ABSTRACT
Cancer cells show enhanced nucleotide biosynthesis, which is essential for their unlimited proliferation, but the underlying mechanisms are not entirely clear. Ubiquitin specific peptidase 29 (USP29) was reported to sustain neuroblastoma progression by promoting glycolysis and glutamine catabolism; however, its potential role in regulating nucleotide biosynthesis in tumor cells remains unknown. In this study, we depleted endogenous USP29 in MYCN-amplified neuroblastoma SK-N-BE2 cells by sgRNAs and conducted metabolomic analysis in cells with or without USP29 depletion, we found that USP29 deficiency caused a disorder of intermediates involved in glycolysis and nucleotide biosynthesis. De novo nucleotide biosynthesis was analyzed using 13C6 glucose as a tracer under normoxia and hypoxia. The results indicated that USP29-depleted cells showed inhibition of nucleotide anabolic intermediates derived from glucose, and this inhibition was more significant under hypoxic conditions. Analysis of RNA sequencing data in SK-N-BE2 cells demonstrated that USP29 promoted the gene expression of metabolic enzymes involved in nucleotide anabolism, probably by regulating MYC and E2F downstream pathways. These findings indicated that USP29 is a key regulator of nucleotide biosynthesis in tumor cells.
Introduction
Nucleotides are essential to many cellular processes including DNA replication, gene transcription, and ribosome biogenesis, although the uptake of nucleotides from extracellular sources is negligible.Citation1 In mammalian cells, nucleotides are synthesized through either the de novo pathway or the salvage pathway. In the de novo pathway, which acts as the main pathway, the cells utilize glucose and amino acids to synthesize both purine and pyrimidine, with glucose and amino acids providing carbon and nitrogen respectively; for the salvage pathway, the nucleoside backbones and free bases derived from the hydrolysis of nucleic acids are recycled for nucleic acid synthesis.Citation2,Citation3 It has been well characterized that cancer cells often showed enhanced nucleotide biosynthesis, thereby sustaining unlimited cell proliferation.Citation2 Cancer cell nucleotide synthesis is regulated by several critical transcription factors, MYC and Rb/E2F in particular, which promote nucleotide synthesis by inducing a series of genes that encode the enzymes in the nucleotide biosynthetic pathways.Citation4,Citation5
Neuroblastoma, the most common extracranial solid tumor of childhood, is thought to originate from undifferentiated neural crest cells.Citation6,Citation7 Several genetic alterations have been shown in neuroblastoma, including amplification of MYCN, a member of the oncogenic MYC family, which is found in approximately 20% of cases and correlates with high-risk disease and poor patient survival; to date, it remains the best-characterized genetic marker of high-risk neuroblastoma.Citation6,Citation7 Although the outcome for patients with high‑risk neuroblastoma has improved, the 5-year overall survival probability for these patients is only approximately 50%.Citation8
USP29 has been reported to play an emerging role in several cancers. In our previous study, we revealed that USP29 promoted tumor cell aerobic glycolysis and glutamine anaplerosis by deubiquitinating and stabilizing the oncogenic MYC family and HIF1α, and systemic knockout of USP29 significantly suppressed tumor progression and prolonged the survival of tumor-bearing mice.Citation9 Consistently, USP29 was also reported to sustain glycolysis and sorafenib resistance in hepatocellular carcinoma by stabilizing HIF1α, indicating the important role of USP29 in regulating tumor cell metabolic reprogramming in various types of cancer.Citation10 Moreover, USP29 was reported to stabilize p53,Citation11 Claspin,Citation12 Cdc25A,Citation13 VIRMACitation14 and Snail1Citation15,Citation16 in tumor cells. However, the potential role of USP29 in regulating nucleotide metabolism remains unclear.
In this study, through an integrated analysis of metabolomics, metabolic flux, and transcriptomics data in neuroblastoma SK-N-BE2 cells with or without USP29 depletion, we revealed that USP29 plays a key role in promoting tumor cell nucleotide synthesis potentially by regulating the MYC and E2F pathways.
Methods
Cell culture
Neuroblastoma SK-N-BE2, BE-2C and SK-N-AS cells were kindly provided by Professor Guoliang Qing (Medical Research Institute, Wuhan University, Wuhan, China), and 293T cells were purchased from Procell Company (Wuhan, China). Neuroblastoma cells were cultured in RPMI-1640 (HyClone) medium with 10% FBS (Gibco) and 1% penicillin/streptomycin (HyClone), and 293T cells were maintained in DMEM (HyClone) containing 10% FBS and 1% penicillin/streptomycin.
