ABSTRACT
With the success of immune checkpoint inhibitors (ICI), such as anti- programmed death-1 (PD-1) antibody for solid tumors and lymphoma immunotherapy, a number of clinical trials with ICIs have been attempted for acute myeloid leukemia (AML) immunotherapy; however, limited clinical efficacy has been reported. This may be due to the heterogeneity of immune microenvironments and various degrees of T cell exhaustion in patients and may be involved in the IFN-γ pathway. In this study, we first characterized the percentage of PD-1+ and T cell immunoglobulin mucin-domain-containing-3 (Tim-3) +IFN-γ+ T cells in peripheral blood (PB) in AML compared with healthy individuals (HIs) by flow cytometry and further discussed the possibility of the reversal of T cell exhaustion to restore the secretion capacity of cytokines in T cells in AML based on blockade of PD-1 or Tim-3 (anti-PD-1 and anti-Tim-3 antibody) in vitro using a cytokine protein chip. A significantly increased percentage of PD-1+, Tim-3+, and PD-1+Tim-3+ IFN-γ+ T cells was observed in PB from patients with AML in comparison with HIs. Moreover, higher PD-1+IFN-γ+CD3+/CD8+ T cell levels were associated with poor overall survival in AML patients. Regarding leukemia cells, the percentage of Tim-3 in CD117+CD34+ AML cells was positively correlated with PD-1 in IFN-γ+CD4+ T cells. Furthermore, blocking PD-1 and Tim-3 may involve multiple cytokines and helper T cell subsets, mainly Th1 and Treg cells. Blockade of PD-1 or Tim-3 tends to restore cytokine secretion to a certain extent, a synergistic effect shown by the co-blockade of PD-1 and Tim-3. However, we also demonstrated the heterogeneity of secretory cytokines in ICI-treated T cells in AML patients.
Introduction
T-cell dysfunction is regarded as an important cause of immune escape in acute myeloid leukemia (AML) and is closely associated with disease progression and prognosis.Citation1,Citation2 The former is mainly reflected in abnormal expression of T cell receptor (TCR), dysregulation of cytokine secretion, imbalance of T cell subsets, aberrant T cell activation, and proliferation, etc.Citation3 It is reported that higher numbers of Treg cells were observed in AML,Citation4 and may suppress cytotoxic T cells through interleukeukin-10 (IL-10), transforming growth factor-β (TGF-β), and IL-35.Citation5 In addition, decreasing IL-2, IL-1β, tumor necrosis factor-α (TNF-α) and IL-6 levels may significantly contribute to T cell exhaustion, the presence of functional T helper (Th) cell populations and the growth and survival of AML cells, which suggests that an imbalance in the cytokine network may be involved in immune escape in AML.Citation6,Citation7 Our previous findings have shown increasing immune checkpoints (IC) such as programmed death-1 (PD-1), cytotoxic T lymphocyte-associated molecule-4 (CTLA-4), and T cell immunoglobulin mucin-domain-containing-3 (Tim-3) in T cell subsets in AML, concurrent with exhaustion phenotypes CD57 and CD244, and mainly in CD8+ T cells.Citation8–12 Moreover, it has been demonstrated that increased PD-1+Vβ+T cells is a common characteristic of AML and related to poor prognosis in AML.Citation13 Thus, blockage of immunosuppressive receptors and ligands may make the possibility to reverse T cell exhaustion and enhance anti-leukemic function in AML.Citation14–16 Nevertheless, immune checkpoint inhibitors (ICI), such as anti-PD-1 antibodies, for AML immunotherapy have been reported to have limited clinical efficacies.Citation17,Citation18 This may be due to the heterogeneity of immune microenvironments and various degrees of T cell exhaustion in different patients.Citation19,Citation20 Clinical trial data have indicated that combined blockage tends to be more effective in reversing T cell exhaustion than single blockage of the PD-1/PD-ligand 1(PD-L1) pathway.Citation21–23 In particular, decreased PD-1 and Tim-3 double-positive T cells were observed in AML-complete remission (CR) patients compared with AML-non-CR (NCR) patients, indicating that Tim-3 may participate in drug resistance to anti-PD-1.Citation24,Citation25 Further verification has demonstrated synergistic anti-leukemia function in the co-blockage of PD-1 and Tim-3 in clinical trials for solid tumors and AML mouse models.Citation26,Citation27 Furthermore, current findings have indicated that the drug resistance resulting from ICIs is related to the IFN-γ pathway.Citation28 On the one hand, IFN-γ plays a positive immunomodulatory role in the upregulation of major histocompatibility complex I (MHC-I) and antigen presentation by dendritic cells (DC) through PI3K-AKT and JAK2-STAT1 pathways.Citation28 On the other hand, IFN-γ takes part in the anti-tumor process by downregulation of PD-L1 in AML cells.Citation29 In general, the IFN-γ pathway plays a critical role in the leukemia effect.Citation30 However, little is known about the exhaustion status and distribution of IFN-γ+ T cells and their role in AML. In this study, we first characterized the percentage of PD-1+ and Tim-3+ IFN-γ+ T cells and further discussed the T cell exhaustion reversal to restore the secretion capacity of cytokines in T cells by blocking PD-1 and Tim-3 in vitro in AML.
