ABSTRACT
The antipsychotic drug pimozide has been demonstrated to inhibit cancer. However, the precise anti-cancer mechanism of pimozide remains unclear. The purpose of this study was to investigate the effects of pimozide on human MCF-7 and MDA-MB-231 breast cancer cell lines, and the potential involvement in the RAF/ERK signaling. The effects of pimozide on cells were examined by 4,5-dimethylthiazol-2-yl-3,5-diphenylformazan, wound healing, colony formation, transwell assays, and caspase activity assay. Flow cytometry and acridine orange and ethidium bromide staining were performed to assess changes in cells. Transmission electron microscopy and monodansylcadaverine staining were used to observe autophagosomes. The cyclic adenosine monophosphate was evaluated using the FRET system. Immunohistochemistry, immunofluorescence, RNA interference, and western blot investigated the expression of proteins. Mechanistically, we focus on the RAF1/ERK signaling. We detected pimozide was docked to RAF1 by Schrodinger software. Pimozide down-regulated the phosphorylation of RAF1, ERK 1/2, Bcl-2, and Bcl-xl, up-regulated Bax, and cleaved caspase-9 to induce apoptosis. Pimozide might promote autophagy by up-regulating cAMP. The enhancement of autophagy increased the conversion of LC3-I to LC3-II and down-regulated p62 expression. But mTOR signaling was not involved in promoting autophagy. The knockdown of RAF1 expression induced autophagy and apoptosis in breast cancer cells, consistent with the results of pimozide or sorafenib alone. Blocked autophagy by chloroquine resulted in the impairment of pimozide-induced apoptosis. These data showed that pimozide inhibits breast cancer by regulating the RAF/ERK signaling pathway and might activate cAMP-induced autophagy to promote apoptosis and it may be a potential drug for breast cancer treatment.
Introduction
Breast cancer, a frequently diagnosed cancer in females, has long been one of the leading causes of cancer-related death in women. Breast cancer alone accounts for 25% of all cancer cases and 15% of all cancer deaths among females.Citation1 Approximately 2.1 million females were estimated to be diagnosed with breast cancer worldwide in 2018, accounting for about one-fourth of cancers in females.Citation2 More than 30% of patients with metastatic breast cancer do not respond to first-line chemotherapy based on anthracyclines and taxanes, and their cancers progress in less than a year.Citation3 Since the first report of breast cancer treatment with tamoxifen in 1985, a variety of cancer drugs have been developed for breast cancer treatment.Citation4 In recent years, the repurposing of approved drugs for the treatment of other diseases has shown efficacy as a new therapeutic approach for new drug development in diseases including cancer.Citation5 Several FDA-approved clinical medications have been examined for the treatment of cancer, providing a diverse selection of cancer treatments and better anticancer effects.Citation6 For example, the FDA-approved drugs metformin, aspirin, and sulfonamide have exhibited anti-cancer effects.Citation7–9 The reuse of existing drugs is more advantageous for safety reasons. Therefore, the continued identification of previously approved drugs that show potent anti-cancer effects will enable new strategies for cancer treatment.
Pimozide, as a D2-type dopamine receptor inhibitor, is a typical antipsychotic drug that is commonly used in the treatment of mental disorders. Recent studies have shown that pimozide also inhibits a variety of cancer cells, such as colorectal cancer, human osteosarcoma, and hepatocellular carcinoma.Citation10–12 Pimozide inhibits ERK signaling in human osteosarcoma and can selectively promote autophagy in glioblastoma.Citation11,Citation13 Previous studies demonstrated that RAF1 had clinical value and prognostic significance in non-small cell lung cancer patients, and we found that pimozide down-regulated the phosphorylation of RAF1.Citation14 Bertolesi et al. reported that pimozide inhibits the growth of MCF-7 breast cancer cells via T-type Ca2+ channels and suggested that its growth inhibitory effects may be mediated by additional mechanisms.Citation15 However, the precise mechanism of action of pimozide in breast cancer remains unclear.
Previous studies have demonstrated that RAF1 has anti-apoptotic functions. RAF1 is an important protein that interfaces between the RAS and MEK/ERK signaling pathways. RAF1 also exerts its cellular functions through pathways such as the Hippo pathway, which is antagonized by RAF1.Citation16,Citation17 RAF1 interaction with MST2 is a link between the MAPK and Hippo pathways.Citation18–21 RAF1 activates extracellular regulated protein kinase (ERK), a member of the MAPK family, which is involved in the proliferation, invasion, and migration of breast cancer cells.Citation22,Citation23 ERK inhibits autophagy by activating mTOR signaling and down-regulates the anti-apoptotic protein Bcl-xl to promote cell survival.Citation24,Citation25 Autophagy plays an important role in maintaining the balance of energy metabolism and cell survival within cells. However, once autophagy is overactivated, apoptosis occurs.Citation26,Citation27 Many studies have focused on simultaneously inducing tumor cell apoptosis while interfering with autophagy for the treatment of cancer. As shown in previous studies, Cucurbitacin I promoted apoptosis and pro-death autophagy via ERK/mTOR/STAT3 signaling, and 3β,11-dihydroxy-9,11-secogorgost-5-en-9-one induced apoptosis due to its ability to promote autophagy.Citation28,Citation29
This study aimed to investigate the mechanism of pimozide inhibition on human MCF-7 (an estrogen receptor-positive cell line) and MDA-MB-231 (a triple negative cell line) breast cancer cells. For this purpose, the growth progression of the treated cells was assessed. Subsequently, the apoptosis and autophagy-related protein expression were evaluated after being treated. A special focus was placed on the RAF/ERK signaling. To investigate the contribution of RAF1 in autophagy and apoptosis, the introduction of inhibitors was considered an effective strategy. In brief, our work was to provide a rationale for pimozide as one of the potential anti-cancer drug candidates.
Results
High RAF1 expression predicts poor prognosis in breast cancer patients
We examined the relationship between RAF1 expression and prognosis in breast cancer patients. As shown in , we analyzed RAF1 mRNA expression levels and protein expression in ER (±), PR (±), and HER2 (±) breast cancer samples using the Kaplan-Meier database.Citation30 We found that HER2 (±) breast cancer cases with RAF1 high expression had a shorter survival time (p = .037, p = .042), and high RAF1 mRNA expression had a shorter survival time (p = .033, p = .018). ER2 (+) breast cancer cases with RAF1 protein and mRNA high expression had a shorter survival time (p = .022, p = .031). ER2 (-) and PR (±) breast cancer cases with RAF1 protein and mRNA high or low expression were not related to survival (Figure S1). We next examined the association of RAF1 mRNA expression with distant metastasis-free survival in HER2 (±) breast cancer samples. Cases with high RAF1 mRNA expression had a lower distant metastasis-free survival in HER2 (-) breast cancers (p = .011), while HER2(+) breast cancer was not related to the distal metastasis-free survival (p = .066) (). We also evaluated breast cancers according to histological subtype.Citation31 As shown in , RAF1 expression was associated with infiltrating ductal carcinoma, infiltrating lobular carcinoma, and mucinous carcinoma and was not associated with other histological subtypes. We examined the expression of RAF1 in breast cancer and adjacent tissues by immunohistochemistry and found that RAF1 expression was related to HER2 (, ). These results suggested that RAF1 expression was associated with a poor prognosis of breast cancer.
Figure 1. RAF1 is expressed in a variety of breast cancer histological subtypes, and high expression of RAF1 is associated with the prognosis of HER-2 positive breast cancer. (a) the Kaplan-Meier database was used to analyze the relationship between the protein expression or mRNA level of RAF1 and overall survival and (b) distant metastasis-free survival in breast cancer patients with HER2 (±) or ER (+) breast cancer. (c) analysis of the expression of RAF1 in different histological subtypes of breast cancer based on the ualcan database (http://ualcan.Path.uab.edu/index.Html) (*, p < .05, ***, p < .001). (d) the expression of RAF1 in breast cancer (right) and adjacent tissues (left).