Lentiviral transduction
The lentiCRISPRv2 was used for plasmid construction and transfected into 293T cells together with the helper plasmids pMD2.G and psPAX2 using Lipo2000 (Thermo Fisher Scientific). Viral supernatant was collected at 48 h post transfection and filtered using a 0.22 μm sterile membrane. For infection of SK-N-BE2 cells, cells grown to 60–80% confluence were incubated with an appropriate amount of viral supernatant and 4 μg/mL polybrene in a final volume of 2 mL for 24 h. The infected cells were selected with 2 μg/mL puromycin for at least 36 h before additional experiments were performed. The USP29 sgRNA sequence was 5’-TTGAGCAGTAGTGCACCTGT-3’, and the control sgRNA was 5’-GGGCGAGGAGCTGTTCACCG-3’.
Immunoblot
Cells were collected using PBS, and total proteins were extracted with lysis buffer (50 mM Tris-HCl, pH 7.4, 150 mM NaCl, 1% NP-40, 1% SDS, 1 mM EDTA). Proteins were separated by SDS‒PAGE and transferred to PVDF membranes (Bio-Rad). After blocking with 5% nonfat dry milk in TBST, membranes were incubated with the primary antibody overnight at 4°C and then a horseradish peroxidase-conjugated secondary antibody. Protein bands were visualized using the SuperSignal chemiluminescence kit (Merck Millipore) and the Bio-Rad ChemiDoc XRS+ imaging system. The antibodies used in this study were actin (ABclonal, AC026) and USP29 antibodies (ABGENT, AP2153C).
Metabolomic analysis
The metabolomic analysis was performed as previously described.Citation17 In brief, ten million SK-N-BE2 cells with or without USP29 knockout were collected and quenched in liquid nitrogen. Metabolite samples were prepared for analysis using standard solvent extraction methods and then subjected to the gas chromatograph system coupled with a Pegasus HT time-of-flight mass spectrometer. The resulting three-dimensional data involving the peak number, sample name, and normalized peak area were fed to the SIMCA14.1 software package (V14.1, Sartorius Stedim Data Analytics AB, Umea, Sweden) for principal component analysis (PCA) and orthogonal projections to latent structures-discriminate analysis (OPLS-DA). PCA showed the distribution of the original data. The first principal component of variable importance in the projection (VIP) was obtained to refine this analysis. VIP values exceeding 1 were first selected as changed metabolites. In step 2, the remaining variables were then assessed by Student’s t test (P value > 0.05), and variables were discarded between two comparison groups.
Stable isotope tracing
13C6-glucose (Cat#110187-42-3) was purchased from the Cambridge Isotope Laboratories. SK-N-BE2 cells were cultured under normoxia or hypoxia (1% O2) for 12 h before the medium was replaced with RPMI-1640 containing the labeled nutrients. Media were replaced with glucose-free RPMI-1640 (Gibco) with 2 mg/mL 13C6-glucose and 10% FBS for 1 or 24 h after cells were washed twice with PBS; for hypoxic tracing, the labeled medium was pretreated in hypoxia for 3 h. Cellular metabolite extraction was performed as described previously.Citation9 Briefly, cells were treated with 80% methanol (prechilled to −80°C) and centrifuged (14,000×g) at 4°C for 15 min. The supernatant was then collected and evaporated. The metabolite analysis was conducted at the Metabolomics Facility Center in the National Protein Science Technology Center of Tsinghua University.
RNA sequencing and gene set enrichment analysis
RNA sequencing was performed as previously described,Citation9 and RNA-Seq data in SK-N-BE2 cells have been deposited in Gene Expression Omnibus with the accession code GSE180797. Gene set enrichment analysis (GSEA) was used to identify pathways and gene clusters.Citation18
RNA isolation and qRT‒PCR
RNA isolation and qRT‒PCR were performed as previously described.Citation9 Briefly, total cellular RNA was extracted with TRIzol reagent (Vazyme, Nanjing, China) according to the manufacturer’s protocol, complementary DNA was prepared using a Strand cDNA Synthesis Kit (+gDNA wiper) (Vazyme, Nanjing, China), and quantitative PCR was conducted using FAST SYBR Green Master Mix (Vazyme, Nanjing, China) on a CFX Connect Real-Time PCR System (Bio-Rad). Relative expression of the mRNA was calculated by the 2−ΔΔCt method and normalized to actin expression using specific primers as shown in Supplementary Table S1.
