ABSTRACT
Metastasis accounts for the vast majority of cancer deaths; however, this complex process has yet to be fully explained. To form metastases, cancer cells must undergo a series of steps, known as the “Metastatic cascade”, each of which requires a specific functional transformation. Cancer stem cells (CSCs) play a vital role in tumor metastasis, but their dynamic behavior and regulatory mechanisms have not been fully elucidated. Based on the “Metastatic cascade” theory, this review summarizes the effect of liver CSCs on the metastatic biological programs that underlie the dissemination and metastatic growth of cancer cells. Liver CSCs have the capacity to initiate distant organ metastasis via EMT, and the microenvironment transformation that supports the ability of these cells to disseminate, evade immune surveillance, dormancy, and regenerate metastasis. Understanding the heterogeneity and traits of liver CSCs in these processes is critical for developing strategies to prevent and treat metastasis of advanced hepatocellular carcinoma (HCC).
Introduction
HCC is the sixth most common cancer and the third most common cause of cancer-related death.Citation1 Surgical therapy is the primary treatment for patients with solitary tumors at an early stage. For these patients, resection is associated with 5-year survival exceeding 60%; however, up to 70% of these patients suffer tumor recurrence by 5 years.Citation2 Because most HCCs occur within a background of liver disease and cirrhosis, liver transplantation is often the optimal surgical treatment option for HCC patients with early stage tumors and accounts for approximately 20–40% of all liver transplantation recipients worldwide.Citation3 As reported previously, the recurrence of HCC after liver transplantation ranges between 10 and 20% and may be higher as the selection criteria are expanded.Citation4 Tumor recurrence after liver transplantation is frequently associated with extrahepatic metastasis, particularly in the lungs and bones.Citation3,Citation5,Citation6 The poor prognosis of HCC remains a great challenge principally due to its high rate of metastasis. Therefore, a deeper understanding of the mechanisms underlying HCC metastasis is urgently required.
The development of metastases requires a variety of cellular mechanisms termed the ‘metastatic cascade’, which includes acquiring invasive potential, developing the capability to disseminate from their primary site, promoting vascular invasion, surviving while circulating in the bloodstream, entering and exiting dormancy, establishing new cellular surroundings in secondary sites, stimulating angiogenesis, and evading the host immune system.Citation7–9 Tumor metastasis is a complex process that takes several years to achieve. Only a small fraction of the cells can develop the capability to metastasize to distant sites. These cells must undergo a complex transformation acquiring a variety of phenotypic traits to survive various stresses during the process and become proficient at initiating metastatic disease outgrowth. This specialized subset of cells responsible for tumor metastasis remains largely incurable. An increasing number of studies have suggested that metastatic colonization of distant organs is initiated by cancer cells with stem cell – like phenotype.Citation10–13
Cancer stem cells (CSCs) are a small subpopulation of stem-like tumor cells capable of self-renewal, differentiation, and tumorigenesis.Citation14 There has been a marked increase in research focused on the identification and characterization of CSCs in general, and specifically liver CSCs in the past two decades, which has brought new hope to the diagnosis and treatment of HCC. Several liver CSC markers have been identified, including CD133, CD90, EpCAM, CD13, CD44, OV6, CD24, α2δ1, ICAM-1, Lgr5, and K19.Citation15,Citation16 Although the mechanisms remain unclear, an increasing number of studies have confirmed the link between liver CSCs and HCC metastasis.Citation17,Citation18 Understanding the role of liver CSCs in the development of HCC cell metastasis could provide new insights into the diagnosis and treatment of metastatic HCC. In this review, numerous studies that have addressed the involvement of liver CSCs in each step of the metastatic process have been examined to further explore the important role of liver CSCs in HCC metastasis and thereby provide ideas for better treatment of HCC metastasis.
Interplay of liver CSCs and EMT in HCC
To investigate and comprehend the molecular mechanisms of tumor metastasis inevitably brings epithelial – mesenchymal transition (EMT) into the picture. EMT is a cellular process by which epithelial cells lose their epithelial characteristics and acquire the phenotype of mesenchymal stem cells. In cancers, aberrant activation of this transformation process can be disastrous, as the differentiated epithelial cells are endowed with migratory and invasive characteristics, thereby initiating the dissemination of tumor metastasis.Citation19 The scope of biological processes that have been found to be driven by EMT extends far beyond the initial steps of cancer cell invasion. Accumulating evidence indicates that experimental activation of EMT confers stemness properties, such as CSCs, on epithelial tumor cells.Citation20,Citation21
An accumulating body of research indicates that EMT in HCC cells is always accompanied by the acquisition of stemness.Citation22–28 Although the full relationship between EMT and HCC stem cells remains unclear, it is widely recognized that TGF-β1-induced EMT promotes tumor cell transformation into the CSC phenotype, including HCC cells.Citation29 HIF‐1α reportedly induced the EMT process of transforming HCC cells into cells with CSC characteristics, which was mediated by Notch1 activation.Citation30 Interestingly, not only does induction of EMT promote stemness, conversely, induction of stemness also promotes the EMT process. MHCC97-L cells were empowered with CSCs properties by ectopic co-expression of stemness-related genes Oct4 and Nanog and underwent EMT to promote tumor migration and invasion/metastasis in vitro and in vivo.Citation31 Another study reported that CSC spheres, induced by culturing in a unique medium containing neural survival factor-1, possessed an increased metastatic potential which promoted the EMT process.Citation32 Nonetheless, certain experimental evidence does not support a correlation between EMT and stemness. For example, Vimentin+CD133- HCC cells demonstrate EMT phenotypes whereas Vimentin-CD133+ stem cells do not have EMT phenotypes, and Vimentin-CD133- cells exhibit more aggressive metastasis than vimentin-CD133+ cells.Citation33 Therefore, the correlation between HCC stem cells and the EMT requires further investigation.
Liver CSCs niche required for CSCs maintenance and survival
The tumor microenvironment (TME) surrounding tumors communicates with tumor cells, leading to the growth, invasion, and metastasis of various tumors, including HCC. It has long been recognized that interactions between cancer cells, endothelial cells, stromal fibroblasts, and immune cells are mediated by a number of cytokines or growth factors, as well as alterations in tissue oxygen tension, and that the architecture of the adjacent extracellular matrix (ECM) profoundly impacts tumor progressionCitation34 (). Mounting evidence has demonstrated the presence and role of environmental inflammatory factors such as TGF-β1,Citation29 chemokine,Citation35 IL-17,Citation36 lipopolysaccharide,Citation37 IL-6,Citation38 and HGFCitation39 in the HCC TME in the regulation of non-CSC-acquired stemness or CSC-maintained stemness (). Under the stimulation of the inflammatory factor IL-6, normal liver stem cells may transform into metastatic liver CSCs, thereby promoting the metastasis of liver cancer.Citation40
Figure 1. Liver CSCs within the niche.