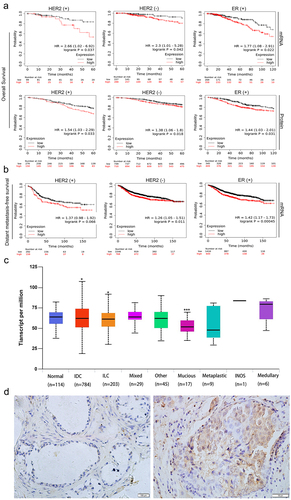
Table 1. RAF1 protein expression in breast cancer samples and adjacent tissue samples.
Table 2. Relationship between RAF1 overexpression and the clinicopathological features in breast cancer.
Pimozide inhibits the proliferation and colony formation of breast cancer cells
Pimozide is a diphenylbutylpiperidine antipsychotic () that was shown to inhibit the growth of breast cancer MCF-7 cells and our previous study found that pimozide competes with L-lactate for binding to oxygen-regulated protein N-myc downstream-regulated gene 3, an upstream signal of RAF1.Citation32,Citation33 Based on these reports, and the discovery in the UALCAN and Kaplan-Meier database, we simulated the binding of pimozide to RAF1 using Schrodinger software. As predicted by Schrodinger software, pimozide might have two binding sites with RAF1: the Site 1 and Site 2 scores were 4.344 and 5.933, respectively ().
Figure 2. Pimozide inhibits breast cancer cell proliferation. (a) the pimozide structure was drawn using ChemDraw. (b) pimozide docked with RAF1 and found two binding sites by Schrodinger software, identified as site 1 and site 2. (c) MTT assay to assess the effects of different concentrations of pimozide on breast cancer cells (6 × 10Citation3 cells/well). (d) effect of 4 μM or 8 μM pimozide on the growth of breast cancer cells (5 × 10Citation4 cells) within 4 days. (e) after treatment with pimozide, the number of clones of breast cancer cells (1 × 10Citation3 cells) was reduced. Percent adhesion was normalized to DMSO control for 3 replicate experiments with 3 or more replicate wells (*, p < .05, ***, p < .001).
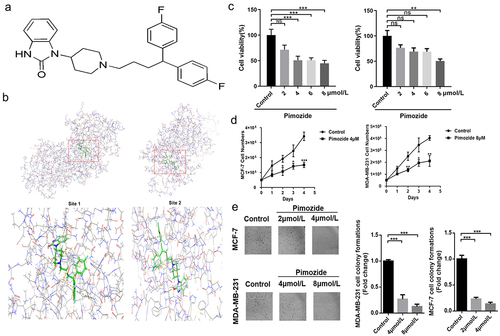
We indicated the effect of pimozide on the viability of human breast cancer cells. MCF-7 and MDA-MB-231 cells were treated with pimozide for 24 h and MTT assays were performed. As shown in , pimozide decreased the viability of MCF-7 and MDA-MB-231 cells with IC50 values of 4 μM and 8 μM, respectively. Furthermore, the proliferative of breast cancer cells treated with pimozide was down-regulated compared with untreated cells (). Our results were similar to previous reports.Citation34,Citation35 To confirm the efficacy of pimozide in inhibiting breast cancer cell proliferation, we also performed colony formation analysis. Pimozide treatment resulted in a reduction of the colony formation ability of both MCF-7 and MDA-MB-231 cells compared with the control groups (). Together these data indicated that pimozide inhibited the proliferation of breast cancer cells.
Pimozide induces apoptosis in breast cancer cells
We next examined the mechanism of the anti-proliferative activity of pimozide by performing flow cytometry. We found that pimozide treatment induced a significant increase in the apoptotic cell population in a concentration-dependent manner both in MCF-7 and MDA-MB-231 cells (). A similar result was obtained with the JC-1 analysis. As shown in , as the concentration of pimozide increased, we observed increased numbers of MCF-7 and MDA-MB-231 cells with decreased membrane potential. We further distinguished live cells and cells in different apoptotic stages by AO-EB staining. As shown in , after treatment with 4 μM pimozide, viable apoptotic cells increased in MCF-7 cells, while MDA-MB-231 cells showed an increase in cells in advanced apoptosis after 8 μM pimozide treatment. These data indicated that pimozide-induced apoptosis contributed to determining the antiproliferative effect observed in breast cancer cells.
Figure 3. Pimozide inhibits breast cancer growth through the RAF/ERK signaling pathway. flow cytometry was used to detect apoptosis (a) and mitochondrial membrane potential (b) in breast cancer cells at different concentrations of pimozide. (c) AO-EB staining to identify normal cells (green), early apoptosis (yellow arrow), late apoptosis (red arrow), dead cells (blue arrow), MCF-7 (1 × 10Citation3 cells), and MDA-MB-231 cells (1 × 10Citation3 cells) were treated with 4 μM and 8 μM pimozide, respectively. (d) Western blot analysis of protein expression increased with the concentration of pimozide. (e) the effect of pimozide on the activities of caspase-6 after treating MCF-7 and MDA-MB-231 cells. Summary data from three replicate experiments with three replicate samples, ns means no statistical significance (*, p < .05, **, p < .01, ***, p < .001).
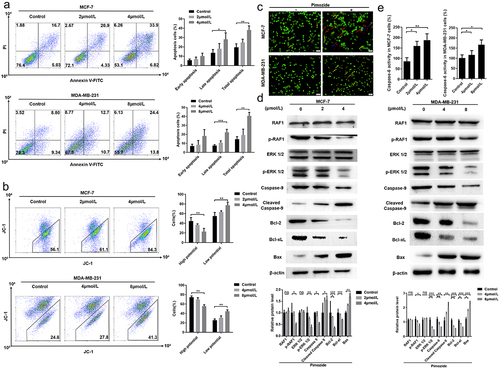
The RAF/ERK pathway is involved in pimozide-induced apoptosis
We further explored the mechanism by which pimozide regulates the relationship between RAF1 and mitochondrial membrane potential. Under the stimulation of apoptotic signals, the membrane potential of mitochondria is lost, and the mitochondrial permeability changes. We next examined the expression levels of proteins in the RAF1 pathway and several regulators of mitochondrial permeability by western blot analysis. As shown in , while RAF1 and ERK 1/2 expression were unaffected by pimozide, both p-RAF1 and p-ERK 1/2 levels were down-regulated by pimozide in a dose-dependent manner in MCF-7 cells. Consistent with our apoptosis results, the levels of the mitochondrial permeability-related proteins Bcl-2 and Bcl-xl were decreased and Bax was increased in pimozide-treated MCF-7 cells. In addition, the apoptosis initiation protein caspase-9 was down-regulated, and cleaved caspase-9 expression increased with increasing pimozide concentration. And the activities of caspase 6 were enhanced in MCF-7 and MDA-MB-231 cells (). These data confirmed that pimozide inhibited the phosphorylation of RAF1 and ERK 1/2 and induced apoptosis in breast cancer cells.
Previous studies reported that RAF1 regulates the Hippo pathway through its interaction with MST2 to alter cell proliferation, migration, and invasion.Citation21,Citation36 We examined the expression of Hippo pathway proteins YAP/TAZ, MST1, and MST2 in MCF-7 cells treated with increasing concentrations of pimozide (Figure S2). However, pimozide had no impact on the expressions of these proteins.
Pimozide promotes autophagy in breast cancer cells
Autophagy plays an important role in providing energy for tumor cells. However, hyperactivation of autophagy is often accompanied by the occurrence of apoptosis. Zielke et al. demonstrated that pimozide promotes autophagic-dependent death in glioma cells.Citation37 To evaluate the effect of pimozide on the induction of autophagy-dependent cell death in breast cancer cells, MDC staining assay and TEM were used to quantify cells undergoing autophagy. We observed autophagosomes by TEM in breast cancer cells and found that pimozide-stimulated cells showed increased autophagosomes compared with controls (). We further examined changes in autophagosomes by MDC staining analysis and the results were consistent with the TEM results. Treatment of MCF-7 and MDA-MB-231 cells with pimozide for 24 h resulted in enhanced autophagy (). Together these findings indicated that pimozide promoted autophagy in breast cancer cells.