Statistical analysis
Data analysis was carried out using GraphPad Prism 6. Statistical significance was calculated by unpaired two-tailed Student’s t test between two groups or by one-way ANOVA with Tukey’s corrections when comparing three or more groups. Differences were considered significant when p < 0.05.
Results
Metabolomic analysis of USP29-depleted SK-N-BE2 cells
To explore the effects of USP29 on tumor metabolism at the global level, we depleted endogenous USP29 in neuroblastoma SK-N-BE2 cells by validated sgRNA (Supplementary Figure S1a). The total metabolites were extracted from cells with or without USP29 depletion and subjected to metabolomics analysis. As shown in , 56 up-regulated metabolites and 22 down-regulated metabolites were found, indicating that USP29 deficiency may cause a major effect on cellular metabolite accumulation. In a previous study, we reported that the depletion of USP29 in tumor cells significantly inhibited glucose consumption and lactate production.Citation9 Interestingly, the metabolomics data showed that representative glycolytic intermediates, including glucose (Glc), fructose 6 phosphate (F6P), pyruvate (Pyr) and lactate (Lac), were markedly accumulated along with USP29 deficiency (). Although the underlying mechanisms are not clear, these results may explain why USP29-depleted tumor cells show less glucose absorption and lactate secretion.
Figure 1. Metabolomic analysis of USP29-depleted SK-N-BE2 cells.
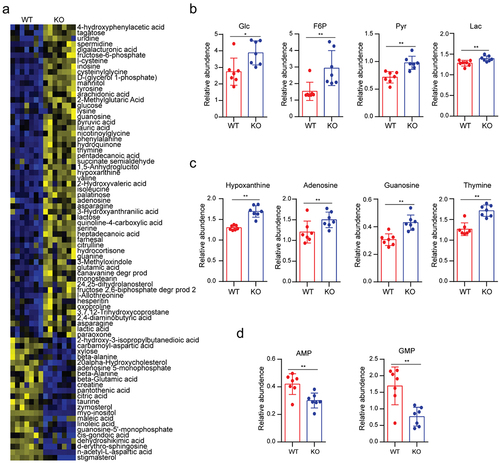
In addition to the alteration of glycolytic intermediates, there was a significant increase in intracellular purine and pyrimidine bases, including hypoxanthine, adenosine, guanosine, and thymine (), which are essential precursors of nucleotide synthesis,Citation19 indicating that the cellular nucleotide synthesis might be compromised. Consistent with this hypothesis, the downstream metabolites adenosine 5-monophosphate (AMP) and guanosine 5-monophosphate (GMP) were down-regulated (). These findings suggested that USP29 may play an important role in regulating cancer cell metabolism, especially in nucleotide biosynthesis.
USP29 deficiency decreases nucleotide biosynthesis
In addition to the use of glucose as an energy source for ATP generation, cancer cells display enhanced uptake of glucose, a major nutrient of mammalian cells, providing an important source of carbon to support the biosynthesis of nucleotides (), lipid production and non-essential amino acids (NEAAs).Citation1,Citation20 We then assessed nucleotide synthesis flux in SK-N-BE2 cells using 13C6 glucose (all six carbons are 13C-labeled, M6) as the tracer. Inosine monophosphate (IMP) is an important metabolic intermediate of purine synthesis, and we observed elevated M5 IMP enrichment when the cells were labeled for 1 h but decreased enrichment for cells labeled for 24 h (). Despite this, USP29 depletion significantly reduced the enrichment of M5 purine intermediates, including AMP, ADP/dGDP, ATP, GDP and GTP, either labeled for 1 or 24 h (). As expected, the enrichment of representative M5 pyrimidine intermediates (including UMP, UDP and UTP) was down-regulated (). These findings suggested that USP29 may play a positive role in de novo nucleotide synthesis.
Figure 2. USP29 deficiency decreases nucleotide biosynthesis.
