ABSTRACT
Smokers with minor spirometric abnormalities can experience persistent activity-related dyspnea and exercise intolerance. Additional resting tests can expose heterogeneous physiological abnormalities, but their relevance and association with clinical outcomes remain uncertain. Subjects included sixty-two smokers (≥20 pack-years), with cough and/or dyspnea and minor airway obstruction [forced expiratory volume in one-second (FEV1) ≥80% predicted and >5th percentile lower limit of normal (LLN) (i.e., z-score >−1.64) using the 2012-Global Lung Function Initiative equations]. They underwent spirometry, plethysmography, oscillometry, single-breath nitrogen washout, and symptom-limited incremental cycle exercise tests. Thirty-two age-matched nonsmoking controls were also studied. Thirty-three (53%) of smokers had chronic obstructive pulmonary disease by LLN criteria. In smokers [n = 62; age 65 ± 11 years; smoking history 43 ± 19 pack-years; post-bronchodilator FEV1 z-score −0.60 ± 0.72 and FEV1/FVC z-score −1.56 ± 0.87 (mean ± SD)] versus controls, peak oxygen uptake (̇VO2) was 21 ± 7 vs. 32 ± 9 ml/kg/min, and dyspnea/̇VO2 slopes were elevated (both p < 0.0001). Smokers had evidence of peripheral airway dysfunction and maldistribution of ventilation when compared to controls. In smokers versus controls: lung diffusing capacity for carbon monoxide (DLCO) was 85 ± 22 vs. 105 ± 17% predicted, and residual volume (RV)/total lung capacity (TLC) was 36 ± 8 vs. 31 ± 6% (both p < 0.01). The strongest correlates of peak ̇VO2 were DLCO% predicted (r = 0.487, p < 0.0005) and RV/TLC% (r = −0.389, p = 0.002). DLCO% predicted was also the strongest correlate of dyspnea/̇VO2 slope (r = −0.352, p = 0.005). In smokers with mild airway obstruction, associations between resting tests of mechanics and pulmonary gas exchange and exercise performance parameters were weak, albeit consistent. Among these, DLCO showed the strongest association with important outcomes such as dyspnea and exercise intolerance measured during standardized incremental exercise tests.
Chronic obstructive pulmonary disease (COPD) is a common inflammatory disease of the airways, alveoli, and microvasculature that is underdiagnosed in smokers at risk for the disease. The majority of COPD patients have mild airway obstruction Citation(1,2). Symptomatic smokers with mild COPD are at higher risk for earlier mortality and poorer perceived quality of life than nonsmokers Citation(3,4). Moreover, dyspnea and activity restriction are common symptoms among smokers with minor spirometric abnormalities Citation(5,6). In fact, recent large epidemiological studies confirmed that a sizable proportion of smokers with normal spirometry had reduced exercise capacity and increased requirement for inhaled medications Citation(7,8).
Tobacco-related inflammatory injury of the lungs manifests as heterogeneous physiological impairment with highly variable clinical expression Citation(9–12). Thus, simple spirometry provides only a crude assessment of disease pathophysiology, especially in the early stages of the disease. Moreover, spirometric assessments using different criteria for airflow limitation [Global Initiative for Chronic Obstructive Lung Disease (GOLD) fixed ratio versus lower limit of normal (LLN) of forced expiratory volume in one-second (FEV1)/forced vital capacity (FVC)] can produce discrepant results in smokers being screened for COPD, leading in some instances to diagnostic confusion Citation(13–15). Accordingly, the question arises whether additional pulmonary function tests are useful for the clinical evaluation of smokers with troublesome respiratory symptoms despite unremarkable spirometry.
Recent physiological studies conducted during exercise in symptomatic smokers with or without mild COPD have highlighted that exercise limitation is common and is multifactorial Citation(9–12,16). Nevertheless, it has now become clear that respiratory factors such as increased dynamic mechanical constraints and reduced ventilatory efficiency are consistent contributory factors Citation(9–12,16). However, since detailed exercise testing is not a realistic option for many clinicians, the current exploratory physiological study was designed to examine the relationship between a number of resting physiological tests that are widely available and exercise performance parameters such as dyspnea intensity and peak oxygen update (̇VO2). We selected smokers with chronic respiratory symptoms but with mild airway obstruction, as arbitrarily defined by a post-bronchodilator FEV1 greater than 80% predicted and the 5th percentile LLN (i.e., >−1.64 z-score) Citation(15), regardless of whether they fitted COPD diagnostic criteria based on fixed FEV1/FVC ratio. However, we planned a post-hoc comparison of resting and exercise tests (and their inter-relationships) in subgroups of healthy controls and smokers with and without COPD by FEV1/FVC criteria.
The overarching aim of the study was to examine the potential clinical relevance of a panel of resting tests that interrogate respiratory mechanics and pulmonary gas exchange. These include spirometry, plethysmographic lung volumes, tests to measure respiratory impedance [e.g., impulse oscillometry (IOS)] and distribution of ventilation [e.g., single-breath nitrogen washout (SBNW)] Citation(17,18); and lung diffusing capacity for carbon monoxide (DLCO).
The specific objective of our study was, therefore, to answer the novel question: which of the available candidate resting physiological markers are most closely linked to increased activity-related dyspnea and reduced exercise tolerance in smokers with respiratory symptoms being screened for COPD?