Figure 4. Pimozide might promote autophagy in breast cancer cells by up-regulating cAMP. TEM (a) and MDC staining (b) observed the number of autophagosomes in breast cancer cells after pimozide (4 μM or 8 μM) (red arrows indicate autophagosomes). (c) Western blot was used to detect autophagy-related proteins, and β-actin was used as an internal reference. (d) detection of changes in cAMP with increasing pimozide concentration based on the FRET system. The left image was the YFP fluorescence signal, the red circle represented the baseline, the other coils surrounded the cell, the right side was the CFP fluorescence signal, and ratio a meant the fluorescence intensity of the CFP was greater than the fluorescence intensity of the YFP. All experiments were performed three times in parallel, ns means no statistical significance (*, p < .05, ***, p < .001).
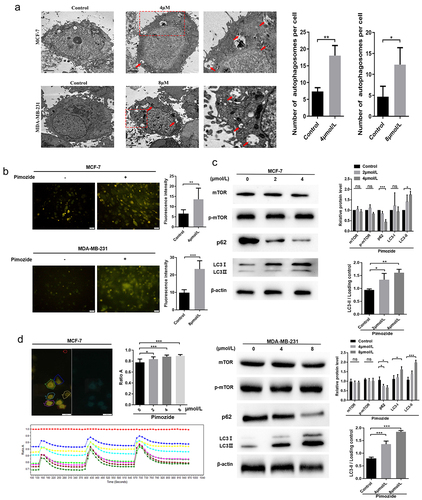
To further research the mechanism involved in pimozide-mediated cell autophagy, we examined the expressions of autophagy-related proteins by western blot. As shown in , the conversion of LC3-I to LC3-II was increased and the level of p62 was significantly decreased in MCF-7 cells treated with pimozide. However, mTOR, an autophagy inhibitor, was not impacted by pimozide treatment (). Previous studies showed that RAF1 interacts with cAMP and upregulation of cAMP can promote autophagy in cells.Citation38,Citation39 We used FRET to detect cAMP changes before and after pimozide treatment in live breast cancer cells (). The data showed that pimozide promoted the up-regulation of cAMP in a dose-dependent manner, cAMP levels reduced after time but did not return to normal levels within the examined time frame. These data suggested that pimozide might promote autophagy by up-regulating cAMP, but mTOR was not involved in pimozide-mediated induction of autophagy in breast cancer cells.
RAF1 plays an important role in the anticancer effects of pimozide
To examine the role of RAF1 in the anticancer effects of pimozide, we used siRNA to interfere with the effects of RAF1 expression on pimozide-induced apoptosis and autophagy (). As shown in , after the RAF1 expression was knocked down, the proliferation of MCF-7 cells was significantly inhibited, similar to the effect of pimozide (4 μM). The MCF-7 cells that knocked down RAF1 were treated with pimozide, and its inhibitory effect was similar to knocking down RAF1 alone or adding pimozide.
Figure 5. The effects of RAF1 interference and pimozide treatment on breast cancer cells. (a) the expression of RAF1 in MCF-7 cells was detected by Western blot after adding siRNA. (b) MTT assay was used to detect the effect of different concentrations of pimozide on the proliferation of breast cancer cells (6 × 10Citation3 cells/well) without pretreatment or knockdown of RAF1 expression. (c) cloning assay was performed to analyze the effects of pimozide (4 μM), siRNA, pimozide, and siRNA on the proliferation of MCF-7 cells (1 × 10Citation3 cells), respectively. (d) after adding pimozide (4 μM), siRNA, pimozide and siRNA, respectively, the expression of proteins was detected by western blot. (e) after adding pimozide (4 μM), Sorafenib (6 nM), pimozide and Sorafenib, respectively, the western blot was used to analyze the expression of proteins. All experiments were performed with DMSO as control and three times in parallel, ns means no statistical significance (*, p < .05, **, p < .01, ***, p < .001).
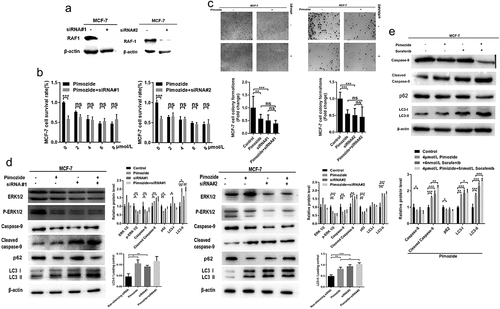
Therefore, we further tested its potential mechanism. As shown in , 6 nM sorafenib, a RAF1 inhibitor, treated breast cancer cells MCF-7 with similar efficacy to pimozide. Pimozide, sorafenib, and knockdown of RAF1 showed similar results, decreased the phosphorylation level of ERK 1/2, the expression of total ERK 1/2 was unaffected, the expression of full-length caspase-9 was decreased, and the expression of cleaved caspase-9 was increased. However, there was no significant difference in the effect of pimozide treatment on MCF-7 cells that RAF1 was knocked down. In addition, cells were treated with pimozide, sorafenib, or knocked down RAF1 reduced autophagy selective substrate p62 expression and increased autophagy marker protein LC3 I to LC3 II. The addition of pimozide and siRNA was similar to the effect alone. Therefore, these data suggested that the RAF/ERK pathway might play an important role in the anti-cancer effect of pimozide.
Pimozide promotes cell death with ongoing autophagy
To examine whether the induction of autophagy contributes to pimozide-induced apoptosis, we treated cells with CQ (50 μM) and pimozide for 24 h. We found that while pimozide promoted autophagy in MCF-7 and MDA-MB-231 cells, CQ treatment reversed pimozide-induced autophagy (). We further examined the expression of the autophagy key protein LC3B and the proliferation-associated protein Ki67 by immunofluorescence staining. Consistent with the flow cytometry results, LC3B expression increased while Ki67 expression was down-regulated by pimozide (). We next examined the effects of the administration of pimozide, CQ, or the combination on apoptosis in breast cancer cells. As shown in , pimozide alone induced apoptosis in breast cancer cells, while CQ treatment did not have substantial effects on apoptosis. Upon pimozide and CQ co-treatment of cells, apoptosis was increased compared to levels in cells treated with CQ. However, in co-treated cells in which autophagy was blocked by CQ, the amount of apoptosis induced by pimozide was reduced compared with cells treated with pimozide alone. These results revealed that pimozide promoted autophagy to induce apoptosis in breast cancer cells.
Figure 6. CQ blocks autophagy and repairs pimozide-induced apoptosis of breast cancer cells. (A) flow cytometry was used to examine the effect of pimozide (4 μM or 8 μM) or CQ (50 μM) alone and their combination on apoptosis of breast cancer cells, and cells supplemented with DMSO were used as controls. (B) cell proliferation and apoptosis marker protein expression were detected by immunofluorescence, and cells not treated with pimozide were used as controls. (C) TEM investigates the effects of pimozide (4 μM or 8 μM) or CQ (50 μM) alone and their combination on apoptosis of breast cancer cells, and the red arrow identifies autophagosomes. (D) after autophagy was blocked by CQ (50 μM), the expression of apoptosis and autophagy marker proteins were detected by western blot. All experiments were performed with DMSO as control and three times in parallel, ns means no statistical significance (*, p<0.05, **, p < .01, ***, p<0.001).
