ABSTRACT
Background
PD-1/PD-L1 inhibitors are approved treatments for patients with esophageal squamous cell carcinoma (ESCC). The present investigation aspired to explore the interrelation between molecular phenotype and PD-L1 expression in ESCC.
Methods
PD-L1 testing and targeted next-generation sequencing (NGS) were performed on tumoral tissues from 139 ESCC patients. Tumor-infiltrating lymphocytes (TILs) were scrutinized using a tyramide signal amplification system combined with immunohistochemistry.
Results
Among enrolled patients, 36.7% displayed high PD-L1 expression (combined positive score [CPS] ≥10). BRCA1 and NF1 gene mutations were significantly associated with high PD-L1 expression (p < .05) while TGFβ pathway alterations were linked to low PD-L1 expression (p = .02). High copy number instability (CNI) and copy number alterations (CNA) were correlated with low PD-L1 expression. Patients with CDKN2A deletion exhibited higher PD-L1 expression. Varying types of TILs were observed across different PD-L1 expression groups. The ratio of CD8+PD-L1+ T cells and CD8+PD-1+ T cells to CD8+ T cells remained comparable in both tumoral and stromal regions, but the ratio of CD68+PD-L1+ macrophages to CD68+ macrophages was higher than the ratio of CD68+PD-1+ macrophages to CD68+ macrophages. CPS was significantly correlated with PD-L1+ lymphocytes and CD68+ macrophages in the tumoral region. CD8+ T cell infiltration was positively correlated with PD-1+ cells in both tumoral and stromal regions.
Conclusion
In this study, we presented the prevalence rates of PD-L1 expression in Chinese ESCC patients. The association of genetic profiles with PD-L1 expression levels also provide the clue that genomic phenotype may interact with the immunologic phenotype in ESCC.
KEYWORDS:
Introduction
Esophageal cancer (EC) ranks as the eighth most prevalent cancer and the sixth leading cause of cancer-related mortality, with a relatively modest five-year overall survival (OS) rate.Citation1,Citation2 Based on histological characteristics, EC is typically classified into two main subtypes: esophageal adenocarcinoma (EAC) and esophageal squamous cell carcinoma (ESCC).Citation2 In China, ESCC is the major subtype of EC, accounting for more than 90% of all EC cases.Citation3 Currently, treatment options for ESCC remain limited, underscoring the urgent imperative to identify more precise biomarkers and effective therapeutic targets to improve the treatment outcomes for these patients.
Immune checkpoint inhibitors (ICIs) have been shown to improve the clinical outcomes in patients with many tumor types. ICIs are divided into two main categories, targeting programmed death-1/programmed death ligand-1 (PD-1/PD-L1) and cytotoxic T lymphocyte antigen 4 (CTL-4), respectively. PD-1/PD-L1 blockade has demonstrated significant responses and clinical benefits across multiple malignancies, including ESCC.Citation4–7 PD-L1 is expressed on various cells within the tumor microenvironment (TME), encompassing immune cells and tumor cells. It couples with PD-1 to restrain the function of T cells in the immune system and is involved in preventing autoimmune responses and excessive inflammation.Citation8 Detection of PD-L1 expression through immunohistochemistry (IHC) has been proposed as a prospective predictive biomarker to identify patients who may derive substantial benefits from anti-PD-1/PD-L1 therapy. This evidence has led to the Food and Drug Administration (FDA) approving its clinical use in a variety of tumor types.Citation9–11
PD-1/PD-L1 inhibitors have shown promising anti-tumor activity in patients with advanced EC.Citation12–14 In the phase III KEYNOTE-181 trial, pembrolizumab did not confer a significant survival benefit over chemotherapy as a second or subsequent line of treatment. However, in the subgroup of patients with a PD-L1 combined positive score (CPS) ≥10, encompassing both PD-L1 expression on both tumors and immune cells, treatment with pembrolizumab was associated with improved overall response rate (ORR) and survival.Citation15 Moreover, in the KEYNOTE-590 study, a noteworthy survival benefit was observed when pembrolizumab was combined with cisplatin-fluoropyrimidine chemotherapy in ESCC patients with CPS ≥ 10.Citation16 Meanwhile, the ATTRACTION-3 and ESCORT studies showed that the PD-1 antibodies nivolumab and camrelizumab were associated with a statistically significant and clinically meaningful improvement in OS, compared to chemotherapy in patients with advanced ESCC.Citation17,Citation18
In this study, the researchers conducted PD-L1 testing and targeted NGS on tumor tissue samples from ESCC patients. The primary objectives were to explore the correlation between the molecular profile and patterns of PD-L1 expression and to identify potential genomic alterations that may contribute to favorable responses to anti-PD-1/PD-L1 immunotherapy.
Materials and methods
Patient information
The samples and data of 139 patients with histologically confirmed ESCC at the First Hospital of Jilin University from 2019 to 2022 were reviewed. The clinical characteristics of the patients were presented in Table S1. Only patients who had both formalin-fixed paraffin-embedded (FFPE) tissues and matched blood samples available for analysis underwent testing using a designed 543-gene NGS panel to evaluate for somatic and germline mutations. Our study adhered to the tenets of the Declaration of Helsinki and was approved by the Ethical Review Board of the First Hospital of Jilin University (No.2018–467).
DNA extraction and sequencing
To identify somatic single nucleotide variants (SNVs) and indel mutations, FFPE tumor samples were tested, and the matched blood samples were used as controls. VarScan2 (version 2.4.2) was used for SNV calling and the identified variants were annotated by ANNOVAR. The exclusion criteria of variants were as follows: (a) sequencing coverage < 30×, (b) silent mutations in non-reference alleles, (c) support reads were < 5, (d) allele frequency ≥ 0.005 in the Exome Aggregation Consortium (ExAC) or Genome Aggregation Database (gnomAD) database, and (e) allele frequency < 0.02 in the tumor sample.