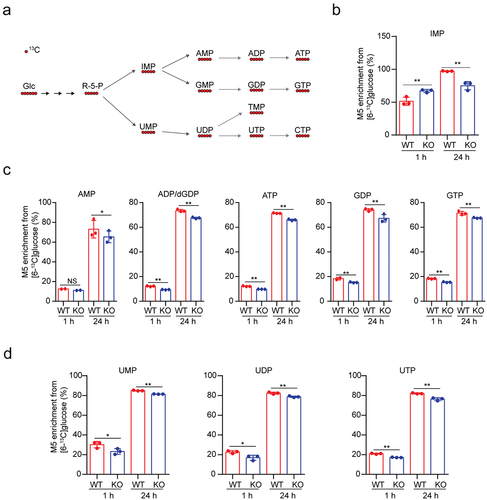
USP29 promotes nucleotide biosynthesis under hypoxia
One of the best-known reasons for altered tumor metabolism is the unique hypoxic stresses that exist within the tumor, and perhaps the most important aspect of how cells respond to this unique microenvironment is the activity of the HIF1α transcription factor.Citation21 USP29 was reported to promote tumor cell glycolysis in normoxia or hypoxia by stabilizing HIF1α,Citation9,Citation10 and thus, we proposed that USP29 may also regulate de novo nucleotide synthesis under hypoxia.
To assess this hypothesis, we depleted endogenous USP29 in SK-N-BE2 cells and then analyzed nucleotide synthesis in cells cultured under hypoxic conditions (1% O2) using 13C6 glucose as a tracer. As expected, we observed a decreased enrichment of 13C6 glucose-derived M5 purine and pyrimidine intermediates (); interestingly, these down-regulations seemed to be more significant when compared to normoxia (). These results showed that USP29 may sustain de novo nucleotide biosynthesis under hypoxia.
Figure 3. USP29 deficiency decreases nucleotide biosynthesis under hypoxia
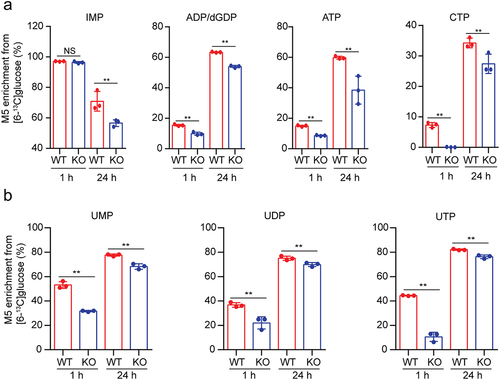
USP29 regulates nucleotide biosynthesis by promoting the MYC and E2F pathways
To explore the potential mechanisms underlying USP29 regulation of nucleotide biosynthesis, we analyzed the transcriptome data of SK-N-BE2 cells with or without depletion (GSE180797) and revealed 18 metabolic enzyme genes that were deeply involved in nucleotide synthesisCitation5 and significantly suppressed (). These genes encode proteins including 7 enzymes (PRPS1, PPAT, GART, PFAS, PAICS, ATIC, and GMPS) that directly participate in the biosynthesis of purine nucleotides from R-5-P (). Nucleotide biosynthesis has feeder pathways, including the serine-glycine pathways and amidotransferase reactions, that provide carbon and nitrogen precursors;Citation5 the expression of six enzymes (GLUD1, GOT2, PHGDH, PSAT1, SHMT1, and SHMT2) involved in these pathways was also depleted (). We confirmed the downregulation of these genes by RT‒qPCR in neuroblastoma SK-N-BE2, BE-2C, and SK-N-AS cells ( and S1). Oncogenic MYC and E2F are two critical transcription factor families that are often overexpressed in cancers and directly transcribe many enzymes involved in nucleotide biosynthesis.Citation5 Our previous study showed that USP29 positively regulates the MYC downstream pathway.Citation9 Interestingly, we also revealed that USP29 depletion markedly suppressed the gene signatures of E2F () by gene set enrichment analysis (GSEA).Citation18 These results indicated that USP29 may promote nucleotide biosynthesis by regulating the MYC and E2F downstream pathways.
Figure 4. Transcriptomic analysis of USP29-depleted SK-N-BE2 cells.
