Abstract
Objective: Recent field data analyses have shown that the safety advantages of rear seats relative to the front seats have decreased in newer vehicles. Separately, the risks of certain injuries have been found to be higher for obese occupants. The objective of this study is to investigate the effects of advanced belt features on the protection of rear-seat occupants with a range of body mass index (BMI) in frontal crashes.
Methods: Whole-body finite element human models with 4 BMI levels (25, 30, 35, and 40 kg/m2) developed previously were used in this study. A total of 52 frontal crash simulations were conducted, including 4 simulations with a standard rear-seat, 3-point belt and 48 simulations with advanced belt features. The parameters varied in the simulations included BMI, load limit, anchor pretensioner, and lap belt routing relative to the pelvis. The injury measurements analyzed in this study included head and hip excursions, normalized chest deflection, and torso angle (defined as the angle between the hip–shoulder line and the vertical direction). Analyses of covariance were used to test the significance (P <.05) of the results.
Results: Higher BMI was associated with greater head and hip excursions and larger normalized chest deflection. Higher belt routing increased the hip excursion and torso angle, which indicates a higher submarining risk, whereas the anchor pretensioner reduced hip excursion and torso angle. Lower load limits decreased the normalized chest deflection but increased the head excursion. Normalized chest deflection had a positive correlation with maximum torso angle. Occupants with higher BMI have to use higher load limits to reach head excursions similar to those in lower BMI occupants.
Discussion and Conclusion: The simulation results suggest that optimizing load limiter and adding pretensioner(s) can reduce injury risks associated with obesity, but conflicting effects on head and chest injuries were observed. This study demonstrated the feasibility and importance of using human models to investigate protection for occupants with various BMI levels. A seat belt system capable of adapting to occupant size and body shape will improve protection for obese occupants in rear seats.
Introduction
Generally, rear-seat occupants were considered to be safer than front-seat occupants in frontal crashes, because they are further away from the impact. However, recent field data analyses have shown that front seats may provide better protection, especially for elderly occupants, than rear seats in newer vehicle models (Bilston et al. Citation2010; Kuppa et al. Citation2005; Smith and Cummings Citation2004). Several studies (Kent et al. Citation2007; Sahraei et al. Citation2010) have found that the relative effectiveness of rear seats for belted adult occupants in newer vehicle models is lower than that in older vehicle models. Brown and Bilston (Citation2014) also found that the rear-seat occupants had higher fatal injury risks than front-seat occupants using linked hospital admission and police-reported data in Australia.
Field data have also shown that in frontal crashes the most frequently injured body region for belted rear-seat adult occupants is the chest, and the major source of these chest injuries is the seat belt (Esfahani and Digges Citation2009; Kuppa et al. Citation2005). Parenteau and Viano (Citation2003) found that in frontal crashes chest injuries accounted for 76% of the total Abbreviated Injury Scale (AIS) 3+ injuries for belted rear-seat occupants older than 12 years old, whereas head and lower extremity injuries only accounted for 9 and 8%, respectively. Most of these head and lower extremity injuries were caused by the contacts to the back of the front seat.
Though chest injury is the major concern for rear-seat adult occupants, obese occupants may sustain increased injury risks on different body regions, including the chest, than occupants with normal body mass index (BMI) levels. For example, by analyzing field data, Cormier (Citation2008) reported that obese occupants had 26 and 33% higher risk of AIS 2+ and AIS 3+ chest injuries, respectively, than lean occupants. By conducting cadaver tests, Forman et al. (Citation2009a, Citation2009b) found that obese occupants experienced greater head, torso, and knee excursions; higher chest deflections; and higher submarining tendencies than lower BMI occupants. Computational studies by Turkovich (Citation2011), Turkovich et al. (Citation2013), and Shi et al. (Citation2015) also showed that an increase in BMI may significantly increase the chest and lower extremity injury risks for front-seat occupants in frontal crashes. It was found that these increased injury risks are associated with greater mass- and body shape–induced poor belt fit for obese occupants.