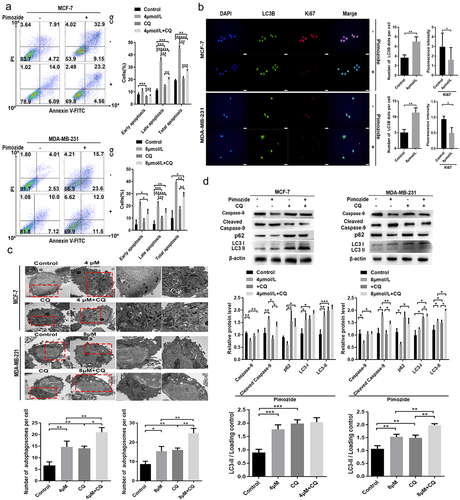
To further determine the mechanism by which pimozide promotes cell death with ongoing autophagy, we examined the expression of the apoptotic initiation protein caspase-9, cleaved caspase-9, and the autophagy key proteins LC3B and p62 by western blot. As shown in , after suppressing the autophagic flow, pimozide-induced apoptosis in breast cancer cells was rescued. CQ reduced pimozide-induced caspase 9 cleavage. CQ caused p62 protein accumulation in breast cancer cells. p62 was inhibited in pimozide-treated breast cancer cells. CQ impaired pimozide activation to inhibit p62. Pimozide or CQ alone caused LC3B accumulation, whereas pimozide and CQ co-treatment decreased the conversion of LC3-I to LC3-II. These data indicated that pimozide-induced apoptosis could be reversed by autophagy inhibitors.
Discussion
Some evidence has shown that antipsychotic drugs are 18 times more likely to produce anticancer activity than random molecules and reports confirmed that the antipsychotic drug pimozide can inhibit the proliferation of cancer cells, including breast cancer cells.Citation33,Citation34,Citation40–42 However, the mechanism by which pimozide inhibits breast cancer has been unknown.
In this study, we found that RAF1 might be associated with a poor prognosis in breast cancer. We also constructed a model combining pimozide and RAF1 using Schrodinger software that suggested that pimozide might bind to RAF1. We found that pimozide inhibited the proliferation and colony formation of breast cancer cells. The results of proliferation marker signal Ki67 and colony formation showed that pimozide inhibited the proliferation of breast cancer cells. Flow cytometry, AO-EB staining and immunoblotting showed that pimozide induced apoptosis in breast cancer cells. These results revealed that pimozide influenced both proliferation and apoptosis of breast cancer cells. We examined whether pimozide inhibits breast cancer by regulating RAF/ERK pathway and found that pimozide treatment caused downregulation of RAF1 phosphorylation in breast cancer cells. Previous reports showed that pimozide, as a STAT3 and STAT5 inhibitor, inhibited phosphorylation of ERK 1/2, thereby inhibiting cancer cell proliferation.Citation43,Citation44 When RAF1 was silenced, ERK 1/2 phosphorylation was subsequently inhibited, and apoptosis and autophagy were promoted in breast cancer cells, indicated that RAF1 signaling functions to regulate apoptosis and autophagy in breast cancer cells. Our results showed that pimozide inhibited RAF1 phosphorylation. Therefore, our work suggests that pimozide functions by modulating RAF1 signaling. Combined with previous findings, it indicated that there might be multiple pathways for pimozide to modulate the same biological signaling. ERK 1/2 is a downstream protein of RAF1, and regulation of RAF1 expression may affect the activation of ERK 1/2 signaling. We found that pimozide treatment resulted in reduced RAF1 phosphorylation and thus inhibition of phosphorylation of RAF1 may play an important role in the anticancer activity of pimozide. Our results suggest that pimozide might exert its anticancer activity in inhibiting the proliferation of breast cancer cells by blocking the RAF/ERK signaling pathway. Bertolesi et al. demonstrated that pimozide inhibited MCF-7 cell growth by interfering with T-type Ca2+ channels.Citation13 which are associated with changes in the mitochondrial membrane potential.Citation45 Our JC-1 assay results demonstrated that pimozide treatment caused an increase in the number of cells with reduced mitochondrial membrane potential. We speculate that these results may be attributed to pimozide inhibiting Ca2+ channels and inducing changes in the expressions of mitochondrial membrane permeability-related proteins Bcl-2, Bcl-xl, and Bax.
Previous studies demonstrated that activation of the RAF1 signal promotes autophagy.Citation46 Interestingly, ERK 1/2 is upregulated in response to mTOR signaling, while mTOR was shown to inhibit autophagy.Citation47 Therefore, we investigated the effect of pimozide on autophagy in breast cancer cells and found that pimozide promoted autophagy in breast cancer cells. Notably, while pimozide did not impact RAF1 and ERK 1/2 protein expression, pimozide down-regulated both RAF1 and ERK 1/2 phosphorylation levels, suggesting that other factors may be involved in the regulation of autophagy. Furthermore, mTOR expression was not affected by pimozide, likely as other signals are involved in the regulation of mTOR, such as the p53 pathway.Citation48 Since previous studies showed that RAF1 can form a complex with phosphodiesterase, which hydrolyzes cAMP, while cAMP can down-regulate RAF1 expression depending on the PKA pathway and cAMP can promote autophagy,Citation36,Citation37,Citation49 we tested the expression of cAMP by FRET and found that pimozide treatment increased the expression of cAMP in breast cancer cells, which may lead to enhanced autophagy in breast cancer cells. Interestingly, overexpression of cAMP regulated the activity of the PKA pathway, thereby promoting apoptosis of cancer cells.Citation50 We synthesized siRNA to interfere with the expression of RAF1. We found that RAF1 was knocked down or treated with pimozide to promote apoptosis and autophagy in breast cancer cells, and there was no significant difference between the two treatments. Interestingly, knockdown of RAF1 resulted in reduced expression of p-ERK 1/2, and caspase-9 was rescued by pimozide. This may be due to the up-regulation of cAMP in cells by pimozide, which has been shown to upregulate phosphorylation of ERK, leading to the combination of pimozide and siRNA to counteract the down-regulation of ERK 1/2 phosphorylation induced by siRNA alone.Citation39 Caspase-9 also produces a cascade reaction as a downstream signal. Since autophagy is associated with apoptosis, we examined the effect of CQ-blocked autophagy on pimozide-induced apoptosis. The data showed that CQ reversed pimozide-induced apoptosis, similar to previous results in glioblastoma cells.Citation37 It is worth noting that pimozide could down-regulate ULK1-related autophagy by inhibiting USP1.Citation51 We speculated that pimozide may be more intense autophagy mediated by cAMP. Pimozide promoted the degradation of ID1 mediated by USP1, which led to interference in cancer progression.Citation52 Reports had shown that ID1 could activate the RAF/MEK signaling pathway in nasopharyngeal carcinoma cells and glioblastoma,Citation53,Citation54 which might reveal that ID1 had a similar effect in breast cancer cells. The addition of the neuroleptic drug pimozide to inhibit ID1 expression enhanced the cytotoxic effects of drug therapy on glioma cells and non-small cell lung cancer,Citation53,Citation55 but the data showed that pimozide had an antagonistic effect on the efficacy of other drugs in non-small cell lung cancer.Citation56 It has been the previous study presented that pimozide inhibited the AKT signaling pathway and epithelial-mesenchymal transition and cell migration in MDA-MB-231 breast cancer cells, and downregulated the expression of MMPs.Citation57 However, in a difference from previous studies, our data demonstrated that pimozide inhibited the phosphorylation of RAF1 while exerting an effect on apoptosis and autophagy in breast cancer cells and that the transient fluctuating rise in cAMP might be associated with cellular autophagy. Hence, our work reveals a potential pathway for pimozide to induce apoptosis in breast cancer. In the follow-up work, we will study the regulation of unknown regulatory factors by pimozide in breast cancer cells, resulting in the blockage of cross-linking between RAF1 and the Hippo pathway. In addition, the elevated level of cAMP expression, whether it means that the PKA pathway is also activated accordingly will also be our focus.