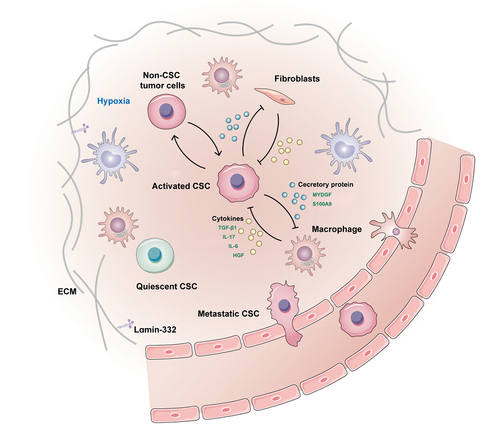
Distinct regions within the microenvironment harboring CSCs are termed the “CSC niche”. As shown in several tumors, interactions between CSCs and their niches are critical for metastatic progression.Citation41 Laminin-332, an extracellular matrix protein complex, is part of the specialized liver CSCs niche in maintaining cell stemness, which leads to chemoresistance and quiescence.Citation42 An hypoxic microenvironment is common in solid cancers, particularly in HCC, and is an important component of the stem cell niche that promotes the stemness of HCC via HIF-1α.Citation43–45 Hypoxia is sufficient to promote invasion and accelerate metastatic outgrowth, which is associated with rapid dedifferentiation of tumor cells into liver CSCs.Citation46 Liver CSCs can construct their niche by secreting proteins that communicate with macrophages. It has been reported that the secretory protein MYDGF released by HCC cells under hypoxic conditions might be involved in the enhanced self-renewal of liver CSCs. Furthermore, MYDGF was also found to induce chemotaxis of macrophages into tumor tissue then release cytokines, which ultimately amplified inflammation of the tumor microenvironment and accelerated HCC progression.Citation47 Secretory protein S100A9 secreted from tumor-associated macrophages may enhance HCC cells to acquire stemness through paracrine signaling.Citation48 Liver CSCs also facilitate migration of macrophages by upregulating chemokine-related genes and elevating metastatic potential by recruiting macrophages in vivo.Citation49 Cancer-associated fibroblasts, a component of the stromal fraction in the TME, contribute to the stemness of liver CSCs by secreting growth factors.Citation50,Citation51 Lymphatic endothelial cells are also important components of the liver CSCs niche microenvironment, and the interaction between liver CSCs and lymphatic endothelial cells promotes the self-renewal and immune escape of hepatoma stem cells via activation of IL-17A signaling.Citation52 From these studies, it is evident that there is close crosstalk between liver CSCs and niche cells, which not only maintains their own stemness but also sows the “seeds” for tumor metastasis ().
Effect of liver CSCs-mediated ECM remodeling on metastasis of HCC
The extracellular matrix (ECM) undergoes dramatic changes during cancer progression and plays a central role in the metastatic process.Citation53 The proliferation, invasion, and dissemination of tumor cells are accompanied by dramatic changes in the mechanical properties (stiffness) of the ECM in the cancer cell niche.Citation54 One study provided evidence that tumor tissues from metastatic HCC patients possessed a higher matrix stiffness than the non-metastatic group.Citation55 Multiple tumor studies have shown that diverse matrix stiffnesses can contribute to the self-renewal of CSCs. In HCC, the link between CSCs stemness and extracellular matrix stiffness remains controversial. Soft matrix stiffness promotes the acquisition of stemness in HCC cells.Citation54,Citation56 A soft spot matrix created by CD133+ liver CSCs through ECM remodeling could enhance stemness maintenance, drug resistance, and metastatic dissemination of HCC cells.Citation57 However, higher matrix stiffness was reported to promote the CSC phenotype and reduce sorafenib-induced apoptosis.Citation58 Recently, Li et al. showed that a stiffer matrix significantly potentiated LCSC stemness compared to a soft matrix by establishing a three-dimensional hydrogel for culturing liver CSCs.Citation59 Another recent study reported that α2δ1+ liver CSCs created a stiff ECM through the secretion of lysyl oxidase (LOX), which was subsequently required for the acquisition and maintenance of HCC CSC characteristics.Citation60 These results would support the postulate that dynamic alterations in the stiffness of the liver CSCs niche could be responsible for regulating CSC self-renewal and phenotypes throughout the natural history of HCC metastasis.
Relationship between liver CSCs and circulating tumor cells
Circulating tumor cells (CTCs) are malignant cells that extravasate into the blood vessels (intravasation) from primary tumors and are considered the “seeds” of distant metastasis.Citation61 Many previous studies have demonstrated that CTCs possess the CSC phenotype.Citation62–64 However, the characteristics of circulating CSCs in HCC are poorly understood. In HCC, 71.4% of patients had CTCs positive for the CSC marker CD44; thus, they had a significant population of CTCs with CSC properties.Citation65 Most current CTC capture methods are based on epithelial cell adhesion molecule (EpCAM) detection, including HCC.Citation66,Citation67 EpCAM is also a commonly used biomarker for liver CSC isolation.Citation18,Citation68 Stem cell – like phenotypes have been confirmed in HCC EpCAM+ CTCs by testing the expression of stem cell-related markers and high tumorigenic ability.Citation69
Numerous studies have found that the circulating CSC count has significant importance in monitoring and prognosis of patients with HCC by detecting liver CSCs in the blood via a variety of surface markers. CD45-CD90+ CSCs were detectable in 90% of HCC patients, and the number of CD45-CD90+ cells in the tumor tissues was significantly positively correlated with the number of CD45-CD90+ cells in the blood samples.Citation70 Detection of circulating CSCs (CD45− CD90+ CD44+cells) is highly accurate in predicting HCC recurrence following hepatectomy.Citation71 Utilizing ICAM-1 as a liver CSC marker, Liu et al. discovered that ICAM-1-positive CTCs were detected in 50% of all cases, and that these cells correlated with worse clinical outcomes.Citation72 Guo et al. found that CTCs with stem-like phenotypes, rather than the entire population of CTCs, might reflect the micrometastatic status, which could not be detected by routine imaging techniques, and enable a more accurate estimate of recurrence risk of HCC patients.Citation73
Quiescence/Dormancy liver CSCs in HCC metastasis
HCC metastasis typically becomes clinically evident years following the removal of a primary tumor by resection or liver transplantation, derived from a small number of disseminated cancer cells that survive as latency-competent cancer (LCC) cells. LCC cells show stem cell-like characteristics that are essential for their survival under immune surveillance, ultimately developing metastatic outgrowth under permissive conditions.Citation74 CSCs, through their immunomodulating features by secreting a variety of factors or cytokines, evade immune surveillance and immune attack, persisting in the form of quiescence and dormancy.Citation75 Thus, CSCs cannot be detected by the immune system before the development of metastasis and recurrence, due to the progression of dormancy.
Understanding the biological properties of dormant CSCs and their reactivation mechanisms is essential for developing therapeutic approaches to prevent cancer recurrence and metastasis. It has been demonstrated that CD13 is a semiquiescent CSC marker in human liver cancer cell lines and clinical samples. CD13 protects cells from apoptosis after genotoxic chemo/radiation stress by causing the cells to predominate in the G0 phase of the cell cycle and reducing ROS-induced DNA damage.Citation76 Another study showed that CD13+ liver CSCs can sustain quiescence and resistance to chemotherapeutic agents via aerobic metabolism of tyrosine.Citation77 Side population (SP) CSCs are surrounded by a specialized CSC niche rich in laminin-332, which maintains liver CSCs in a quiescent state.Citation42
The effect of liver CSCs in immune evasion
In HCC, immune evasion is established early and is a progressive and continual process that peaks at intermediate stage II tumors.Citation78 Cancer cells, dissociated from the immunosuppressive microenvironment of the primary tumor, become vulnerable to immune surveillance which requires escape from immune-mediated elimination if they are to form metastases.Citation79 CSC immune evasion is key to maintaining the tumorigenic process of CSCs by changing their own molecular expression and reprogramming the immune response.Citation80,Citation81 Natural killer (NK) cells have been demonstrated to play critical roles in the first line of immunological defense against cancer development, including HCC.Citation82 EpCAM+ liver CSCs have been shown to be resistant to NK cell-mediated cytotoxicity by upregulating CEACAM1 expression.Citation83 CD133+ liver CSCs show a strong immune escape ability by interacting with lymphatic endothelial cells and activating IL-17A signaling.Citation52 One study reported that liver CSCs can evade the adaptive immune response through activated regulatory CD4+/CD25+/FoxP3+ T cells (Tregs) in a paracrine manner.Citation84 The mechanism of liver CSCs immune evasion requires further investigation.