Although the injury concerns and injury mechanism of obese occupants are fairly clear, research on enhancing the protection of obese occupants is rare. Given the fact that advanced restraint features are rarely available for rear-seat occupants, advanced restraint designs for rear-seat occupants, including obese occupants, are needed. The literature reveals that researchers have performed crash tests and computational simulations to evaluate the feasibility of introducing seat belt features, such as load limiters and pretensioners, into the rear-seat restraint system. For example, Kent et al. (Citation2007) conducted MADYMO simulations using seat belts with advanced features for rear-seat occupants. It was found that although there is a tradeoff between occupant chest deflection and head excursion, belt load limiters and pretensioners can maintain or reduce head excursion while significantly reducing chest deflection. Forman et al. (Citation2008) performed 48 frontal sled tests with 4 different sizes of anthropomorphic test devices (ATDs), including Hybrid III (HIII) 6-year-old, HIII 5th percentile female, HIII 50th percentile male, and THOR-NT, at 2 impact speeds. They found that a seat belt with a dual-stage progressive load limiter and a pretensioner decreased chest deflection by 29–38% for different ATDs with little or no increase in head excursion.
Though seat belt systems with advanced features have shown significant benefit for reducing injury risks to rear-seat occupant, especially to the chest, no efforts have been made to study the specific designs that can enhance the protection for obese rear-seat occupants. Therefore, the objective of this study is to investigate the effects of advanced belt features on the protection of rear-seat occupants with different body shapes using computational simulations with finite element (FE) human models.
Methods
Rear Seat Model Development and Validation
In this study, an FE model was built to represent the rear-seat compartment based on a compact vehicle. In our validation sled test (to be described later), the floor pan of the vehicle under the rear seat was removed and replaced with a simple sheet metal box section, reinforced with foam board inside. Therefore, in the current study, the same structure was used to simulate the rear-seat cushion panel, which could deform due to the contact force from the occupant buttock in a high-speed collision. The rear-seat model was integrated with a validated TRW 3-point seat belt model, which includes a load limiter, retractor pretensioner, and anchor pretensioner. The webbing storage on the retractor spool can also be changed based on the size of the occupant, which will affect the actual shoulder belt force. Each of the pretensioner models included a small airbag model to simulate the pyrotechnic mechanism with a given combustion energy, so that they can behave the same way as those in real productions. The firing time of the pretensioners can be adjusted as needed.
To validate the rear-seat and seat belt models, a 56 km/h sled test using a 50th percentile HIII ATD was conducted under a crash pulse shown in . In this test, a standard rear-seat, 3-point belt was used; therefore, the retractor pretensioner, anchor pretensioner, and load limiter were all disabled in the simulation. In this simulation, the LSTC 50th HIII ATD model was used, and LS-DYNA 971-R610 was used for all simulations. A comparison of the ATD kinematics between the test and the simulation is shown in . The ATD started to move forward at about 15 ms, and the maximum head excursion occurred at about 100 ms in the test and the simulation. The model-predicted ATD accelerations, axial upper neck force, and chest deflection were also compared to the test data in . Good correlations between the simulation and test results were achieved with an average CORrelation and Analysis (CORA) correlation score of 86%.
Obese Human Body Model Development and Validation
In our previous work (Shi et al. Citation2015), different FE human body models representing occupants with different BMIs (20, 25, 30, 35, and 40 kg/m2) and a stature of 175 cm were generated using mesh morphing techniques based on the THUMS 4 mid-size male model. The human models with different BMIs were validated against several sets of cadaver tests, including abdomen high-speed loading test (Foster et al. Citation2006) and whole-body cadaver rear-seat sled tests (Forman et al. Citation2009a). Foster et al. (Citation2006) performed a series of cadaver tests to quantify abdomen response to lap belt loading with a seat belt wrapped around the anterior surface of the abdomen. Compared to the test with an obese cadaver at BMI of 32 kg/m2, our model predicted the lap belt force and abdomen penetration reasonably well. In the study of Kent et al. (Citation2010), several tests were performed using 3-point belt-restrained obese cadavers (mean BMI value of 40.1 kg/m2) and 5 non-obese cadavers (mean BMI value of 20.3 kg/m2). In our previous work (Shi et al. Citation2015), simulations using the human body models with BMI 20 and 40 kg/m2 predicted very similar trends in body excursions as those in the cadaver tests reported by Kent et al. (Citation2010).