Tumour mutational burden (TMB)
TMB (mutations/Mb) was calculated using a previously established algorithm.Citation19 Nonsynonymous somatic mutations at the exonic and splicing regions were quantified to determine the TMB value of a sample, with a variant frequency of not less than 0.7%. The total number of mutations counted was divided by the size of the coding region of the target panel to calculate the TMB per megabase.
Copy number instability (CNI)
GC content and length of target region were first corrected using proprietary algorithms for each region. Subsequently, read counts were transformed into log2 ratios and further transformed to Z-score based on Gaussian transformations, comparing them against a normal control group (n = 30). Finally, those target regions were retained whose Z-scores were greater than the 95th percentile and whose absolute standard deviation was twice that of the normal control group. The remaining Z-scores were summed as the CNI score.
Copy number analysis
For copy number analysis, a set of 30 normal blood samples served as controls to calculate the gene specificity score (GCS). A joint statistical significance test involving GCS and the absolute value of the copy number was employed to determine CNV. If the copy number value was > 4, it was defined as CNV gain, and if the copy number value was < 1, it was defined as CNV loss, respectively. The copy number alteration (CNA) burden was estimated based on the total number of genes exhibiting copy number gains and losses across the samples under investigation.
PD-L1 expression
IHC staining was performed to assess the PD-L1 expression on the surface of tumor cells (TC) and tumor-infiltrating immune cells (IC). FFPE tissue blocks were sliced up into 4-mm thick sections and stained with anti-PD-L1 22C3 primary antibody (1:50, Dako, M3653) using a Ventana GX automated system (Ventana, AZ, USA). PD-L1 CPS was calculated as the number of PD-L1 positive cells (tumor cells, macrophages and lymphocytes) divided by the total number of tumor cells.
Multiplex immunohistochemistry
For multiplex immunohistochemical staining, FFPE tissue blocks were sliced up into 4-mm thick sections. The immune biomarker panel included CD68 (1:500, Beijing Zhongshan Golden Bridge Biotechnology, ZM0060), CD8 (1:100, Beijing Zhongshan Golden Bridge Biotechnology, ZA0508), PD-1 (1:50, Beijing Zhongshan Golden Bridge Biotechnology, ZM0381) and PD-L1 (1:25, Roche Diagnostics, 740–4859). TSA visualization was performed using an Opal ™ 7-color IHC Kit (PerkinElmer Inc., Boston, MA, USA) according to the manufacturer’s instructions. The fluorophores used in the experiment were Opal 520 (CD8), Opal 570 (PD-L1), Opal 650 (CD68) and Opal 690 (PD-1), with intensity values fixed at 81%. Nuclear counterstaining was performed with DAPI. A PerkinElmer Vectra imaging system (Vectra3.0.5; PerkinElmer, Massachusetts, USA) was used to scan the sections. Regions of interest (ROI) were selected using the Phenochart viewer (Akoya Bioscience) analyzed using inForm Advanced Image Analysis software (inForm 2.3.0; PerkinElmer, Massachusetts, USA). The density of positively stained cells was counted for tumor parenchyma (tumor), distant stroma and total regions respectively.
Statistical analysis
All statistical analyses were performed using the R software (version 4.1.1; https://www.r-project.org/, Institute of Statistics and Mathematics, Vienna, Austria). Fisher’s exact test was used for comparisons between two categorical variables, and Mann – Whitney U test was used for comparisons between two continuous variables. All differences with P < .05 were considered statistically significant.
Results
Basic information and PD-L1 expression distribution in our cohort
A total of 139 ESCC patients were eligible for this study. Their samples were subjected to targeted NGS and PD-L1 IHC staining. Out of these patients, 27 patients had sufficient samples for multiplex immunofluorescence (mIF).
Findings from KEYNOTE-181 and KEYNOTE-590 showed that only patients with a high CPS (≥10) benefited from the PD-1 blockades. In this study, a good correlation was observed between CPS and tumor proportion score (TPS) (R = 0.941, p < 2.2e-16) (). Therefore, we used CPS to represent PD-L1 expression and used CPS = 10 as the cutoff to divide patients into two groups, the high PD-L1 and low PD-L1 groups. We observed that 36.7% of the ESCC patients in this study had high PD-L1 expression (CPS ≥10) while the rest (63.3%) were in the range of 0 to 10 (), which is consistent with the observations from a previous study.Citation20 The distribution of age, sex and stage was not significantly different between the high and low PD-L1 expression groups (Table S1).
Mutation profiles of the high and low PD-L1 groups
The mutational profiles of patients in the two groups were examined, and the frequencies of mutated genes were compared (). Similar to a previous study,Citation21 the most frequently mutated genes in this study were TP53 (91%), NOTCH1 (30%), KMT2D (22%) and PIK3CA (21%). Notably, mutations in BRCA1 and NF1 genes were the only two genes that were more significantly enriched in patients with high PD-L1 expression than in patients with low PD-L1 expression (BRCA1, p = .02; NF1, p = .03) (). Furthermore, the PD-L1 expression in patients with BRCA1 or NF1 mutations was higher than in those with wild-type (WT) tumors (BRCA1, p = .06; NF1, p = .035) ().
Figure 2. Somatic mutations within the PD-L1 subgroups. (a) mutational landscape of the high PD-L1 and low PD-L1 groups. (b) comparison of the frequency of mutated genes in the high PD-L1 and low PD-L1 groups. (c) expression of PD-L1 in patients with and without BRCA1 mutations. (d) expression of PD-L1 in patients with and without NF1 mutations. (e) correlation between CPS and TMB. (f) comparison of TMB in the high PD-L1 and low PD-L1 groups.
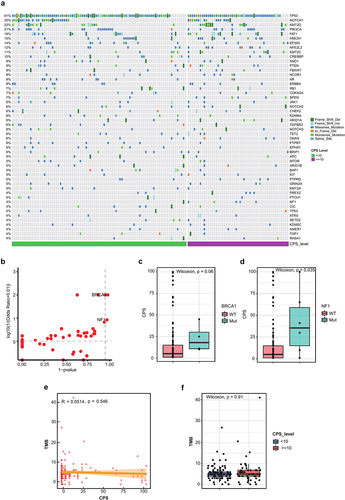
TMB has previously been shown to independently predict ICI responseCitation22,Citation23 but was not associated with PD-L1 expression in several cancer types.Citation24,Citation25 In this study, we observed a similar finding, where PD-L1 expression did not show any significant association with TMB, whether considered as a continuous or categorical variable ().
