ABSTRACT
The fiber length, chemical composition, and mycototoxins of oat and barley hulls were analyzed in order to study the suitability to utilize hulls for paper and paperboard manufacturing. Oat fibers were observed to be slightly longer than those of barley (0.80 ± 0.19 mm and 0.51 ± 0.17 mm, respectively). There were no differences in the composition of the hemicellulose sugars, with the predominance of xylose and glucose. The amount of Klason lignin in oat and barley hulls was at almost the same level (23% and 25% of dry weight, respectively). Guaiacyl (G) units derived from coniferyl alcohol dominated as shown by pyrolysis-GC-MS. To achieve fibrillation beneficial for the papermaking, oat and barley hulls were refined with a disc refiner. Oat hulls were observed to be harder than barley hulls. This affected both calendering and the appearance of the sheets produced. Laboratory sheets were prepared in a sheet-former with different percentage of softwood pulp and oat and barley hulls. Hull-containing paper mostly met the same quality requirements as the commercial paper except for the burst strength in sheets containing either species’ hulls, and the tensile strength in sheets containing barley hulls.
摘要
为了研究燕麦和大麦皮在造纸和纸板生产中的适用性, 对燕麦和大麦皮的纤维长度, 化学成分和真菌毒素进行了分析. 燕麦纤维比大麦纤维稍长 (分别为0.80±0.19mm和0.51±0.17mm) . 半纤维素糖的组成没有差异, 主要是木糖和葡萄糖. 大麦和燕麦壳中Klason木质素的含量几乎相同 (分别为干重的23%和25%) . 热解-GC-MS显示, 愈创木酰 (G) 单元主要来自松柏醇. 为了实现有利于造纸的纤维化, 燕麦和大麦壳用盘磨机精炼. 燕麦壳比大麦壳硬. 这影响了压延和所生产板材的外观. 实验室用不同比例的软木浆, 燕麦和大麦壳在成纸机中制备板材. 除含有任一种外壳的纸张的耐破强度和含有大麦外壳的纸张的抗拉强度外, 含有外壳的纸张基本上满足与商业纸张相同的质量要求.
Introduction
The aim of today’s industry is to convert all available biomass to high-quality products. For example, food industry generates a large quantity of side streams that could be utilized in various product applications. Barley and oats are among the seven most cultivated cereals in the world in terms of grain production (Welch Citation2005). The global production of oats and barley was 23 and 159 million tons in 2019, respectively (FAO Citation2019). Industrial processes for food production produce traceable, fiber-rich by-product fractions, hulls, that could be used in the circular economy. Oat milling or dehulling aims to remove the tough, fibrous hulls to yield groats, and the cutting and/or rolling of the groats produces various types of oatmeal such as pinhead oats or rolled oat flakes (Ganssmann and Vorwerck Citation1995). Oat hulls, or husks, make up about a quarter of oat grains by weight (Ganssmann and Vorwerck Citation1995), and yet have a much lower packing density than the groats they surround.
The major chemical constituents of oat hulls have been reported to be cellulose and hemicellulose (Welch, Citation2005). Similar to that in cereal straw, lignin concentration of the hulls is negatively correlated with digestibility (Crosbie et al. Citation1984; Thompson et al. Citation2000). The total phenolic content has been found to be high in the hulls, with ca. 80% of the grain hydroxycinnamic acids (ferulic and p-coumaric acid) being present in the hulls and aleurone layers (Du and Yu Citation2011), whereas the antioxidant activity is higher in the groats (Emmons and Peterson Citation1999). Hulls contain high amounts of xylan (Crosbie et al. Citation1984). Hence, this side product is an interesting source for the isolation of xylan and production of xylitol (Saake et al. Citation2004). The hulls of barley contain four cell layers (Olkku et al. Citation2005) and constitute 10–23 wt.% of the grain whole crop (Du and Yu Citation2011; Salo and Kotilainen Citation1970). They lack high-value-added applications, although they have been reported to contain valuable compounds such as hemicelluloses (ca. 30 wt.% of the solid material) with arabinoxylan as the primary hemicellulose (Aspinall and Ferrier, Citation1957; Krawczyk et al. Citation2008). In addition, cellulose and lignin are present, and a small amount of protein (ca. 3%) (Olkku et al. Citation2005). The structure and chemical features of oat and barley grains have been studied extensively in the food industry but much less information is available for hulls. Bioactive compound groups of barley and oat groats has been reviewed by Gangopadhyay et al. (Citation2015). No such information exists for the hulls.
Paper made of Kraft pulp must possess specific mechanical properties. Thus, the aims of the present work were to analyze the suitability of hulls for paper making, i.e. the fiber length, cell wall composition (cellulose, hemicelluloses, lignin), as well as fiber separation and fiber wall fibrillation. Extractive content and composition were studied since they may influence defibrillation, papermaking and the utilization of hull in food packaging material. The main aim was to investigate whether oat or barley hulls could partially replace conventional softwood pulp in packaging material like paperboard. Further goal was to optimize the pre-treatment of the hulls, to make the paper visually appealing.
Materials and methods
Hulls of oats (Avena sativa L.) were obtained from Oy Karl Fazer Ab, Fazer Mill & Mixes, Lahti, Finland. All oats they use are cultivated in the Nordic countries. Hulls of barley (Hordeum vulgare L.) were obtained from Altia Oyj, Koskenkorva Plant/Distillery, Ilmajoki, Finland. Dry hulls were pulverized with a ball mill (Retsch GmbH, type MM 400, Haan, Germany) or with a KCH-Analytical mill A 10 (Kinematica AG, Littau-Lucerne, Switzerland).
