S, Chater JM, Wang X, Cui Y, Yu L, Zhou R, Jia Q, Traband R, et al. CancerMIRNome: an interactive analysis and visualization database for miRnome profiles of human cancer. Nucleic Acids Research. 2022;50(D1):D1139–D1146. doi:10.1093/nar/gkab784.
- Li X, Liang L, Huang L, Ma X, Li D, Cai S. High expression of protein phosphatase 4 is associated with the aggressive malignant behavior of colorectal carcinoma. Mol Cancer. 2015;14(1):95. doi:10.1186/s12943-015-0356-7.