ABSTRACT
Double minutes (DMs), extrachromosomal gene fragments found within certain tumors, have been noted to carry onco- and drug resistance genes contributing to tumor pathogenesis and progression. After screening for SUMO-related molecule expression within various tumor sample and cell line databases, we found that SUMO-conjugating enzyme UBC9 has been associated with genome instability and tumor cell DM counts, which was confirmed both in vitro and in vivo. Karyotyping determined DM counts post-UBC9 knockdown or SUMOylation inhibitor 2-D08, while RT-qPCR and Western blot were used to measure DM-carried gene expression in vitro. In vivo, fluorescence in situ hybridization (FISH) identified micronucleus (MN) expulsion. Western blot and immunofluorescence staining were then used to determine DNA damage extent, and a reporter plasmid system was constructed to detect changes in homologous recombination (HR) and non-homologous end joining (NHEJ) pathways. Our research has shown that UBC9 inhibition is able to attenuate DM formation and lower DM-carried gene expression, in turn reducing tumor growth and malignant phenotype, via MN efflux of DMs and lowering NHEJ activity to increase DNA damage. These findings thus reveal a relationship between heightened UBC9 activity, increased DM counts, and tumor progression, providing a potential approach for targeted therapies, via UBC9 inhibition.
Introduction
Double minutes (DMs) have been identified as a major manifestation of extrachromosomal oncogene amplification, which have been highly associated with tumorigenesis and chemoresistance.Citation1,Citation2 They have been found in a variety of tumor cells and are indicative of tumor genomic instability,Citation3 defined as changes in the rate at which whole chromosomes or large chromosomal fragments are gained or lost.Citation4 Indeed, DMs have played unique roles in tumorigenesis, where they have been involved in accelerating cancer evolution and therapeutic resistance.Citation5 As a result, DM counts have often been related to the degree of tumor malignancy.
DMs are small circular DNA molecules, lacking centromeres, that appear in pairs outside chromosomes; they range in size from 100s kb-Mb and are able to replicate autonomously. Indeed, they have recently been defined as a type of extrachromosomal DNA (ecDNA), which has been identified to be involved in a wide variety of biological processes.Citation6–8 In fact, ecDNAs are common in primary cancers,Citation9 where they promote oncogene expression,Citation6 both as a form of oncogene amplification, as well as serving as mobile “super-enhancer” elements for regulating the expression level of other oncogenes.Citation10 ecDNA occurrence has been reported to be closely connected to DNA damage.Citation11 With respect to DMs, they have also been found in cancer cells.Citation12 Their formation has been documented by previous studies to be associated with DNA damage and repair (DDR) responses, such as chromothripsisCitation13 and reconnection of chromosomal breakages.Citation14 Indeed, DDR levels could influence DM formation,Citation15,Citation16 as shown in a Sei-1 overexpression study, in which less DDR was associated with higher DM numbers.Citation16 As a result, regulating DM homeostasis within tumor cells could influence carcinoma development,Citation17,Citation18 and thus could serve as a target for developing anti-tumor therapeutic strategies.
Small ubiquitin-related modifier (SUMO), a type of post-translational modification (PTM), plays an important role in cell growth, maintaining genomic integrity and stability, signal transduction, gene replication, and transcriptional regulation, as well as tumor formation and development.Citation19–21 SUMOylation involves the E1 activating enzyme, comprising SUMO-activating enzyme subunit (SAE) 1 and 2, the E2 conjugating enzyme, and E3 ligases, such as protein inhibitor of activated STAT (PIAS)1–4. The sole SUMOylating E2 enzyme is ubiquitin conjugating enzyme E2I (UBE2I, also known as UBC9), which has been reported to be involved in tumor development.Citation22,Citation23 The isopeptidase activity of SUMO-specific proteases (SENPs), facilitating the de-conjugation of SUMO proteins, maintains the balance between SUMOylated and un-SUMOylated proteins. SUMOylation is involved in DDR, via acting as a “molecular glue” to recruit multiple repair factors and assemble large protein complexes.Citation24
DM formation, as mentioned above, is closely related to DDR. However, whether SUMOylation is involved in DM formation, as well as the exact underlying mechanisms connecting SUMOylation, DDR, and DM, remains unclear. In this study, we attempted to elucidate the role of SUMO-related molecules in genomic instability and DM formation through bioinformatics analysis. We found that tumor cell malignancy, as well as DM counts, was reduced with either knockdown or inhibition with 2-D08 (2‘,3’,4’−trihydroxy flavone), of SUMOylating E2 enzyme UBC9, both in vitro and in vivo, indicating the presence of homogeneously staining regions (HSR) has a strong correlation between SUMOylation and tumor cell DM production. Therefore, a possible anti-tumor therapeutic approach could be to inhibit UBC9 and eliminate DMs, in turn reducing ecDNA amplification of oncogenes within tumor cells.
Results
SUMOylation-related molecules have been associated with genome instability and DMs
DMs, a form of extrachromosomal amplification, have been associated with genome instability. Therefore, in this study, the first set of analyses examined the impact of SUMOylation molecules on different levels of genome instability, among different colorectal cancer samples obtained from the cBioPortal database.Citation25 We found that the expression levels for molecules involved in SUMOylation, such as UBC9 and SUMO1–3, were significantly higher in tumor samples with greater genomic instability (MSI and CIN), compared to stable ones (GS; ). This pattern also held true for our analysis of RNA-seq data of breast cancer samples from the TCGA database, in which the samples were classified into ones without (no DMs) or with DMs. Here, for all aforementioned molecules, their expression was also positively correlated with DM presence (). We then divided tumor cells from CCLE into three groups: no DMs, DMs+, and DMs++, according to their karyotypes, and found that this association of increased SUMOylation molecule expression with higher DM levels also applied for SAE2 and PIAS1, and that DMs++ had the greatest expression levels for UBC9, SAE2, PIAS1, and SUMO2 ( and S1). Therefore, based on these bioinformatics analyses, increased genomic instability and DM counts are likely associated with increased SUMOylation-related molecule expression. We then further confirmed the association of increased SUMOylation molecule expression with DMs in vitro by comparing two cell lines with similar genetic backgrounds: UACC-1598DM/UACC-1598HSR and Colo320DM/Colo320HSR, using RT-qPCR. In particular, from both lines, SUMO1–2, SAE2, and UBC9 were more highly expressed among the DM-containing cell lines, validating the association between SUMOylation and DM formation (). Out of those molecules, UBC9 and SAE2 had the most significant difference in expression levels for both cell lines. This observation was confirmed by Western blot of UBC9, in which its protein expression was significantly higher in DM cell lines (UACC-1598DM, COLO320DM, SK-PN-DW, and NCI-H716), though it was not the case for SAE2, which was actually downregulated in UACC-1598DM ( and Supplementary Figure). Our findings thus suggest that increased UBC9 expression may serve as the basis behind the link between SUMOylation and increased DMs within different types of tumor cells, which is in line with it being the only E2 conjugation enzyme. Therefore, UBC9, with respect to DM counts and tumor cell behavior, was chosen as the main focus of analysis for subsequent experiments.