Metabolism reprogramming is crucial to maintain liver CSC stemness
Metabolic reprogramming is considered a major hallmark of tumorigenesis. This reprogramming provides supplementary energy, cellular building blocks, and redox deficits for their eventual survival and metastases.Citation85,Citation86 CSCs exhibit altered metabolism and energy balance to meet a hostile TME for survival and stemness maintenance.Citation87,Citation88 In HCC, cells have been shown to retrodifferentiate to CSCs, accompanied by metabolic reprogramming, including changes in mitochondrial activity with reduced membrane potential, low ATP production, and high lactate production.Citation89 Wei et al. demonstrated that liver CSCs regulate stemness properties by regulating the mitochondrial respiratory function.Citation90
Glucose metabolism is essential for generating sufficient energy to maintain complex tissues, including tumors. Cancer cells must undergo critical changes to meet their altered energy needs by decoupling glycolysis from pyruvate oxidation, thereby increasing the glycolysis pathway despite the availability of sufficient oxygen, a process known as the Warburg effect.Citation91 Studies have shown that glucose metabolism reprogramming, including upregulation of glycolysis,Citation92–94 increased oxidative phosphorylation (OXPHOS),Citation95 and elevated glucose uptakeCitation96 regulate the stemness of liver CSCs.
Reprogramming of amino acid metabolism is also crucial for maintaining CSC stemness. Chemoresistant HCC cells were found to exhibit a CSC phenotype with altered metabolism in a metabolically quiescent state with glucose independence and relied on glutamine metabolism compared to chemo-sensitive HCC cells.Citation97 Similarly, glutamine metabolism has been confirmed to play an important role in liver CSC maintenance, and that targeting glutamine metabolism or glutaminase 1 (GLS1) could suppress CSC traits in HCC.Citation98 These studies indicated that the survival and self-renewal of liver CSCs depended on glutamine. However, a recent study found that liver CSCs could maintain their stemness characteristics even upon glutamine deprivation.Citation99 CD13+ liver CSCs were reported to be dependent on the aerobic metabolism of tyrosine rather than glucose as an energy source, and tyrosine metabolism could also generate nuclear acetyl-CoA to acetylate and stabilize Foxd3, thereby allowing CD13+ CSCs cells to sustain quiescence and resistance to chemotherapeutic agents.Citation77 Alanine-glyoxylate aminotransferase (AGXT) has been demonstrated to regulate the metabolic processes of serine, glycine, and alanine, and one study implied that the metabolic processes of serine, glycine, and alanine regulated by AGXT were crucial for liver CSC stemness.Citation100
Lipid metabolism appears to be a critical property of liver CSCs. Fatty acid metabolism is the core of lipid status harmonization in lipid metabolism. In malignant cells, carbon is characteristically diverted from energy production to fatty acids for biosynthesis of membranes and signaling molecules. Fatty acid oxidation (FAO)-mediated energy generation is critical to cancer cell survival and metastasis, and ectopic activation of FAO maintains CSCs under conditions of glycolytic deficiency.Citation101 It has been reported that activation of FAO and inhibition of oxidative phosphorylation and ROS production play an important role in the maintenance of liver CSCs stemness.Citation102 Another study showed that an increase in FAO activity facilitates the maintenance and self-renewal of liver CSCs.Citation103
Liver CSCs promote angiogenesis
Solid tumors require new blood vessels for their growth and metastasis. Considerable evidence has characterized links between CSCs and tumor angiogenesis in the surrounding microenvironment, which are directly associated with cancer development and metastasis. Studies have shown that CSCs from several cancers, such as glioblastoma,Citation104 ovarian cancer,Citation105 lung cancerCitation106 and breast cancer,Citation107 can transdifferentiate into endothelial cells and further form functional neovasculature. Yang et al. determined that high expression levels of liver CSC biomarkers were related to tumor angiogenesis and poor prognosis of HCC.Citation108 It was previously demonstrated that cancer stem-like sphere cells from HCC cells were able to differentiate into endothelial cells, and this process was independent of VEGF and NOTCH signaling, but dependent on the activation of Akt and IKK.Citation109 Conigliaro et al. demonstrated that CD90+ liver CSCs could affect endothelial cells and promote angiogenesis in a pro-metastatic manner by releasing exosomes containing H19 lncRNA.Citation110 CD133+ liver CSCs have also been shown to promote tumor angiogenesis through neurotensin/interleukin-8/CXCL1 signaling.Citation111 The stem cell-associated transcription factor Oct4 was recently shown to regulate the transition of CSCs to tumor endothelial-like cells in human liver cancer.Citation112 These studies suggest that liver CSCs predominantly promote angiogenesis through paracrine effects or transdifferentiation into endothelial cells, which may contribute to the development of recurrence, metastasis, and angiogenic treatment resistance.
Conclusions
Metastasis is a complex tumor process that is the most lethal characteristic of cancer. Although usually not appearing clinically for years after initial discovery of a primary cancer, the presence of a tiny fraction of the malignant cells which are treatment-resistant, phenotypically CSCs, have been identified as responsible for tumor metastasis. Therefore, there is a need for more effective therapies that can effectively eradicate these residual cells. However, the development of effective treatments for metastases depends on the understanding of the mechanisms underlying the metastatic process from start to finish. Not all cells in a tumor can migrate from the primary site and metastasize. Only the relatively few cells circulating in the blood have been implicated, CSCs. An increasing number of studies have revealed that liver CSCs play an important role in various processes of HCC metastasis, including cell dissemination into the blood, adaptation to harsh environments, and construction of metastatic foci (). As such, liver CSCs could become metastasis-initiating cells (MICs) by several cellular processes, including EMT, CSC niche interactions, ECM remodeling, then intravasate into the blood vessels, retaining their original stem cell phenotype. Liver CSCs can survive in the blood or other harsh environments through dormancy, sustained quiescence, and metabolic reprogramming. Liver CSCs play an important role in angiogenesis which enables the formation of metastatic lesions. In this review we have summarized the role of liver CSCs throughout the biological programs of the metastatic cascade. Understanding the role of liver CSCs in regulating HCC metastasis provides the critical foundation from which innovative new approaches and therapies can be developed to prevent or treat metastatic HCC.
Disclosure statement
No potential conflict of interest was reported by the author(s).
Additional information
Funding
Notes on contributors
Qinghui Niu
Qinghui Niu, Graduated from The Affiliated Hospital of Qingdao University and is now a physician at Liver Disease Center, The Affiliated Hospital of Qingdao University.
Susu Ye
SuSu Ye, Graduated from the Peking Union Medical College Hospital and is now a physician at Liver Disease Center, The Affiliated Hospital of Qingdao University.
Liu Zhao
Liu Zhao, Graduated from the Harbin Medical University and is now a physician at Liver Disease Center, The Affiliated Hospital of Qingdao University.
Yanzhi Qian
Yanzhi Qian, Graduated from the Chongqing Medical University and is now a physician at School Hospital, Qingdao University of Science and Technology.