Materials and methods
Subjects and study design
This study analyzed data compiled from 62 symptomatic smokers (n = 21 current, n = 41 former) who were screened for COPD for the Canadian Respiratory Research Network database at the Respiratory Investigation Unit (RIU), Queen's University and Kingston General Hospital between 2011 and 2014. Selection criteria included: age ≥40 years; smoking history ≥20 pack-years; body mass index (BMI) 18–35 kg/m2; and a preserved FEV1 (≥80% predicted and greater than the 5th percentile LLN (z-score >−1.64) using Global Lung Function Initiative (GLI) 2012 reference equations) Citation(15). Symptoms included cough/sputum and/or activity-related dyspnea [modified Medical Research Council (MRC) dyspnea scale ≥2 or COPD Assessment Test (CAT) ≥10 or Baseline Dyspnea Index ≤9] Citation(19,20). Thirty-two age-matched nonsmoking healthy subjects from the RIU data registry were also included if they had an FEV1 ≥80% predicted and FEV1/FVC >0.7 and both with z-scores >−1.64 using the GLI-2012 equations Citation(15). Subjects with bronchial asthma and/or other medical conditions that could contribute to dyspnea/exercise limitation or contraindicate exercise testing were excluded. Some subjects were included in previously published studies Citation(10,11,Citation16); however, the current data set is not the same, and there is no overlap with any of these previous analyses.
This study received ethical approval from the Queen's University and Affiliated Teaching Hospitals Research Ethics Board (DMED-1674-14). After signing written informed consents, participants completed two visits to the RIU. Visit 1 included screening for eligibility, medical history, symptom, and activity assessment questionnaires Citation(19–22), Charlson Comorbidity Index Citation(23), pre- and post-bronchodilator (400 μg salbutamol) pulmonary function tests, small airway function tests, and an incremental cardiopulmonary exercise test (CPET) for familiarization. Visit 2 included spirometry followed by an incremental CPET. Subjects refrained from smoking and avoided caffeine, heavy meals, alcohol, and vigorous exercise ≥4 hours before visits. Subjects were not tested if they had respiratory tract infections within 4 weeks. A high-resolution computed tomography (HRCT) scan of the thorax was available for smokers with COPD, and was used for the quantitative assessment of the extent of emphysema Citation(24).
Procedures
Pulmonary function and small airway function tests (SBNW and IOS) Citation(17,18) were performed using automated equipment (Vmax229d and Autobox V62J; and MasterScreen IOS; SensorMedics, Yorba Linda, CA); measurements were expressed relative to predicted normal values Citation(15,25–27). We used predictive equations for DLCO of Burrows et al. Citation(26); these equations worked well in a similar population of 128 healthy men and women previously tested in our laboratory Citation(28). CPETs were conducted on an electronically braked cycle ergometer (Ergoline 800 s; SensorMedics) using a SensorMedics Vmax229d system according to guidelines, as previously described Citation(9). CPETs included steady-state rest followed by stepwise increments (20-watt/2-min) until symptom limitation. Exercise measurements included: breath-by-breath cardiorespiratory and breathing pattern parameters; oxygen saturation (pulse oximetry); heart rate (HR, 12-leads ECG); dynamic lung volumes calculated from inspiratory capacity (IC) with rest-to-peak decrease in IC, reflecting the magnitude of dynamic hyperinflation (DH) Citation(29). Dyspnea and leg discomfort intensity were measured by the modified 10-point Borg scale Citation(30). Exercise measurements were expressed as % predicted normal values when applicable Citation(31–33). The anaerobic threshold (AT) was assessed using the V-slope method, and we also employed the ventilatory equivalent method Citation(34). Furthermore, two different investigators independently verified the identification of the AT.
Statistical analysis
Primary end points included peak ̇VO2, the slope of dyspnea intensity over ̇VO2 and rest-to-peak change (∆) in IC. A sample size of 30 per group should provide sufficient power (>80%) to detect a significant difference in each of these primary end points based on the assumptions: α = 0.05, β = 0.80, a two-tailed test of significance, a 20% between-group difference in peak ̇VO2 and dyspnea/̇VO2 slopes or a 0.2 L difference in ∆IC, and mean and SD values obtained in similar populations tested at our laboratory Citation(9).
Unpaired t-tests were used to compare between-group differences in pulmonary function tests and dyspnea intensity, cardiorespiratory parameters at rest, iso-work rates, and peak exercise. Results are reported as means±SD unless otherwise specified. Statistical significance was set at p < 0.05.
Within the smokers, associations between each of the primary end points and relevant independent variables were tested using Pearson's correlations. Independent resting variables included: post-bronchodilator FEV1/FVC and FEV1, IC, functional residual capacity (FRC), residual volume (RV), total lung capacity (TLC), RV/TLC, DLCO, resistance at 5 Hz (R5), change in airway resistance from 5 to 20 Hz (R5-20), distal capacitive reactance (X5), and phase-III N2 slope. Spirometry and lung volume measurements were expressed as % predicted, and ratios were expressed as % in this analysis. Based on descriptive statistics such as means, Pearson's correlation, and literature review, variables were entered as an important predictor in a linear regression model for each of the primary outcome. The final regression model was based on the diagnostic test such as F-test, adjusted r-square, collinearity test, and normal probability plot. Relationships between important outcomes were tested across smoker subgroups (former versus current smokers, COPD versus non-COPD) using a regression model incorporating an interaction term (group × independent variable). p-values less than 0.05 were used for statistical significance, and all the analyses were conducted using statistical software SAS version 9.3.