Results
Increasing PD-1 and Tim-3 expression on IFN-γ+ T cell subsets in AML
Previous studies have reported higher expression of PD-1 and Tim-3 in T cells from AML patients with an exhausted phenotype.Citation8,Citation9 In this study, we first analyzed CD3, CD4, CD8, IFN-γ, PD-1, and Tim-3 expression in total CD3+ T cells in peripheral blood (PB) from newly diagnosed AML patients and found an obvious increasing trend toward PD-1+ and Tim-3+ T cells in AML in comparison with HIs, with decreasing IFN-γ+ T cells in AML (). Simultaneously, we detected the frequencies of PD-1 and Tim-3 expression and co-expression in IFN-γ+ T cell subsets (). Significantly, a higher percentage of PD-1+IFN-γ+CD3+ (median: 24.9 vs 10.5, P < .001), Tim-3+IFN-γ+CD3+ (median: 4.94 vs 1.69, P < .001), and PD-1+Tim-3+IFN-γ+CD3+ T cells (median: 3.23 vs 0.33, P = .001) was found in patients with AML than in those with HIs (). Similarly, increasing percentage of PD-1+, Tim-3 and PD-1+Tim-3+ T cells were shown in IFN-γ+CD4+ T (Th1) cells (median: 34.9 vs 12.4, P < .001) (median: 5.24 vs 1.43, P < .001) (median: 3.21 vs 0.45, P < .001) and IFN-γ+CD8+ T (Tc1) cells (median: 21.9 vs 13.0, P = .004) (median: 3.11 vs 1.34, P < .001) (median: 0.91 vs 0.18, P = .001) (). In addition, comparing the different patterns of PD-1 and Tim-3 expression in Th1 and Tc1 cells, we found that the percentage of PD-1+Tim-3+ and Tim-3-PD-1+ T cells tended to be higher in Th1 cells than in Tc1 cells (). Furthermore, we analyzed the correlation between the percentages of PD-1 and Tim-3 in IFN-γ+ T cell subsets in AML (). The results demonstrated that PD-1 was positively correlated with Tim-3 expression in IFN-γ+CD3+ T (rs = 0.393, P = .035) and IFN-γ+CD4+ T cells (rs = 0.398, P = .033), whereas there was no statistically significant difference in IFN-γ+CD8+ cells (rs = 0.087, P = .653).
Figure 1. Increasing PD-1 and Tim-3 on IFN-γ+ T cell subsets in PB in AML.
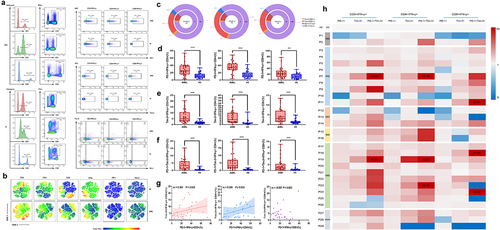
Our previous studies have found that varying degrees of T cell immunodeficiency exist in patients with AML of different subtypes.Citation4 In this study, we characterized the distribution of PD-1 and Tim-3 on IFN-γ+ T cells in 29 patients with AML. According to the heatmap made by taking the median of the HI group as the standard and then standardizing the patient data, a higher tendency of PD-1+, Tim-3+, and PD-1+Tim-3+ on IFN-γ+ T cells, especially Th1 cells, was detected in the M2 and M5 groups, followed by the M0 and M3 groups in comparison with HIs (), indicating the heterogeneity of PD-1 and Tim-3 expression on IFN-γ+ T cell subsets in AML.
Higher expression of PD-1 and Tim-3 on IFN-γ+ T cells resulting in inferior prognosis in AML
To evaluate the relationship between PD-1 and Tim-3 expression and AML prognosis, we compared the differences in the distribution of IFN-γ+ T cell subsets between the AML-CR and AML-NCR groups after chemotherapy. A persistent, lower skewed PD-1+ IFN-γ+CD3+, Tim-3+ IFN-γ+CD3+ and PD-1+Tim-3+IFN-γ+CD3+ T cell distributions were demonstrated for AML-CR patients (median: 13.6 vs. 35.9, P = .012) (median: 2.18 vs. 6.48, P = .036) (median: 1.22 vs. 4.55, P = .046) (). Interestingly, we found a significantly decreased PD-1+ T cell percentage in IFN-γ+CD4+ T cells in the AML-CR group compared to the AML-NCR group (median: 10.3 vs. 25.3, P = .022) (), while the differences were not statistically significant in PD-1+IFN-γ+CD8+ T cells (median: 21.2 vs. 43.5, P = .072) (), which may be related to the immune suppression of chemotherapy. We further analyzed the relationship between the expression of PD-1/Tim-3 and achieving CR after first treatment through a receiver operating characteristic (ROC) curve and observed that increasing expression of PD-1+IFN-γ+CD3+/CD4+/CD8+ T cells showed a high probability for de novo AML patients to achieve CR after the first chemotherapy treatment (Area Under Curve (AUC) = 0.786, P = .043) (AUC = 0.833, P = .018) (AUC = 0.798, P = .035) ().
Figure 2. Higher PD-1 and Tim-3 on IFN-γ+ T cell subsets are related with poor prognosis in AML.
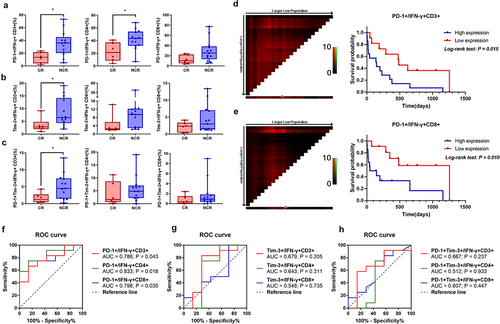
We have gone a step further to follow up the survival status of 29 newly diagnosed patients with AML since their diagnosis, with four cases lost. Subsequently, the Kaplan–Meier survival curve and log-rank analysis showed that a higher number of PD-1+IFN-γ+CD3+ (X2 = 5.892, P = .015) () and PD-1+IFN-γ+CD8+ T cells (X2 = 6.623, P = .015) () indicated an inferior prognosis.