Figure 1. Higher expression of SUMOylation-related molecules are associated with increased genomic instability and double minute (DM) counts.

UBC9 knockdown is associated with lowered DMs and DM-carried gene expression
To evaluate the association between UBC9 and DMs, a shRNA vector targeting UBC9 was constructed, to deplete its expression in UACC-1598DM and Colo320DM cells. After confirming the efficacy of the vector in knocking down UBC9 using Western blot in both cell lines (), DM counts were also found to have decreased post-UBC9 knockdown (), in which for UACC-1598DM, DMs decreased from 65.45 ± 43.38 to 28.53 ± 25.73 and 32.90 ± 27.87, while for Colo320DM, DMs decreased from 66.18 ± 38.18 to 35.11 ± 38.18 and 33.72 ± 25.34. To determine whether UBC9 knockdown, and subsequent lowering of DM counts, would affect expression levels for DM-carried genes, we examined copy numbers for those genes, where it was observed that for oncogenes EIF5A2, MYCN, and MCL-1 in UACC-1598DM and CDX2, MYC, and FAM84B in Colo320DM, which would otherwise be significantly higher (as shown in the scrambled shRNA control [sh-control]) for their respective cell lines, copy numbers were lowered upon UBC9 knockdown (). This lowered copy number also corresponded with lowered protein expression, at least for EIF5A2 and MYCN in UACC-1598DM, as well as for CDX2 and MYC Colo320DM, under Western blot after UBC9 knockdown ().
Figure 2. UBC9 knockdown is associated with lowered DMs and DM-carried gene expression.
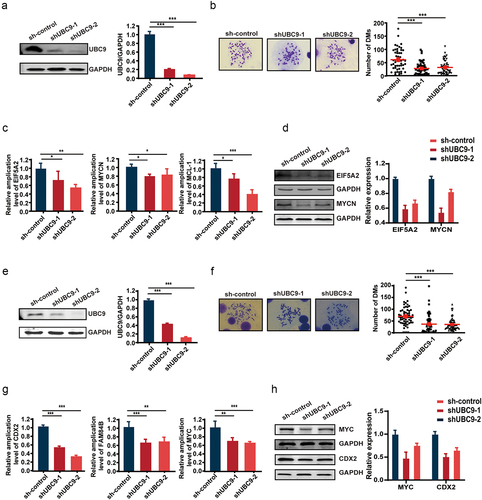
UBC9 inhibition by 2-D08 yields similar results as UBC9 knockdown due to reduced SUMOylation
To further confirm that reducing UBC9 activity resulted in lowered DM counts and DM-carried gene expression, owing to reduced SUMOylation, we applied the UBC9 inhibitor, 2-D08, which blocked SUMO transfer, and subsequently SUMOylation, in UACC-1598DM cells. As high 2-D08 concentrations were observed to significantly lower cell survival, a concentration of 30 µM was determined, based on testing a range of concentrations (Figure S1G), to be the most optimal for our studies. Indeed, SUMO1/2 expression was significantly lower among the UACC-1598DM cells exposed to 2-D08, compared to DMSO control (), and average DM count decreased from 62.59 ± 39.62 to 45.27 ± 30.57 between DMSO and 2-D08 groups (). It is also worth noting that UACC-1598DM cell growth rate and the ability of migration were significantly lowered after 2-D08 administration, compared to DMSO group (Figure S2A-C). This DM count reduction, in turn, corresponded to reduced copy number () and expression levels for DM-carried genes EIF5A2, MYCN, and MCL-1 in terms of mRNA (), and the former two genes for protein (). Similar results were found for 2-D08 treatment of Colo320DM, which was also associated with lowered growth rates (data not shown). In addition, DM counts decreased from 64.1 ± 50.38 in DMSO to 38.0 ± 39.50 in the 2-D08 group (), which corresponded to lowered copy number () and expression levels of DM-carried genes CDX2, FAM84B, and MYC for mRNA () and the former two genes for protein (). All these findings thus demonstrate that UBC9 inhibition results in reduced SUMOylation by blocking SUMO transfer, in turn leading to lowered DM counts and DM-carried gene expression.
Figure 3. UBC9 inhibition by the 2-D08 inhibitor yields similar results as UBC9 knockdown, due to reduced SUMOylation.
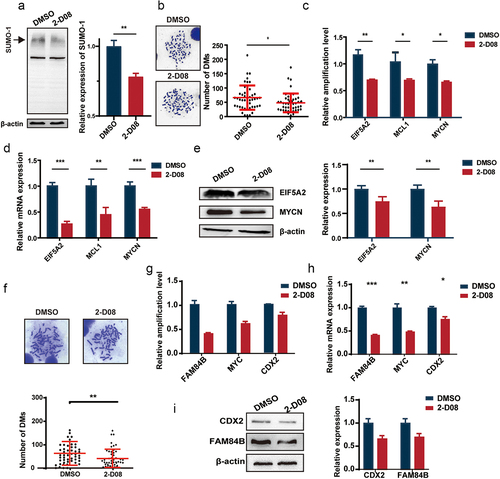
UBC9 knockdown reduces tumor malignancy and growth
High DM counts have been associated with malignant tumor phenotypes, owing to onco- and drug-resistance genes being carried on DMs. Therefore, to further validate the correlation between UBC9 expression and malignant tumor behavior, UACC-1598DM and Colo320DM cell proliferation rates were compared between UBC9 knockdown groups (shUBC9–1 and −2) versus the sh-control, in which UBC9 knockdown lowered proliferation in both cell lines (). Furthermore, UACC-1598DM cell colonies were significantly reduced after UBC9 knockdown (). UBC9 knockdown also reduced UACC-1598DM and Colo320DM cell migration, indicating that UBC9 plays a role in their invasive capabilities (). Bioinformatics analysis, utilizing the GEPIA database to analyze TCGA, was then performed to confirm whether UBC9 is associated with tumor progression. We found that UBC9 was highly expressed in multiple tumors, including cholangiocarcinoma (CHOL), diffuse large B cell lymphoma (DLBC), glioblastoma multiforme (GBM), pancreatic adenocarcinoma (PAAD), stomach adenocarcinoma (STAD), and thymoma (THYM), confirming that UBC9 was linked to tumor development (). As the cell lines used in our studies, UACC-1598DM/UACC-1598HSR and Colo320DM/Colo320HSR, are derived, respectively, from ovarian and colorectal cancers, we investigated the relationship between UBC9 levels and patient survival. Kaplan-Meier Plotter was used to analyze survival prognoses, based on ovarian cancer datasets GSE51373, GSE63885, and GSE30161, as well as colorectal cancer dataset GSE41258, where it was found that higher UBC9 expression was associated with worse prognoses (). All these findings thus suggest that heightened UBC9 expression was associated with tumor progression and poorer survival outcomes.