A pathway analysis was then performed to assess the alterations between the two groups (). Similar to another report,Citation21 most mutations were found to be enriched in the p53 pathway (91%), followed by the Notch (50%) and Ras (46%) pathways. Alterations in the TGFβ pathway were observed more frequently in the low PD-L1 group than in the high PD-L1 group (p = .02) (). Furthermore, lower PD-L1 expression was observed in patients with TGFβ pathway alterations (). TGFBR2, SMAD4 and TGFBR1 were included in the TGFβ pathway () and their mutations were also found to be enriched in the low PD-L1 group compared to the high PD-L1 group ().
Figure 3. Pathway alterations of the PD-L1 subgroups. (a) pathway alteration landscape of the high PD-L1 and low PD-L1 groups. (b) comparison of the frequency of altered pathways in the high PD-L1 and low PD-L1 groups. (c) expression of PD-L1 in patients with and without TGFβ pathway alteration. (d) comparison of the frequency of mutated genes involved in TGFβ pathway in the high PD-L1 and low PD-L1 groups. (e – g) expression of PD-L1 in patients with and without TGFBR1, TGFBR2 or SMAD4 mutations.
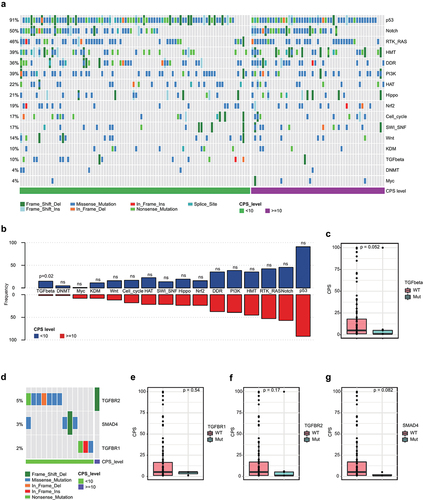
CNA in the high and low PD-L1 groups
As CNI and CNA are predictive of response to ICIs responses,Citation25–28 the CNI and CNA of the two groups were assessed. Our findings showed that both CNI and CNA were negatively associated with PD-L1 expression (R = −0.285, p = .00451;R = −0.193, p = .0194) (), and the CNI and CNA levels of the low PD-L1 group were higher than those of the high PD-L1 group (p = .044; p = .036) (). The CNA profiles of the two groups are illustrated in . CDKN2A deletion emerged as one of the most prevalent CNAs observed in the ESCC patients, accounting for 29% of cases. Interestingly, we observed that PD-L1 expression was significantly lower in patients with CDKN2A deletion (). Additionally, we identified that amplifications in the TP63 and PRKCI genes were enriched in the PD-L1 low expression group ().
Figure 4. Copy number alterations (CNA) in the PD-L1 subgroups. (a) correlation between copy number instability (CNI) and CPS. (b) comparison of CNI in the high PD-L1 and low PD-L1 groups. (c) correlation between CNA and CPS. (d) comparison of CNA in the high PD-L1 and low PD-L1 groups. (e) copy number alteration landscape of the high PD-L1 and low PD-L1 groups. (f) expression of PD-L1 in patients with and without deletion of CDKN2A. (g) comparison of the frequency of PRKCI amplification in the high PD-L1 and low PD-L1 groups. (h) comparison of the frequency of TP63 amplification in the high PD-L1 and low PD-L1 groups.
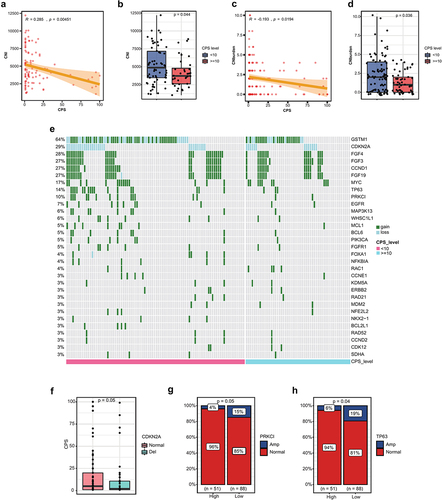
Tumour-infiltrating lymphocytes (TILs) and PD-L1 expression
Tumor-infiltrating lymphocytes play an important role in the tumor immune environment, and PD-L1 is expressed on the membrane of various TILs, including B and T lymphocytes, dendritic cells, macrophages and mast cells.Citation29 Immunohistochemical staining for multiple markers, including CD8, CD68, PD-L1 and PD-1, was performed to assess the infiltration of TILs in 27 ESCC patients ().
Figure 5. Association between tumor-infiltrating lymphocytes (TILs) and PD-L1 expression. (a) Representative mIHC staining figures of the group with high PD-L1 expression. (b) Representative mIHC staining figures of the group with low PD-L1 expression. (c) the ratio of PD-L1+ or PD-1+ CD8+ T cells to CD8+ T cells. (d) the ratio of PD-L1+ or PD-1+ CD68+ macrophage to CD68+ macrophage. (e) correlation between CPS and TILs in the tumoral and stromal region. (f) correlation matrix between TILs in tumor and stroma region. (***, p < .001; **, p < .01; *, p < .05).