Results
The smoker and control groups had similar age, height, and BMI (). Thirty-three (53%) smokers had COPD based on GLI classification (post-bronchodilator FEV1/FVC z-score <−1.64): those with COPD had similar resting (FRC, IC) and dynamic lung volumes, DLCO, IOS, peak ̇VO2 and dyspnea/̇VO2 slopes compared with those without COPD. Both smoker groups (with and without COPD by LLN criteria) were different from controls with regard to respiratory symptoms and resting lung functions (online supplement). Forty-four (71%) smokers met GOLD grade 1 criteria, and eighteen (29%) met the formerly named GOLD 0 (at risk): both groups had similar resting (FRC, IC) and dynamic lung volumes, IOS, and peak ̇VO2. Smokers had a significant smoking history (43 ± 19 pack-years), and controls were never-smokers except for five subjects who had an insignificant smoking history and had stopped smoking >20 years before the study. Smokers had worse activity-related dyspnea, higher CAT scores, lower physical activity (CHAMPS), poorer health status (SGRQ), and higher Charlson Index compared with controls (). 36 of 44 smokers with COPD had chest HRCT scans, and the results revealed 15 ± 9% (range: 2–41%) of their lungs as low-attenuation areas (LAA, less than −950 Hounsfield Units). Comorbidities in the smoker and control groups, respectively, included stable: hypertension (43.5 and 9.3%), gastroesophageal reflux disease (35.5 and 9.3%), hypercholesterolemia (30.6 and 12.5%), osteoarthritis (21.0 and 21.9%), hypothyroidism (14.5 and 9.3%), diabetes mellitus (9.7 and 6.3%), stable angina (8.1 and 0%), and previous myocardial infarction (1.6 and 0%). Inhaled and noninhaled medications are shown in .
Table 1. Subject characteristics and medications.
Pulmonary function and small airway function
summarizes pulmonary function tests. Smokers [post-bronchodilator FEV1/FVC z-score −1.56 ± 0.87 (65 ± 8%); FEV1 z-score −0.60 ± 0.72 (90 ± 11% predicted)] had evidence of pulmonary gas trapping (higher RV/TLC), peripheral airway dysfunction (lower maximal mid-expiratory flow, higher R5-20 and resonant frequency), and maldistribution of ventilation (higher phase-III N2 slope) compared with controls (). Average DLCO was within normal limits in smokers, but significantly reduced compared with controls (85 ± 22 versus 105 ± 17% predicted). In addition, 40% of smokers had DLCO values <80% predicted compared with only 3% of controls. VA/TLC ratio was normal (>0.8) in both groups. A weak correlation was found between DLCO% predicted and degree of emphysema (LAA), (r = −0.386, p = 0.02). In support of successful asthma exclusion, none of the smokers demonstrated significant bronchodilator reversibility.
Table 2. Resting pulmonary function.
Figure 1. Measures of small airway dysfunction, pulmonary gas trapping, and lung diffusing capacity in smokers and age-matched healthy controls. (A) R5-20, (B) X5, (C) phase-III N2 slope, (D) FEF25–75% (% predicted), (E) RV/TLC (%), and (F) DLCO (% predicted). Values in box plots represent the median, 10th, 25th, 75th, and 90th percentile. *p < 0.05 smokers vs. healthy controls. Abbreviations: DLCO = diffusing capacity of the lung for carbon monoxide; FEF25–75% = maximal mid-expiratory flow; N2 = nitrogen; R5-20 = change in airway resistance from 5 to 20 Hz during impulse oscillometry (IOS); RV = residual volume; TLC = total lung capacity; X5 = distal capacitive reactance during IOS.
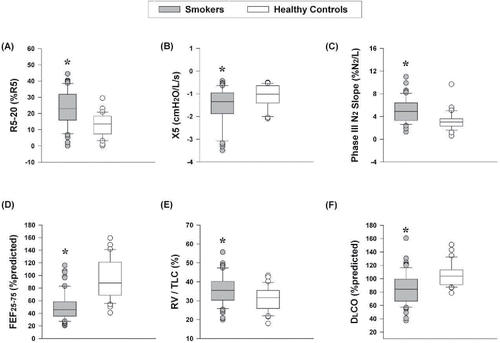
Exercise responses
summarizes peak exercise measurements. Peak work rate, ̇VO2, ventilation (̇VE), and HR were lower in smokers than in controls (all p < 0.0001). HR responses were higher throughout exercise in smokers compared with controls. None of our subjects showed any ischemic electrocardiographic changes during exercise. Smokers had significantly higher ̇VE, ventilatory equivalent for carbon dioxide (̇VE/̇VCO2), and lower end-tidal CO2 at submaximal exercise compared with controls (). Resting ̇VE/̇VCO2 was higher in smokers than controls (47 ± 8 versus 41 ± 5, p < 0.001). The ̇VE to ̇VCO2 relationships expressed as nadir, slope, and intercept were 31 ± 4 versus 27 ± 3 (p < 0.0001), 28 ± 4 versus 27 ± 3 (p = 0.055), and 4.6 ± 1.5 versus 3.3 ± 1.8 (p = 0.000) in smokers versus controls, respectively. Within smokers, resting ̇VE/̇VCO2 correlated well with ̇VE/̇VCO2 nadir (r = 0.611, p < 0005). In turn, ̇VE/̇VCO2 nadir correlated with DLCO% predicted (r = −0.353, p = 0.005). Smokers had lower IC and inspiratory reserve volume (IRV) during submaximal exercise than controls (). Rest-to-peak ΔIC was −0.19 ± 0.47 and 0.09 ± 0.52 L (p = 0.006) in smokers versus controls, respectively. Smokers (with and without COPD) had higher dyspnea intensity and leg discomfort during submaximal exercise than controls (, E1). Dyspnea/̇VE, dyspnea/̇VO2 and leg discomfort/̇VO2 slopes were higher in smokers versus controls (0.12 ± 0.06 versus 0.07 ± 0.04 units/L/min, 0.47 ± 0.38 versus 0.22 ± 0.14 and 0.53 ± 0.32 versus 0.27 ± 0.14 units/ml/kg/min, respectively, all p < 0.0001); differences were also present when ̇VO2 was expressed as % predicted [0.13 ± 0.10 versus 0.07 ± 0.05 (p = 0.001) and 0.16 ± 0.14 versus 0.08 ± 0.05 (p = 0.003), Borg scale units/% predicted]. AT occurred at a lower ̇VO2 in smokers versus controls (1.30 ± 0.40 versus 1.63 ± 0.49 L/min, p = 0.001).