Fengchao Liu
Fengchao Liu, Graduated from the Chongqing Medical University and is now a physician at Liver Disease Center, The Affiliated Hospital of Qingdao University.
References
- Forner A, Reig M, Bruix J. Hepatocellular carcinoma. Lancet. 2018;391(10127):1301–9. doi:10.1016/S0140-6736(18)30010-2.
- Villanueva A, Longo DL. Hepatocellular Carcinoma. N Engl J Med. 2019;380(15):1450–62. doi:10.1056/NEJMra1713263.
- Sapisochin G, Bruix J. Liver transplantation for hepatocellular carcinoma: outcomes and novel surgical approaches. Nat Rev Gastroenterol Hepatol. 2017;14(4):203–17. doi:10.1038/nrgastro.2016.193.
- Vibert E, Schwartz M, Olthoff KM. Advances in resection and transplantation for hepatocellular carcinoma. J Hepatol. 2020;72(2):262–76. doi:10.1016/j.jhep.2019.11.017.
- Bodzin AS, Lunsford KE, Markovic D, Harlander-Locke MP, Busuttil RW, Agopian VG. Predicting mortality in patients developing recurrent hepatocellular carcinoma after liver transplantation: impact of treatment modality and recurrence characteristics. Ann Surg. 2017;266(1):118–25. doi:10.1097/SLA.0000000000001894.
- Sapisochin G, Goldaracena N, Astete S, Laurence JM, Davidson D, Rafael E, Castells L, Sandroussi C, Bilbao I, Dopazo C, et al. Benefit of treating hepatocellular carcinoma recurrence after liver transplantation and analysis of prognostic factors for survival in a large Euro-American Series. Ann Surg Oncol. 2015;22(7):2286–94. doi:10.1245/s10434-014-4273-6.
- Castaneda M, den Hollander P, Kuburich NA, Rosen JM, Mani SA. Mechanisms of cancer metastasis. Semin Cancer Biol. 2022;87:17–31. doi:10.1016/j.semcancer.2022.10.006.
- Ganesh K, Massague J. Targeting metastatic cancer. Nat Med. 2021;27(1):34–44. doi:10.1038/s41591-020-01195-4.
- Fares J, Fares MY, Khachfe HH, Salhab HA, Fares Y. Molecular principles of metastasis: a hallmark of cancer revisited. Signal Transduct Target Ther. 2020;5(1):28. doi:10.1038/s41392-020-0134-x.
- Massague J, Ganesh K. Metastasis-initiating cells and ecosystems. Cancer Discov. 2021;11(4):971–94. doi:10.1158/2159-8290.CD-21-0010.
- de Sousae Melo F, Kurtova AV, Harnoss JM, Kljavin N, Hoeck JD, Hung J, Anderson JE, Storm EE, Modrusan Z, Koeppen H, et al. A distinct role for Lgr5(+) stem cells in primary and metastatic colon cancer. Nature. 2017;543(7647):676–680. doi:10.1038/nature21713.
- Peitzsch C, Tyutyunnykova A, Pantel K, Dubrovska A. Cancer stem cells: the root of tumor recurrence and metastases. Semin Cancer Biol. 2017;44:10–24. doi:10.1016/j.semcancer.2017.02.011.
- Zhou J, Liu S, Wang Y, Dai W, Zou H, Wang S, Zhang J, Pan J. Salinomycin effectively eliminates cancer stem-like cells and obviates hepatic metastasis in uveal melanoma. Mol Cancer. 2019;18(1):159. doi:10.1186/s12943-019-1068-1.
- Batlle E, Clevers H. Cancer stem cells revisited. Nat Med. 2017;23(10):1124–34. doi:10.1038/nm.4409.
- Nio K, Yamashita T, Kaneko S. The evolving concept of liver cancer stem cells. Mol Cancer. 2017;16(1):4. doi:10.1186/s12943-016-0572-9.
- Dai X, Guo Y, Hu Y, Bao X, Zhu X, Fu Q, Zhang H, Tong Z, Liu L, Zheng Y, et al. Immunotherapy for targeting cancer stem cells in hepatocellular carcinoma. Theranostics. 2021;11(7):3489–501. doi:10.7150/thno.54648.
- Chen Z, Lu T, Huang L, Wang Z, Yan Z, Guan Y, Hu W, Fan Z, Zhu P. Circular RNA cia-MAF drives self-renewal and metastasis of liver tumor-initiating cells via transcription factor MAFF. J Clin Invest. 2021;131(19). doi:10.1172/JCI148020.
- Yamashita T, Honda M, Nakamoto Y, Baba M, Nio K, Hara Y, Zeng SS, Hayashi T, Kondo M, Takatori H, et al. Discrete nature of EpCAM+ and CD90+ cancer stem cells in human hepatocellular carcinoma. Hepatology. 2013;57(4):1484–97. doi:10.1002/hep.26168.
- Jayachandran A, Dhungel B, Steel JC. Epithelial-to-mesenchymal plasticity of cancer stem cells: therapeutic targets in hepatocellular carcinoma. J Hematol Oncol. 2016;9(1):74. doi:10.1186/s13045-016-0307-9.
- Mani SA, Guo W, Liao MJ, Eaton EN, Ayyanan A, Zhou AY, Brooks M, Reinhard F, Zhang CC, Shipitsin M, et al. The epithelial-mesenchymal transition generates cells with properties of stem cells. Cell. 2008;133(4):704–15. doi:10.1016/j.cell.2008.03.027.
- Shibue T, Weinberg RA. EMT, CSCs, and drug resistance: the mechanistic link and clinical implications. Nat Rev Clin Oncol. 2017;14(10):611–29. doi:10.1038/nrclinonc.2017.44.
- Huang Y, Zhou B, Luo H, Mao J, Huang Y, Zhang K, Mei C, Yan Y, Jin H, Gao J, et al. ZnAs@SiO2nanoparticles as a potential anti-tumor drug for targeting stemness and epithelial-mesenchymal transition in hepatocellular carcinoma via SHP-1/JAK2/STAT3 signaling. Theranostics. 2019;9(15):4391–4408. doi:10.7150/thno.32462.
- Chen Z, Xiang L, Li L, Ou H, Fang Y, Xu Y, Liu Q, Hu Z, Huang Y, Li X, et al. TGF-β1 induced deficiency of linc00261 promotes epithelial–mesenchymal-transition and stemness of hepatocellular carcinoma via modulating SMAD3. J Transl Med. 2022;20(1):75. doi:10.1186/s12967-022-03276-z.
- Huan HB, Yang DP, Wen XD, Chen X-J, Zhang L, Wu L-L, Bie P, Xia F. HOXB7 accelerates the malignant progression of hepatocellular carcinoma by promoting stemness and epithelial-mesenchymal transition. J Exp Clin Cancer Res. 2017;36(1):86. doi:10.1186/s13046-017-0559-4.
- Zhou JN, Zeng Q, Wang HY, Zhang B, Li S-T, Nan X, Cao N, Fu C-J, Yan X-L, Jia Y-L, et al. MicroRNA-125b attenuates epithelial-mesenchymal transitions and targets stem-like liver cancer cells through small mothers against decapentaplegic 2 and 4. Hepatology. 2015;62(3):801–15. doi:10.1002/hep.27887.