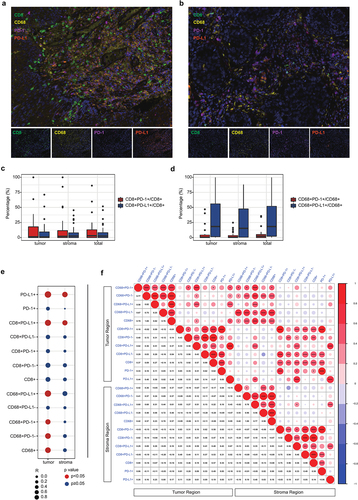
First, the ratio of PD-L1+ or PD-1+ CD8+ T cells to CD8+ T cells and PD-L1+ or PD-1+ CD68+ macrophages to CD68+ macrophages was assessed, respectively. The ratio of CD8+PD-L1+ T cells and CD8+PD-1+ T cells to CD8+ T cells was found to be similar in both the tumoral and stromal regions (), whereas the ratio of CD68+PD-L1+ macrophages to CD68+ macrophages was higher than the ratio of CD68+PD-1+ macrophages to CD68+ macrophages ().
Second, we examined the association between PD-L1 expression and TILs. Correlation analysis showed that CPS was significantly correlated with the density of PD-L1+ lymphocytes and CD68+ cells in the tumoral region but not in the stromal region (). The correlation analysis between TILs is shown in .
In a previous study, the abundance of CD8+ T cells was found to be associated with a favorable response to anti-PD-1/PD-L1 therapy.Citation30 In this study, we found that the infiltration of CD8+ T cells was positively correlated with PD-1+ cells in both the tumoral and stromal regions. However, CD8+PD-L1+ T cells were strongly correlated with CD68+ macrophages (including CD68+PD-1+ and CD68+PD-L1+ macrophages) in the tumor region only ().
Discussion
Understanding the correlation between molecular alterations and PD-L1 expression may help identify more clinically reliable factors associated with anti-PD-1/PD-L1 treatment response and improve the clinical outcomes. This study describes diverse molecular features and TILs profiles at different PD-L1 expression levels in ESCC patients, providing suggestions to further enable precision therapy with anti-PD-1/PD-L1 treatment.
This study identified several molecular alterations associated with differential PD-L1 expression. Mutations in single genes including BRCA1 and NF1 demonstrated significant correlations with high PD-L1 expression in ESCC patients. The NF1 gene encodes neurofibromin, which is ubiquitously expressed in human tissues and is involved in the Hippo-YAP and RAS signaling pathwayCitation31–33 (YAP has been shown to be involved in the positive regulation of CD274 transcription.Citation34,Citation35 The inactive mutations of NF1 may lead to YAP translocation to the nucleus and activates the transcription of CD274 (PD-L1), which probably explains the association between NF1 mutation and PD-L1 expression. It has been reported that melanoma patients with mutated NF1 gene showed favorable responses to anti-PD–1/PD-L1,Citation36 suggesting that NF1 mutations may correlate with the outcome of ESCC patients receiving anti-PD-1/PD-L1 therapy. According to previous studies, only alterations in the BRCA2 gene, but not BRCA1, were observed to predict ICIs response in some cancers.Citation37,Citation38 Conversely, in our study, only BRCA1 mutation was associated with high PD-L1 expression, suggesting an inconsistent impact of BRCA1/2 alterations in different cancer types.
In this study, we have additionally ascertained that alterations in the TGFβ pathway were associated with low PD-L1 expression. TGFβ shapes the tumor microenvironment by limiting T-cell infiltration and attenuating the tumor response to PD-L1 blockade.Citation39 In a two-part, single-arm, multinational, Phase Ib study,Citation40 the combination of the TGFβ RI inhibitor galunisertib with PD-L1 inhibitor durvalumab in patients with pancreatic cancer demonstrated promising clinical activity with a DCR of 25.0% and an ORR of 3.1%. The results of this study suggest that ESCC patients with high PD-L1 expression and TGFβ pathway mutation may benefit from this novel treatment strategy. Mechanically, exogenous TGFβ can elevate CD274 transcription through Smad binding elements.Citation41,Citation42 Conversely, the inactivation of TGFβ would result in the downregulation of CD274 transcription, subsequently leading to decreased PD-L1 expression. Our findings align harmoniously with the aforementioned studies.
TMB has previously been shown to independently predict anti-PD-1/PD-L1 responseCitation22,Citation23 but was not associated with PD-L1 expression in several cancers.Citation24,Citation25 Similarly, our present investigation revealed that the TMB of ESCC patients possessing high and low PD-L1 expression levels remained comparable.
The predictive role of CNA for outcomes in patients with various cancers undergoing ICIs has been reported.Citation25–27 For instance, a lower burden of copy number loss (CNloss) was observed in responders to ICIs treatment in melanoma.Citation25 In gastrointestinal cancer, lower CNA was associated with better response to ICIsCitation43 including PD-1/PD-L1 blockade. In our study, CNI and CNburden were both significantly but negatively correlated with PD-L1 expression, indicating that CNI and CNburden might also be predictors of PD-1/PD-L1 blockade in ESCC. Notably, CDKN2A CNloss is more common in patients with PD-L1 low expression. CDKN2A represents a tumor suppressor gene located on chromosome 9p21.3, encoding proteins that govern the cell cycle.Citation44 This gene ranks among the most frequently altered genes in human cancers, with its deletion being reported in over 30% of ESCC in the Asian population,Citation45 a proportion closely resembling our findings. Genomic deletions, so-called “deep deletions” or homozygous deletions, which usually extend to other genes on 9p21.3, are the most common alterations affecting CDKN2A.Citation46 Loss of CDKN2A could induce the activation of CDK4/CDK6 which could contribute to destabilization of PD-L1 protein.Citation47 Several studies have examined the impact of CDKN2A alterations on the tumor immune microenvironment and the ICI response.Citation39,Citation48 In melanoma, immune checkpoint blockade is less effective when CDKN2A is absent.Citation49,Citation50 In a study of 909 NSCLC tumor specimens, CDKN2A pure deletion was significantly associated with negative PD-L1 expression.Citation51 In uroepithelial cancers, CDKN2A deletion was found to be associated with a reduced benefit from ICI therapy.Citation52 In our study, PD-L1 expression was significantly higher in patients with CDKN2A deletion than in those with normal CDKN2A, suggesting that CDKN2A deletion might be associated with the response to anti-PD-1/PD-L1 immunotherapy in ESCC patients.