Table 3. Measurements at the peak of symptom-limited incremental cycle exercise.
Figure 2. Selected parameters of responses to incremental cycle exercise plotted against work rate in smokers and age-matched healthy controls. (A) Exertional dyspnea intensity using Borg scale, (B) ̇VE, (C) ̇VE/̇VCO2, (D) PetCO2, (E) IC, (F) IRV. Values are mean ± SEM. Square symbols represent the points at the highest equivalent work arte of 60 watts. *p < 0.05 smokers vs. healthy controls at rest, standardized work rates or at peak exercise. Abbreviations: IC = inspiratory capacity; IRV = inspiratory reserve volume; PetCO2 = partial pressure of end-tidal carbon dioxide; TLC = total lung capacity; ̇VE = minute ventilation; ̇VE/̇VCO2 = ventilatory equivalent for carbon dioxide.
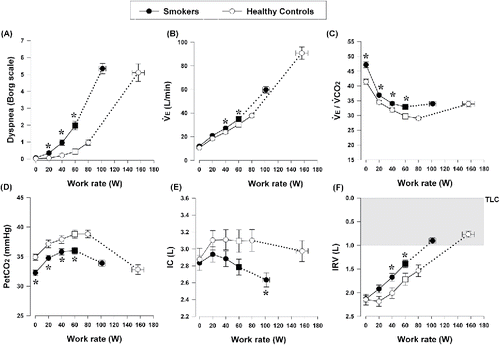
Physiological correlations
Resting physiological correlates of each of the primary end points within smokers are summarized in . DLCO% predicted and RV/TLC% were the strongest correlates of peak ̇VO2 (ml/kg/min); their combination explained 35% of the variance in peak ̇VO2. In the secondary analysis conducted across smoker subgroups, significant relationships were found to be similar in former versus current smokers as well as in those with COPD versus without COPD. Although the cutoffs are arbitrary, it is interesting that 80% of smokers with a combination of high RV/TLC (>35%) Citation(35) and low DLCO (<80% predicted) had reduced peak ̇VO2 (≤20 ml/kg/min) Citation(36).
Table 4. Correlates of each of the primary outcomes within smokers.
Peak ̇VO2 (ml/kg/min) also correlated with exercise slopes of the intensity of dyspnea/̇VO2 (r = −0.381, p = 0.002) and leg discomfort/̇VO2 (r = −0.452, p < 0.0005). In turn, dyspnea/̇VO2 correlated best with DLCO% predicted (r = −0.352, p = 0.005) (). Slopes of leg discomfort/̇VO2 were not associated with any resting respiratory measurements but did correlate inversely with ̇VO2 at the AT (r = −0.324, p = 0.012). summarizes inter-relationships between DLCO% predicted, peak ̇VO2, dyspnea/̇VO2 slope and ̇VE/̇VCO2 nadir within the smoker group.
Figure 3. Inter-relationships between DLCO% predicted, peak ̇VO2, dyspnea/̇VO2 slope, and ̇VE/̇VCO2 nadir within the smoker group. DLCO correlated with peak ̇VO2 (r = 0.487, p < 0.0005), dyspnea/̇VO2 slope (r = −0.352, p = 0.005), and ̇VE/̇VCO2 nadir (r = −0.353, p = 0.005). Abbreviations: DLCO = diffusing capacity of the lung for carbon monoxide; ̇VE/̇VCO2 = ventilatory equivalent for carbon dioxide; ̇VO2 = oxygen uptake.
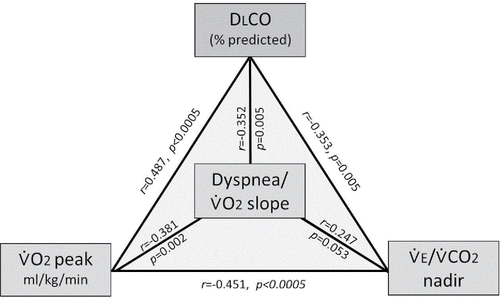
The best independent correlates of rest-to-peak ΔIC were, in order of significance: IOS measurements (R5-20, X5), FEV1/FVC, and resting IC% predicted (). The combination of R5-20, X5, and IC% predicted explained 39% of the variance in DH.
Discussion
The main findings of this study were that symptomatic smokers with a preserved FEV1 had heterogeneous respiratory impairment as exposed by a number of sensitive tests. Among these, decreased DLCO and increased RV/TLC provided the most consistent associations with reduced exercise tolerance. A low DLCO was also the best correlate of increased exertional dyspnea. Beyond the FEV1, these additional resting tests helped expose the nature and extent of the respiratory impairment in individual smokers and provided insights into the source of their symptoms.