Figure 4. UBC9 knockdown reduces tumor malignancy and growth.
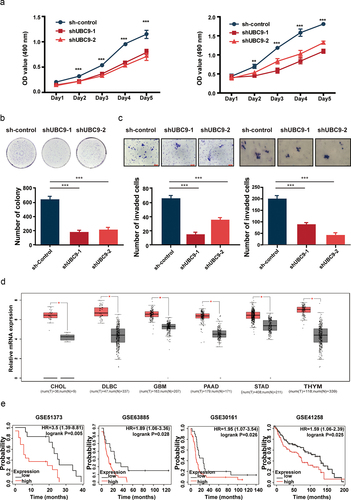
In vivo inhibition of UBC9 decreases the rate of tumor growth via DM elimination
To confirm whether our in vitro findings also hold true in vivo, we subcutaneously injected 6-week-old SCID female mice with UACC-1598DM cells. After stable transplanted tumors formed in these mice, with volumes up to 100 mm3, 2-D08 was injected intratumorally, at a dose of 10 mg/kg, every other day, from Days 17 to 27, as shown in . Control animals were injected with the same dose of DMSO. Tumor volumes and weights were evaluated on the days of injection, and at sacrifice in Day 27 (). The results showed that the growth in tumor volume was significantly lower among 2-D08-injected mice, compared to DMSO control (); these mice also had significantly lower tumor weights (). DM counts were determined by isolating primary cells from the transplanted tumor tissues, in which among the three mouse pairs, the 2-D08 group had lower DMs, respectively, 28.68 ± 26.34, 27.30 ± 20.49, and 25.5 ± 25.18, versus DMSO control, at 58.54 ± 30.31, 66.44 ± 21.91, and 57.18 ± 25.21 (). DNA, RNA, and protein were then extracted from tumor tissues, and it was found that the 2-D08 group had lower copy numbers (), as well as mRNA and protein expression levels for the DM-carried genes (), compared to DMSO control, for the three pairs. All these findings were further supported by immunofluorescence staining, showing lowered protein levels for EIF5A2 and MYCN (Figure S2E). In addition, UACC-1598DM with sh-control, shUBC9–1 and shUBC9–2 were subcutaneously injected in 6-week-old SCID female mice. The growth rate of control group was faster compared with shUBC9–1 and shUBC9–2 group (Figure S2D). These results thus confirm that the in vitro findings regarding UBC9 also are applicable in vivo.
Figure 5. UBC9 inhibition in vivo decreases the rate of tumor growth, via eliminating DMs.

Lowered UBC9 activity is associated with increased micronucleus (MN) expulsion, DNA damage, and decreased DDR
To further examine the mechanism behind the DM reduction in UBC9 knockdown, FISH was used to detect MN expulsion extent among UACC-1598DM cells. MNs were classified into three categories: Signal-, Signal+, and Signal++, based on the proportion of DM signal present in MN (). After UBC9 knockdown, increased expulsion of DM-containing MNs occurred. For each group, 130 cells were included and analyzed, with the percentage of such MNs increasing from 40.77% in sh-control group to 47.69% and 52.30%, respectively, in shUBC9–1 and −2 groups (). This result suggested that silencing UBC9 could lead to DM expulsion, via their encapsulation within MNs.
Figure 6. Lowered UBC9 activity is associated with increased micronucleus (MN) expulsion, DNA damage, and decreased DNA damage repair.
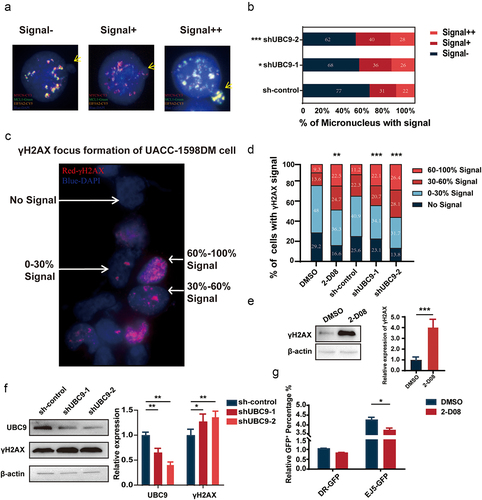
As DM formation has been related to DDR, we measured the extent of DNA damage present in UACC-1598DM cells, in the form of γ-H2AX foci content (). Based on the percentage area of γ-H2AX signal within the whole nucleus, shUBC9–1 and −2 groups had significantly increased proportion of cells with 60%–100% signal, at respectively 22.14% and 26.42%, compared to 11.20% in sh-control (). A similar percentage to those two groups was also observed in UACC-1598DM subject to 2-D08 inhibitor, where 22.47% of cells had 60%–100% γ-H2AX signal, compared to 9.29% in DMSO control. All of these findings corresponded to increased γ-H2AX protein levels among 2-D08 (), as well as shUBC9–1 and −2 groups (). All of these findings indicate that DNA damage increased with UBC9 knockdown or inhibition, which could serve as a contributing factor reduced DMs. To further examine whether this increase in DNA damage is associated with changes in DDR, a reporter plasmid system was used to detect changes in the DDR pathways of HR and NHEJ. After establishing the stable transfection cell lines of pHPRT-DRGFP, representing HR, and pimEJ5GFP, representing NHEJ, DMSO, and 2-D08 were used to treat these two groups for 1 week, followed by transient transfection pCBASceI and subsequent flow cytometry to detect changes in DDR pathway functioning, in the form of the degree of fluorescence present. We found that 2-D08 administration, compared to DMSO control, repressed NHEJ, as demonstrated by significantly lowered fluorescence levels. By contrast, HR remained unaffected (). Collectively, these results indicate that UBC9 inhibition reduced NHEJ activity, resulting in increased DNA damage and lowered DM counts through increasing MN expulsion.