TILs have been shown to play an important role in the tumor immune environment. It was previously reported that PD-L1 was expressed in both tumor epithelial cells and TILs. In our study, we found that PD-L1 expression was positively correlated with the infiltration of CD8+PD-L1+ T cells and CD68+PD-L1+ macrophages. CD8+ T cells have been reported to play a key role in killing tumor cells. Moreover, a comprehensive meta-analysis has demonstrated that CD8+ T cells hold the ability to prognosticate treatment outcomes in patients undergoing ICIs, be it as a standalone therapy or in conjunction with other treatment modalities, spanning diverse types of cancers.Citation53 Besides, in an immunotherapy cohort, higher infiltration of CD8+PD-L1+ T cells was significantly associated with better response to anti-PD-1 treatment in NSCLC patients.Citation54 High infiltration of CD68+PD-L1+ macrophages has been reported to be associated with improved survival in primary testicular lymphoma and triple-negative breast cancer.Citation55,Citation56 These reports suggest that PD-L1 expression and infiltration of PD-L1+ macrophages might also contribute to favorable responses with PD-L1/PD-L1 blockade in ESCC patients.
Conclusion
This study highlights the correlation between PD-L1 expression and molecular alterations in patients with ESCC. It delves into the status of TILs concerning various patterns of PD-L1 expression, suggesting that these factors may influence the outcomes of anti-PD-1/PD-L1 immunotherapy. The significance of these findings warrants validation through future investigations involving more extensive cohorts of ESCC patients. The interpretation of potential biological connections between genomic profiling and PD-L1 expression might provide new insights to further improve precision therapy with PD-L1 blockade.
Author contributions
All authors contributed to the study conception and design. Study design and material preparation were performed by XH, SZ and YJ. Data collection and analysis were performed by XH, SZ, PZ, AL, LC, DW, JH and YJ. The first draft of the manuscript was written by XH and SZ and all authors commented on previous versions of the manuscript. All authors read and approved the final manuscript.
Ethics statement
The study was conducted in accordance with the principles of the Declaration of Helsinki and was approved by the ethical review board of the First Hospital of Jilin University (No.2018–467).
Supplemental Material
Download MS Word (17.3 KB)Disclosure statement
No potential conflict of interest was reported by the author(s).
Data availability statement
The data that support the findings of this study are available from the corresponding author Yanfang Jiang, upon reasonable request.
Supplementary material
Supplemental data for this article can be accessed online at https://doi.org/10.1080/15384047.2023.2256927
Additional information
Funding
Notes on contributors
Songchen Zhao
Songchen Zhao is currently working toward the Ph.D. degree on Oncology in Department of Medical Oncology, Shanghai Pulmonary Hospital, Tongji University School of Medicine, Shanghai, People's Republic of China.
Xintong Hu
Xintong Hu received the B.S. degree in Medical examination from Beihua University, Jilin, China, in 2014. And the Ph.D. degree in Clinical laboratory diagnostics from Jilin University, Changchun, China, in 2020. Her research interests include tumor gene research and precision medicine.
Peiwen Zhou
Peiwen Zhou graduated from Jilin University with a bachelor's degree in clinical medicine. Changchun in 2021. Pursuing PhD in Jilin University, Changchun. Her research interests include tumor gene research and precision medicine.
Ang Li
Ang Li received the medical master degree in Clinical Laboratory Diagnostics from Jiangsu University. Jiangsu. China. in 2018 and the medical doctor degree in Clinical Laboratory Diagnostics from Jilin University. Changchun. China in 2023. His research interests include tumor gene research and precision medicine.
Liguo Chen
Liguo Chen received the B.S. degree in bioengineering from Nanjing Agricultural University. Nanjing. China, in 2012. His research interests include sequencing and analysis of tumor genes.
Duo Wang
Duo Wang received the B.S. degree in Medical Laboratory Technology from Beihua University. Jilin. China, in 2018 and the M.S. degree in Clinical Laboratory Diagnostics from Beihua University.Jilin. China, in 2021. Her research interests include sequencing and analysis of tumor genes.
Jiaxue He
Jiaxue He received the B.S. degree in Animal Medical Science from Jilin Agricultural University. Changchun. China. in 2006 and the M.S. degree in Preventive Veterinary Medicine from Jilin Agricultural University. Changchun, China in 2009, the Ph.D. degree in Pathology and Pathophysiology from Jilin University. Changchun. China in 2013. Her research interests include tumor gene research and precision medicine.
Yanfang Jiang
Yanfang Jiang received the B.S. degree in Medicine and Japanese from Norman Bethune Medical University. Changchun. China, in 1995 and the M.S. degree in Medicine from Norman Bethune Medical University. Changchun. China, in 1998. She received the Ph.D. degree in Medicine from Jilin University. Changchun. China in 2005. Her research interests include precision medicine, molecular diagnosis and immunology.
References
- Zhang Y. Epidemiology of esophageal cancer. World J Gastroenterol. 2013;19(34):5598–11. doi:10.3748/wjg.v19.i34.5598.
- Rustgi AK, El-Serag HB, Ingelfinger JR. Esophageal carcinoma. N Engl J Med. 2014;371(26):2499–2509. doi:10.1056/NEJMra1314530.
- Chen W. 2015. Cancer statistics: updated cancer burden in China. Chin J Cancer Res. 27:1.
- Janjigian YY, Bendell J, Calvo E, Kim JW, Ascierto PA, Sharma P, Ott PA, Peltola K, Jaeger D, Evans J, et al. CheckMate-032 study: efficacy and Safety of Nivolumab and Nivolumab plus ipilimumab in patients with metastatic esophagogastric cancer. J Clin Oncol. 2018;36(28):2836–2844. doi:10.1200/JCO.2017.76.6212.
- Massari F, Santoni M, Ciccarese C, Santini D, Alfieri S, Martignoni G, Brunelli M, Piva F, Berardi R, Montironi R, et al. PD-1 blockade therapy in renal cell carcinoma: current studies and future promises. Cancer Treat Rev. 2015;41(2):114–121. doi:10.1016/j.ctrv.2014.12.013.