Our group of smokers who had persistent respiratory symptoms (cough, sputum, and/or activity-related dyspnea) was recruited as a result of targeted screening for COPD. As anticipated Citation(37), different diagnostic spirometric criteria for COPD yielded discrepant results: 44 of 62 met GOLD grade 1 criteria, but 33 of 62 had COPD using the more stringent GLI criteria, which accounts for effects of aging. Smokers with/without COPD were similar in baseline lung function and peak exercise measures, but both the groups were significantly different from controls (online supplement). An a priori selection of smokers with FEV1 ≥80% predicted and > the 5th percentile LLN was made to increase the likelihood of recruiting smokers without significant airway obstruction. Among such individuals, those with persistent symptoms have been shown in several population studies to have increased morbidity and mortality compared with nonsmokers Citation(38,39). Indeed, the smoker group of the current study had poorer perceived health status, lower self-reported daily physical activity, and greater chronic activity-related dyspnea (measured by various questionnaires) compared with controls (). This was corroborated by the reduced peak ̇VO2 (by over 30% on average) and higher dyspnea intensity ratings in smokers. In accordance with previous studies Citation(9–12,Citation16), reduced exercise tolerance in smokers was multifactorial and included severe dyspnea and leg effort. Our results confirmed that contributing respiratory factors included reduced ventilatory efficiency, higher ̇VE, lower IC, and critical dynamic respiratory mechanical constraints and associated respiratory discomfort at relatively lower exercise intensities compared with controls (). Notably, in smokers, IRV decreased toward its minimal value at a peak work rate that was 38% lower than that of healthy controls.
There has recently been renewed interest in IOS and N2 washout tests and their potential utility in early detection of respiratory impairment in smokers. In the current study, these tests, on average, showed greater respiratory impedance and maldistribution of ventilation in smokers than in healthy controls (). Interestingly, R5-20 correlated with the extent of DH during exercise, both measurements indicating an exaggerated frequency dependence of dynamic resistance in smokers. Abnormal configuration of the mid-volume maximal expiratory flow-volume loop and increased pulmonary gas trapping were more likely in smokers compared with healthy controls (): this pointed to the presence of extensive small airway dysfunction despite a mean FEV1 of 90% predicted and plethysmographic lung volumes and resting IC within the normal range.
Lung microvascular inflammation, disruption of the alveolar-capillary interface and reduced pulmonary perfusion are increasingly identified in smokers with minor spirometric abnormalities although precise quantification of these derangements is challenging Citation(40,41). In this context, a recent imaging study has provided evidence of reduced pulmonary blood flow (by 30%) even in mild COPD patients Citation(41). We have shown that reduced ventilatory efficiency during exercise (high ̇VE/̇VCO2 nadir) is common in mild COPD and that this mainly reflects high physiological dead space (rather than alveolar hyperventilation or relatively reduced tidal volume) and thus a preponderance of lung units with high ventilation–perfusion ratios Citation(11).
The determinants of DLCO include: ventilation distribution, ventilation/perfusion matching, resistance at the alveolar-capillary membrane, and combination rates with hemoglobin. It is noteworthy that in the current study, DLCO and KCO (DLCO/VA), while within the low normal range by the American Thoracic Society criteria, were consistently lower in smokers than in controls by an average of 20%, with no difference in VA/TLC (a crude measure of ventilatory inhomogeneity) Citation(42). DLCO provides a window into the microvasculature, and low values have previously been linked with activity-related dyspnea (Borg scale and Baseline Dyspnea Index) and low peak ̇VO2 in moderate-to-severe COPD Citation(29,43,Citation44). A plausible mechanism is increased chemostimulation of ventilation secondary to effects of high physiological dead space as a result of presumed emphysema and reduced surface area for gas exchange
Modest but consistent reduction in DLCO has been previously reported in smokers with or without mild COPD in the absence of structural changes of emphysema on computed tomography (CT) Citation(37,45,Citation46). In one study by Kirby et al. Citation(40), low DLCO correlated with reduced 6-minute walking distance in ex-smokers with normal chest CT scans and spirometry. The observation in the current study that DLCO was associated with high ̇VE/̇VCO2 nadir, low peak ̇VO2, and increased exertional dyspnea ratings within smokers (regardless of the presence of COPD by GLI criteria) supports the notion that similar physiological mechanisms of dyspnea may exist as have been postulated in more advanced COPD () Citation(29,43,Citation44). It is noteworthy that smokers who met GOLD grade 1 criteria had minor upper lobe structural changes consistent with centrilobular emphysema (15% LAA <−950 HU). In this group, we found a weak correlation between % LAA and DLCO consistent with the results of other studies in more advanced COPD Citation(47–49). Thus, in the smoker group, the lower DLCO likely reflects the presence of greater ventilation/perfusion inequalities, reduced efficiency of CO2 elimination, and consequent higher ventilatory stimulation. We postulate, based on the results of previous physiological studies in mild COPD that Citation(9–12), that this higher demand in the presence of increased peripheral airway dysfunction and expiratory flow limitation forces earlier dynamic mechanical constraints at lower exercise intensities than in nonsmoker controls. In some individuals, ventilatory demand was likely further amplified by lower ventilatory thresholds reflecting earlier metabolic acidosis due to deconditioning (). This basic physiological construct is in line with recent exercise studies in smokers at risk for COPD or in those with mild COPD where increased exertional dyspnea (compared with healthy controls) at standardized ventilation was explained by the relatively higher fractional inspiratory neural drive to the diaphragm Citation(12,16).