Tim-3 in AML cells positively correlated with PD-1 in IFN-γ+CD4+ T cells in AML
It is well known that the ligands of PD-1 are PD-L1 and PD-ligand 1 (PD-L2) and the ligand of Tim-3 is Gal-9. To discuss the characteristics of immunosuppressive ligands in AML tumor cells, we determined the percentage of PD-L1, PD-L2, Gal-9, and Tim-3 in CD117+CD34+ and CD117+HLA-DR+ AML cells (). The frequency of PD-L1+/PD-L2+/Gal-9+/Tim-3+CD117+CD34+ AML cells in PB was relatively different, ranging from 0.85% to 32.5% for PD-L1, 0.21%–7.53% for PD-L2, 2.94%–99.1% for Gal-9, and 0.61%–17.3% for Tim-3. In this study, we had not found the significant difference of ligand expression in AML cells in PB and BM (). We further followed up patients who achieved CR after the first treatment and analyzed the difference between the AML-CR and NCR groups in PB. However, there was no significant difference in the expression of PD-L1/PD-L2/Gal-9/Tim-3 between the CR and NCR groups (). In addition, we obtained a step further to establish correlations between ligands in AML cells and receptors in T cells. Interestingly, a positive correlation was found between Tim-3 in CD117+CD34+ AML cells and PD-1 in IFN-γ+CD4+ T cells (rs = 0.572, P = .022), while no correlation was found between Tim-3 in CD117+CD34+ AML cells and PD-1 in CD4+ T cells (rs = 0.056, P = .819) ().
Figure 3. Distribution and frequency of PD-L1/PD-L2/Gal-9/Tim-3 in AML cells in PB and BM.
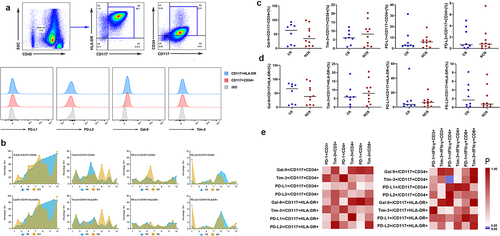
Blockade of PD-1 or Tim-3 tends to enhance the secretion of cytokines in vitro in AML
As described above, the higher expression of PD-1 and Tim-3 in T cell subsets may be associated with T cell exhaustion and disease prognosis. It is unclear whether PD-1 and Tim-3 inhibitors can restore anti-leukemia function in T cells. We further performed a cytokine protein chip analysis of the supernatant of T cells and the AML cell co-culture system treated with or without anti-PD-1 or anti-Tim-3 in three samples from AML patients (AML#1 BM, AML#2 BM, AML#3 BM, and AML#3 PB). The characteristics of the 24 cytokines in the three BM samples are shown in . It was also found that blockade of PD-1 or Tim-3 may bring about a change in different types of cytokines or variable quantities, which demonstrated heterogeneity of the secretory cytokines in ICI-treated T cells in AML (). We found correlations among cytokines in groups treated with ICI and observed that IL-10 was positively correlated with TGF-β, which was reported to be negatively regulated by cytokines. Moreover, strong positive correlations were observed between IL-17, TNF-β, IL-13, IL-2, IL-4, and IFN-γ (). In addition, we divided T cell subsets into Th1 (IL-2, IFN-γ, TNF-α, and TNF-β), Th2 (IL-4, IL-5, and IL-13), Th17 (IL-17 and IL-22), follicular helper (Tfh) cells (IL-21), and Treg (IL-10 and TGF-β) according to the cytokines and found that anti-PD-1 and anti-Tim-3 inhibitors may be mainly affected by Th1 and Treg cell functions (). We further performed an individual analysis and found that blocking PD-1 or Tim-3 can enhance the secretion of cytokines to a certain extent (). As for AML#1, increased secretion levels of IFN-γ, IL-1β, IL-6, and macrophage inflammatory protein 3α (MIP-3α) were observed in the anti-PD-1 group compared to those in the anti-PD-1-ISO group (P = .008; P = .001; P = .001; P < .001); increased secretion levels of IFN-γ, IL-6, IL-17, TNF-α, and MIP-3α were observed in the anti-Tim-3 group compared to those in the anti-Tim-3-ISO group (P < .001; P = .003; P = .001; P = .034; P = .017). Interestingly, the co-blockade of PD-1 and Tim-3 synergistically enhanced the cytokine secretion of IFN-γ, TNF-α, and IL-17 (P < .001; P = .011; P < .001) (). A similar trend was observed for the secretory cytokines in AML#2 (). In addition, higher levels of IL-21 (P = .003), IL-2 (P < .001), TNF-β (P = .007), IL-5 (P < .001), and TNF-α (P = .004), as well as lower levels of IL-10 (P < .001) were observed in AML#3-BM than in AML#3-PB co-cultured with AML cells and treated with ICI in vitro (Supplementary Figure S1A-B).
Figure 4. The characteristics of the secretory cytokines in ICI-treated T cells in vitro detected by protein chip.