Parametric Study
Simulations With a Standard Rear-Seat, 3-Point Belt
Using the validated rear-seat model and the human body models, 4 simulations were performed with a standard 3-point belt without pretensioner and load limiter and with occupants at 4 levels of BMI (25, 30, 35, and 40 kg/m2). Though the front seat is available in the model, to reduce the complexity of the simulations, the occupant-to–front seat contact was not assigned. Consequently, the head and knee excursions were considered as the measurements of the head and lower extremity injuries, respectively. The front seat was set as a reference to check whether occupant head-to–front seat contact occurred. To position the human models, the same torso angle was assigned for different BMIs as that from the dummy model in the validation simulation. The human body models were positioned such that the same compressions/penetrations to the seat back were achieved across BMI levels, and the human model with BMI 25 was positioned 8 mm higher than those at BMI 30 and higher. The human model at BMI 30 nearly fully compressed the seat cushion; therefore, higher BMI occupants were positioned with the same seat cushion compression as that for BMI 30. shows the locations of the H-point for all occupant models with different BMIs. As the BMI increased, the H-point was slightly more forward and upward due to the larger thigh and torso in occupants with higher BMIs.
Table 1 Sitting position for occupants with different BMI levels
Reed et al. (Citation2012) reported that every 10 kg/m2 increase in BMI was associated with a 130-mm increase in lap belt webbing length and a 60-mm increase in shoulder belt webbing length. Based on a study by Forman et al. (Citation2009a), for normal-weight occupants (BMI = 20–25 kg/m2), about 750–850 mm of webbing remained on the retractor spool. Therefore, in the current study, the webbing that remained on the spool was set as 780, 710, 640, and 570 mm for occupants with BMIs of 25, 30, 35, and 40 kg/m2, respectively. In all 4 baseline simulations, the lap belt was positioned at low position across the conjunction between the thigh and the torso. The horizontal distances between the head vertex and the front seat were 578, 575, 581, and 573 mm for BMIs of 25, 30, 35, and 40 kg/m2, respectively.
Previous field data analysis (Kuppa et al. Citation2005) showed that the most commonly injured body region for adult rear-seat occupants is the chest. Therefore, in this study, chest deflection was considered as the major injury measure. Because occupants with different BMIs have different chest depths, normalized chest deflection was used in this study as the chest injury measurement. The normalized chest deflection is defined in EquationEq. (1)(1) , in which DInitial is the initial chest depth between the point on the sternum and the corresponding point on the spine. DDef is the chest depth during the crash simulation. Normalized chest deflection was measured at 6 locations () corresponding to the T3, T5, T7, T8, T9, and T12. Maximum normalized chest deflection among the 6 measured locations was used to evaluate the chest injury risk.
(1)
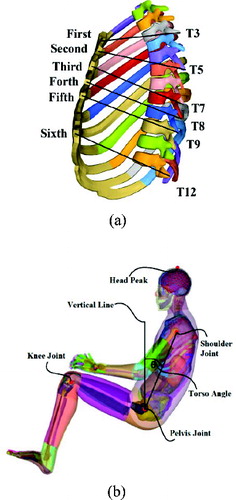
Body excursions and torso angle were also output from the simulations. shows landmark locations on the human body model, from which excursion data were measured. The head vertex and the center of knee joint were used to measure the head excursion and knee excursion. The torso angle was defined as the angle between the hip–shoulder line and the vertical direction. Based on the torso angle definition, a greater torso angle indicates a higher submarining risk.
Simulations With Advanced Restraint Systems
A parametric study was performed using the human body models with different BMIs to investigate the effect of advanced belt features, including the load limiter, retractor pretensioner, and anchor pretensioner, on the protection of rear-seat occupants.