Discussion
DMs consist of tiny spherical chromatin bodies, each of which is a few Mb in size. They are often found in pairs within a wide variety of tumor cells and play important roles in tumor development. In the present study, we found from bioinformatic analysis of cancer databases that SUMOylation is an important contributor to genomic instability and DM counts in cancer cells. One molecule involved in SUMOylation, the E2 conjugating enzyme UBC9, was more highly expressed among tumor cells with DMs, and its knockdown or inhibition with 2-D08 resulted in reduced DM counts, DM-carried gene expression, and tumor growth, both in vitro and in vivo. These outcomes were found to be linked to increased DM-containing MN expulsion, as well as lowered NHEJ activity, upon UBC9 knockdown or inhibition. Therefore, targeting the SUMOylation process could serve as an approach to halt cancer progression.
As a PTM, SUMOylation regulates hundreds of proteins closely associated with numerous biological processes, such as DDR, macromolecular assembly, chromatin organization, transcription, molecular transport, and intracellular signaling. Molecules involved in SUMOylation have been found in previous studies to be upregulated in tumor cells,Citation26 one of which is UBC9, the only E2 conjugating enzyme, which has been documented to play important roles in tumorigenesis and tumor development.Citation27 UBC9 has also been found to increase tumor drug resistance,Citation28 and a relationship between this molecule and DDR has been shown.Citation29,Citation30 Other studies have investigated UBC9 as a target for anti-tumor therapies.Citation31,Citation32 A UBC9 inhibitor, 2-D08, has been developed,Citation33 and has been shown to reduce tumor viability in acute myelogenous leukemia,Citation34 as well as being able to promote cancer cell sensitivity to DNA damage agents.Citation35 The results from our study are in line with previous findings indicating that the extent of SUMOylation is related to the degree of malignancy, which also corresponds to increased DM counts, formed during DDR. Additionally, 2-D08 was able to decrease tumor growth, via reducing DM counts and DM-carried gene expression, both in vitro and in vivo. However, future studies will be needed to determine if the changes in DM counts, stemming from UBC9 knockdown or inhibition, are actually related to changes in tumor properties.
SUMOylation has also been documented to be involved in regulating genomic stability and plays important roles in various DDR pathways,Citation36 such as HR, NHEJ, base excision repair (BER), Fanconi anemia pathway, and post-replication repair. In particular, SUMOylation modifies replication protein A1 in the HR pathway, which promotes RAD51 localization to DNA.Citation37,Citation38 Other HR molecules modified by SUMOylation include breast cancer gene 1 (BRCA1), receptor-associated protein 80, and Bloom syndrome protein, all of which are involved in maintaining genome stability.Citation39,Citation40 As for NHEJ, it has been reported to decrease after SAE1 inhibition, which in turn contributes to the SUMOylation level of XRCC4.Citation41 In BER, the SUMOylation of thymine DNA glycosylase is essential for it to be released after it hydrolyzes mismatched nucleotides.Citation42 Aside from its involvement in DDR pathways, SUMOylation also maintains genome stability via its activity on other molecular processes, such as DNA replication factors,Citation43 topoisomerase II,Citation44 and multiple essential telomere-protecting proteins.Citation45 In fact, high throughput mass-spectrometry‑based studies have revealed that >6,000 proteins could be SUMOylated.Citation46,Citation47 Additionally, it has been reported that SUMO-interacting motifs also take part in NHEJ and are associated with tumorigenesis, further underlying the importance of SUMO modification in DDR and tumor development.Citation48,Citation49 Our study also showed that SUMOylation is involved in DDR; at the very least, UBC9 was involved in NHEJ, as its knockdown reduced NHEJ activity in tumor cells.
With respect to DMs, they most likely arise as a result of aberrant excision and ligation processes, stemming from replication slippage, during DDR.Citation3 Indeed, previous studies have documented that DDR is involved in DM formation, such as one where DM counts decrease after BRCA1 inhibition.Citation16 Due to BRCA1 being a key HR molecule and target of SUMOylation, it is thus very likely that SUMOylation plays a significant role in DM formation. NHEJ also has been postulated to play a role in DM formation, as small palindromic sequences, which may be generated by this DDR process, have been documented to be characteristic of DMs.Citation15,Citation50 Our study agreed with these findings, as higher NHEJ activity was associated with higher DM counts and vice versa when UBC9 was knocked down or inhibited. However, only UBC9 was investigated in this study; other SUMOylation molecules may be involved in DM formation, as they have been documented to be involved in DDR processes. Therefore, future studies should examine the role these molecules play in DM formation, to further validate the connection between DM and SUMOylation.
Methods
Cell culture
The human ovarian cancer cell line, UACC-1598, with spontaneous DMs, was a gift from Dr. Xinyuan Guan. As previously described, UACC-1598DM and UACC-1598HSR subclones contain DMs and homogeneously staining regions (HSRs), respectively.Citation51 We also obtained human colon cancer cell lines, Colo320HSR and Colo320DM, with similar genetic characteristics, except for the former containing chromosomal HSR, and the latter extrachromosomal DMs, with respect to gene amplification forms. All four cell lines were cultured in RPMI-1640 (Gibco BRL), supplemented with 10% fetal bovine serum (FBS), at 37°C in 5% CO2, and maintained in mycoplasma-free conditions.
Karyotyping of metaphase chromosomes
Cells from all four cell lines, upon reaching the logarithmic growth phase, were seeded to obtain approximately 70% confluence, after which they were treated with 20 µg/L colchicine for 1 h, then harvested and incubated in hypotonic solution (.075 mol/L KCl) at 37°C for 15 min. After centrifugation and resuspension in PBS, cells were fixed in a mixture of methanol and glacial acetic acid (3:1 ratio) overnight at 4°C, then spread onto pre-chilled glass slides and dried. After drying, slides were stained with Giemsa, and 50 metaphase spreads were calculated for each aberration.