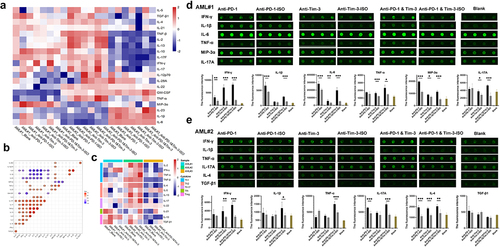
Discussion
Helper T cell function may be compromised in AML, and several observational studies have found that Th1 populations have a decreased frequency and lower expression of IFN-γ in both the PB and BM of AML patients.Citation3,Citation31 In this study, we presented t-SNE visualizations that illustrated generally lower IFN-γ expression and higher PD-1 and Tim-3 expression in AML than in HIs, which was consistent with previous reports.Citation32 In order to better evaluate the immune function status of T cells in AML, we characterized PD-1 and Tim-3 expression on IFN-γ+ T cell subsets and found increasing PD-1+, Tim-3+, PD-1, and Tim-3 double positive T cells in IFN-γ+CD3+/CD4+/CD8+ T cells in AML, illustrating that T cell exhaustion is obviously present in both Th1 and Tc1 cells and is associated with leukemia. Our previous findings have reported an increasing trend toward PD-1+CD4+ T cells in PB in AML.Citation8 Interestingly, a higher percentage of PD-1+IFN-γ+CD4+ T cells was found, indicating that PD-1/PD-L1 pathways may impact CD4+ T cell function through the tumor-infiltrating inflammatory cytokines IFN-γ.Citation33 Further comparative analysis of different patterns of PD-1 and Tim-3 on IFN-γ+ T cells found that PD-1 and Tim-3-mediated T cell exhaustion were principally involved in Th1 rather than Tc1, with Tim-3 mainly in the form of co-expression with PD-1. The former was once more time to be attested in the positive relationship between PD-1 and Tim-3 in Th1, but not in Tc1, suggesting that PD-1 and Tim-3 are involved in the negative immunoregulation of IFN-γ+ T cells, especially Th1 cells in AML. In addition, the distribution of PD-1 and Tim-3 on IFN-γ+ T cells varied in different AML subsets, emphasizing the phenotypic heterogeneity of T cell exhaustion in AML patients.Citation3 It is well known that one of the important aspects of clinical practice is to evaluate the risk of disease. In this study, according the follow-up data, we have pointed out that PD-1/Tim-3 on IFN-γ+ T cells may be an independent risk factor for AML patients whether they achieved CR after chemotherapy. Higher PD-1 expression in IFN-γ+ T cell subsets is associated with poor overall survival in patients with AML, providing evidence for the possibility of reserving T cell exhaustion based on blockage of PD-1 and Tim-3, which may restore the secretion capacity of cytokines in Th cells.
It has been reported that Tim-3 is not only expressed on immune cells, particularly T cells and NK cells, but is also expressed on leukemic stem cells (LSCs) in AML, which plays a double immunosuppressive role in the anti-tumor response.Citation34,Citation35 In this study, we also detected PD-L1/PD-L2/Gal-9/Tim-3 expression in primary AML cells; however, we had not found the significant difference of ligand expression in AML cells in PB, and there was no significant difference of IC ligand expression between samples from PB and BM, even we know that leukemia bone marrow as tumor microenvironment may play immune suppression which may enhance the IC and ligand expression. In this study, we are unable to evaluate the result, one of the reasons may be due to the limit sample size to make effective comparison, we will continue to collect more AML samples to confirm the result.Citation24 Overall, on the other hand, the positive correlation between PD-1+IFN-γ+CD4+ T cells and Tim-3+CD117+CD34+ AML cells demonstrated that PD-1 in Th1 cells may synergistically promote T cell exhaustion with Tim-3 in AML cells, which was indirectly supported by Silva et al.Citation36
Thus, we tried to reverse T cell exhaustion by blocking or co-blocking PD-1 and Tim-3 in primary AML patients in vitro. The protein chip demonstrated the heterogeneity of secretory cytokines in ICI-treated T cells in AML, which may depend on the expression of ICs and individualized tumor immune microenvironments.Citation1,Citation20 We make inferences based on changes in cytokines and find that blocking PD-1 and Tim-3 may involve numerous T cell subsets, mainly on Th1 and Tregs, which, respectively, play a positive and negative role in the regulation of immunity. Binder et al. illustrated that anti-inflammatory mediators such as TGF-β and IL-10 appear to impede AML progression, while pro-inflammatory mediators such as IL-1β, TNF-α, and IL-6 tend to increase AML aggressiveness,Citation6 which is consistent with the results reported in this study. Moreover, it is an indirect proof that the reversal of PD-1-mediated T-cell exhaustion in AML helps restore the secretory capacity of IL-2, IFN-γ, and TNF-α.Citation37 In this study, the Blockade of PD-1 or Tim-3 tended to enhance cytokine secretion to a certain extent, with a synergistic effect (generally TNF-α, IFN-γ, and IL-17A) shown in the co-blockade of PD-1 and Tim-3. Nevertheless, the limitations of the synergistic effects resulting from co-blockage, such as IL-1β, require a large number of investigations to explore the underlying mechanism. At present, the preclinical experiments and clinical trials of ICI for solid tumors and hematological malignancies have emerged drug resistance and the clinical efficiency seemed relative heterogeneity in different individuals from the same disease, such as AML and MDS, the main reason may be related to different immune suppression status in patients.Citation38 It is reported that dysregulated cytokine expression, which is a general hallmark of chronic inflammation and auto-inflammatory diseases, may also promote the development of hematological malignancies.Citation6 Current findings have revealed that the drug resistance resulting from ICIs is related to the IFN-γ pathway that IFN-γ takes part in the anti-tumor process by downregulation of PD-L1 in AML cells.Citation30 Moreover, Tim-3 is a molecule originally identified as being selectively expressed on IFN-γ–secreting Th1 and Tc1 cells.Citation39 These indicated that the possibility of combinational approaches to enhancing PD-L1/PD-1 pathways blockade efficacy with several cytokines such as IL-2, IL-10, and IFN-α may result in additional benefits for AML patient immunotherapy based on IC blockade.Citation40
Conclusion
In conclusion, we first made a novel observation that increased PD-1 and Tim-3 expression on IFN-γ+ T cells may contribute to T cell exhaustion and impair anti-leukemia function and is associated with poor prognosis in AML. Co-blockade of PD-1 and Tim-3 synergistically enhanced cytokine secretion based on the distribution of ICs.