Input variables in the parametric study included BMI (25, 30, 35, and 40 kg/m2), load limiter levels (4.0, 5.6, and 6.8 kN), lap belt positions (low, high), and anchor pretensioner (yes, no). Occupants with BMIs ranging from 25 to 40 kg/m2 covered almost 94% of the American overweight occupants in 2009–2010 (Flegal et al. Citation2010, 2012). Though a BMI of 25 kg/m2 is not considered obese, it was included in this study as a reference for obese occupants (BMI ≥ 30) for comparison. The load limit levels were selected to be high enough to prevent excessive head excursions based on the research by Foret-Bruno et al. (Citation2001). A belt-fit study by Reed et al. (Citation2012) provided the ranges of the lap belt routing positions for occupants with different BMI levels. Two positions, a low position and a high position, were chosen in this study for each BMI level. shows the selected lapsbelt positions for occupants with different BMIs. The value of the X and Z directions were the horizontal and vertical distances between the lap belt and the anterior superior iliac spine (ASIS) of the pelvis as shown in . shows the selected lap belt positions relative to the volunteer data. The low position corresponds to the average lap belt position with a given BMI, and the high position is associated with higher and further forward lap belt position relative to the ASIS. In simulations with an anchor pretensioner, the pretensioner was fired at 10 ms. In all simulations, the retractor pretensioner was always fired.
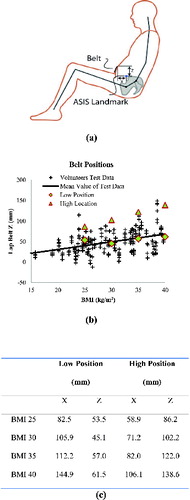
A full-factorial design was used to set up the simulations, which resulted in 48 (4 × 3 × 2 × 2) simulations (shown in Table A1, see online supplement). Analysis of covariance was performed using SPSS 22.0 (SPSS Inc., Chicago, IL) with alpha =.05 to test the significance of the factors for each injury measurement. In the statistical model, the input variables were BMI, load limiter level, lap belt position, and anchor pretensioner. The dependent variables were head excursion, shoulder excursion, pelvis excursion, knee excursion, maximum normalized chest deflection, and torso angle.
Results
Simulations With Baseline 3-Point Belt Without Pretensioner and Load Limiter
The head and knee excursions, normalized chest deflection, and torso angle for occupants with different BMIs are shown in Figures A1 and A2, in which a standard 3-point belt without pretensioner and load limiter were used. The head and knee excursions increased as the BMI increased. The maximum head excursion for a BMI of 25 was 567 mm and it was 666 mm for a BMI of 40, resulting in a 99 mm difference. If a front seat were present in the simulation, the head of an occupant with a BMI of 25 would not contact the front seat, whereas occupants with a BMI higher than 30 would sustain head-to–front seat contact. Similarly, the knee excursion increased from 208 to 320 mm with an increase in BMI from 25 to 40. This increase (112 mm) is greater than that for the head excursion. The increase in BMI from 25 to 40 also increased the maximum normalized chest deflection from 0.295 to 0.335. The obesity effect on the torso angles is not significant. There was only a slight decrease from 5° at a BMI of 25 to 1° at a BMI of 40.
Simulation Results With Advanced Restraint Systems
Parametric simulation results with advanced restraint systems are shown in . Detailed simulation results are shown in Table A2 (see online supplement). Overall, the factor effects on the head and shoulder excursions are almost identical, and the factor effects on the knee and pelvis excursions are very similar as well. BMI is a dominating factor affecting all of the injury measures significantly. In particular, an increase in BMI increased all of the excursions (P =.000) as well as the normalized chest deflection (P =.000). As BMI increased, the torso angle decreased (P =.000). Because the load limiter is on the shoulder belt retractor, it only affected head and shoulder excursions significantly (P =.000) but was not significant for the knee (P =.896) and pelvis excursions (P =.975). With greater load limit levels, head and shoulder excursions decreased significantly, whereas normalized chest deflection (P =.000) and torso angle (P =.000) increased. Lap belt location significantly affected all of the injury measures except normalized chest deflection (P =.413). In particular, higher lap belt position induced higher knee (P =.000) and pelvis (P =.000) excursions, lower head (P =.001) and shoulder (P =.024) excursions, and higher torso angle (P =.000), all of which indicate a higher submarining risk. Because the anchor pretensioner is on the lap belt only, it did not affect the head (P =.063) and shoulder excursion (P =.101) significantly or the normalized chest deflection (P =.740). However, adding an anchor pretensioner can significantly reduce the knee (P =.000) and pelvis (P =.000) excursions as well as torso angle (P =.000), all of which indicate a reduced submarining risk.