In summary, our results revealed that pimozide inhibits cell proliferation by inducing apoptosis and autophagy in breast cancer cells (). We found that pimozide inhibits the colony formation activities of breast cancer cells. Moreover, we demonstrated that pimozide inhibits breast cancer by down-regulating the RAF/ERK pathway. The Hippo pathway was not regulated by pimozide in breast cancer cells. Pimozide might promote autophagy in breast cancer cells by up-regulating cAMP, rather than by inhibiting mTOR signaling. The autophagy inhibitor CQ rescued apoptosis induced by pimozide. These data suggested that pimozide may induce apoptosis of breast cancer cells by promoting autophagy. Our study provided evidence that pimozide could be a potential drug for the clinical therapy of breast cancer.
Materials and methods
Reagents
Pimozide was purchased from J&K Scientific (P447800). Chloroquine (CQ) was purchased from Solarbio (IC4440). Sorafenib was purchased from Beyotime Biotechnology (SC0236). The caspase-6 activity assay kit and diamidino-phenyl-indole were purchased from Solarbio (BC3860). Epac-SH187 was purchased from Vector Biolabs. MTT was purchased from Solarbio (M8180). Antibodies against RAF1, phospho-ERK 1/2 (Thr202/Tyr204), and Bax were purchased from Elabscience (E-AB-60020, E-AB-70310, E-AB-10049). Antibodies against ERK 1/2, phosphor-RAF1 (Ser338), cleaved caspase-9, Bcl-xl, and Hippo signaling antibody sampler kit were purchased from Cell Signaling Technology (9102, 9427, D8I9E, 54H6, 8579). Antibodies against mTOR, phospho-mTOR (phospho S2448), LC3B, and SQSTM1/p62 were purchased from Abcam (ab32028, ab109268, ab51520, ab109012). Antibodies against Bcl-2 and β-actin were purchased from Bioss (bs-0032 R, bs-0061 R/bsm -33,036 M). Antibodies against Ki-67 were purchased from Zhongshanjinqiao Biological Technology Ltd (TA500265).
Tissue samples
The samples were purchased from Shanghai Outdo Biotech Co., LTD. The samples were routinely processed with 10% buffered formalin fixation and paraffin-embedded. The appropriate paraffin block was selected for this study.
Immunohistochemistry (IHC)
IHC used a 2-step plus poly-HRP anti-rabbit/mouse IgG detection kit (Elabscience, E-IR-R213). After the slides were washed by dewaxing and phosphate-buffered saline, the antigen was repaired by immersing the slides in 0.01 M sodium citrate buffer at 80°C, and then the slides were cooled at room temperature for 1 hour. After dipping in PBS, 3% hydrogen peroxide covers the slides for 15 minutes at room temperature in a humid chamber. The slides were incubated with RAF1 antibody in a humid chamber overnight at 4°C. After washing with PBS, the slides were incubated with the secondary antibody for 1 hour, stained with 3, 30-diaminobenzidine tetrahydrochloride (Zhongshanjinqiao, ZLI-9018), and then Mayer’s hematoxylin counterstained. The rabbit IgG isotype was used as a control to show negative results. The staining of the entire tissue sample was evaluated at high magnification (×20, BX53, Olympus Corporation, Tokyo, Japan).
Cell culture and treatment
The human breast cell lines MCF-7 and MDA-MB-231 were donated by Professor Changliang Shan of Nankai University and were cultured in high glucose Dulbecco’s Modified Eagle’s Medium (HyClone, SH30022.01) supplemented with penicillin (100 unit/ml), streptomycin (100 μg/ml), and 10% fetal bovine serum (Gemini, 900–108) in an atmosphere of 5% CO2 at 37°C. Cells were exposed 24 h to each concentration of pimozide and CQ.
Drug virtual docking analysis
Download the RAF1 protein crystal structure (3OMV) from the Protein Data Bank (http://www.rcsb.org/), this crystal structure was unmodified or not combined with other molecules, derived from the complete crystal structure of homo sapiens. The pimozide structural formula (CAS number 2062-78-4) was downloaded at Drugbank (https://www.drugbank.ca/). Dock pimozide and RAF1 using Schrodinger 2017 (Schrodinger, New York, USA) according to the guidelines provided by the software manufacturer.
Cell viability assay
The cytotoxic potential of pimozide in MCF-7 and MDA-MB-231 cells was determined by MTT assay. Cells were seeded into 96-well plates at a cell density of 6 × 10Citation3 cells/well in the growth medium. Cell growth was then assayed by the addition of 20 μL of MTT to each well, and the plate was incubated at 37°C for 4 h. The reaction was stopped by the addition of 200 μL DMSO (Applichem, A3672.0250). Optical density was measured at 570 nm.
For cell counting, 5 × 10Citation4 cells were seeded in 6-well plates, and MCF-7 and MDA-MB-231 cells were treated with 4 μM and 8 μM pimozide, respectively. Surviving cells were counted under the microscope (×10, BX53, Olympus Corporation, Tokyo, Japan) after 1, 2, 3, and 4 days of drug treatment.
Colony formation assay
Cells were seeded in 6-well plates and treated with DMSO or pimozide in triplicate for 14 days. Colonies were fixed with the cold MeOH, stained with 0.2% crystal violet, and counted manually.
Immunofluorescence
The 1 × 10Citation3 cells were seeded in 6-well plates and treated with DMSO or pimozide for 12 h, and then prefixed in MeOH for 30 min at room temperature, followed by permeating in 0.5% Triton X-100 for 20 min. The sections were then rinsed thrice in PBS and blocked with 5% skim milk at room temperature for 1 h. After washing thrice with PBS, the slides were incubated at 4°C overnight with primary antibodies. The sides were then rinsed thrice in PBS, incubated for 2 h at room temperature with fluorescence-labeled secondary antibody, and treated with DAPI at room temperature for 10 min. Finally, the sections were observed with a fluorescence microscope (Olympus, Tokyo, Japan) after washing with PBS thrice.
Acridine orange and ethidium bromide (AO-EB) staining
The 1 × 10Citation3 cells were seeded in a 6-well plate and made into a slide. Washed twice with PBS to remove residual medium and unattached cells, and added new PBS. Add 20 ul of AO-EB (Solarbio, CA1140) working solution per ml of PBS. After standing at room temperature for 5 min, it was washed twice with PBS to remove the residual fluorescent dye. Add a new PBS to observe under a fluorescence microscope.
Caspase activity assay
The cells were collected in a centrifuge tube, and the supernatant was discarded after centrifugation. The 100 μL of reagent 2 was added to the centrifuge tube, and the pellet was resuspended. Then let it stand on ice for 15 min. Centrifuged it at 15,000 g for 15 min at 4°C. Then performed operations according to the manufacturer’s instructions, and detected the activity of caspase 6 at 405 nm.
Flow cytometry analysis for cell apoptosis
Annexin V-FITC Apoptosis Detection Kit (BD 556,547) was used to evaluate the percentage of apoptotic cells. MCF-7 and MDA-MB-231 cells were treated with pimozide for 24 h. Then, 5 × 10Citation5 treated cells were centrifuged and re-suspended in cold 1 × binding buffer, after which 1.25 μl of Annexin V-FITC and 10 μl of propidium iodide were added and the suspension was analyzed by flow cytometry (Beckman Coulter, CA, USA).
JC-1 analysis
The mitochondrial membrane potential was detected by using JC-1 staining. Cells were harvested, washed with PBS, and incubated with 5 µM JC-1 (Beyotime, C2006) for 30 minutes in the incubator. Then, the cells were washed and data were analyzed using Flowjo software.
RNA interference
Predesigned pools of siRNA oligonucleotides (Guangzhou RiboBio Co., Ltd.) were used for silencing the expression of RAF1 (target sequence: GCACCAAAGTACCTACTAT#1, GCAGCAGCCTCTACAAACA#2). The MCF-7 cells were transfected with 50 nM siRNA against RAF1. The cells were incubated for 48 h in the growth medium containing the transfection complexes. Transfections with siRNA were performed in serum-free DMEM following the manufacturer’s instructions.