This is the first study to examine the relative strength of the association of a wide range of resting pulmonary function tests to peak symptom-limited ̇VO2 and exertional dyspnea intensity in smokers with a preserved FEV1. We found that although exercise limitation was undoubtedly multifactorial and included both respiratory and nonrespiratory factors, the combination of reduced DLCO and respiratory mechanical factors (increased RV/TLC) explained 35% of the variance in peak ̇VO2. This relation was not affected by differences in FEV1/FVC within the smokers and was similar in active and former smokers. The practical implication is that mechanical factors such as increased RV are amenable to inhaled bronchodilators and have the potential to improve exertional dyspnea, at least based on the results of a previous study in patients with GOLD grade 1 COPD Citation(50). By contrast, a reduced DLCO is currently not responsive to pharmacological treatment but improves with smoking cessation Citation(51).
Clearly, nonrespiratory factors also contributed to the lower peak symptom-limited ̇VO2 in the smoker group who reported higher intensity of perceived leg discomfort and had lower AT compared with controls. This suggests a higher prevalence of skeletal muscle deconditioning in smokers, which is potentially amenable to multimodality exercise training.
Limitations
Our study sample was confined to symptomatic Canadian Caucasian smokers at risk for COPD who were targeted for screening according to recent guidelines. Our results may not therefore be generalizable to asymptomatic smokers. However, we and others have observed that so-called “asymptomatic” smokers (by modified MRC and CAT criteria) often have greater dyspnea and exercise intolerance than healthy controls when challenged with formal exercise testing Citation(52). DLCO was not corrected for between-subject differences in circulating hemoglobin, and although smokers were instructed to refrain from smoking prior to the test, baseline carboxyhemoglobin was not collected. Factors such as reduced physical activity and increased leg discomfort contributed to the lower peak ̇VO2 in smokers; however, this did not detract from our analysis of the associations between the panel of tests of respiratory impairment and activity-related dyspnea.
Conclusions/implications
This study adds to the evidence that cardiopulmonary exercise testing is useful in uncovering significant and often unsuspected physiological derangements in smokers with preserved FEV1. The overarching message is that the presence of persistent respiratory symptoms in smokers—regardless of whether they meet COPD LLN criteria—should not be ignored. Such individuals who were formerly designated “smokers at risk” (or GOLD 0) likely have combinations of peripheral airway dysfunction and some degree of pulmonary gas exchange inefficiency to explain their relatively reduced exercise tolerance. While the value of screening of symptomatic smokers using spirometry is undisputed, the results show that mild reduction in FEV1 may mask physiological impairment that can contribute to negative clinical outcomes in some smokers. In this context, it is evident that among the available resting physiological tests, those that measure lung diffusing capacity and pulmonary gas trapping show the strongest associations with the common symptoms of exercise intolerance and dyspnea.
ICOP_A_1281901_supplemental.docx
Download MS Word (111.4 KB)Funding
Prof. Denis O'Donnell has received funding for this research through Ontario Thoracic Society; William Spear/Richard Start Endowment Fund, Queen's University; Canadian Respiratory Research Network (CRRN). The CRRN is supported by grants from the Canadian Institutes of Health Research (CIHR)—Institute of Circulatory and Respiratory Health; Canadian Lung Association (CLA)/Canadian Thoracic Society (CTS); British Columbia Lung Association; and Industry Partners Boehringer Ingelheim Canada Ltd, AstraZeneca Canada Inc., and Novartis Canada Ltd. Amany F. Elbehairy has received financial support through the John Alexander Stewart scholar award from Queen's University.
Declaration of interest
The funders had no role in the study design, data collection and analysis, or preparation of the manuscript. Amany F. Elbehairy, Azmy Faisal, Jordan A. Guenette, Dennis Jensen, Katherine A. Webb, Rashid Ahmed and J. Alberto Neder have no conflicts of interest that are directly relevant to the content of this article.
Author contributions
All the authors played a role in the content and writing of the manuscript. In addition, D.E.O. was the principal investigator and provided the original idea for the study; D.E.O. and K.A.W. had input into the study design and conduct of study; K.A.W., J.A.G., and A.F.E. collected the data; A.F.E., A.F., J.A.G., K.A.W., and R.A. performed data analysis and prepared it for presentation.
References
- Mannino DM, Homa DM, Akinbami LJ, Ford ES, Redd SC. Chronic obstructive pulmonary disease surveillance—United States, 1971–2000. Respir Care 2002; 47(10):1184–1199.
- Buist AS, McBurnie MA, Vollmer WM, Gillespie S, Burney P, Mannino DM, et al. BOLD Collaborative Research Group. International variation in the prevalence of COPD (the BOLD study): a population based prevalence study. Lancet 2007; 370:741–750.
- Antonelli-Incalzi R, Imperiale C, Bellia V, Catalano F, Scichilone N, Pistelli R, et al. SaRA Investigators. Do GOLD stages of COPD severity really correspond to differences in health status? Eur Respir J 2003; 22:444–449.
- Sin DD, Wu L, Man SF. The relationship between reduced lung function and cardiovascular mortality: a population-based study & a systematic review of the literature. Chest 2005; 127:1952–1959.
- Furlanetto KC, Mantoani LC, Bisca G, Morita AA, Zabatiero J, Proença M, et al. Reduction of physical activity in daily life and its determinants in smokers without airflow obstruction. Respirology 2014; 19:369–375.
- Vozoris NT, O'Donnell DE. Smoking, activity level and exercise test outcomes in a young population sample without cardiopulmonary disease. J Sports Med Phys Fitness 2015; 55(7–8):787–796.
- Woodruff PG, Barr RG, Bleecker E, Christenson SA, Couper D, Curtis JL, et al. SPIROMICS Research Group. Clinical significance of symptoms in smokers with preserved pulmonary function. N Engl J Med 2016; 374(19):1811–1821.