RT-qPCR
DNA was extracted with QIAamp DNA Extract Kit (Qiagen), and the copy number was detected with RT-qPCR. Total RNA was extracted using the Trizol reagent (Sigma), and reverse-transcribed using the Transcriptor First Strand cDNA Synthesis Kit (Roche). Real-time PCR (qPCR) was conducted with SYBR Green (Roche), using the LightCycler480 real-time PCR system (Roche), and target gene expression levels were normalized to GAPDH. Primers were designed using the Gene Runner software and are listed in Table S1.
Western blot
Western blot was performed using the standard protocol, as follows: Briefly, protein samples were loaded onto SDS-PAGE for protein separation, then transferred to PVDF membranes, and blocked by placing the membranes within a blocking buffer (PBS with 5% skim milk) on an orbital shaker, for 1 h at room temperature. Primary antibody was diluted in PBS-.1% Tween-20 (PBST), followed by incubation of the membranes overnight at 4°C. Afterward, membranes were washed three times with PBST, then incubated with secondary antibody at room temperature for 1 h. Signal detection was carried out by the Odyssey fluorescence imaging system. Primary and secondary antibodies used are provided in Table S2.
UBC9 knockdown using short hairpin RNA (shRNA)
For shRNA-mediated knockdown, shRNA targeting UBC9 were purchased from GenecopoeiaTM; its sequence can be found in Table S3. UACC-1598DM/Colo320DM cells in the logarithmic growth phase were inoculated into 6-well plates, cultured until reaching 80% confluence, and shRNA transfection performed using Lipofectamine 3000 (Invitrogen), following the manufacturer’s protocols. Transfected cells were then selected by puromycin, and successful UBC9 knockdown was detected by Western blot.
Report system for DDR pathway
A reporter plasmid system was established to evaluate the roles played for different DDR pathways, using three plasmids from Addgene: pCBASceI#26477, pHPRT-DRGFP#26476, pimEJ5GFP#44026. In brief, pHPRT-DRGFP#26476 and pimEJ5GFP#44026 were used to establish stable transfection cell lines for examining, respectively, HR and NHEJ activity, which were then treated with 30 µM of the inhibitor 2-D08. As for pCBASceI#26477, its expression was transient in nature, in order to induce double-strand breaks, and subsequently enhanced green fluorescent protein (GFP) synthesis upon activation of HR or NHEJ in the aforementioned cell lines. Flow cytometry was then utilized to detect the changes in the activities for different DDR pathways.
Murine xenograft model
All procedures for mouse care, handling, and sacrifice received the approval of the Medical Ethics Committee of Harbin Medical University and followed the Guide for the Care and Use of Laboratory Animals from the National Institutes of Health. The murine xenograft model was established by subcutaneously injecting UACC-1598DM cells into 6-week-old female SCID mice (Beijing Vital River Laboratory Animal Technology). After the resulting tumor reached a volume of ~100 mm3, mice received intra-tumoral injections of 2-D08 inhibitor, dissolved in DMSO, 5 times every other day, at a dose of 10 mg/kg. The same dosage, but with DMSO, was used as control. A Vernier caliper was used to measure the length and width of the subcutaneous xenograft, and tumor volumes were calculated with the following formula: volume = (length × width2)/2. Mice weights were also measured and recorded.
Cell proliferation, colony formation, and migration assays
The cell proliferation assay used was CellTiter 96®AQUEOUS One Solution Cell Proliferation Assay (Promega, WI). Briefly, cells were incubated into 96-well plates and incubated at 37°C for 24 h. Afterward, culture medium was discarded, and a mixture of 100 μL culture medium and 20 μL MTS solution was added to each well, followed by incubation at 37°C for 4 h. Each well was then measured with a microplate reader to obtain the absorbance value, representing the extent of cell proliferation.
For colony formation assays, cells in the logarithmic growth phase were used to form a single-cell suspension, then equal numbers of cells, with or without UBC9 knockdown, were counted and inoculated into 6-well plates at a low density (~1000 cells per well), followed by 2 weeks of culturing. Afterward, 2 mL of methanol was added into every well for fixation (15 min, room temperature). Staining was then conducted using Giemsa dye solution for 9 min, and pictures of the resulting cell colonies were taken with a digital camera for counting.
Cell migration was measured using the trans-well assay, in which cells were diluted to 3 × 104 cells/mL and plated into the upper chambers of a 24-well trans-well plate in serum-free medium, while medium containing 15% FBS was added to the lower chambers. After incubation for 72 h at 37°C, cells that did not migrate were removed by cotton swabs, while the remaining migrated cells were fixed in methanol for 10 min, stained with Giemsa solution for 7 min, then washed twice with PBS. After drying, films from the trans-well plate were cut off, fixed onto glass slides using neutral gum, and photographed for cell counting.
Fluorescent in situ hybridization (FISH)
FISH was carried out using probes, based on BAC clones of RP11-355H10, RP11-54A4, and RP11-654K19, all of which were purchased from BACPAC Resources Center (Children’s Hospital Oakland, Oakland, CA, USA). These probes were added to each action area and incubated in a wet box at 37°C for 48 h. Afterward, slides were fixed with 50% formamide, preheated at 44°C, for 15 min. The slides were then dehydrated and stained with 4′,6-diamidino-2-phenylindole (DAPI) prior to observation.
Immunofluorescence staining
Frozen tissue sections were prepared by embedding tumor tissues into optimal cutting temperature gel, followed by sectioning using a cryostat (Leica). The resulting 5 µm sections were fixed using 4% paraformaldehyde for 20 min, washed, and permeabilized by incubation within 2 mL of .1% Triton X-100 in PBS, at 4°C, for 10 min. Sections were then blocked with 4% bovine serum albumin solution in PBS, for 30 min at 37°C, followed by incubation overnight at 4°C with primary antibody (1:500), then with fluorescence-conjugated secondary antibody (1:1000) at 37°C for 1 h. Slides were then stained using DAPI prior to observation under a fluorescence microscope (Leica).