Methods
Samples
PB samples for detecting PD-1 and Tim-3 on IFN-γ+ T cells were collected from 29 de novo AML patients, including 17 males and 12 females (median age: 52 years, range: 23–81 years) named P1 to P29, as well as 29 PB in healthy individuals (HIs), including 17 males and 12 females (median age: 44 years, range: 22–81 years) served as controls. In addition, 23 PB samples (male: female = 12:11, median age: 44 years, range: 14–83 years) from de novo AML patients were collected to detect the expression of PD-L1/programmed death-ligand 2 (PD-L2)/Gal-9/Tim-3 from AML cells, named P1, P2, P9-P13, P16, and P22–P36, as well as 13 BM samples from matched AML patients. Finally, three samples, P2, P37, and P38, were used to perform cytokine protein chip analysis after treatment with ICI in vitro. Clinical data of the patients are presented in Supplementary Table S1. All human samples were obtained with informed consent, and ethical approval was obtained from the Ethics Committee of the Medical School of Jinan University.
Flow cytometry analysis
First, mononuclear cells were separated from fresh blood samples. Cells were stimulated with PMA/ionomycin (CS1002, Multisciences, China) and Brefeldin A (BFA) (00–4975, eBioscience, USA) and cultured at 37°C in 5% CO2 for 5 h. The cells were then stained with CD45, CD3, CD4, CD8, PD-1, and Tim-3 antibodies for 15 min at room temperature in the dark. The cells were washed twice with Phosphate Buffered Saline (PBS), fixed with Fixation Buffer (420801, BioLegend, USA) in the dark for 15 min, permeabilized with 1X Intracellular Staining Perm Wash Buffer (421002, BioLegend, USA) twice, and then intracellularly stained for IFN-γ in the dark for 15 min. Finally, 30,000 CD3+ cells were acquired for analysis with a BD FACS Canto flow cytometer (BD Biosciences, San Jose, USA) and subsequent analysis using Flowjo software (Flowjo LLC, USA).
Meanwhile, 100 μL of BM or PB blood samples were collected and mixed with Red Blood Cell Lysis Buffer in the dark at room temperature for 8 min. Cell surface staining analysis for CD45, HLA-DR, CD117, CD34, PD-L1, PD-L2 Tim-3, and corresponding isotypes (ISO) and intracellular staining analysis for Gal-9 were performed. The expression was detected and analyzed using flow cytometry and FlowJo software, as mentioned above. The antibodies used in this study are listed in Supplementary Table S2.
Protein chip
CD3+ T cells were obtained by magnetic bead sorting and co-cultured with AML (CD3-) cells at a ratio of 1:10. The cells were divided into groups and treated with Anti-PD-1 (A2002, Selleck, USA), Anti-PD-1-ISO (403702, BioLegend, USA), Anti-Tim-3 (345004, BioLegend, USA), Anti-Tim-3-ISO (400124, BioLegend, USA), Anti-PD-1/Tim-3 and Anti-PD-1/Tim-3-ISO at 37°C in 5% CO2 for 48 h. The cellular supernatant was collected and 24 cytokines were detected using a RayBiotech human GSH-TH17-1 antibody array.
Statistical analysis
Data analyses were performed using the Mann–Whitney test with SPSS software (version 22.0, IBM, Armonk, NY, USA), GraphPad Prism (version 8.4.2, CA, USA), and R (version 3.6.1, https://www.r-project.org/). Correlations were analyzed using Spearman’s correlation analysis. The frequencies of different T-cell subsets are presented as medians. According to optimal cutoff values, PD-1/Tim-3 expression was divided into high and low expression groups. Kaplan–Meier analysis was used to analyze the expression of ICs and the survival of patients with AML. Moreover, the heatmap was using “Pheatmap” in R software and a correlation heatmap was generated using the “ggcorrplot” package. Differences were considered statistically significant at P < .05.
List of abbreviations
AML | = | acute myeloid leukemia |
AUC | = | Area Under Curve |
BM | = | bone marrow |
CR | = | complete remission |
CTLA-4 | = | cytotoxic T lymphocyte-associated molecule-4 |
DC | = | dendritic cell |
Gal-9 | = | galectin 9 |
His | = | healthy individuals |
IC | = | Immune checkpoint |
ICI | = | Immune checkpoint inhibitor |
IFN-γ | = | interferon-γ |
IL-10 | = | interleukin 10 |
ISO | = | Isotypes |
PB | = | peripheral blood |
PBS | = | Phosphate Buffered Saline |
PD-1 | = | programmed cell death receptor-1 |
PD-L1 | = | programmed death-ligand 1 |
PD-L2 | = | programmed death-ligand 2 |
LAG-3 | = | T cell lymphocyte activation gene-3 |
MHC-I | = | major histocompatibility complex I |
MIP-3α | = | macrophage inflammatory protein 3 α |
NCR | = | non complete remission |
ROC | = | receiver operating characteristic |
TCR | = | T cell receptor |
Tfh | = | Follicular helper cells |
TGF-β | = | transforming growth factor-β |
Th cells | = | T helper cells |
Tim-3 | = | T cell immunoglobulin mucin-domain-containing-3 |
TNF-α | = | tumor necrosis factor-α |
Treg | = | regulatory T. |
Author contribution
Yangqiu Li and Shaohua Chen contributed to concept development and study design. Shuxin Huang and Yujie Zhao performed experiments. Xianfeng Zha, Jiaxiong Tan and Xue Zheng collected clinical data. Shuxin Huang and Wenpu Lai contributed to the data analysis and figure preparation. Yangqiu Li, Shaohua Chen and Shuxin Huang drafted the manuscript. All authors have read and approved the final manuscript.
Availability of data and materials
The materials supporting the conclusions of this research article are included within the article.