- Ou H, Chen Z, Xiang L, Fang Y, Xu Y, Liu Q, Hu Z, Li X, Huang Y, Yang D, et al. Frizzled 2-induced epithelial-mesenchymal transition correlates with vasculogenic mimicry, stemness, and Hippo signaling in hepatocellular carcinoma. Cancer Sci. 2019;110(4):1169–82. doi:10.1111/cas.13949.
- Fung SW, Cheung PF, Yip CW, Ng LWC, Cheung TT, Chong CCN, Lee C, Lai PBS, Chan AWH, Tsao GSW, et al. The ATP-binding cassette transporter ABCF1 is a hepatic oncofetal protein that promotes chemoresistance, EMT and cancer stemness in hepatocellular carcinoma. Cancer Lett. 2019;457:98–109. doi:10.1016/j.canlet.2019.05.010.
- Modi SJ, Kulkarni VM. Discovery of VEGFR-2 inhibitors exerting significant anticancer activity against CD44+ and CD133+ cancer stem cells (CSCs): reversal of TGF-beta induced epithelial-mesenchymal transition (EMT) in hepatocellular carcinoma. Eur J Med Chem. 2020;207:112851. doi:10.1016/j.ejmech.2020.112851.
- Fan QM, Jing YY, Yu GF, Kou X-R, Ye F, Gao L, Li R, Zhao Q-D, Yang Y, Lu Z-H, et al. Tumor-associated macrophages promote cancer stem cell-like properties via transforming growth factor-beta1-induced epithelial–mesenchymal transition in hepatocellular carcinoma. Cancer Lett. 2014;352(2):160–168. doi:10.1016/j.canlet.2014.05.008.
- Jing L, Ruan Z, Sun H, Li Q, Han L, Huang L, Yu S, Wang Y, Guo H, Jiao M, et al. Epithelial–mesenchymal transition induced cancer-stem-cell-like characteristics in hepatocellular carcinoma. J Cell Physiol. 2019;234(10):18448–18458. doi:10.1002/jcp.28480.
- Yin X, Zhang BH, Zheng SS, Gao D-M, Qiu S-J, Wu W-Z, Ren Z-G. Coexpression of gene Oct4 and nanog initiates stem cell characteristics in hepatocellular carcinoma and promotes epithelial-mesenchymal transition through activation of Stat3/Snail signaling. J Hematol Oncol. 2015;8(1):23. doi:10.1186/s13045-015-0119-3.
- Nishiyama M, Tsunedomi R, Yoshimura K, Hashimoto N, Matsukuma S, Ogihara H, Kanekiyo S, Iida M, Sakamoto K, Suzuki N, et al. Metastatic ability and the epithelial-mesenchymal transition in induced cancer stem-like hepatoma cells. Cancer Sci. 2018;109(4):1101–9. doi:10.1111/cas.13527.
- Mitra A, Satelli A, Xia X, Cutrera J, Mishra L, Li S. Cell-surface Vimentin: a mislocalized protein for isolating csVimentin(+) CD133(-) novel stem-like hepatocellular carcinoma cells expressing EMT markers. Int J Cancer. 2015;137(2):491–496. doi:10.1002/ijc.29382.
- Suhail Y, Cain MP, Vanaja K, Kurywchak PA, Levchenko A, Kalluri RK. Systems biology of cancer metastasis. Cell Syst. 2019;9(2):109–127. doi:10.1016/j.cels.2019.07.003.
- Jiang J, Ye F, Yang X, Zong C, Gao L, Yang Y, Zhao Q, Han Z, Wei L. Peri-tumor associated fibroblasts promote intrahepatic metastasis of hepatocellular carcinoma by recruiting cancer stem cells. Cancer Lett. 2017;404:19–28. doi:10.1016/j.canlet.2017.07.006.
- Gasmi I, Machou C, Rodrigues A, Brouillet A, Nguyen TC, Rousseau B, Guillot A, Rodriguez C, Demontant V, Ait-Ahmed Y, et al. Interleukin-17 programs liver progenitor cell transformation into cancer stem cells through miR-122 downregulation with increased risk of primary liver cancer initiation. Int J Biol Sci. 2022;18(5):1944–60. doi:10.7150/ijbs.70408.
- Lai FB, Liu WT, Jing YY, Yu G-F, Han Z-P, Yang X, Zeng J-X, Zhang H-J, Shi R-Y, Li X-Y, et al. Lipopolysaccharide supports maintaining the stemness of CD133+ hepatoma cells through activation of the NF-κB/HIF-1α pathway. Cancer Lett. 2016;378(2):131–141. doi:10.1016/j.canlet.2016.05.014.
- Wan S, Zhao E, Kryczek I, Vatan L, Sadovskaya A, Ludema G, Simeone DM, Zou W, Welling TH. Tumor-associated macrophages produce interleukin 6 and signal via STAT3 to promote expansion of human hepatocellular carcinoma stem cells. Gastroenterology. 2014;147(6):1393–1404. doi:10.1053/j.gastro.2014.08.039.
- Li Y, Wang R, Xiong S, Wang X, Zhao Z, Bai S, Wang Y, Zhao Y, Cheng B. Cancer-associated fibroblasts promote the stemness of CD24(+) liver cells via paracrine signaling. J Mol Med (Berl). 2019;97(2):243–255. doi:10.1007/s00109-018-1731-9.
- Mitra A, Yan J, Xia X, Zhou S, Chen J, Mishra L, Li S. IL6-mediated inflammatory loop reprograms normal to epithelial-mesenchymal transition+ metastatic cancer stem cells in preneoplastic liver of transforming growth factor beta–deficient β2-spectrin+/− mice. Hepatology. 2017;65(4):1222–1236. doi:10.1002/hep.28951.
- Plaks V, Kong N, Werb Z. The cancer stem cell niche: how essential is the niche in regulating stemness of tumor cells? Cell Stem Cell. 2015;16(3):225–38. doi:10.1016/j.stem.2015.02.015.
- Govaere O, Wouters J, Petz M, Vandewynckel Y-P, Van den Eynde K, Van den Broeck A, Verhulst S, Dollé L, Gremeaux L, Ceulemans A, et al. Laminin-332 sustains chemoresistance and quiescence as part of the human hepatic cancer stem cell niche. J Hepatol. 2016;64(3):609–17. doi:10.1016/j.jhep.2015.11.011.
- Cui CP, Wong CC, Kai AK, Ho DWH, Lau EYT, Tsui Y-M, Chan L-K, Cheung T-T, Chok KSH, Chan ACY, et al. SENP1 promotes hypoxia-induced cancer stemness by HIF-1α deSumoylation and SENP1/HIF-1α positive feedback loop. Gut. 2017;66(12):2149–59. doi:10.1136/gutjnl-2016-313264.
- Ling S, Shan Q, Zhan Q, Ye Q, Liu P, Xu S, He X, Ma J, Xiang J, Jiang G, et al. USP22 promotes hypoxia-induced hepatocellular carcinoma stemness by a HIF1α/USP22 positive feedback loop upon TP53 inactivation. Gut. 2020;69(7):1322–1334. doi:10.1136/gutjnl-2019-319616.
- Won C, Kim BH, Yi EH, Choi K-J, Kim E-K, Jeong J-M, Lee J-H, Jang J-J, Yoon J-H, Jeong W-I, et al. Signal transducer and activator of transcription 3-mediated CD133 up-regulation contributes to promotion of hepatocellular carcinoma. Hepatology. 2015;62(4):1160–73. doi:10.1002/hep.27968.