Bioinformatics analysis
The microarray data from The Cancer Genome Atlas (TCGA) database for breast cancer samples was first divided into two groups using R software: samples containing normal copy numbers, and those with DMs, defined as having copy numbers >12 and genetic material segment length >100 kb (ideally >500 kb). It is also worth noting that samples with copy numbers <4 were considered as part of the normal copy number group, in line with previous studies.Citation52,Citation53 Additionally, the extent of genomic instability for the tumor samples in TGCA, after downloading them from the cBioPortal database (https://www.cbioportal.org/) was evaluated using copy number analyses and detecting microsatellite instability, leading to these samples being divided into microsatellite instability (MSI), chromosome instability (CIN), and genome stability groups (GS)Citation25 under unsupervised clustering. UBC9 expression in different tumor types was also evaluated using TCGA data, and expression differences between tumor and non-cancerous samples were analyzed by the GEPIA database (http://gepia.cancer-pku.cn/). Cancer Cell Line Encyclopedia (CCLE; https://portals.broadinstitute.org/ccle_legacy/home) was then used to obtain high-throughput sequencing data for 1019 tumor cell lines, and karyotypes for each cell line was queried by the American Type Culture Collection (ATCC; https://www.atcc.org/), Japanese Collection of Research Bioresources (JCRB; https://cellbank.nibiohn.go.jp/english/), German Collection of Microorganisms and Cell Cultures (DSMZ; https://www.dsmz.de/), European Collection of Authenticated Cell Cultures (ECACC; https://www.phe-culturecollections.org.uk/collections/ecacc.aspx), and Novartis Institute for Biomedical Research (NIBRI; https://acronyms.thefreedictionary.com/NIBRI), to determine DM counts. The distinction between DMs+ and DMs++ refers to the proportion of cells containing double minutes (DMs). In the annotations accompanying the chromosomal details of cell lines, descriptions like “many”, “every”, or “more than 50%” of cells contain DMs will be classified as DMs++. Cell lines with description like “in few cells”, “occurred infrequently”, and “less than 50%” will be classified as DMs+. Cell lines without mentions of DMs in their chromosomal notes or those only referencing HSR were grouped under “no DMs.” Based on these counts, cells were divided into three groups: none (no DMs), partial (DMs+), and DM groups (DMs++). From the “no DMs”, 50 cell lines were randomly selected for the purposes of this study.
Survival and prognosis analysis data were obtained from the Gene Expression Omnibus (GEO) database, in the form of GSE51373, GSE63885, GSE30161, and GEO41258 datasets. Kaplan-Meier Plotter (http://kmplot.com/analysis/) and bioinfo (bioinfo.henu.edu.cn) online tools were used for data analyses.
Statistical analysis
All statistical analyses were conducted using GraphPad Prism 7 (GraphPad Inc., La Jolla, CA, USA), and data were expressed as mean ± standard deviation (SD). Comparisons between two groups were performed by student’s t-test, and three or more groups by one-way analysis of variance (ANOVA) with Tukey’s post-hoc test. p < .05 were considered statistically significant.
Conclusion
In summary, SUMOylation, in the form of the E2 conjugating enzyme UBC9, has been demonstrated to be involved in tumorigenesis and tumor development through increasing DM counts. UBC knockdown, or inhibition with the 2-D08 inhibitor, yields lowered DM counts and DM-carried gene expression, both in vitro and in vivo, which in turn leads to reduced tumor growth rates and malignancy. These results are owed to increased expulsion of DM-containing MNs, as well as lowered NHEJ activity resulting in greater levels of DNA damaged and reduced DDR responses. Our study thus identifies UBC9 inhibition as a possible novel therapeutic approach for cancer treatment.
Authors’ contributions
J.W., X.J., and W.S. contributed to the conception of the work and H.Z. and P.L. designed the work; software, B.Y., H.M., W.J., and L.X. contributed to the acquisition, analysis, and interpretation of data; N.S., X.Z., Y.W., and M.C. drafted the work and Y.K., Y.Q., and S.F. substantively revised it. All authors have read and approved the submitted version.
Supplemental Material
Download Zip (11.5 MB)Disclosure statement
No potential conflict of interest was reported by the author(s).
Data availability statement
The datasets supporting the conclusion of this article are included within the article.
Supplementary material
Supplemental data for this article can be accessed online at https://doi.org/10.1080/15384047.2024.2323768
Additional information
Funding
References
- Qiu H, Shao ZY, Wen X, Zhang LZ. New insights of extrachromosomal DNA in tumorigenesis and therapeutic resistance of cancer. Am J Cancer Res. 2020;10(12):4056–14.
- Jia X, Guan R, Cui X, Zhu J, Liu P, Zhang L, Wang D, Zhang Y, Dong K, Wu J, et al. Molecular structure and evolution mechanism of two populations of double minutes in human colorectal cancer cells. J Cell Mol Med. 2020; 24(24):14205–14216. doi:10.1111/jcmm.16035.
- Paulsen T, Kumar P, Koseoglu MM, Dutta A. Discoveries of extrachromosomal circles of DNA in normal and tumor cells. Trends Genet. 2018;34(4):270–278. doi:10.1016/j.tig.2017.12.010.
- Burrell RA, McClelland SE, Endesfelder D, Groth P, Weller MC, Shaikh N, Domingo E, Kanu N, Dewhurst SM, Gronroos E, et al. Replication stress links structural and numerical cancer chromosomal instability. Nature. 2013;2013(4947438):492–496. doi:10.1038/nature11935.
- Storlazzi CT, Lonoce A, Guastadisegni MC, Trombetta D, D’Addabbo P, Daniele G, L’Abbate A, Macchia G, Surace C, Kok K, et al. Gene amplification as double minutes or homogeneously staining regions in solid tumors: origin and structure. Genome Res. 2010; 20(9):1198–206. doi:10.1101/gr.106252.110.
- Wu S, Turner KM, Nguyen N, Raviram R, Erb M, Santini J, Luebeck J, Rajkumar U, Diao Y, Li B, et al. Circular ecDNA promotes accessible chromatin and high oncogene expression. Nature. 2019;575(7784):699–703. doi:10.1038/s41586-019-1763-5.
- Liao Z, Jiang W, Ye L, Li T, Yu X, Liu L. Classification of extrachromosomal circular DNA with a focus on the role of extrachromosomal DNA (ecDNA) in tumor heterogeneity and progression. Biochim Biophys Acta Rev Cancer. 1874;2020(1):188392. doi:10.1016/j.bbcan.2020.188392.