Supplemental Material
Download Zip (33.5 KB)Acknowledgments
We want to thank the flow facility of the Analysis and Testing Center at Jinan University as well as the research assistant Mr. Xinqiang Lai, who helped arrange the study. We would also like to thank the volunteers who donated blood for this study.
Disclosure statement
No potential conflict of interest was reported by the author(s).
Supplementary material
Supplemental data for this article can be accessed online at https://doi.org/10.1080/15384047.2023.2278229
Additional information
Funding
Notes on contributors
Shuxin Huang
Shuxin Huang received her bachelor's and master's degree from Jinan University in 2018 and 2021. Now, she studies a Doctoral degree in Medical Science as a major at Jinan University. Her research focuses on T cell immunity and immunotherapy in hematologic malignancies.
Yujie Zhao
Yujie Zhao received her master's degree from Jinan University in 2022. After graduation, she worked as a doctor in the First Affiliated Hospital of Xinxiang Medical University. At present, she is pursuing the Doctoral degree in Medical Science at Capital Medical University with a major focus on the mechanisms and treatment of hemophagocytic lymphohistiocytosis.
Wenpu Lai
Wenpu Lai obtained his bachelor's and master's degrees from Guangdong Medical University and Guangzhou Medical University in 2017 and 2020, respectively. He is currently pursuing his Ph.D. in Jinan University. His primary research interests include bioinformatics, machine learning, immunosenescence, and tumor immunology. He excels in the analysis of multi-omics sequencing data, biomedical big data, and the development of artificial intelligence models.
Jiaxiong Tan
Jiaxiong Tan received his master's degree from Jinan University in 2018. After graduation, he worked as a doctor in the Department of Hematology in the the First Affiliated Hospital of Jinan University. At present, he is pursuing the Doctoral degree in Medical Science at Tianjin Medical University with a major focus on immunopathogenesis and immunotherapy for malignant tumors.
Xue Zheng
Xue Zheng received her Bachelor in 2015 from Southern Medical University, and Doctoral degree in 2021 from Sichuan University. Currently, she is a doctor in the Department of Hematology in the First Affiliated Hospital of Jinan University. Her research focuses on the basic and clinical research of acute leukemia.
Xianfeng Zha
Xianfeng Zha is a deputy chief technician of Department of Clinical Laboratory First Affiliated Hospital, Jinan University. He received his Bachelor in 2005 from Anhui Medical University and Doctoral degree in 2012 from Jinan University. His major scientific interests include anti-leukemia cellular immune based on T cell repertoire characterization and molecular characterization of gene alterations in hematopoietic malignancies.
Yangqiu Li
Yangqiu Li is a Professor of Medicine and the Director of Institute of Hematology, School of Medicine, and PI of Key Laboratory for Regenerative Medicine of Ministry of Education, Jinan University. She received her Bachelor in 1986, Master in 1989 from School of Medicine of Jinan University and Dr. med in 1997 from Humboldt University, Berlin, Germany. Her major scientific interests include T cell immunosuppressive and immune reconstruction, anti-leukemia cellular immune based on T cell repertoire characterization, and molecular characterization of gene alterations and targeted therapy in T cell malignancies.
Shaohua Chen
Shaohua Chen is a Professor of Institute of Hematology of School of Medicine, and Key Laboratory for Regenerative Medicine of Ministry of Education, Jinan University. She received her master’s degree from School of Medicine of Jinan University in 2009. Her research focuses on T cell immunosuppressive and immunotherapy in hematologic malignancies.
References
- Daver N, Alotaibi AS, Bücklein V, Subklewe M. T-cell-based immunotherapy of acute myeloid leukemia: current concepts and future developments. Leukemia. 2021 05 07;35(7):1843–9. doi:10.1038/s41375-021-01253-x.
- Wang M, Bu J, Zhou M, Sido J, Lin Y, Liu G, Lin Q, Xu X, Leavenworth JW, Shen E. CD8(+)T cells expressing both PD-1 and TIGIT but not CD226 are dysfunctional in acute myeloid leukemia (AML) patients. Clin Immunol. 2018;190:64–73. 2017 09 13. doi:10.1016/j.clim.2017.08.021.
- Li Z, Philip M, Ferrell PB. Alterations of T-cell-mediated immunity in acute myeloid leukemia. Oncogene. 2020 03 05;39(18):3611–3619. doi:10.1038/s41388-020-1239-y.
- Huang S, Liang C, Zhao Y, Deng T, Tan J, Zha X, Li Y, Chen S. Increased TOX expression concurrent with PD-1, Tim-3, and CD244 expression in T cells from patients with acute myeloid leukemia. Cytometry B Clin Cytom. 2022;102(2):143–152. 2021 12 17. doi:10.1002/cyto.b.22049.
- Chen X, Du Y, Lin X, Qian Y, Zhou T, Huang Z, CD4+CD25+ regulatory T cells in tumor immunity. Int Immunopharmacol. 2016 03 20;34:244–249. doi:10.1016/j.intimp.2016.03.009.
- Binder S, Luciano M, Horejs-Hoeck J. The cytokine network in acute myeloid leukemia (AML): a focus on pro- and anti-inflammatory mediators. Cytokine Growth Factor Rev. 2018 09 06;43:8–15. doi:10.1016/j.cytogfr.2018.08.004.
- Luciano M, Krenn PW, Horejs-Hoeck J. The cytokine network in acute myeloid leukemia. Front Immunol. 2022 10 18;13:1000996. doi:10.3389/fimmu.2022.1000996.