- Wu Z, Man S, Sun R, Li Z, Wu Y, Zuo D. Recent advances and challenges of immune checkpoint inhibitors in immunotherapy of non-small cell lung cancer. Int Immunopharmacol. 2020;85:106613. doi:10.1016/j.intimp.2020.106613.
- Mahoney KM, Freeman GJ, McDermott DF. The next immune-checkpoint inhibitors: PD-1/PD-L1 blockade in melanoma. Clin Ther. 2015;37(4):764–782. doi:10.1016/j.clinthera.2015.02.018.
- Keir ME, Butte MJ, Freeman GJ, Sharpe AH. PD-1 and its ligands in tolerance and immunity. Annu Rev Immunol. 2008;26(1):677–704. doi:10.1146/annurev.immunol.26.021607.090331.
- FDA approves pembrolizumab for advanced esophageal squamous cell cancer.
- FDA granted accelerated approval to pembrolizumab (KEYTRUDA, Merck & Co.) in combination with chemotherapy for the treatment of patients with locally recurrent unresectable or metastatic triple-negative breast cancer (TNBC) whose tumours express PD-L1 (CPS ≥10) as determined by an FDA approved test.
- FDA approved pembrolizumab (Keytruda, Merck and Co. Inc.) for patients with recurrent or metastatic cervical cancer with disease progression on or after chemotherapy whose tumours express PD-L1 (CPS ≥1) as determined by an FDA-approved test.
- Kudo T, Hamamoto Y, Kato K, Ura T, Kojima T, Tsushima T, Hironaka S, Hara H, Satoh T, Iwasa S, et al. Nivolumab treatment for oesophageal squamous-cell carcinoma: an open-label, multicentre, phase 2 trial. Lancet Oncol. 2017;18(5):631–639. doi:10.1016/S1470-2045(17)30181-X.
- Shah MA, Kojima T, Hochhauser D, Enzinger P, Raimbourg J, Hollebecque A, Lordick F, Kim S-B, Tajika M, Kim HT, et al. Efficacy and Safety of pembrolizumab for heavily pretreated patients with advanced, metastatic adenocarcinoma or squamous cell carcinoma of the esophagus: the phase 2 KEYNOTE-180 study. JAMA Oncol. 2019;5(4):546–550. doi:10.1001/jamaoncol.2018.5441.
- Huang J, Xu B, Mo H, Zhang W, Chen X, Wu D, Qu D, Wang X, Lan B, Yang B, et al. Safety, activity, and biomarkers of SHR-1210, an Anti-PD-1 antibody, for patients with advanced esophageal carcinoma. Clin Cancer Res. 2018;24(6):1296–1304. doi:10.1158/1078-0432.CCR-17-2439.
- Kojima T, Shah MA, Muro K, Francois E, Adenis A, Hsu CH, Doi T, Moriwaki T, Kim S-B, Lee S-H, et al. Randomized phase III KEYNOTE-181 study of pembrolizumab versus chemotherapy in advanced esophageal cancer. J Clin Oncol. 2020;38(35):4138–4148. doi:10.1200/JCO.20.01888.
- Sun JM, Shen L, Shah MA, Enzinger P, Adenis A, Doi T, Kojima T, Metges J-P, Li Z, Kim S-B, et al. Pembrolizumab plus chemotherapy versus chemotherapy alone for first-line treatment of advanced oesophageal cancer (KEYNOTE-590): a randomised, placebo-controlled, phase 3 study. Lancet. 2021;398(10302):759–771. doi:10.1016/S0140-6736(21)01234-4.
- Kato K, Cho BC, Takahashi M, Okada M, Lin CY, Chin K, Kadowaki S, Ahn M-J, Hamamoto Y, Doki Y, et al. Nivolumab versus chemotherapy in patients with advanced oesophageal squamous cell carcinoma refractory or intolerant to previous chemotherapy (ATTRACTION-3): a multicentre, randomised, open-label, phase 3 trial. Lancet Oncol. 2019;20(11):1506–1517. doi:10.1016/S1470-2045(19)30626-6.
- Huang J, Xu J, Chen Y, Zhuang W, Zhang Y, Chen Z, Chen J, Zhang H, Niu Z, Fan Q, et al. Camrelizumab versus investigator’s choice of chemotherapy as second-line therapy for advanced or metastatic oesophageal squamous cell carcinoma (ESCORT): a multicentre, randomised, open-label, phase 3 study. Lancet Oncol. 2020;21(6):832–842. doi:10.1016/S1470-2045(20)30110-8.
- Chalmers ZR, Connelly CF, Fabrizio D, Gay L, Ali SM, Ennis R, Schrock A, Campbell B, Shlien A, Chmielecki J, et al. Analysis of 100,000 human cancer genomes reveals the landscape of tumour mutational burden. Genome Med. 2017;9(1):34. doi:10.1186/s13073-017-0424-2.
- Guo W, Wang P, Li N, Shao F, Zhang H, Yang Z, Li R, Gao Y, He J. Prognostic value of PD-L1 in esophageal squamous cell carcinoma: a meta-analysis. Oncotarget. 2018;9(17):13920–13933. doi:10.18632/oncotarget.23810.
- Gao YB, Chen ZL, Li JG, Hu XD, Shi XJ, Sun ZM, Zhang F, Zhao Z-R, Li Z-T, Liu Z-Y, et al. Genetic landscape of esophageal squamous cell carcinoma. Nat Genet. 2014;46(10):1097–1102. doi:10.1038/ng.3076.
- Rizvi H, Sanchez-Vega F, La K, Chatila W, Jonsson P, Halpenny D, Plodkowski A, Long N, Sauter JL, Rekhtman N, et al. Molecular determinants of response to anti–programmed cell death (PD)-1 and anti–programmed death-ligand 1 (PD-L1) blockade in patients with non–small-Cell lung cancer profiled with targeted next-generation sequencing. J Clin Oncol. 2018;36(7):633–641. doi:10.1200/JCO.2017.75.3384.