Discussion
Body Excursions, Normalized Chest Deflection, and Torso Angle
As shown in , there was a clear conflict between the normalized chest deflection and the head excursion associated with load limiter levels. Increasing the load limit reduced the head excursion but increased the normalized chest deflection and vice versa. The correlation between the head excursion and the normalized chest deflection in this study was shown in . In each BMI, there was a strong negative linear relationship between the head excursion and the normalized chest deflection. At the same head excursion level, occupants with higher BMI would experience higher normalized chest deflection. In this study, even though the head-to–front seat contact was not simulated, 600 mm distance between the front seat and the occupant was measured with the front seat in the mid-track location. To prevent head-to–front seat contact, a higher load limit is necessary, but it will likely increase the chest injury risk. Therefore, for an occupant with a specific BMI, we should choose the load limit as low as possible but still high enough to prevent head-to–front seat contact. However, it should be noted that for occupants with BMI of 35 or 40, the highest load limit (6.8 kN) in this study cannot prevent a head-to–front seat contact based on the simulation results.
shows that the chest deflection patterns are different between the human model and the dummy model. In particular, the chest deflection is uniformly distributed in the dummy model, and the largest chest deflection occurred at the upper sternum area. The model-predicted human rib deflection pattern was consistent with the postmortem human subject test results reported by Michaelson et al. (Citation2008), in which the normalized chest deflection at the fourth rib was generally much greater than that at the eighth rib for rear-seat occupants.
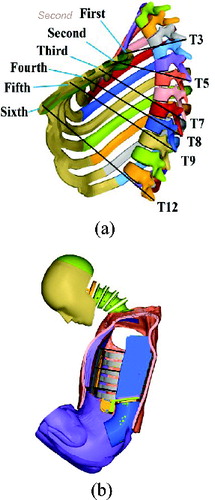
As shown in , the torso angle was significantly affected by all of the input variables. Reducing the load limit, lowering the lap belt, and adding an anchor pretensioner resulted in smaller torso angle across different BMI levels, indicating a lower submarining risk. However, the BMI effect on the torso angle is difficult to explain. Intuitively, one would think that higher BMI will be associated with a higher torso angle and, in turn, a higher submarining risk. However, this is not the case in the current study. Our results in and showed that occupants with higher BMIs sustained slightly lower torso angles. This is mainly due to the fact that the same load limit level was used among occupants with different BMIs, and occupants with higher BMIs tend to have higher head and shoulder excursions with a constant level of load limit. and show the correlation between the body excursions and the torso angle. It is clear that higher pelvis excursions and lower head excursions were generally associated with larger torso angles across different BMIs. The correlation between the torso angle and the normalized chest deflection is shown in . A larger torso angle is associated with higher normalized chest deflection, indicating that submarining type of kinematics may increase the risk of chest injury. Furthermore, at the same torso angle level, occupants with higher BMIs tend to experience higher normalized chest deflection.
Lap Belt Position and Anchor Pretensioner
Higher lap belt routing has significant effects on occupant kinematics. Specifically, during a crash, a lap belt with a higher routing location can easily move above the ASIS of the pelvis and load into the abdomen, which is often referred to as submarining. Submarining will result not only in a higher risk of abdominal injury but also in greater knee and pelvis excursions and higher normalized chest deflection. On the other hand, submarining will likely reduce the head and shoulder excursions due to a larger torso angle.
Our simulation results showed that anchor pretensioners are very effective for reducing the risk of submarining regardless of lap belt locations. Figure A3 shows the torso angles for an occupant with a BMI of 40 with a 6.8 kN load limiter at 4 different belt configurations: (1) low belt-routing position without anchor pretensioner, (2) high belt-routing position without anchor pretensioner, (3) low belt-routing position with anchor pretensioner, and (4) high belt-routing position with anchor pretensioner. The occupant with a high belt-routing position and no anchor pretensioner had a minimum torso angle of 19.4°, and the occupant with a low belt-routing position and anchor pretensioner had a minimum torso angle of −14°. On average, an anchor pretensioner can reduce the torso angle by 13° for an occupant with a BMI of 40.