- Tan J, Chen S, Lu Y, Yao D, Xu L, Zhang Y, Yang L, Chen J, Lai J, Yu Z, et al. Higher PD-1 expression concurrent with exhausted CD8+ T cells in patients with de novo acute myeloid leukemia. Chin J Cancer Res. 2017 11 17;29(5):463–470. doi:10.21147/j.issn.1000-9604.2017.05.11.
- Tan J, Huang S, Huang J, Yu Z, Chen Y, Lu Y, Li Y, Chen S. Increasing Tim-3+CD244+, Tim-3+CD57+, and Tim-3+PD-1+ T cells in patients with acute myeloid leukemia. Asia Pac J Clin Oncol. 2020 02 08;16(3):137–141. doi:10.1111/ajco.13304.
- Chen Y, Tan J, Huang S, Huang X, Huang J, Chen J, Yu Z, Lu Y, Weng J, Du X, et al.Higher frequency of the CTLA-4+LAG-3 + T-cell subset in patients with newly diagnosed acute myeloid leukemia. Asia Pac J Clin Oncol. 2020;16(2):e12–e18. 2019 10, 16. doi:10.1111/ajco.13236.
- Zhao Y, Liao P, Huang S, Deng T, Tan J, Huang Y, Zhan H, Li Y, Chen S, Zhong L. Increased TOX expression associates with exhausted T cells in patients with multiple myeloma. Exp Hematol Oncol. 2022;11(1):12. 2022 03, 6. doi:10.1186/s40164-022-00267-0.
- Chen C, Li Y. Predictive value of co-expression patterns of immune checkpoint molecules for clinical outcomes of hematological malignancies. Chin J Cancer Res. 2023 07 13;35(3):245–251. doi:10.21147/j.issn.1000-9604.2023.03.04.
- Huang J, Tan J, Chen Y, Huang S, Xu L, Zhang Y, Lu Y, Yu Z, Chen S, Li Y. A skewed distribution and increased PD-1+Vβ+CD4+/CD8+ T cells in patients with acute myeloid leukemia. J Leukoc Biol. 2019 05 29;106(3):725–732. doi:10.1002/JLB.MA0119-021R.
- Abbas HA, Hao D, Tomczak K, Barrodia P, Im JS, Reville PK, Alaniz Z, Wang W, Wang R, Wang F, et al. Single cell T cell landscape and T cell receptor repertoire profiling of AML in context of PD-1 blockade therapy. Nat Commun. 2021 10, 20;12(1):6071. doi:10.1038/s41467-021-26282-z.
- Williams KM. Checkpoint-blocked T cells checkmate AML. Blood. 2021 06 11;137(23):3155–3156. doi:10.1182/blood.2021011497.
- Hao F, Sholy C, Wang C, Cao M, Kang X. The role of T cell immunotherapy in acute myeloid leukemia. Cells. 2021 12 25;10(12):3376. doi:10.3390/cells10123376.
- Daver N, Garcia-Manero G, Basu S, Boddu PC, Alfayez M, Cortes JE, Konopleva M, Ravandi-Kashani F, Jabbour E, Kadia T, et al. Efficacy, safety, and biomarkers of response to azacitidine and nivolumab in relapsed/refractory acute myeloid leukemia: a nonrandomized, open-label, phase II study. Cancer Discov. 2019 11 10;9(3):370–383. doi:10.1158/2159-8290.Cd-18-0774.
- Ravandi F, Assi R, Daver N, Benton CB, Kadia T, Thompson PA, Borthakur G, Alvarado Y, Jabbour EJ, Konopleva M, et al. Idarubicin, cytarabine, and nivolumab in patients with newly diagnosed acute myeloid leukaemia or high-risk myelodysplastic syndrome: a single-arm, phase 2 study. Lancet Haematol. 2019 08 12;6(9):e480–e488. doi:10.1016/S2352-3026(19)30114-0.
- Koyama S, Akbay EA, Li YY, Herter-Sprie GS, Buczkowski KA, Richards WG, Gandhi L, Redig AJ, Rodig SJ, Asahina H, et al. Adaptive resistance to therapeutic PD-1 blockade is associated with upregulation of alternative immune checkpoints. Nat Commun. 2016 02 18;7(1):10501. doi:10.1038/ncomms10501.
- Wu K, Feng J, Xiu Y, Li Z, Lin Z, Zhao H, Zeng H, Xia W, Yu L, Xu B, Vδ2 T cell subsets, defined by PD-1 and TIM-3 expression, present varied cytokine responses in acute myeloid leukemia patients. Int Immunopharmacol. 2020 01 20;80:106122. doi:10.1016/j.intimp.2019.106122.
- Liao D, Wang M, Liao Y, Li J, Niu T, A review of efficacy and safety of checkpoint inhibitor for the treatment of acute myeloid leukemia. Front Pharmacol. 2019 06 28;10:609. doi:10.3389/fphar.2019.00609.
- Yang X, Ma L, Zhang X, Huang L, Wei J. Targeting PD-1/PD-L1 pathway in myelodysplastic syndromes and acute myeloid leukemia. Exp Hematol Oncol. 2022 03 04;11(1):11. doi:10.1186/s40164-022-00263-4.
- Abedi Kiasari B, Abbasi A, Ghasemi Darestani N, Adabi N, Moradian A, Yazdani Y, Sadat Hosseini G, Gholami N, Janati S, Combination therapy with nivolumab (anti-PD-1 monoclonal antibody): a new era in tumor immunotherapy. Int Immunopharmacol. 2022 11 05;113:109365. doi:10.1016/j.intimp.2022.109365.
- Tan J, Yu Z, Huang J, Chen Y, Huang S, Yao D, Xu L, Lu Y, Chen S, Li Y. Increased PD-1+Tim-3+ exhausted T cells in bone marrow may influence the clinical outcome of patients with AML. Biomark Res. 2020 02 23;8(1):6. doi:10.1186/s40364-020-0185-8.