- Bhatt SP, Soler X, Wang X, Murray S, Anzueto AR, Beaty TH, et al. Association between functional small airway disease and FEV1 decline in chronic obstructive pulmonary disease. Am J Respir Crit Care Med. 2016; 194(2):178–184.
- Ofir D, Laveneziana P, Webb KA, Lam YM, O'Donnell DE. Mechanisms of dyspnea during cycle exercise in symptomatic patients with GOLD stage I chronic obstructive pulmonary disease. Am J Respir Crit Care Med 2008; 177:622–629.
- Elbehairy A, Raghavan N, Cheng S, Yang L, Webb KA, Neder JA, et al. Canadian Respiratory Research Network. Physiological characterization of the chronic bronchitis phenotype in GOLD grade 1B COPD. Chest 2015; 147:1235–1245.
- Elbehairy AF, Ciavaglia CE, Webb KA, Guenette JA, Jensen D, Mourad SM, et al. Canadian Respiratory Research Network. Pulmonary gas exchange abnormalities in mild COPD: implications for exercise intolerance. Am J Respir Crit Care Med 2015; 191:1384–1394.
- Guenette JA, Chin RC, Cheng S, Dominelli PB, Raghavan N, Webb KA, et al. Mechanisms of exercise intolerance in Global Initiative for Chronic Obstructive Lung Disease grade 1 COPD. Eur Respir J 2014; 44:1177–1187.
- van Dijk W, Tan W, Li P, Guo B, Li S, Benedetti A, et al. CanCOLD Study Group. Clinical relevance of fixed ratio vs. lower limit of normal of FEV1/FVC in COPD: patient-reported outcomes from the CanCOLD cohort. Ann Fam Med 2015; 13(1):41–48.
- Global Initiative for Chronic Obstructive Lung Disease. Global strategy for the diagnosis, management, and prevention of chronic obstructive pulmonary disease. 2016. Available from: http://www.goldcopd.org (last accessed: 15 March 2016).
- Quanjer PH, Stanojevic S, Cole TJ, Baur X, Hall GL, Culver BH, et al. the ERS Global Lung Function Initiative. Multi-ethnic reference values for spirometry for the 3–95 years age range: the Global Lung Function 2012 equations. Eur Respir J 2012; 40:1324–1343.
- Elbehairy AF, Guenette JA, Faisal A, Ciavaglia CE, Webb KA, Jensen D, et al. Canadian Respiratory Research Network. Mechanisms of exertional dyspnoea in symptomatic smokers without COPD. Eur Respir J 2016; 48(3):694–705.
- Oostveen E, MacLeod D, Lorino H, Farré R, Hantos Z, Desager K, et al. ERS Task Force on Respiratory Impedance Measurements. The forced oscillation technique in clinical practice: methodology, recommendations and future developments. Eur Respir J 2003; 22:1026–1041.
- Buist AS, Ross BB. Quantitative analysis of the alveolar plateau in the diagnosis of early airway obstruction. Am Rev Respir Dis 1973; 108:1078–1087.
- Jones PW, Harding G, Berry P, Wiklund I, Chen WH, Kline Leidy N, et al. Development and first validation of the COPD Assessment Test. Eur Respir J 2009; 34:648–654.
- Mahler DA, Weinberg DH, Wells CK, Feinstein AR. The measurement of dyspnea. Contents, interobserver agreement, and physiologic correlates of two new clinical indexes. Chest 1984; 85:751–758.
- Jones PW, Quirk FH, Baveystock CM, Littlejohns P. A self-complete measure of health status for chronic airflow limitation. The St. George's Respiratory Questionnaire. Am Rev Respir Dis 1992; 145:1321–1327.
- Stewart AL, Mills KM, King AC, Haskell WL, Gillis D, Ritter PL, et al. CHAMPS physical activity questionnaire for older adults: outcomes for interventions. Med Sci Sports Exerc 2001; 33:1126–1141.
- Charlson ME, Pompei P, Ales KL, MacKenzie CR. A new method of classifying prognostic comorbidity in longitudinal studies: development and validation. J Chronic Dis 1987; 40:373–383.
- Coxson HO, Rogers RM, Whittall KP, D'yachkova Y, Pare´ PD, Sciurba FC, et al. quantification of the lung surface area in emphysema using computed tomography. Am J Respir Crit Care Med 1999; 159:851–856.
- Crapo RO, Morris AH, Clayton PD, Nixon CR. Lung volumes in healthy nonsmoking adults. Bull Eur Physiopathol Respir 1982; 18:419–425.
- Burrows B, Kasik JE, Niden AH, Barclay WR. Clinical usefulness of the single-breath pulmonary diffusing capacity test. Am Rev Respir Dis 1961; 84:789–806.
- Briscoe WA, Dubois AB. The relationship between airway resistance, airway conductance and lung volume in subjects of different age and body size. J Clin Invest 1958; 37:1279–1285.
- Deesomchok A, Webb KA, Forkert L, Lam YM, Ofir D, Jensen D, et al. Lung hyperinflation and its reversibility in patients with airway obstruction of varying severity. COPD 2010; 7(6):428–437.
- O'Donnell DE, Revill SM, Webb KA. Dynamic hyperinflation and exercise intolerance in chronic obstructive pulmonary disease. Am J Respir Crit Care Med 2001; 164(5):770–777.
- Borg GA. Psychophysical bases of perceived exertion. Med Sci Sports Exerc 1982; 14:377–381.