- Govaert KM, Emmink BL, Nijkamp MW, Cheung ZJ, Steller EJA, Fatrai S, de Bruijn MT, Kranenburg O, Borel Rinkes IHM. Hypoxia after liver surgery imposes an aggressive cancer stem cell phenotype on residual tumor cells. Ann Surg. 2014;259(4):750–759. doi:10.1097/SLA.0b013e318295c160.
- Wang X, Mao J, Zhou T, Chen X, Tu H, Ma J, Li Y, Ding Y, Yang Y, Wu H, et al. Hypoxia-induced myeloid derived growth factor promotes hepatocellular carcinoma progression through remodeling tumor microenvironment. Theranostics. 2021;11(1):209–21. doi:10.7150/thno.49327.
- Wei R, Zhu WW, Yu GY, Wang X, Gao C, Zhou X, Lin Z-F, Shao W-Q, Wang S-H, Lu M, et al. S100 calcium-binding protein A9 from tumor-associated macrophage enhances cancer stem cell-like properties of hepatocellular carcinoma. Int J Cancer. 2021;148(5):1233–44. doi:10.1002/ijc.33371.
- Muramatsu S, Tanaka S, Mogushi K, Adikrisna R, Aihara A, Ban D, Ochiai T, Irie T, Kudo A, Nakamura N, et al. Visualization of stem cell features in human hepatocellular carcinoma reveals in vivo significance of tumor-host interaction and clinical course. Hepatology. 2013;58(1):218–28. doi:10.1002/hep.26345.
- Loh JJ, Li TW, Zhou L, Wong T-L, Liu X, Ma VWS, Lo C-M, Man K, Lee TK, Ning W, et al. FSTL1 secreted by activated fibroblasts promotes hepatocellular carcinoma metastasis and stemness. Cancer Res. 2021;81(22):5692–705. doi:10.1158/0008-5472.CAN-20-4226.
- Liu C, Liu L, Chen X, Cheng J, Zhang H, Zhang C, Shan J, Shen J, Qian C. LSD1 stimulates cancer-associated fibroblasts to drive Notch3-dependent self-renewal of liver cancer stem–like cells. Cancer Res. 2018;78(4):938–949. doi:10.1158/0008-5472.CAN-17-1236.
- Wei Y, Shi D, Liang Z, Liu Y, Li Y, Xing Y, Liu W, Ai Z, Zhuang J, Chen X, et al. IL-17A secreted from lymphatic endothelial cells promotes tumorigenesis by upregulation of PD-L1 in hepatoma stem cells. J Hepatol. 2019;71(6):1206–15. doi:10.1016/j.jhep.2019.08.034.
- Kai F, Drain AP, Weaver VM. The extracellular matrix modulates the metastatic journey. Dev Cell. 2019;49(3):332–46. doi:10.1016/j.devcel.2019.03.026.
- Schrader J, Gordon-Walker TT, Aucott RL, van Deemter M, Quaas A, Walsh S, Benten D, Forbes SJ, Wells RG, Iredale JP, et al. Matrix stiffness modulates proliferation, chemotherapeutic response, and dormancy in hepatocellular carcinoma cells. Hepatology. 2011;53(4):1192–205. doi:10.1002/hep.24108.
- Wang C, Jiang X, Huang B, Zhou W, Cui X, Zheng C, Liu F, Bi J, Zhang Y, Luo H, et al. Inhibition of matrix stiffness relating integrin β1 signaling pathway inhibits tumor growth in vitro and in hepatocellular cancer xenografts. BMC Cancer. 2021;21(1):1276. doi:10.1186/s12885-021-08982-3.
- Tian B, Luo Q, Ju Y, Song G. A soft matrix enhances the cancer stem cell phenotype of HCC cells. Int J Mol Sci. 2019;20(11):2831. doi:10.3390/ijms20112831.
- Ng KY, Shea QT, Wong TL, Luk ST, Tong M, Lo C-M, Man K, Yun J-P, Guan X-Y, Lee TK, et al. Chemotherapy-enriched THBS2-deficient cancer stem cells drive hepatocarcinogenesis through matrix softness induced histone H3 modifications. Adv Sci (Weinh). 2021;8(5):2002483. doi:10.1002/advs.202002483.
- Wei J, Yao J, Yang C, Mao Y, Zhu D, Xie Y, Liu P, Yan M, Ren L, Lin Y, et al. Heterogeneous matrix stiffness regulates the cancer stem-like cell phenotype in hepatocellular carcinoma. J Transl Med. 2022;20(1):555. doi:10.1186/s12967-022-03778-w.
- Li H, Sun Y, Li Q, Luo Q, Song G. Matrix stiffness potentiates stemness of liver cancer stem cells possibly via the Yes-Associated Protein Signal. ACS Biomater Sci Eng. 2022;8(2):598–609. doi:10.1021/acsbiomaterials.1c00558.
- Zhao W, Lv M, Yang X, Zhou J, Xing B, Zhang Z. Liver tumor-initiating cells initiate the formation of a stiff cancer stem cell microenvironment niche by secreting LOX. Carcinogenesis. 2022;43(8):766–78. doi:10.1093/carcin/bgac035.
- Lin D, Shen L, Luo M, Zhang K, Li J, Yang Q, Zhu F, Zhou D, Zheng S, Chen Y, et al. Circulating tumor cells: biology and clinical significance. Signal Transduct Target Ther. 2021;6(1):404. doi:10.1038/s41392-021-00817-8.
- Liu T, Xu H, Huang M, Ma W, Saxena D, Lustig RA, Alonso-Basanta M, Zhang Z, O’Rourke DM, Zhang L, et al. Circulating Glioma Cells Exhibit Stem Cell-like Properties. Cancer Res. 2018;78(23):6632–42. doi:10.1158/0008-5472.CAN-18-0650.
- Theodoropoulos PA, Polioudaki H, Agelaki S, Kallergi G, Saridaki Z, Mavroudis D, Georgoulias V. Circulating tumor cells with a putative stem cell phenotype in peripheral blood of patients with breast cancer. Cancer Lett. 2010;288(1):99–106. doi:10.1016/j.canlet.2009.06.027.
- Grillet F, Bayet E, Villeronce O, Zappia L, Lagerqvist EL, Lunke S, Charafe-Jauffret E, Pham K, Molck C, Rolland N, et al. Circulating tumour cells from patients with colorectal cancer have cancer stem cell hallmarks in ex vivo culture. Gut. 2017;66(10):1802–10. doi:10.1136/gutjnl-2016-311447.
- Wan S, Kim TH, Smith KJ, Delaney R, Park G-S, Guo H, Lin E, Plegue T, Kuo N, Steffes J, et al. New labyrinth microfluidic device detects circulating tumor cells expressing cancer stem cell Marker and circulating tumor microemboli in hepatocellular carcinoma. Sci Rep. 2019;9(1):18575. doi:10.1038/s41598-019-54960-y.
- Schulze K, Gasch C, Staufer K, Nashan B, Lohse AW, Pantel K, Riethdorf S, Wege H. Presence of EpCAM-positive circulating tumor cells as biomarker for systemic disease strongly correlates to survival in patients with hepatocellular carcinoma. Int J Cancer. 2013;133(9):2165–2171. doi:10.1002/ijc.28230.
- Ahn JC, Teng PC, Chen PJ, Posadas E, Tseng H-R, Lu SC, Yang JD. Detection of circulating tumor cells and their implications as a biomarker for diagnosis, prognostication, and therapeutic monitoring in hepatocellular carcinoma. Hepatology. 2021;73(1):422–436. doi:10.1002/hep.31165.