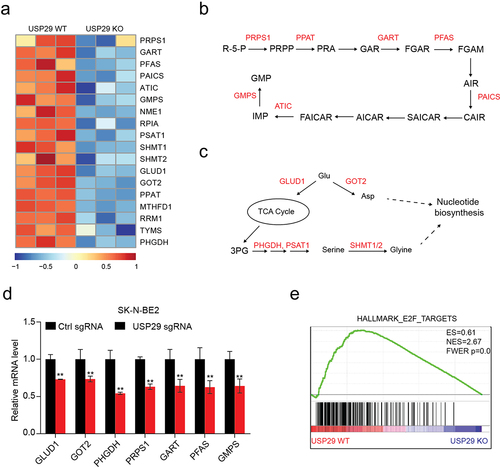
Discussion
Deubiquitinases were reported to be involved in many aspects of metabolic processes by regulating metabolic enzymes, transporters, transcription factors, and/or signaling pathways.Citation22 In neuroblastoma, we previously revealed that USP29 promotes glycolysis and glutamine catabolism in normoxia and/or hypoxia.Citation9 Considering that glucose and glutamine are two major nutrients of tumor cells, it is conceivable that USP29 may also influence nucleotide synthesis by regulating the feeder pathways; however, the specific role and underlying mechanisms of USP29 in de novo nucleotide biosynthesis remain unclear. In this study, we found that USP29-depleted SK-N-BE2 cells showed significant suppression of glucose-derived nucleotide biosynthesis and gene expression of metabolic enzymes, which were involved in both de novo nucleotide biosynthesis processes and feeder pathways, in both normoxia and hypoxia. Analysis of the USP29-mediated transcriptome suggested that it may function by regulating the MYC and E2F pathways, which play a critical role in regulating de novo nucleotide biosynthesis.Citation5 Together with previous studies,Citation9,Citation10 this study revealed that USP29 acts as a key regulator of tumor cell metabolic reprogramming by affecting several oncogenic pathways.
Dysregulated cancer cell metabolism provides not only proliferative advantages but also new opportunities for cancer therapy, and targeting tumor cell nucleotide biosynthesis has been successfully used in modern chemotherapy.Citation23 Unfortunately, inhibitors of nucleotide metabolism (also known as antimetabolites), including methotrexate, 5-fluorouracil, 6-mercaptopurine and pemetrexed, which antagonize the activity of enzymes involved in nucleotide biosynthesis, frequently cause severe side effects due to on-target inhibition of the same enzymes in normal cells. Deubiquitinases have been regarded as potential therapeutic targets in cancer metabolism and treatment.Citation22,Citation24,Citation25 In this study, we revealed the key role of USP29 in promoting nucleotide biosynthesis, given that USP29 may be an alternative target for targeting cancer cell nucleotide metabolism. Indeed, USP29 depletion has been reported to suppress malignant phenotypes in several other cancers, including gastric cancer,Citation15,Citation16 colorectal cancerCitation14,Citation26 and hepatocellular carcinoma cells.Citation10
In summary, we revealed a key role of USP29 in regulating cancer cell nucleotide biosynthesis, and our findings highlight that USP29 may become a potential therapeutic target for neuroblastoma.
Ethical approval
This article does not contain any studies with human participants or animals performed by any of the authors.
Supplemental Material
Download MS Word (1.8 MB)Disclosure statement
No potential conflict of interest was reported by the author(s).
Data availability statement
All data generated or analyzed during this study are included in this article. The datasets used and/or analyzed during the current study are available from the corresponding authors on reasonable requests.