FRET analysis
The cells were seeded in a 6-well plate, and the cAMP fluorescent probe Epac-SH187 was transfected with adenovirus, and a 2 μl virus was added to each well. After 12 hours, the OptoLED fluorescence imaging system (Cairn Research Ltd, UK) and the equipped CoolSNAP HQ2 digital CCD camera (Photometrics, Tucson, AZ, USA) and a beam splitter (DV2, Photometrics, USA) connected to the Nikon inverted microscope were used to detect cAMP changes in living cells in real-time.
Monodansylcadaverine (MDC) staining
Cells were seeded on sterile coverslips in 6-well plates and incubated in pimozide or DMSO for 24 hours. After the incubation, the cells were digested with trypsin, centrifuged at 800 g for 5 min, and the cells were collected. The cells were washed once with 300 μl of 1×wash buffer, and the supernatant was discarded. The cells were resuspended by adding 500 μl of 1×wash buffer, and the cell concentration was counted and adjusted to 10Citation6/ml. An appropriate amount of 90 μl of the cell suspension was added to a new tube, and 10 μl of MDC Stain (Solarbio, G0170) was added. Stain at room temperature for 30 min. After centrifugation at 800 g for 5 min, the cells were collected, the cells were washed twice with 300 μl of 1×wash buffer, and the supernatant was discarded. The cells were resuspended by adding 100 μl of collection buffer and added dropwise to the glass. Observe and photograph under a fluorescence microscope (×20, BX53, Olympus Corporation, Tokyo, Japan).
Transmission electron microscopy (TEM) analysis
The 1 × 10Citation7 Cells were treated with pimozide and CQ for 24 h. After treatment, the cells were harvested and fixed with 2.5% glutaraldehyde overnight at 4°C. After washing with 0.1% sodium cacodylate buffer, the cells were post-fixed with 1% osmium tetroxide for 30 min, stained with 2% uranyl acetate at 4°C, dehydrated in a graded ethanol series, and embedded in spur resin. Ultra-thin (70 nm) sections were cut on a Reichert Ultra cut microtome, post-stained with uranyl acetate and lead citrate, and washed with water. The sections were examined by a transmission electron microscope JEM-2100 (JEOL, Musashino, Akishima, Tokyo) operated at 200 kV.
Western blot
The total proteins were collected from MCF-7 and MDA-MB-231 cells cultured with various concentrations of pimozide for 24 hours. Protein concentrations were analyzed by the BCA Protein Assay Kit (Solarbio, PC0020). Then, the proteins were separated by 10% SDS-PAGE and transferred onto PVDF membranes (EMD Millipore, Billerica, MA, USA). The membranes were blocked with 5% nonfat milk for 1 hour at room temperature followed by incubation overnight at 4°C with a primary antibody (RAF1, p-RAF1, ERK 1/2, p-ERK 1/2, Bcl-2, Bcl-xl, Bax, mTOR, p-mTOR, β-actin) at a dilution of 1:1,000 or LC3B, p62 at a dilution of 1:3,000. Subsequently, the membranes were washed three times with PBST and incubated with the appropriate HRP-conjugated secondary antibody (1:2500) for 1 hour at room temperature. Protein bands were visualized in the darkroom and quantitated using the ImageJ software. β-actin was used as an internal control.
Statistical analysis
All statistical analyses were performed using GraphPad Prism 5.0 (San Diego, CA). Values were presented as the ratio ± SD of the control. The paired student’s t-test was used to analyze statistical significance. p < .05 was considered to indicate statistical significance. Each experiment was performed in triplicate.
Author contributions
XZ and SL wrote the paper; GJ, QZ, and SL conceived and designed the study; GJ, XZ, XW, LY, and XT, did the experiments; SL, QZ, and GJ collected the specimens and analyzed the data. All authors read and approved the final manuscript.
Ethics approval
Pathology slides were obtained from commercial companies. This work described has been carried out in accordance with the declaration of Helsinki and approved by the Research Ethics Committee of Shanghai Outdo Biotech (SHYJS-CP-1901010).
Supplemental material.docx
Download MS Word (4.9 MB)Acknowledgments
We thank Edanz Group (www.edanzediting.com/ac) for editing a draft of this manuscript and Professor Shan from Nankai University for giving us MCF-7 and MDA-MB-231 cells as gifts.
Disclosure statement
No potential conflict of interest was reported by the authors.
Data availability statement
The data that support the findings of this study are available from the corresponding author, [S.L], upon reasonable request.
Supplementary material
Supplemental data for this article can be accessed online at https://doi.org/10.1080/15384047.2024.2302413
Additional information
Funding
Notes on contributors
Ge Jiang
Ge Jiang, Professor, works in Bioengineering College of Dalian University, is mainly engaged in the research of gene-drug transportation carriers and the mechanism of action of anticancer drugs.
Xingzhi Zhou
Xingzhi Zhou, Assistant Technician, works in the Medical College of Dalian University, focusing on the mechanism of action of anticancer drugs.
Ye Hu
Ye Hu, M.S., graduated from the Medical College of Dalian University, focusing on the mechanism of action of anticancer drugs.
Xiaoyu Tan
Xiaoyu Tan, supervising technician, works in Department of Clinical Laboratory of Xin Hua Hospital Affiliated to Dalian University, mainly engaged in the research work related to immune diseases.
Dan Wang
Dan Wang, Lecturer, works in the Medical College of Dalian University, focusing on the pharmacology of various diseases.
Lina Yang
Lina Yang, M.S., graduated from the Medical College of Dalian University, focusing on the mechanism of action of anticancer drugs.
Qinggao Zhang
Qinggao Zhang, Professor, Director of the Department of Medicine, Dalian University, is mainly engaged in the research of immune diseases and the mechanism of action of anti-cancer drugs.
Shuangping Liu
Shuangping Liu, Professor, Vice Dean of the Medical College of Dalian University, is mainly engaged in the research of mechanism of action of anticancer drugs and screening of tumor diagnostic markers.
References
- Torre LA, Bray F, Siegel RL, Ferlay J, Lortet-Tieulent J, Jemal A. Global cancer statistics, 2012. CA Cancer J Clin. 2015;65(2):87–15. doi:10.3322/caac.21262.
- Bray F, Ferlay J, Soerjomataram I, Siegel RL, Torre LA, Jemal A. Global cancer statistics 2018: GLOBOCAN estimates of incidence and mortality worldwide for 36 cancers in 185 countries. CA Cancer J Clin. 2018;68(6):394–424. doi:10.3322/caac.21492.
- Yardley DA. Drug resistance and the role of combination chemotherapy in improving patient outcomes. Int J Breast Cancer. 2013;2013:1–15. doi:10.1155/2013/137414.
- Cuzick J, Baum M. Tamoxifen and contralateral breast cancer. Lancet. 1985;2(8449):282. doi:10.1016/S0140-6736(85)90338-1.
- Del Barco S, Vazquez-Martin A, Cufí S, Oliveras-Ferraros C, Bosch-Barrera J, Joven J, Martin-Castillo B, JA M. Metformin: multi-faceted protection against cancer. Oncotarget. 2011;2(12):896–917. doi:10.18632/oncotarget.387.
- Boguski MS, Mandl KD, Sukhatme VP. Drug discovery. Repurposing with a difference. Sci. 2009;324(5933):1394–1395. doi:10.1126/science.1169920.
- Bashir MN. Epidemiology of prostate cancer. Asian Pac J Cancer Prev. 2015;16(13):5137–5141. doi:10.7314/APJCP.2015.16.13.5137.
- Hossain MA, Kim DH, Jang JY, Kang YJ, Yoon JH, Moon JO, Chung HY, Kim GY, Choi YH, Copple BL, et al. Aspirin induces apoptosis in vitro and inhibits tumor growth of human hepatocellular carcinoma cells in a nude mouse xenograft model. Int J Oncol. 2012;40(4):1298–1304. doi:10.3892/ijo.2011.1304.