- Nio K, Yamashita T, Okada H, Kondo M, Hayashi T, Hara Y, Nomura Y, Zeng SS, Yoshida M, Hayashi T, et al. Defeating EpCAM(+) liver cancer stem cells by targeting chromatin remodeling enzyme CHD4 in human hepatocellular carcinoma. J Hepatol. 2015;63(5):1164–1172. doi:10.1016/j.jhep.2015.06.009.
- Sun YF, Xu Y, Yang XR, Guo W, Zhang X, Qiu S-J, Shi R-Y, Hu B, Zhou J, Fan J, et al. Circulating stem cell-like epithelial cell adhesion molecule-positive tumor cells indicate poor prognosis of hepatocellular carcinoma after curative resection. Hepatology. 2013;57(4):1458–68. doi:10.1002/hep.26151.
- Yang ZF, Ngai P, Ho DW, Yu WC, Ng MNP, Lau CK, Li MLY, Tam KH, Lam CT, Poon RTP, et al. Identification of local and circulating cancer stem cells in human liver cancer. Hepatology. 2008;47(3):919–28. doi:10.1002/hep.22082.
- Fan ST, Yang ZF, Ho DW, Ng MN, Yu WC, Wong J. Prediction of posthepatectomy recurrence of hepatocellular carcinoma by circulating cancer stem cells: a prospective study. Ann Surg. 2011;254(4):569–76. doi:10.1097/SLA.0b013e3182300a1d.
- Liu S, Li N, Yu X, Xiao X, Cheng K, Hu J, Wang J, Zhang D, Cheng S, Liu S, et al. Expression of intercellular adhesion molecule 1 by hepatocellular carcinoma stem cells and circulating tumor cells. Gastroenterology. 2013;144(5):1031–1041.e10. doi:10.1053/j.gastro.2013.01.046.
- Guo W, Sun YF, Shen MN, Ma X-L, Wu J, Zhang C-Y, Zhou Y, Xu Y, Hu B, Zhang M, et al. Circulating tumor cells with stem-like phenotypes for diagnosis, prognosis, and therapeutic response evaluation in hepatocellular carcinoma. Clin Cancer Res. 2018;24(9):2203–13. doi:10.1158/1078-0432.CCR-17-1753.
- Malladi S, Macalinao DG, Jin X, He L, Basnet H, Zou Y, de Stanchina E, Massagué J. Metastatic latency and immune evasion through autocrine inhibition of WNT. Cell. 2016;165(1):45–60. doi:10.1016/j.cell.2016.02.025.
- Maccalli C, Rasul KI, Elawad M, Ferrone S. The role of cancer stem cells in the modulation of anti-tumor immune responses. Semin Cancer Biol. 2018;53:189–200. doi:10.1016/j.semcancer.2018.09.006.
- Haraguchi N, Ishii H, Mimori K, Tanaka F, Ohkuma M, Kim HM, Akita H, Takiuchi D, Hatano H, Nagano H, et al. CD13 is a therapeutic target in human liver cancer stem cells. J Clin Invest. 2010;120(9):3326–39. doi:10.1172/JCI42550.
- Sun L, Zhang L, Chen J, Li C, Sun H, Wang J, Xiao H. Activation of tyrosine metabolism in CD13+ cancer stem cells drives relapse in hepatocellular carcinoma. Cancer Res Treat. 2020;52(2):604–621. doi:10.4143/crt.2019.444.
- Nguyen PHD, Wasser M, Tan CT, Lim CJ, Lai HLH, Seow JJW, DasGupta R, Phua CZJ, Ma S, Yang J, et al. Trajectory of immune evasion and cancer progression in hepatocellular carcinoma. Nat Commun. 2022;13(1):1441. doi:10.1038/s41467-022-29122-w.
- Mohme M, Riethdorf S, Pantel K. Circulating and disseminated tumour cells — mechanisms of immune surveillance and escape. Nat Rev Clin Oncol. 2017;14(3):155–167. doi:10.1038/nrclinonc.2016.144.
- Bayik D, Lathia JD. Cancer stem cell-immune cell crosstalk in tumour progression. Nat Rev Cancer. 2021;21(8):526–536. doi:10.1038/s41568-021-00366-w.
- Hsu JM, Xia W, Hsu YH, Chan L-C, Yu W-H, Cha J-H, Chen C-T, Liao H-W, Kuo C-W, Khoo K-H, et al. STT3-dependent PD-L1 accumulation on cancer stem cells promotes immune evasion. Nat Commun. 2018;9(1):1908. doi:10.1038/s41467-018-04313-6.
- Sung PS, Jang JW. Natural killer cell dysfunction in hepatocellular carcinoma: Pathogenesis and clinical implications. Int J Mol Sci. 2018;19(11):3648. doi:10.3390/ijms19113648.
- Park DJ, Sung PS, Kim JH, Lee GW, Jang JW, Jung ES, Bae SH, Choi JY, Yoon SK. EpCAM-high liver cancer stem cells resist natural killer cell–mediated cytotoxicity by upregulating CEACAM1. J Immunother Cancer. 2020;8(1):e000301. doi:10.1136/jitc-2019-000301.
- Lo Re O, Mazza T, Giallongo S, Sanna P, Rappa F, Vinh Luong T, Li Volti G, Drovakova A, Roskams T, Van Haele M, et al. Loss of histone macroH2A1 in hepatocellular carcinoma cells promotes paracrine-mediated chemoresistance and CD4+CD25+FoxP3+regulatory T cells activation. Theranostics. 2020;10(2):910–924. doi:10.7150/thno.35045.
- Wei Q, Qian Y, Yu J, Wong CC. Metabolic rewiring in the promotion of cancer metastasis: mechanisms and therapeutic implications. Oncogene. 2020;39(39):6139–56. doi:10.1038/s41388-020-01432-7.
- Martinez-Reyes I, Chandel NS. Cancer metabolism: looking forward. Nat Rev Cancer. 2021;21(10):669–80. doi:10.1038/s41568-021-00378-6.
- Xie Y, Ma S, Tong M. Metabolic plasticity of cancer stem cells in response to microenvironmental cues. Cancers (Basel). 2022;14(21):5345. doi:10.3390/cancers14215345.
- Wong TL, Che N, Ma S. Reprogramming of central carbon metabolism in cancer stem cells. Biochim Biophys Acta Mol Basis Dis. 2017;1863(7):1728–1738. doi:10.1016/j.bbadis.2017.05.012.
- Fekir K, Dubois-Pot-Schneider H, Desert R, Daniel Y, Glaise D, Rauch C, Morel F, Fromenty B, Musso O, Cabillic F, et al. Retrodifferentiation of human tumor hepatocytes to Stem Cells Leads to Metabolic Reprogramming and Chemoresistance. Cancer Res. 2019;79(8):1869–83. doi:10.1158/0008-5472.CAN-18-2110.
- Wei Z, Jia J, Heng G, Xu H, Shan J, Wang G, Liu C, Xia J, Zhou H, Wu M, et al. Sirtuin-1/Mitochondrial ribosomal protein S5 axis enhances the metabolic flexibility of liver cancer stem cells. Hepatology. 2019;70(4):1197–213. doi:10.1002/hep.30622.
- Vander Heiden MG, Cantley LC, Thompson CB. Understanding the Warburg effect: the metabolic requirements of cell proliferation. Science. 2009;324(5930):1029–33. doi:10.1126/science.1160809.