- Klein O, Kee D, Markman B, Carlino MS, Underhill C, Palmer J, Power D, Cebon J, Behren A. Evaluation of TMB as a predictive biomarker in patients with solid cancers treated with anti-PD-1/CTLA-4 combination immunotherapy. Cancer Cell. 2021;39(5):592–593. doi:10.1016/j.ccell.2021.04.005.
- Davoli T, Uno H, Wooten EC, Elledge SJ. Tumour aneuploidy correlates with markers of immune evasion and with reduced response to immunotherapy. Science. 2017;355(6322). doi:10.1126/science.aaf8399.
- Roh W, Chen PL, Reuben A, Spencer CN, Prieto PA, Miller JP, Gopalakrishnan V, Wang F, Cooper ZA, Reddy SM, et al. Integrated molecular analysis of tumour biopsies on sequential CTLA-4 and PD-1 blockade reveals markers of response and resistance. Sci Transl Med. 2017. 9(379). doi:10.1126/scitranslmed.aah3560.
- Van Allen EM, Miao D, Schilling B, Shukla SA, Blank C, Zimmer L, Sucker A, Hillen U, Geukes Foppen MH, Goldinger SM, et al. Genomic correlates of response to CTLA-4 blockade in metastatic melanoma. Science. 2015;350(6257):207–211. doi:10.1126/science.aad0095.
- Cristescu R, Mogg R, Ayers M, Albright A, Murphy E, Yearley J, Sher X, Liu XQ, Lu H, Nebozhyn M, et al. Pan-tumor genomic biomarkers for PD-1 checkpoint blockade–based immunotherapy. Science. 2018. 362(6411). doi:10.1126/science.aar3593.
- Weiss GJ, Beck J, Braun DP, Bornemann-Kolatzki K, Barilla H, Cubello R, Quan W, Sangal A, Khemka V, Waypa J, et al. Tumor cell–free DNA copy number instability predicts therapeutic response to immunotherapy. Clin Cancer Res. 2017;23(17):5074–5081. doi:10.1158/1078-0432.CCR-17-0231.
- Woo SR, Turnis ME, Goldberg MV, Bankoti J, Selby M, Nirschl CJ, Bettini ML, Gravano DM, Vogel P, Liu CL, et al. Immune inhibitory molecules LAG-3 and PD-1 synergistically regulate T-cell function to promote tumoural immune escape. Cancer Res. 2012;72(4):917–927. doi:10.1158/0008-5472.CAN-11-1620.
- Lee JS, Ruppin E. Multiomics prediction of response rates to therapies to inhibit programmed cell death 1 and programmed cell death 1 ligand 1. JAMA Oncol. 2019;5(11):1614–1618. doi:10.1001/jamaoncol.2019.2311.
- Washington EN, Placket TP, Gagliano RA, Kavolius J, Person DA. Diffuse plexiform neurofibroma of the back: report of a case. Hawaii Med J. 2010;69(8):191–193.
- Zhang YY, Vik TA, Ryder JW, Srour EF, Jacks T, Shannon K, Wade Clapp D. Nf1 regulates hematopoietic progenitor cell growth and ras signaling in response to multiple cytokines. J Exp Med. 1998;187(11):1893–1902. doi:10.1084/jem.187.11.1893.
- Chen Z, Mo J, Brosseau J-P, Shipman T, Wang Y, Liao C-P, Cooper JM, Allaway RJ, Gosline SJC, Guinney J, et al. Spatiotemporal loss of NF1 in Schwann cell lineage leads to different types of cutaneous neurofibroma susceptible to modification by the hippo pathway. Cancer Discov. 2019;9(1):114–129. doi:10.1158/2159-8290.CD-18-0151.
- Miao J, Hsu PC, Yang YL, Xu Z, Dai Y, Wang Y, Chan G, Huang Z, Hu B, Li H, et al. YAP regulates PD-L1 expression in human NSCLC cells. Oncotarget. 2017;8(70):114576–114587. doi:10.18632/oncotarget.23051.
- Hsu P-O, Miao J, Wang YC, Zhang WQ, Yang YL, Wang CW, Yang C-T, Huang Z, You J, Xu Z, et al. Inhibition of yes-associated protein down-regulates PD-L1 (CD274) expression in human malignant pleural mesothelioma. J Cell Mol Med. 2018;22(6):3139–3148. doi:10.1111/jcmm.13593.
- Johnson DB, Frampton GM, Rioth MJ, Yusko E, Xu Y, Guo X, Ennis RC, Fabrizio D, Chalmers ZR, Greenbowe J, et al. Targeted next generation sequencing identifies markers of response to PD-1 blockade. Cancer Immunol Res. 2016;4(11):959–967. doi:10.1158/2326-6066.CIR-16-0143.
- Zhou Z, Li M. Evaluation of BRCA1 and BRCA2 as indicators of response to immune checkpoint inhibitors. JAMA Netw Open. 2021;4(5):e217728. doi:10.1001/jamanetworkopen.2021.7728.
- Samstein RM, Krishna C, Ma X, Pei X, Lee KW, Makarov V, Kuo F, Chung J, Srivastava RM, Purohit TA, et al. Mutations in BRCA1 and BRCA2 differentially affect the tumour microenvironment and response to checkpoint blockade immunotherapy. Nat Cancer. 2021;1(12):1188–1203. doi:10.1038/s43018-020-00139-8.
- Mariathasan S, Turley SJ, Nickles D, Castiglioni A, Yuen K, Wang Y, Kadel III EE, Koeppen H, Astarita JL, Cubas R, et al. TGFβ attenuates tumour response to PD-L1 blockade by contributing to exclusion of T cells. Nature. 2018;554(7693):544–548. doi:10.1038/nature25501.
- Melisi D, Oh DY, Hollebecque A, Calvo E, Varghese A, Borazanci E, Macarulla T, Merz V, Zecchetto C, Zhao Y, et al. Safety and activity of the TGFβ receptor I kinase inhibitor galunisertib plus the anti-PD-L1 antibody durvalumab in metastatic pancreatic cancer. J Immunother Cancer. 2021;9(3):e002068. doi:10.1136/jitc-2020-002068.