Supplementary material
Supplemental data for this article can be accessed online at https://doi.org/10.1080/15384047.2023.2237200
Additional information
Funding
Notes on contributors
Ye Kang
Ye Kang Assistant research fellow, Department of Cancer Precision Medicine, The MED-X Institute, The First Affiliated Hospital of Xi’an Jiaotong University, Xi’an, China; Email: [email protected]
Yahuan Yu
Yahuan Yu Graduate student, Department of Nephrology, The Affiliated Hospital of Qingdao University, Qingdao, China; Email: [email protected]
Yijia Liu
Yijia Liu Technician, Precision Medicine Center, The First Affiliated Hospital of Xi’an Jiaotong University, Xi’an, China; Email: [email protected]
Yiwen Pan
Yiwen Pan Technician, Precision Medicine Center, The First Affiliated Hospital of Xi’an Jiaotong University, Xi’an, China; Email: [email protected]
Ru Zhang
Ru Zhang Technician, Department of Cancer Precision Medicine, The MED-X Institute, The First Affiliated Hospital of Xi’an Jiaotong University, Xi’an, China; Email: [email protected]
Doudou Ren
Doudou Ren Technician, Department of Cancer Precision Medicine, The MED-X Institute, The First Affiliated Hospital of Xi’an Jiaotong University, Xi’an, China; Email: [email protected]
Zeqiong Cai
Zeqiong Cai Technician, Department of Cancer Precision Medicine, The MED-X Institute, The First Affiliated Hospital of Xi’an Jiaotong University, Xi’an, China; Email: [email protected]
Junpeng Ma
Junpeng Ma Technician, Center for Molecular Diagnosis and Precision Medicine, Department of Clinical Laboratory, The First Affiliated Hospital of Nanchang University, Nanchang, China; Email: [email protected]
Xiaofan Xiong
Xiaofan Xiong Assistant research fellow, Department of Tumor and Immunology, Precision Medicine Institute, Building 21, Western China Science and Technology Innovation Harbor, Xi’an, China; Email: [email protected]
Qi Zhang
Qi Zhang Assistant research fellow, Department of Emergency Surgery, Union Hospital, Tongji Medical College, Huazhong University of Science and Technology, Wuhan, China; Email: [email protected]
Chengsheng Zhang
Chengsheng Zhang Director, Center for Molecular Diagnosis and Precision Medicine, Department of Clinical Laboratory, The First Affiliated Hospital of Nanchang University, Nanchang, China; Email: [email protected]
Rongfu Tu
Rongfu Tu Assistant research fellow, Department of Cancer Precision Medicine, The MED-X Institute, The First Affiliated Hospital of Xi’an Jiaotong University, Xi’an, China; Email: [email protected]
References
- Zhu J, Thompson CB. Metabolic regulation of cell growth and proliferation. Nat Rev Mol Cell Biol. 2019;20(7):436–8. doi:10.1038/s41580-019-0123-5.
- Aird KM, Zhang R. Nucleotide metabolism, oncogene-induced senescence and cancer. Cancer Lett. 2015;356(2):204–210. doi:10.1016/j.canlet.2014.01.017.
- Lunt SY, Vander Heiden MG. Aerobic glycolysis: meeting the metabolic requirements of cell proliferation. Annu Rev Cell Dev Biol. 2011;27(1):441–464. doi:10.1146/annurev-cellbio-092910-154237.
- Dong Y, Tu R, Liu H, Qing G. Regulation of cancer cell metabolism: oncogenic MYC in the driver’s seat. Curr Signal Transduct Ther. 2020;5(1):124. doi:10.1038/s41392-020-00235-2.
- Lane AN, Fan TW. Regulation of mammalian nucleotide metabolism and biosynthesis. Nucleic Acids Res. 2015;43(4):2466–2485. doi:10.1093/nar/gkv047.
- Maris JM. Recent advances in neuroblastoma. N Engl J Med. 2010;362(23):2202–2211. doi:10.1056/NEJMra0804577.
- Huang M, Weiss WA. Neuroblastoma and MYCN. Cold Spring Harb Perspect Med. 2013;3(10):a014415–a014415. doi:10.1101/cshperspect.a014415.
- Pinto NR, Applebaum MA, Volchenboum SL, Matthay KK, London WB, Ambros PF, Nakagawara A, Berthold F, Schleiermacher G, Park JR, et al. Advances in risk classification and treatment strategies for neuroblastoma. J Clin Oncol. 2015;33(27):3008–3017. doi:10.1200/JCO.2014.59.4648.
- Tu R, Kang W, Yang M, Wang L, Bao Q, Chen Z, Dong Y, Wang J, Jiang J, Liu H, et al. USP29 coordinates MYC and HIF1α stabilization to promote tumor metabolism and progression. Oncogene. 2021;40(46):6417–6429. doi:10.1038/s41388-021-02031-w.
- Gao R, Buechel D, Kalathur RKR, Morini MF, Coto-Llerena M, Ercan C, Piscuoglio S, Chen Q, Blumer T, Wang X, et al. USP29-mediated HIF1α stabilization is associated with sorafenib resistance of hepatocellular carcinoma cells by upregulating glycolysis. Oncogenesis. 2021;10(7):52. doi:10.1038/s41389-021-00338-7.
- Liu J, Chung H-J, Vogt M, Jin Y, Malide D, He L, Dundr M, Levens D. JTV1 co-activates FBP to induce USP29 transcription and stabilize p53 in response to oxidative stress. Embo J. 2011;30(5):846–858. doi:10.1038/emboj.2011.11.