- Kong Y, Zhang J, Claxton DF, Ehmann WC, Rybka WB, Zhu L, Zeng H, Schell TD, Zheng H. PD-1(hi)TIM-3(+) T cells associate with and predict leukemia relapse in AML patients post allogeneic stem cell transplantation. Blood Cancer J. 2015 08 01;5(7):e330. doi:10.1038/bcj.2015.58.
- Zhou Q, Munger ME, Veenstra RG, Weigel BJ, Hirashima M, Munn DH, Murphy WJ, Azuma M, Anderson AC, Kuchroo VK, et al. Coexpression of Tim-3 and PD-1 identifies a CD8+ T-cell exhaustion phenotype in mice with disseminated acute myelogenous leukemia. Blood. 2011 03 10;117(17):4501–4510. doi:10.1182/blood-2010-10-310425.
- De Mello RA, Zhu JH, Iavelberg J, Potim AH, Simonetti D, Silva Jr JA, Castelo-Branco P, Pozza DH, Tajima CC, Tolia M, et al. Current and future aspects of TIM-3 as biomarker or as potential targeted in non-small cell lung cancer scope: is there a role in clinical practice? Transl Lung Cancer Res. 2020;9(6):2311–2314. doi:10.21037/tlcr-20-625.
- Kalbasi A, Ribas A. Tumour-intrinsic resistance to immune checkpoint blockade. Nat Rev Immunol. 2020;20(1):25–39. 2019 10, 2. doi:10.1038/s41577-019-0218-4.
- Topalian SL, Drake CG, Pardoll DM. Immune checkpoint blockade: a common denominator approach to cancer therapy. Cancer Cell. 2015;27(4):450–461. 2015 04, 11. doi:10.1016/j.ccell.2015.03.001.
- Corradi G, Bassani B, Simonetti G, Sangaletti S, Vadakekolathu J, Fontana MC, Pazzaglia M, Gulino A, Tripodo C, Cristiano G, et al. Release of IFNγ by acute myeloid leukemia cells remodels bone marrow immune microenvironment by inducing regulatory T cells. Clin Cancer Res. 2022 03 30;28(14):3141–3155. doi:10.1158/1078-0432.Ccr-21-3594.
- Sun YX, Kong HL, Liu CF, Yu S, Tian T, Ma D-X, Ji C-Y. The imbalanced profile and clinical significance of T helper associated cytokines in bone marrow microenvironment of the patients with acute myeloid leukemia. Hum Immunol. 2014;75(2):113–118. 2013 11, 26. doi:10.1016/j.humimm.2013.11.014.
- Jiang W, He Y, He W, Wu G, Zhou X, Sheng Q, Zhong W, Lu Y, Ding Y, Lu Q, et al. Exhausted CD8+T cells in the tumor immune microenvironment: new pathways to therapy. Front Immunol. 2020;11:622509. 2021 02, 27. doi:10.3389/fimmu.2020.622509.
- Abbas HA, Alaniz Z, Mackay S, Cyr M, Zhou J, Issa GC, Alfayez M, Matthews J, Kornblau SM, Jabbour E, et al. Single-cell polyfunctional proteomics of CD4 cells from patients with AML predicts responses to anti–PD-1–based therapy. Blood Adv. 2021 09 24;5(22):4569–4574. doi:10.1182/bloodadvances.2021004583.
- Rezaei M, Tan J, Zeng C, Li Y, Ganjalikhani-Hakemi M, TIM-3 in Leukemia; Immune Response and Beyond. Front Oncol. 2021 10 19;11:753677. doi:10.3389/fonc.2021.753677.
- Hong J, Xia L, Huang Z, Yuan X, Liang X, Dai J, Wu Z, Liang L, Ruan M, Long Z, et al. TIM-3 expression level on AML blasts correlates with presence of core binding factor translocations rather than clinical outcomes. Front Oncol. 2022 05 03;12:879471. doi:10.3389/fonc.2022.879471.
- Gonçalves Silva I, Yasinska IM, Sakhnevych SS, Fiedler W, Wellbrock J, Bardelli M, Varani L, Hussain R, Siligardi G, Ceccone G, et al. 2017 07 29. The Tim-3-galectin-9 secretory pathway is involved in the immune escape of human acute myeloid leukemia cells. EBioMedicine. 22:44–57. doi:10.1016/j.ebiom.2017.07.018.
- Zhong M, Gao R, Zhao R, Huang Y, Chen C, Li K, Yu X, Nie D, Chen Z, Liu X, et al. BET bromodomain inhibition rescues PD-1-mediated T-cell exhaustion in acute myeloid leukemia. Cell Death Disease. 2022 08 03;13(8):671. doi:10.1038/s41419-022-05123-x.
- Wu M, Huang Q, Xie Y, Wu X, Ma H, Zhang Y, Xia Y. Improvement of the anticancer efficacy of PD-1/PD-L1 blockade via combination therapy and PD-L1 regulation. J Hematol Oncol. 2022 03 14;15(1):24. doi:10.1186/s13045-022-01242-2.
- Monney L, Sabatos CA, Gaglia JL, Ryu A, Waldner H, Chernova T, Manning S, Greenfield EA, Coyle AJ, Sobel RA, et al. Th1-specific cell surface protein Tim-3 regulates macrophage activation and severity of an autoimmune disease. Nature. 2002 02 02;415(6871):536–541. doi:10.1038/415536a.
- Rahimi Kalateh Shah Mohammad G, Ghahremanloo A, Soltani A, Fathi E, Hashemy SI. Cytokines as potential combination agents with PD-1/PD-L1 blockade for cancer treatment. J Cell Physiol. 2020 01 24;235(7–8):5449–5460. doi:10.1002/jcp.29491.