- Yi M, Niu M, Xu L, Luo S, Wu K-O . Regulation of PD-L1 expression in the tumour microenvironment. J Hematol Oncol. 2021;14(1):10. doi:10.1186/s13045-020-01027-5.
- David JM, Dominguez C, McCampbell KK, Gulley JL, Schlom J, Palena C. A novel bifunctional anti-PD-L1/TGF-β trap fusion protein (M7824) efficiently reverts mesenchymalization of human lung cancer cells. OncoImmunology. 2017;6(10):e1349589. doi:10.1080/2162402X.2017.1349589.
- Lu Z, Chen H, Li S, Gong J, Li J, Zou J, Wu L, Yu J, Han W, Sun H, et al. Tumour copy-number alterations predict response to immune-checkpoint-blockade in gastrointestinal cancer. J Immunother Cancer. 2020;8(2):8. doi:10.1136/jitc-2019-000374.
- Zhao R, Choi BY, Lee MH, Bode AM, Dong Z. Implications of Genetic and epigenetic alterations of CDKN2A (p16(INK4a)) in cancer. EBioMedicine. 2016;8:30–39. doi:10.1016/j.ebiom.2016.04.017.
- Sawada G, Niida A, Uchi R, Hirata H, Shimamura T, Suzuki Y, Shiraishi Y, Chiba K, Imoto S, Takahashi Y, et al. Genomic landscape of esophageal squamous cell carcinoma in a Japanese population. Gastroenterology. 2016;150(5):1171–1182. doi:10.1053/j.gastro.2016.01.035.
- Tam KW, Zhang W, Fau - Soh J, Soh J, Fau - Stastny V, Stastny V, Fau - Chen M, Chen M, Fau - Sun H, Sun H, et al. CDKN2A/p16 inactivation mechanisms and their relationship to smoke exposure and molecular features in non–small-Cell lung cancer. J Thorac Oncol. 2013;8(11):1378–1388. doi:10.1097/JTO.0b013e3182a46c0c.
- Zhang J, Bu X, Wang H, Zhu Y, Geng Y, Nihira NT, Tan Y, Ci Y, Wu F, Dai X, et al. Cyclin D–CDK4 kinase destabilizes PD-L1 via cullin 3–SPOP to control cancer immune surveillance. Nature. 2018;553(7686):91–95. doi:10.1038/nature25015.
- DeLeon TT, Almquist D-O, Kipp BR, Langlais BT, Mangold A-O, Winters JL, Kosiorek HE, Joseph RW, Dronca RS, Block MS, et al. Assessment of clinical outcomes with immune checkpoint inhibitor therapy in melanoma patients with CDKN2A and TP53 pathogenic mutations. PloS One. 2020;15(3):e0230306. doi:10.1371/journal.pone.0230306.
- Brenner E, Schörg B-O, Ahmetlić F, Wieder T, Hilke FJ, Simon N, Schroeder C, Demidov G, Riedel T, Fehrenbacher B, et al. Cancer immune control needs senescence induction by interferon-dependent cell cycle regulator pathways in tumours. Nat Commun. 2020. 11(1). doi:10.1038/s41467-020-14987-6.
- Hilke F-O, Sinnberg T-O, Gschwind A-O, Niessner H-O, Demidov G-O, Amaral T-O, Ossowski S, Bonzheim I, Röcken M, Riess O, et al. Distinct mutation patterns reveal melanoma subtypes and influence immunotherapy response in advanced melanoma patients. LID. 2020;12(9):2359. doi:10.3390/cancers12092359.
- Lamberti G, Spurr LF, Li Y, Ricciuti B, Recondo G, Umeton R, Nishino M, Sholl LM, Meyerson ML, Cherniack AD, et al. Clinicopathological and genomic correlates of programmed cell death ligand 1 (PD-L1) expression in nonsquamous non-small-cell lung cancer. Ann Oncol. 2020;31(6):807–814. doi:10.1016/j.annonc.2020.02.017.
- Adib Elio, Nassar Amin H, Akl Elie W, Abou Alaiwi S, Nuzzo PV, Mouhieddine TH, Sonpavde G, Haddad RI, Mouw KW, Giannakis M, et al. CDKN2A alterations and response to immunotherapy in solid tumours. Clin Cancer Res. 2021:27(14):4025–4035. doi:10.1158/1078-0432.CCR-21-0575.
- Li F, Li C, Cai X, Xie Z, Zhou L, Cheng B, Zhong R, Xiong S, Li J, Chen Z, et al. The association between CD8+ tumour-infiltrating lymphocytes and the clinical outcome of cancer immunotherapy: a systematic review and meta-analysis. EClinicalMedicine. 2021;41:101134. doi:10.1016/j.eclinm.2021.101134.
- Zhang L, Chen Y, Wang H, Xu Z, Wang Y, Li S, Liu J, Chen Y, Luo H, Wu L, et al. Massive PD-L1 and CD8 double positive TILs characterize an immunosuppressive microenvironment with high mutational burden in lung cancer. J Immunother Cancer. 2021;9(6):e002356. doi:10.1136/jitc-2021-002356.
- Pollari M, Bruck O, Pellinen T, Vahamurto P, Karjalainen-Lindsberg ML, Mannisto S, Kallioniemi O, Kellokumpu-Lehtinen P-L, Mustjoki S, Leivonen S-K, et al. PD-L1 + tumor-associated macrophages and PD-1 + tumor-infiltrating lymphocytes predict survival in primary testicular lymphoma. Haematologica. 2018;103(11):1908–1914. doi:10.3324/haematol.2018.197194.
- Wang J, Browne L, Slapetova I, Shang F, Lee K, Lynch J, Beretov J, Whan R, Graham PH, Millar EKA, et al. Multiplexed immunofluorescence identifies high stromal CD68(+)PD-L1(+) macrophages as a predictor of improved survival in triple negative breast cancer. Sci Rep. 2021;11(1):21608. doi:10.1038/s41598-021-01116-6.