- Martin Y, Cabrera E, Amoedo H, Hernández-Pérez S, Domínguez-Kelly R, Freire R. USP29 controls the stability of checkpoint adaptor Claspin by deubiquitination. Oncogene. 2015;34(8):1058–1063. doi:10.1038/onc.2014.38.
- Chandrasekaran AP, Woo SH, Sarodaya N, Rhie BH, Tyagi A, Das S, Suresh B, Ko NR, Oh SJ, Kim K-S, et al. Ubiquitin-specific protease 29 regulates Cdc25A-Mediated tumorigenesis. Int J Mol Sci. 2021;22(11):5766. doi:10.3390/ijms22115766.
- Li J, Yang J, Chen Z, Liu L, Wang H, Deng Q, Chen Y, Que Y, Fu Z. Promotive role of USP29-mediated deubiquitination in malignant proliferation of colorectal cancer cells via the KIAA1429/SOX8 axis. Bosn J of Basic Med Sci. 2022. doi:10.17305/bjbms.2022.7930.
- Qian W, Li Q, Wu X, Li W, Li Q, Zhang J, Li M, Zhang D, Zhao H, Zou X, et al. Deubiquitinase USP29 promotes gastric cancer cell migration by cooperating with phosphatase SCP1 to stabilize Snail protein. Oncogene. 2020;39(44):6802–6815. doi:10.1038/s41388-020-01471-0.
- Wu Y, Zhang Y, Wang D, Zhang Y, Zhang J, Zhang Y, Xu L, Wang T, Wang S, Zhang Q, et al. USP29 enhances chemotherapy-induced stemness in non-small cell lung cancer via stabilizing Snail1 in response to oxidative stress. Cell Death Disease. 2020;11(9):796. doi:10.1038/s41419-020-03008-5.
- Hu J, Wang T, Xu J, Wu S, Wang L, Su H, Jiang J, Yue M, Wang J, Wang D, et al. WEE1 inhibition induces glutamine addiction in T-cell acute lymphoblastic leukemia. Haematologica. 2021;106(7):1816–1827. doi:10.3324/haematol.2019.231126.
- Subramanian A, Tamayo P, Mootha VK, Mukherjee S, Ebert BL, Gillette MA, Paulovich A, Pomeroy SL, Golub TR, Lander ES, et al. Gene set enrichment analysis: a knowledge-based approach for interpreting genome-wide expression profiles. Proc Natl Acad Sci USA. 2005;102(43):15545–15550. doi:10.1073/pnas.0506580102.
- Pareek V, Pedley AM, Benkovic SJ. Human de novo purine biosynthesis. Crit Rev Biochem Mol Biol. 2021;56(1):1–16. doi:10.1080/10409238.2020.1832438.
- Pavlova NN, Thompson CB. The emerging hallmarks of cancer metabolism. Cell Metab. 2016;23(1):27–47. doi:10.1016/j.cmet.2015.12.006.
- Denko NC. Hypoxia, HIF1 and glucose metabolism in the solid tumour. Nat Rev Cancer. 2008;8(9):705–713. doi:10.1038/nrc2468.
- Tu R, Ma J, Zhang P, Kang Y, Xiong X, Zhu J, Li M, Zhang C. The emerging role of deubiquitylating enzymes as therapeutic targets in cancer metabolism. Cancer Cell Int. 2022;22(1):130. doi:10.1186/s12935-022-02524-y.
- Vander Heiden MG. Targeting cancer metabolism: a therapeutic window opens. Nat Rev Drug Discov. 2011;10(9):671–684. doi:10.1038/nrd3504.
- D’Arcy P, Wang X, Linder S. Deubiquitinase inhibition as a cancer therapeutic strategy. Pharmacology & Therapeutics. 2015;147:32–54. doi:10.1016/j.pharmthera.2014.11.002.
- Harrigan JA, Jacq X, Martin NM, Jackson SP. Deubiquitylating enzymes and drug discovery: emerging opportunities. Nat Rev Drug Discov. 2018;17(1):57–78. doi:10.1038/nrd.2017.152.
- Chandrasekaran AP, Suresh B, Sarodaya N, Ko N-R, Oh S-J, Kim K-S, Ramakrishna S. Ubiquitin specific protease 29 functions as an oncogene promoting tumorigenesis in colorectal carcinoma. Cancers Basel. 2021;13(11):2706. doi:10.3390/cancers13112706.