- Liu Q, Li Y, Niu Z, Zong Y, Wang M, Yao L, Lu Z, Liao Q, Zhao Y. Atorvastatin (lipitor) attenuates the effects of aspirin on pancreatic cancerogenesis and the chemotherapeutic efficacy of gemcitabine on pancreatic cancer by promoting M2 polarized tumor associated macrophages. J Exp Clin Cancer Res. 2016;35(1):33. doi:10.1186/s13046-016-0304-4.
- Ren Y, Tao J, Jiang Z, Guo D, Tang J. Pimozide suppresses colorectal cancer via inhibition of Wnt/β-catenin signaling pathway. Life Sci. 2018;209:267–273. doi:10.1016/j.lfs.2018.08.027.
- Cai N, Zhou W, Ye LL, Chen J, Liang QN, Chang G, Chen JJ. The STAT3 inhibitor pimozide impedes cell proliferation and induces ROS generation in human osteosarcoma by suppressing catalase expression. Am J Transl Res. 2017;9:3853–3866.
- Fako V, Yu Z, Henrich CJ, Ransom T, Budhu AS, Wang XW. Inhibition of Wnt/β-catenin signaling in hepatocellular carcinoma by an antipsychotic drug pimozide. Int J Biol Sci. 2016;12(7):768–775. doi:10.7150/ijbs.14718.
- Elmaci I, Altinoz MA. Targeting the cellular schizophrenia. Likely employment of the antipsychotic agent pimozide in treatment of refractory cancers and glioblastoma. Crit Rev Oncol Hematol. 2018;128:96–109. doi:10.1016/j.critrevonc.2018.06.004.
- Tian H, Yin L, Ding K, Xia YY, Wang XH, Wu JZ, He X. Raf1 is a prognostic factor for progression in patients with non‑small cell lung cancer after radiotherapy. Oncol Rep. 2018;39:1966–1974. doi:10.3892/or.2018.6277.
- Bertolesi GE, Shi C, Elbaum L, Jollimore C, Rozenberg G, Barnes S, Kelly ME. The Ca 2+ Channel Antagonists Mibefradil and Pimozide Inhibit Cell Growth via Different Cytotoxic Mechanisms. Mol Pharmacol. 2002;62(2):210–219. doi:10.1124/mol.62.2.210.
- Desideri E, Cavallo AL, Baccarini M. Alike but different: RAF paralogs and their signaling outputs. Cell. 2015;161(5):967–970. doi:10.1016/j.cell.2015.04.045.
- Jeric I, Maurer G, Cavallo AL, Raguz J, Desideri E, Tarkowski B, Parrini M, Fischer I, Zatloukal K, Baccarini M. A cell-autonomous tumour suppressor role of RAF1 in hepatocarcinogenesis. Nat Commun. 2016;7(1):13781. doi:10.1038/ncomms13781.
- Ma SQ, Cao BR, Zhang H, Luo LP, Ren Y, Hu T, Chen CM. The lack of raf-1 kinase feedback regulation enhances antiapoptosis in cancer cells. Oncogene. 2017;36(14):2014–2022. doi:10.1038/onc.2016.384.
- Castro L, Alves S, Chaves SR, Costa JL, Soares P, Preto A. RAF-1 promotes survival of thyroid cancer cells harboring RET/PTC1 rearrangement independently of ERK activation. Mol Cell Endocrinol. 2015;415:64–75. doi:10.1016/j.mce.2015.08.006.
- Dai H, Chen H, Liu W, You Y, Tan J, Yang A, Lai X, Bie P. Effects of Raf kinase inhibitor protein expression on pancreatic cancer cell growth and motility: an in vivo and in vitro study. J Cancer Res Clin Oncol. 2016;142(10):2107–2117. doi:10.1007/s00432-016-2206-4.
- Feng R, Gong J, Wu L, Wang L, Zhang B, Liang G, Zheng H, Xiao H. MAPK and Hippo signaling pathways crosstalk via the RAF-1/MST-2 interaction in malignant melanoma. Oncol Rep. 2017;38(2):1199–1205. doi:10.3892/or.2017.5774.
- Liu B, Xing X, Li X, Guo Q, Xu T, Xu K. ZNF259 promotes breast cancer cells invasion and migration via ERK/GSK3β/snail signaling. Cancer Manag Res. 2018;10:3159–3168. doi:10.2147/CMAR.S174745.
- Ren H, Ma J, Si L, Ren B, Chen X, Wang D, Hao W, Tang X, Li D, Zheng Q. Low dose of acacetin promotes breast cancer MCF-7 cells proliferation through the activation of ERK/PI3K/AKT and cyclin signaling pathway. Recent Pat Anticancer Drug Discov. 2018;13(3):368–377. doi:10.2174/1574892813666180420154012.
- Decuypere JP, Parys JB, Bultynck G. Regulation of the autophagic bcl-2/beclin 1 interaction. Cells. 2012;1(3):284–312. doi:10.3390/cells1030284.
- Basit F, Cristofanon S, Fulda S. Obatoclax (GX15-070) triggers necroptosis by promoting the assembly of the necrosome on autophagosomal membranes. Cell Death Differ. 2013;20(9):1161–1173. doi:10.1038/cdd.2013.45.
- Liu Y, Fan D. Ginsenoside Rg5 induces apoptosis and autophagy via the inhibition of the PI3K/Akt pathway against breast cancer in a mouse model. Food Funct. 2018;9(11):5513–5527. doi:10.1039/C8FO01122B.
- Cao C, Huang W, Zhang N, Wu F, Xu T, Pan X, Peng C, Han B. Narciclasine induces autophagy-dependent apoptosis in triple-negative breast cancer cells by regulating the AMPK-ULK1 axis. Cell Prolif. 2018;51(6):e12518. doi:10.1111/cpr.12518.
- Ni Y, Wu S, Wang X, Zhu G, Chen X, Ding Y, Jiang W. Cucurbitacin I induces pro-death autophagy in A549 cells via the ERK-mTOR-STAT3 signaling pathway. J Cell Biochem. 2018;119(7):6104–6112. doi:10.1002/jcb.26808.
- Weng JR, Chiu CF, Hu JL, Feng CH, Huang CY, Bai LY, Sheu JH. A sterol from soft coral induces apoptosis and autophagy in MCF-7 breast cancer cells. Mar Drugs. 2018;16(7):e238. doi:10.3390/md16070238.
- Gyorffy B. Survival analysis across the entire transcriptome identifies biomarkers with the highest prognostic power in breast cancer, comput. Struct Biotechnol J. 2021;19:4101–4109. doi:10.1016/j.csbj.2021.07.014.
- Chandrashekar DS, Bashel B, Balasubramanya SAH, Creighton CJ, Rodriguez IP, BVSK C, Varambally S. UALCAN: a portal for facilitating tumor subgroup gene expression and survival analyses. Neoplasia. 2017;19(8):649–658. doi:10.1016/j.neo.2017.05.002.
- Cao HY, Wu YH, Zhou XZ, Zheng XF, Jiang G. In silico prediction and validation of oxygen-regulated protein N-myc downstream regulated gene 3 and virtual screening of competitive inhibitors of L-Lactate as therapeutics. Lett Drug Des Discov. 2018;16(6):637–644. doi:10.2174/1570180815666180816130621.
- Strobl JS, Kirkwood KL, Lantz TK, Lewine MA, Peterson VA, Worley JF. Inhibition of human breast cancer cell proliferation in tissue culture by the neuroleptic agents pimozide and thioridazine. Cancer Res. 1990;50(17):5399–5405.
- Wiklund ED, Catts VS, Catts SV, Ng TF, Whitaker NJ, Brown AJ, Lutze-Mann LH. Cytotoxic effects of antipsychotic drugs implicate cholesterol homeostasis as a novel chemotherapeutic target. Int J Cancer. 2010;126(1):28–40. doi:10.1002/ijc.24813.