- Wu S, Bafna V, Mischel PS. Extrachromosomal DNA (ecDNA) in cancer pathogenesis. Curr Opin Genet Dev. 2021. 66:78–82. doi:10.1016/j.gde.2021.01.001.
- Kim H, Nguyen NP, Turner K, Wu S, Gujar AD, Luebeck J, Liu J, Deshpande V, Rajkumar U, Namburi S, et al. Extrachromosomal DNA is associated with oncogene amplification and poor outcome across multiple cancers. Nat Genet. 2020; 52(9):891–897. doi:10.1038/s41588-020-0678-2.
- Zhu Y, Gujar AD, Wong CH, Tjong H, Ngan CY, Gong L, Chen YA, Kim H, Liu J, Li M, et al. Oncogenic extrachromosomal DNA functions as mobile enhancers to globally amplify chromosomal transcription. Cancer Cell. 2021;39(5):694–707 e7. doi:10.1016/j.ccell.2021.03.006.
- Verhaak RGW, Bafna V, Mischel PS. Extrachromosomal oncogene amplification in tumour pathogenesis and evolution. Nat Rev Cancer. 2019;19(5):283–288. doi:10.1038/s41568-019-0128-6.
- Cox D, Yuncken C, Spriggs AI. Minute chromatin bodies in malignant tumours of childhood. Lancet. 1965;1(7402):55–58. doi:10.1016/S0140-6736(65)90131-5.
- Shoshani O, Brunner SF, Yaeger R, Ly P, Nechemia-Arbely Y, Kim DH, Fang R, Castillon GA, Yu M, Li JSZ, et al. Chromothripsis drives the evolution of gene amplification in cancer. Nature. 2021;591(7848):137–141. doi:10.1038/s41586-020-03064-z.
- Shimizu N, Misaka N, Utani K. Nonselective DNA damage induced by a replication inhibitor results in the selective elimination of extrachromosomal double minutes from human cancer cells. Genes Chromosomes Cancer. 2007;46(10):865–74. doi:10.1002/gcc.20473.
- Cai M, Zhang H, Hou L, Gao W, Song Y, Cui X, Li C, Guan R, Ma J, Wang X, et al. Inhibiting homologous recombination decreases extrachromosomal amplification but has no effect on intrachromosomal amplification in methotrexate-resistant colon cancer cells. Int J Cancer. 2019; 144(5):1037–1048. doi:10.1002/ijc.31781.
- Meng X, Qi X, Guo H, Cai M, Li C, Zhu J, Chen F, Guo H, Li J, Zhao Y, et al. Novel role for non-homologous end joining in the formation of double minutes in methotrexate-resistant colon cancer cells. J Med Genet. 2015; 52(2):135–44. doi:10.1136/jmedgenet-2014-102703.
- Yu L, Zhao Y, Quan C, Ji W, Zhu J, Huang Y, Guan R, Sun D, Jin Y, Meng X, et al. Gemcitabine eliminates double minute chromosomes from human ovarian cancer cells. PloS One. 2013; 8(8):e71988. doi:10.1371/journal.pone.0071988.
- Canute GW, Longo SL, Longo JA, Shetler MM, Coyle TE, Winfield JA, Hahn PJ. The hydroxyurea-induced loss of double-minute chromosomes containing amplified epidermal growth factor receptor genes reduces the tumorigenicity and growth of human glioblastoma multiforme. Neurosurgery. 1998;1998(33):609–616. doi:10.1097/00006123-199803000-00031.
- Flotho A, Melchior F. Sumoylation: a regulatory protein modification in health and disease. Annu Rev Biochem. 2013;82(1):357–85. doi:10.1146/annurev-biochem-061909-093311.
- Bergink S, Jentsch S. Principles of ubiquitin and SUMO modifications in DNA repair. Nature. 2009;458(7237):461–7. doi:10.1038/nature07963.
- Chang HM, Yeh ETH. SUMO: from bench to bedside. Physiol Rev. 2020;100(4):1599–1619. doi:10.1152/physrev.00025.2019.
- Zhu S, Sachdeva M, Wu F, Lu Z, Mo YY. Ubc9 promotes breast cell invasion and metastasis in a sumoylation-independent manner. Oncogene. 2010;2010(2912):1763–1772. doi:10.1038/onc.2009.459.
- Lopez I, Chalatsi E, Ellenbroek SIJ, Andrieux A, Roux PF, Cerapio JP, Jouvion G, van Rheenen J, Seeler JS, Dejean A. An unanticipated tumor-suppressive role of the SUMO pathway in the intestine unveiled by Ubc9 haploinsufficiency. Oncogene. 2020;39(43):6692–6703. doi:10.1038/s41388-020-01457-y.
- Sarangi P, Zhao X. SUMO-mediated regulation of DNA damage repair and responses. Trends Biochem Sci. 2015;40(4):233–42. doi:10.1016/j.tibs.2015.02.006.
- Cancer genome atlas research, N. Comprehensive molecular characterization of gastric adenocarcinoma. Nature. 2014;5137517:202–209. 10.1038/nature13480.
- Seeler JS, Dejean A. SUMO and the robustness of cancer. Nat Rev Cancer. 2017;17(3):184–197. doi:10.1038/nrc.2016.143.
- Mo YY, Yu Y, Theodosiou E, Ee PL, Beck WT. A role for Ubc9 in tumorigenesis. Oncogene. 2005;2005(2416):2677–2683. doi:10.1038/sj.onc.1208210.
- Fang S, Qiu J, Wu Z, Bai T, Guo W. Down-regulation of UBC9 increases the sensitivity of hepatocellular carcinoma to doxorubicin. Oncotarget. 2017;8(30):49783–49795. doi:10.18632/oncotarget.17939.
- Moschos SJ, Mo YY. Role of SUMO/Ubc9 in DNA damage repair and tumorigenesis. J Mol Histol. 2006;37(5–7):309–319. doi:10.1007/s10735-006-9030-0.
- Li Y, Jiang X, Zhang Y, Gao Z, Liu Y, Hu J, Hu X, Li L, Shi J, Gao N. Nuclear accumulation of UBC9 contributes to SUMOylation of lamin A/C and nucleophagy in response to DNA damage. J Exp Clin Cancer Res. 2019;38(1):67. doi:10.1186/s13046-019-1048-8.