- Blackie SP, Fairbarn MS, McElvaney GN, Morrison NJ, Wilcox PG, Pardy RL, et al. Prediction of maximal oxygen uptake and power during cycle ergometry in subjects older than 55 years of age. Am Rev Respir Dis 1989; 139(6):1424–1429.
- Jones NL. Clinical Exercise Testing, 3rd ed. Philadelphia, PA: WB Saunders Co, 1988, 152–155.
- Pellegrino R, Viegi G, Brusasco V, Crapo RO, Burgos F, Casaburi R, et al. Interpretative strategies for lung function tests. Eur Respir J 2005; 26(5):948–968.
- Gaskill SE, Ruby BC, Walker AJ, Sanchez OA, Serfass RC, Leon AS, et al. Validity and reliability of combining three methods to determine ventilatory threshold. Med Sci Sports Exerc 2001; 33(11):1841–1848.
- Jain VV, Abejie B, Bashir MH, Tyner T, Vempilly J. Lung volume abnormalities and its correlation to spirometric and demographic variables in adult asthma. J Asthma 2013; 50(6):600–605.
- Weber KT, Kinasewitz GT, Janicki JS, Fishman AP. Oxygen utilization and ventilation during exercise in patients with chronic cardiac failure. Circulation 1982; 65:1213–1223.
- Wollmer P, Frantz S, Engström G, Dencker M, Löfdahl CG, Nihlén U, et al. Fixed ratio or lower limit of normal for the FEV1/VC ratio: relation to symptoms and extended lung function tests. Clin Physiol Funct Imaging. Published Online First Oct 2016; 6. doi: 10.1111/cpf.12294.
- Bridevaux PO, Gerbase MW, Probst-Hensch NM, Schindler C, Gaspoz JM, Rochat T, et al. Long term decline in lung function, utilisation of care and quality of life in modified GOLD stage 1 COPD. Thorax 2008; 63:768–774.
- Stavem K, Sandvik L, Erikssen J. Can Global Initiative for Chronic Obstructive Lung Disease stage 0 provide prognostic information on long-term mortality in men? Chest 2006; 130:318–325.
- Kirby M, Owrangi A, Svenningsen S, Wheatley A, Coxson HO, Paterson NA, et al. On the role of abnormal DL(CO) in ex-smokers without airflow limitation: symptoms, exercise capacity and hyperpolarised heliu-3 MRI. Thorax 2013; 68:752–759.
- Hueper K, Vogel-Claussen J, Parikh MA, Austin JH, Bluemke DA, Carr J, et al. Pulmonary microvascular blood flow in mild chronic obstructive pulmonary disease and emphysema. The MESA COPD Study. Am J Respir Crit Care Med 2015; 192(5):570–580.
- Neder JA, O'Donnell CD, Cory J, Langer D, Ciavaglia CE, Ling Y, et al. Ventilation distribution heterogeneity at rest as a marker of exercise impairment in mild-to-advanced COPD. COPD 2015; 12(3):249–256.
- Farkhooy A, Janson C, Arnardóttir RH, Malinovschi A, Emtner M, Hedenström H, et al. Impaired carbon monoxide diffusing capacity is the strongest predictor of exercise intolerance in COPD. COPD 2013; 10(2):180–185.
- Nishimura K, Yasui M, Nishimura T, Oga T. Airflow limitation or static hyperinflation: which is more closely related to dyspnea with activities of daily living in patients with COPD? Respir Res 2011; 12:135.
- Harvey BG, Strulovici-Barel Y, Kaner RJ, Sanders A, Vincent TL, Mezey JG, et al. Risk of COPD with obstruction in active smokers with normal spirometry and reduced diffusion capacity. Eur Respir J 2015; 46(6):1589–1597.
- Gordon C, Gudi K, Krause A, Sackrowitz R, Harvey BG, Strulovici-Barel Y, et al. Circulating endothelial microparticles as a measure of early lung destruction in cigarette smokers. Am J Respir Crit Care Med. 2011; 184(2):224–232.
- Crisafulli E, Alfieri V, Silva M, Aiello M, Tzani P, Milanese G, et al. Relationships between emphysema and airways metrics at High-Resolution Computed Tomography (HRCT) and ventilatory response to exercise in mild to moderate COPD patients. Respir Med. 2016; 117:207–214.
- Lee SL, Ra SW, Chae EJ, Seo JB, Lim SY, Kim TH, et al. Validation of the lower limit of normal diffusing capacity for detecting emphysema. Respiration 2011; 81(4):287–293.
- Nambu A, Zach J, Schroeder J, Jin GY, Kim SS, Kim YI, et al. Relationships between diffusing capacity for carbon monoxide (DLCO), and quantitative computed tomography measurements and visual assessment for chronic obstructive pulmonary disease. Eur J Radiol 2015; 84(5):980–985.
- O'Donnell DE, Laveneziana P, Ora J, Webb KA, Lam YM, Ofir D, et al. Evaluation of acute bronchodilator reversibility in patients with symptoms of GOLD stage I COPD. Thorax 2009; 64(3):216–223.
- Dhariwal J, Tennant RC, Hansell DM, Westwick J, Walker C, Ward SP, et al. Smoking cessation in COPD causes a transient improvement in spirometry and decreases micronodules on high-resolution CT imaging. Chest 2014; 145(5):1006–1015.
- Soumagne T, Laveneziana P, Veil-Picard M, Guillien A, Claudé F, Puyraveau M, et al. Asymptomatic subjects with airway obstruction have significant impairment at exercise. Thorax 2016; 71(9):804–811.