- Roos A, Dhruv HD, Peng S, Inge LJ, Tuncali S, Pineda M, Millard N, Mayo Z, Eschbacher JM, Loftus JC, et al. EGFRvIII–Stat5 signaling enhances glioblastoma cell migration and survival. Mol Cancer Res. 2018;16(7):1185–1195. doi:10.1158/1541-7786.MCR-18-0125.
- Romano D, Nguyen LK, Matallanas D, Halasz M, Doherty C, Kholodenko BN, Kolch W. Protein interaction switches coordinate raf-1 and MST2/Hippo signalling. Nat Cell Biol. 2014;16(7):673–684. doi:10.1038/ncb2986.
- Zielke S, Meyer N, Mari M, Abou-El-Ardat K, Reggiori F, van Wijk SJL, Kogel D, Fulda S, van Wijk SJL. Loperamide, pimozide, and STF-62247 trigger autophagy-dependent cell death in glioblastoma cells. Cell Death Disease. 2018;9(10):994. doi:10.1038/s41419-018-1003-1.
- Basole CP, Nguyen RK, Lamothe K, Vang A, Clark R, Baillie GS, Epstein PM, Brocke S. PDE8 controls CD4+ T cell motility through the PDE8A-Raf-1 kinase signaling complex. Cell Signal. 2017;40:62–72. doi:10.1016/j.cellsig.2017.08.007.
- Ugland H, Naderi S, Brech A, Collas P, Blomhoff HK. cAMP induces autophagy via a novel pathway involving ERK, cyclin E and beclin 1. Autophagy. 2011;7(10):1199–1211. doi:10.4161/auto.7.10.16649.
- Strobl JS, Melkoumian Z, Peterson VA, Hylton H. The cell death response to γ-radiation in MCF-7 cells is enhanced by a neuroleptic drug, pimozide. Breast Cancer Res Treat. 1998;51(1):83–95. doi:10.1023/A:1006046604062.
- Goncalves JM, Silva CAB, Rivero ERC, Cordeiro MMR. Inhibition of cancer stem cells promoted by pimozide. Clin Exp Pharmacol Physiol. 2019;2(2):116–125. doi:10.1111/1440-1681.13049.
- Kim JY, Park Y, Lee BM, Kim HS, Yoon S. P-gp inhibition by the anti-psychotic drug pimozide increases apoptosis, as well as expression of prb and ph2ax in highly drug-resistant kbv20c cells. Anticancer Res. 2017;38(10):5685–5692. doi:10.21873/anticanres.12905.
- Zhou W, Chen MK, Yu HT, Zhong ZH, Cai N, Chen GZ, Zhang P, Chen JJ. The antipsychotic drug pimozide inhibits cell growth in prostate cancer through suppression of STAT3 activation. Int J Oncol. 2016;48(1):322–328. doi:10.3892/ijo.2015.3229.
- Nelson EA, Walker SR, Weisberg E, Bar-Natan M, Barrett R, Gashin LB, Terrell S, Klitgaard JL, Santo L, Addorio MR, et al. The STAT5 inhibitor pimozide decreases survival of chronic myelogenous leukemia cells resistant to kinase inhibitors. Blood. 2011;117(12):3421–3429. doi:10.1182/blood-2009-11-255232.
- Kostic M, Katoshevski T, Sekler I. Allosteric regulation of NCLX by mitochondrial membrane potential links the metabolic state and Ca signaling in mitochondria. Cell Rep. 2018;25(12):3465–3475. doi:10.1016/j.celrep.2018.11.084.
- Zhang P, Zheng Z, Ling L, Yang X, Zhang N, Wang X, Hu M, Xia Y, Ma Y, Yang H, et al. w09, a novel autophagy enhancer, induces autophagy-dependent cell apoptosis via activation of the EGFR-mediated RAS-RAF1-MAP2K-MAPK1/3 pathway. Autophagy. 2017;13(7):1093–1112. doi:10.1080/15548627.2017.1319039.
- Kim KY, Park KI, Kim SH, Yu SN, Park SG, Kim YW, Seo YK, Ma JY, Ahn SC. Inhibition of autophagy promotes salinomycin-induced apoptosis via reactive oxygen species-mediated PI3K/AKT/mTOR and ERK/p38 MAPK-dependent signaling in human prostate cancer cells. Int J Mol Sci. 2017;18(5):E1088. doi:10.3390/ijms18051088.
- Guo Y, Li Y, Wang FF, Xiang B, Huang XO, Ma HB, Gong YP. The combination of nutlin-3 and Tanshinone IIA promotes synergistic cytotoxicity in acute leukemic cells expressing wild-type p53 by co-regulating MDM2-P53 and the AKT/mTOR pathway. Int J Biochem Cell Biol. 2019;106:8–20. doi:10.1016/j.biocel.2018.10.008.
- Skalhegg BS, Landmark BF, Doskeland SO, Hansson V, Lea T, Jahnsen T. Cyclic AMP-dependent protein kinase type I mediates the inhibitory effects of 3‘,5‘-cyclic adenosine monophosphate on cell replication in human T lymphocytes. J Biol Chem. 1992;267(22):15707–15714. doi:10.1016/S0021-9258(19)49593-8.
- Persaud L, Mighty J, Zhong X, Francis A, Mendez M, Muharam H, Redenti SM, Das D, Aktas BH, Sauane M. IL-24 promotes apoptosis through cAMP-dependent PKA pathways in human breast cancer cells. Int J Mol Sci. 2018;19(11):3561. doi:10.3390/ijms19113561.
- Raimondi M, Cesselli D, Di Loreto C, La Marra F, Schneider C, Demarchi F. USP1 (ubiquitin specific peptidase 1) targets ULK1 and regulates its cellular compartmentalization and autophagy. Autophagy. 2019;15(4):613–630. doi:10.1080/15548627.2018.1535291.
- Mistry H, Hsieh G, Buhrlage SJ, Huang M, Park E, Cuny GD, Galinsky I, Stone RM, Gray NS, D’Andrea AD, et al. Small-molecule inhibitors of USP1 target ID1 degradation in leukemic cells. Mol Cancer Ther. 2013;12(12):2651–62. doi:10.1158/1535-7163.MCT-13-0103-T.
- Sachdeva R, Wu MG, Smiljanic S, Kaskun O, Ghannad-Zadeh K, Celebre A, Isaev K, Morrissy AS, Guan J, Tong JF, et al. ID1 is critical for tumorigenesis and regulates chemoresistance in glioblastoma. Cancer Res. 2019;79(16):4057–4071. doi:10.1158/0008-5472.CAN-18-1357.
- Cheung HW, Ling MT, Tsao SW, Wong YC, Wang XH. Id-1-induced Raf/MEK pathway activation is essential for its protective role against taxol-induced apoptosis in nasopharyngeal carcinoma cells. Carcinogenesis. 2004;25(6):881–887. doi:10.1093/carcin/bgh087.
- Chen JJ, Dexheimer TS, Ai YX, Liang Q, Villamil MA, Inglese J, Maloney DJ, Jadhav A, Simeonov A, Zhuang ZH. Selective and cell-active inhibitors of the USP1/UAF1 deubiquitinase complex reverse cisplatin resistance in non-small cell lung cancer cells. Chem Biol. 2011;18(11):1390–400. doi:10.1016/j.chembiol.2011.08.014.
- Fortney K, Griesman J, Kotlyar M, Pastrello C, Angeli M, Sound-Tsao M, Jurisica I, Tucker-Kellogg G. Prioritizing therapeutics for lung cancer: an integrative meta-analysis of cancer gene signatures and chemogenomic data. PLoS Comput Biol. 2015;11(3):e1004068. doi:10.1371/journal.pcbi.1004068.
- el-H D, Pickard A, Srivastava K, McCrudden CM, Gross SR, Lloyd S, Zhang SD, Margariti A, Morgan R, Rudland PS, et al. The anti-psychotic drug pimozide is a novel chemotherapeutic for breast cancer. Oncotarget. 2018;9(79):34889–34910. doi:10.18632/oncotarget.26175.