- Chen YY, Wang WH, Che L, Lan Y, Zhang L-Y, Zhan D-L, Huang Z-Y, Lin Z-N, Lin Y-C. BNIP3L-Dependent mitophagy promotes HBx-induced cancer stemness of hepatocellular carcinoma cells via glycolysis metabolism reprogramming. Cancers (Basel). 2020;12(3):655. doi:10.3390/cancers12030655.
- Bi L, Ren Y, Feng M, Meng P, Wang Q, Chen W, Jiao Q, Wang Y, Du L, Zhou F, et al. HDAC11 regulates Glycolysis through the LKB1/AMPK signaling pathway to maintain hepatocellular carcinoma stemness. Cancer Res. 2021;81(8):2015–28. doi:10.1158/0008-5472.CAN-20-3044.
- Gu Y, Ji F, Liu N, Zhao Y, Wei X, Hu S, Jia W, Wang XW, Budhu A, Ji J, et al. Loss of miR-192-5p initiates a hyperglycolysis and stemness positive feedback in hepatocellular carcinoma. J Exp Clin Cancer Res. 2020;39(1):268. doi:10.1186/s13046-020-01785-7.
- Liu G, Luo Q, Li H, Liu Q, Ju Y, Song G. Increased oxidative phosphorylation is required for stemness maintenance in liver cancer stem cells from hepatocellular carcinoma cell line HCCLM3 cells. Int J Mol Sci. 2020;21(15):5276. doi:10.3390/ijms21155276.
- Zhang HL, Wang MD, Zhou X, Qin C-J, Fu G-B, Tang L, Wu H, Huang S, Zhao L-H, Zeng M, et al. Blocking preferential glucose uptake sensitizes liver tumor-initiating cells to glucose restriction and sorafenib treatment. Cancer Lett. 2017;388:1–11. doi:10.1016/j.canlet.2016.11.023.
- Lee ACK, Lau PM, Kwan YW, Kong SK. Mitochondrial fuel dependence on glutamine drives chemo-resistance in the cancer stem cells of hepatocellular carcinoma. Int J Mol Sci. 2021;22(7):3315. doi:10.3390/ijms22073315.
- Li B, Cao Y, Meng G, Qian L, Xu T, Yan C, Luo O, Wang S, Wei J, Ding Y, et al. Targeting glutaminase 1 attenuates stemness properties in hepatocellular carcinoma by increasing reactive oxygen species and suppressing Wnt/beta-catenin pathway. EBioMedicine. 2019;39:239–54. doi:10.1016/j.ebiom.2018.11.063.
- Zhang HL, Chen P, Yan HX, Fu G-B, Luo F-F, Zhang J, Zhao S-M, Zhai B, Yu J-H, Chen L, et al. Targeting mTORC2/HDAC3 inhibits stemness of liver cancer cells against glutamine starvation. Adv Sci (Weinh). 2022;9(20):e2103887. doi:10.1002/advs.202103887.
- Ye P, Chi X, Yan X, Wu F, Liang Z, Yang WH. Alanine–glyoxylate aminotransferase sustains cancer stemness properties through the Upregulation of SOX2 and OCT4 in hepatocellular carcinoma cells. Biomolecules. 2022;12(5):668. doi:10.3390/biom12050668.
- Li H, Feng Z, He ML. Lipid metabolism alteration contributes to and maintains the properties of cancer stem cells. Theranostics. 2020;10(16):7053–69. doi:10.7150/thno.41388.
- Chen CL, Uthaya Kumar DB, Punj V, Xu J, Sher L, Tahara S, Hess S, Machida K. NANOG metabolically reprograms tumor-initiating stem-like cells through tumorigenic changes in oxidative phosphorylation and fatty acid metabolism. Cell Metab. 2016;23(1):206–219. doi:10.1016/j.cmet.2015.12.004.
- Li H, Song J, He Y, Liu Y, Liu Z, Sun W, Hu W, Lei Q-Y, Hu X, Chen Z, et al. CRISPR/Cas9 screens reveal that Hexokinase 2 enhances cancer stemness and Tumorigenicity by activating the ACSL4-fatty acid β -oxidation pathway. Adv Sci (Weinh). 2022;9(21):e2105126. doi:10.1002/advs.202105126.
- Gagliardi F, Narayanan A, Gallotti AL, Pieri V, Mazzoleni S, Cominelli M, Rezzola S, Corsini M, Brugnara G, Altabella L, et al. Enhanced SPARCL1 expression in cancer stem cells improves preclinical modeling of glioblastoma by promoting both tumor infiltration and angiogenesis. Neurobiol Dis. 2020;134:104705. doi:10.1016/j.nbd.2019.104705.
- Krishnapriya S, Sidhanth C, Manasa P, Sneha S, Bindhya S, Nagare RP, Ramachandran B, Vishwanathan P, Murhekar K, Shirley S, et al. Cancer stem cells contribute to angiogenesis and lymphangiogenesis in serous adenocarcinoma of the ovary. Angiogenesis. 2019;22(3):441–55. doi:10.1007/s10456-019-09669-x.
- Li L, Li JC, Yang H, Zhang X, Liu L-L, Li Y, Zeng T-T, Zhu Y-H, Li X-D, Li Y, et al. Expansion of cancer stem cell pool initiates lung cancer recurrence before angiogenesis. Proc Natl Acad Sci U S A. 2018;115(38):E8948–E57. doi:10.1073/pnas.1806219115.
- Tang W, Yu F, Yao H, Cui X, Jiao Y, Lin L, Chen J, Yin D, Song E, Liu Q, et al. miR-27a regulates endothelial differentiation of breast cancer stem like cells. Oncogene. 2014;33(20):2629–38. doi:10.1038/onc.2013.214.
- Yang XR, Xu Y, Yu B, Zhou J, Qiu S-J, Shi G-M, Zhang B-H, Wu W-Z, Shi Y-H, Wu B, et al. High expression levels of putative hepatic stem/progenitor cell biomarkers related to tumour angiogenesis and poor prognosis of hepatocellular carcinoma. Gut. 2010;59(7):953–62. doi:10.1136/gut.2008.176271.
- Zhao Z, Gao J, Li C, Xu X, Hu Y, Huang S. Reactive oxygen species induce endothelial differentiation of liver cancer stem-like sphere cells through the activation of Akt/IKK signaling pathway. Oxid Med Cell Longev. 2020;2020:1–11. doi:10.1155/2020/1621687.
- Conigliaro A, Costa V, Lo Dico A, Saieva L, Buccheri S, Dieli F, Manno M, Raccosta S, Mancone C, Tripodi M, et al. CD90+ liver cancer cells modulate endothelial cell phenotype through the release of exosomes containing H19 lncRNA. Mol Cancer. 2015;14(1):155. doi:10.1186/s12943-015-0426-x.
- Tang KH, Ma S, Lee TK, Chan YP, Kwan PS, Tong CM, Ng IO, Man K, To K-F, Lai PB, et al. CD133(+) liver tumor-initiating cells promote tumor angiogenesis, growth, and self-renewal through neurotensin/interleukin-8/CXCL1 signaling. Hepatology. 2012;55(3):807–820. doi:10.1002/hep.24739.
- Liu HL, Tang HT, Yang HL, Deng T-T, Xu Y-P, Xu S-Q, Peng L, Wang Z, Fang Q, Kuang X-Y, et al. Oct4 Regulates the Transition of cancer Stem-Like Cells to tumor Endothelial-Like Cells in Human liver cancer. Front Cell Dev Biol. 2020;8:563316. doi:10.3389/fcell.2020.563316.