- Mo YY, Moschos SJ. Targeting Ubc9 for cancer therapy. Expert Opin Ther Targets. 2005;9(6):1203–16. doi:10.1517/14728222.9.6.1203.
- Mo YY, Yu Y, Ee PL, Beck WT. Overexpression of a dominant-negative mutant Ubc9 is associated with increased sensitivity to anticancer drugs. Cancer Res. 2004;64(8):2793–8. doi:10.1158/0008-5472.CAN-03-2410.
- Kim YS, Keyser SG, Schneekloth JS Jr. Synthesis of 2‘,3’,4’-trihydroxyflavone (2-D08), an inhibitor of protein sumoylation. Bioorg Med Chem Lett. 2014;24(4):1094–1097. doi:10.1016/j.bmcl.2014.01.010.
- Zhou P, Chen X, Li M, Tan J, Zhang Y, Yuan W, Zhou J, Wang G. 2-D08 as a SUMOylation inhibitor induced ROS accumulation mediates apoptosis of acute myeloid leukemia cells possibly through the deSumoylation of NOX2. Biochem Biophys Res Commun. 2019;513(4):1063–1069. doi:10.1016/j.bbrc.2019.04.079.
- Zhou L, Zheng L, Hu K, Wang X, Zhang R, Zou Y, Zhong L, Wang S, Wu Y, Kang T. Sumoylation stabilizes hSSB1 and enhances the recruitment of NBS1 to DNA damage sites. Signal Transduct Target Ther. 2020;5(1):80. doi:10.1038/s41392-020-0172-4.
- Xie M, Yu J, Ge S, Huang J, Fan X. SUMOylation homeostasis in tumorigenesis. Cancer Lett. 2020. 469:301–309. doi:10.1016/j.canlet.2019.11.004.
- West SC. Molecular views of recombination proteins and their control. Nat Rev Mol Cell Biol. 2003;4(6):435–45. doi:10.1038/nrm1127.
- Zou Y, Liu Y, Wu X, Shell SM. Functions of human replication protein a (RPA): from DNA replication to DNA damage and stress responses. J Cell Physiol. 2006;208(2):267–73. doi:10.1002/jcp.20622.
- Bohm S, Bernstein KA. The role of post-translational modifications in fine-tuning BLM helicase function during DNA repair. DNA Repair (Amst). 2014. 22:123–32. doi:10.1016/j.dnarep.2014.07.007.
- Morris JR. SUMO in the mammalian response to DNA damage. Biochem Soc Trans. 2010;38(Pt 1):92–97. doi:10.1042/BST0380092.
- Zhang M, Jiang D, Xie X, He Y, Lv M, Jiang X. miR-129-3p inhibits NHEJ pathway by targeting SAE1 and represses gastric cancer progression. Int J Clin Exp Pathol. 2019;12(5):1539–1547.
- Baba D, Maita N, Jee JG, Uchimura Y, Saitoh H, Sugasawa K, Hanaoka F, Tochio H, Hiroaki H, Shirakawa M. Crystal structure of thymine DNA glycosylase conjugated to SUMO-1. Nature. 2005;2005(4357044):979–982. doi:10.1038/nature03634.
- Wei L, Zhao X. Roles of SUMO in replication initiation, progression, and termination. Adv Exp Med Biol. 1042;2017:371–393. doi:10.1007/978-981-10-6955-0_17.
- Bachant J, Alcasabas A, Blat Y, Kleckner N, Elledge SJ. The SUMO-1 isopeptidase Smt4 is linked to centromeric cohesion through SUMO-1 modification of DNA topoisomerase II. Mol Cell. 2002;9(6):1169–82. doi:10.1016/S1097-2765(02)00543-9.
- Potts PR, Yu H. The SMC5/6 complex maintains telomere length in ALT cancer cells through SUMOylation of telomere-binding proteins. Nature Structural & Molecular Biology. 2007;14(7):581–90. doi:10.1038/nsmb1259.
- Hendriks IA, Lyon D, Young C, Jensen LJ, Vertegaal AC, Nielsen ML. Site-specific mapping of the human SUMO proteome reveals co-modification with phosphorylation. Nature Structural & Molecular Biology. 2017;24(3):325–336. doi:10.1038/nsmb.3366.
- Hendriks IA, Vertegaal AC. A comprehensive compilation of SUMO proteomics. Nat Rev Mol Cell Biol. 2016;17(9):581–95. doi:10.1038/nrm.2016.81.
- Li YJ, Stark JM, Chen DJ, Ann DK, Chen Y. Role of SUMO: SIM-mediated protein–protein interaction in non-homologous end joining. Oncogene. 2010;2010(2924):3509–3518. doi:10.1038/onc.2010.108.
- Guzzo CM, Berndsen CE, Zhu J, Gupta V, Datta A, Greenberg RA, Wolberger C, Matunis MJ. RNF4-dependent hybrid SUMO-ubiquitin chains are signals for RAP80 and thereby mediate the recruitment of BRCA1 to sites of DNA damage. Sci Signal. 2012;5(253):ra88. doi:10.1126/scisignal.2003485.
- Zhu J, Yu Y, Meng X, Fan Y, Zhang Y, Zhou C, Yue Z, Jin Y, Zhang C, Yu L, et al. De novo-generated Small palindromes are characteristic of amplicon boundary junction of double minutes. Int J Cancer. 2013; 133(4):797–806. doi:10.1002/ijc.28084.
- Sun W, Quan C, Huang Y, Ji W, Yu L, Li X, Zhang Y, Zheng Z, Zou H, Li Q, et al. Constitutive ERK1/2 activation contributes to production of double minute chromosomes in tumour cells. J Pathol. 2015; 235(1):14–24. doi:10.1002/path.4439.
- Sanborn JZ, Salama SR, Grifford M, Brennan CW, Mikkelsen T, Jhanwar S, Katzman S, Chin L, Haussler D. Double minute chromosomes in glioblastoma multiforme are revealed by precise reconstruction of oncogenic amplicons. Cancer Res. 2013;73(19):6036–45. doi:10.1158/0008-5472.CAN-13-0186.
- Nikolaev S, Santoni F, Garieri M, Makrythanasis P, Falconnet E, Guipponi M, Vannier A, Radovanovic I, Bena F, Forestier F, et al. Extrachromosomal driver mutations in glioblastoma and low-grade glioma. Nat Commun. 2014; 5(1):5690. doi:10.1038/ncomms6690.