Optimal Seat Belt Designs for Occupants With Different BMIs
Based on the results from the parametric study, advanced seat belt features, including the anchor pretensioner and load limiter, have significant impacts on occupant injury measures. However, unlike the anchor pretensioner, whose effects are consistent across occupants with different BMIs, a load limiter can cause conflicting effects on occupant head excursion and rib deflection ratio. In order to protect occupants with higher BMIs, a higher load limit level is necessary to prevent contact between the head and front seat, but this load limit increase will likely increase the chest injury risk. Table A3 (see online supplement) shows a comparison of impact responses between the baseline belt without pretensioner and load limiter and the optimal belts for occupants with different BMIs. As shown in Table A3, all of the selected designs with a pretensioner and load limiter reduced the normalized chest deflections while keeping the head excursions at a level similar to the designs without a pretensioner and load limiter. However, different load limits are needed for different BMIs, indicating that a switchable load limiter (whose load limit can adapt to the weight of the occupant) is needed to provide optimal protection to occupants with different BMIs. For occupants with a BMI of 35 or 40, even the highest load limit (6.8 kN) in the current study cannot prevent a head-to–front seat contact. In these cases, extra padding on the back of the front seat or even an airbag could potentially reduce head injury risks.
Limitations and Future Work
This study is not without limitations. First, the morphed obese human body models were validated only against limited cadaver tests. Further validations are necessary to improve their quality. Second, the human body models are with a single stature and with the same material properties; the rear-seat model is based on a compact vehicle; and a single crash pulse was used. These constraints on occupants and crash conditions will likely limit the scope of the design space. Future studies on a range of rear-seat compartments and different crash pulses are necessary. Third, in this study, contact between the occupant and the front seat was not defined to reduce the complexity of the simulations, which is consistent with previous cadaver tests by Forman et al. (Citation2009a). However, this may affect the occupant kinematics depending on the location and contact characteristics of the front seat. With a front seat close to the knees of the rear-seat occupants, the contact loads to the knees could potentially reduce the lower extremity excursions and in turn affect the submarining status and even the loads to the chest. However, such kinematic change can be covaried with the size of the occupant, the space of the rear-seat compartment, the front-seat location, and the contact characteristics of the front seat back. Future simulation studies with occupant-to–front seat contact are needed to gain a better understanding of those effects.
Conclusions
The effects of advanced belt features on the protection of rear-seat occupants with a range of BMIs were investigated in frontal crashes. Using human body FE models with 4 BMIs generated in our previous work and a newly developed and validated rear-seat FE model, parametric studies were performed to investigate the effect of load limiter, anchor pretensioner, lap belt routing location, and BMI on occupant responses. The simulation results confirmed the findings from previous cadaver tests that occupants with higher BMIs sustain significantly higher body excursions and normalized chest deflections with a standard rear-seat 3-point belt than those for lower BMI occupants. Parametric simulations suggest that optimizing the load limiter and adding pretensioner(s) can reduce injury risks associated with obesity, but conflicting effects on head and chest injuries are observed. Because in general the restraint design process highly relies on the performance of ATDs without considering the obesity effects, our simulations demonstrated the feasibility of using human FE models to investigate the protection for occupants with various BMI levels. A seat belt system capable of adapting to occupant size and body shape will improve protection for obese occupants in rear seats.
Funding
This study was supported by the National Science Foundation (Award No. 1300815) and a grant from TRW.
Supplemental Materials
Supplemental data for this article can be accessed on the publisher's website
Appendix
Download PDF (326.8 KB)References
- Bilston LE, Du W, Brown J. A matched-cohort analysis of belted front and rear seat occupants in newer and older model vehicles shows that gains in front occupant safety have outpaced gains for rear seat occupants. Accid Anal & Prev. 2010;42:1974–1977.
- Brown J, Bilston LE. The scope and nature of injuries to rear seat passengers in NSW using linked hospital admission and police data. Traffic Inj Prev. 2014;15:462–469.
- Cormier JM. The influence of body mass index on thoracic injuries in frontal impacts. Accid Anal Prev. 2008;40:610–615.
- Esfahani ES, Digges K. Trend of Rear Occupant Protection in Frontal Crashes Over Model Years of Vehicles. Warrendale, PA: SAE; 2009. SAE No. 2009-01-0377.
- Flegal KM, Carroll MD, Kit BK, Ogden CL. Prevalence of obesity and trends in the distribution of body mass index among US adults, 1999–2010. JAMA. 2012;307:491–497.
- Flegal KM, Carroll MD, Ogden CL, Curtin LR. Prevalence and trends in obesity among US adults, 1999–2008. JAMA. 2010;303:235–241.
- Foret-Bruno J-Y, Trosseille X, Page Y, et al. Comparison of Thoracic Injury Risk in Frontal Car Crashes for Occupant Restrained Without Belt Load Limiters and Those Restrained With 6 kN and 4 kN Belt Load Limiters. Warrendale, PA: SAE; 2001. SAE No. 2001-22-0009.
- Forman J, Lopez-Valdes FJ, Lessley D, Kindig M, Kent R, Bostrom O. The effect of obesity on the restraint of automobile occupants. Ann Adv Automot Med. 2009a;53:25–40.
- Forman J, Lopez-Valdes F, Lessley D, et al. Rear Seat Occupant Safety: An Investigation of a Progressive Force-Limiting, Pretensioning 3-Point Belt System Using Adult PMHS in Frontal Sled Tests. Warrendale, PA: SAE; 2009b. SAE No. 2009-22-0002.
- Forman J, Michaelson J, Kent R, Kuppa S, Bostrom O. Occupant restraint in the rear seat: ATD responses to standard and pre-tensioning, force-limiting belt restraints. Ann Adv Automot Med. 2008;2:141–154.
- Foster CD, Hardy WN, Yang KH, King AI, Hashimoto S. High-Speed Seatbelt Pretensioner Loading of the Abdomen. Warrendale, PA: SAE; 2006. SAE No. 2006-22-0002.
- Kent R, Forman JL, Bostrom O. Is there really a “cushion effect”?: a biomechanical investigation of crash injury mechanisms in the obese. Obesity. 2010;18:749–753.
- Kent R, Forman J, Parent D, Kuppa S. Rear seat occupant protection in frontal crashes and its feasibility. Paper presented at: 20th International Conference on the Enhanced Safety of Vehicles; 2007.
- Kuppa S, Saunders J, Fessahaie O. Rear seat occupant protection in frontal crashes. Paper presented at: 19th International Technical Conference on the Enhanced Safety of Vehicles (ESV); 2005; Washington.
- Michaelson J, Forman J, Kent R, Kuppa S. Rear Seat Occupant Safety: Kinematics and Injury of PMHS Restrained by a Standard 3-Point Belt in Frontal Crashes. Warrendale, PA: SAE; 2008. SAE No. 2008-22-0012.
- Parenteau C, Viano DC. Field Data Analysis of Rear Occupant Injuries Part I: Adults and Teenagers. Warrendale, PA: SAE; 2003. SAE No. 2003-01-0153.
- Reed MP, Ebert-Hamilton SM, Rupp JD. Effects of obesity on seat belt fit. Traffic Inj Prev. 2012;13:364–372.
- Sahraei E, Digges K, Marzougui D. Reduced protection for belted occupants in rear seats relative to front seats of new model year vehicles. Ann Adv Automot Med. 2010;54:149–158.
- Shi X, Cao L, Reed MP, Rupp JD, Hu J. Effects of obesity on occupant responses in frontal crashes: a simulation analysis using human body models. Comput Methods Biomech Biomed Eng. 2015;18:1280–1292.
- Smith KM, Cummings P. Passenger seating position and the risk of passenger death or injury in traffic crashes. Accid Anal Prev. 2004;36:257–260.
- Turkovich M. The Effects of Obesity on Occupant Injury Risk in Frontal Impact: A Computer Modeling Approach [PhD Dissertation]. Pittsburgh, PA: University of Pittsburgh; 2011.
- Turkovich M, Hu J, van Roosmalen L, Brienza D. Computer simulations of obesity effects on occupant injury in frontal impacts. Int J Crashworthiness. 2013;18:502–515.