Microscopy
Oat and barley hulls were macerated with either a nitric acid maceration method (modified from Mahesh, Kumar, and Ansari Citation2015), or with a H2O2-acetic acid maceration method (modified from Franklin Citation1945) followed by microscopy in order to study fiber length and fibrillation. The macerated samples were examined with a light microscope (Olympus BX50, Tokyo, Japan), imagined, and lengths of the fibers measured with the Image-Pro Plus 7 software (Media Cybernetics, Inc., Rockville, MD, USA). The micrographs were taken with a digital microscope (Olympus DSX1000, Tokyo, Japan).
Chemical analysis
The ground hull samples were analyzed for extractives by ASE extraction-gas chromatography (GC), hemicelluloses by acid methanolysis-GC, cellulose by acid hydrolysis-GC and lignin content by two stage sulfuric acid hydrolysis as described in Korkalo et al. (Citation2020) and Raitanen et al. (Citation2020). The lignin subunit composition was analyzed with pyrolysis-GC-MS in the Åbo Akademi University in the Wood and Paper Chemistry laboratory, Finland. The analysis is described in Viljamaa et al. (Citation2018).
A batch of oat hulls used in this study was analyzed for Fusarium mycotoxins using an accredited GC-MS method (Hietaniemi et al. Citation2016). In brief, 10 g of ground hull samples were extracted with 84% acetonitrile (VWR, Prolabo, Leuven, Belgium). The raw extract was purified with MycoSep #227 SPE column (BioPure, Romer Labs, Tulln, Austria), and the cleaned-up extract was transferred into a silylated test tube and evaporated to dryness. After derivatization with trimethylsilyl iodine (Thermo Fischer Scientific Oy, Vantaa, Finland), the compounds were analyzed by GC-MS and their elution times and peak areas were compared to those of trimethylsilyl (TMS)-derivatives of external reference compounds and 19-nortestosterone (an internal standard). Pure trichothecene compounds deoxynivalenol (DON), diacetoxyscirpenol (DAS), 3-acetyldeoxynivalenol (3-AcDON), 15-acetyldeoxynivalenol (15-AcDON), fusarenon-X (F-X), nivalenol (NIV), T-2 toxin, HT-2 toxin were purchased from BioPure, Romer Labs (Tulln, Austria). They were derivatized in the same way as the sample extracts and used for quantification. The limit of quantitation was 25 µg/kg. The results were confirmed by a recovery test in the same sample matrix.
Papermaking
To aid fibrillation beneficial for the package-making, oat and barley hulls were refined with a 12” Sprout Waldron disc refiner (Sprout, Waldron & Co., Inc., Muncy, PA, USA) containing a refiner plate type D2C505. The two-stage refining conditions are listed in .
Table 1. Refining conditions for oat and barley hulls.
For the hull materials in the first stage, a 1-min preheating (130°C) with 1.8 bar pressure was applied with a disc rotation speed 2500 rpm. In the second refining stage, the refiner pressure was increased, and the plate gap was decreased (). Also, the production rate was decreased.
According to the pretests, hull supplements of 10% and 20% were selected to be added into the softwood (pine and spruce) pulp (Metsä Fiber, Rauma, Finland). The pulp was beaten according to ISO 5264/1-1979 (E) standard. After the beating process, the pulp was dewatered in a filter cloth by pressing it manually. The dry matter content of the dewatered pulp was determined, and the pulp was used for papermaking.
Laboratory sheets were prepared in a sheet-former (wire screen 0.165 m x 0.165 m) according to ISO 5269–1:1998. The target grammage of the sheets was 60 g/m2. Five pulp suspensions of 0.817 g/L were prepared according to . The pressed sheets were finally dried in a drying cylinder (Oy E. Sarlin Ab, Helsinki, Finland) for 4 hours at 90°C. The dried sheets were conditioned according to ISO 187:1990 at 23°C and 50% relative humidity. The conditioned sheets were cut into 0.15 m x 0.15 m squares and half of the sheets were calendered (Tradek Oy, Pietarsaari, Finland) five times using a nip load of 120 kN/m.
Table 2. Stocks for obtaining laboratory sheets with specific grammage (60 g/m2).
Mechanical, physical and optical properties of the paper sheets were measured. Thickness was measured with a micrometer (Lorentzen & Wettre/ABB, Stockholm, Sweden). Surface smoothness/roughness and air permeability were analyzed with a Parker Print Surf (PPS) meter (Messmer Büchel, model 14590500, Veneendaal, the Netherlands). Optical properties were measured with a color meter (Minolta, CM-3610d, Osaka, Japan). A tensile tester (Lorentzen & Wettre/ABB, Stockholm, Sweden) was used to determine the tensile strength and the breaking strength. The bursting strength of the sheets was measured with a bursting strength meter (Lorentzen & Wettre/ABB, Stockholm, Sweden), and the tear strength with a tearing strength meter (Lorentzen & Wettre/ABB, Stockholm, Sweden).
Results
In the present work, two maceration methods were used for the oat and barley hulls. Nitric acid maceration worked better than the H2O2-acetic acid treatment (data not shown). This was probably due to the amount of lignin in the hulls. Oat fibers were observed to be slightly longer than those of barley (0.80 ± 0.19 mm and 0.51 ± 0.17 mm, respectively). The macerated hull samples can be seen in
Figure 1. Micrographs of macerated hull samples; barley (a), oats (b). Thick-walled fibers and aggregations of epidermial cells are visible. The tissue is only partially macerated.
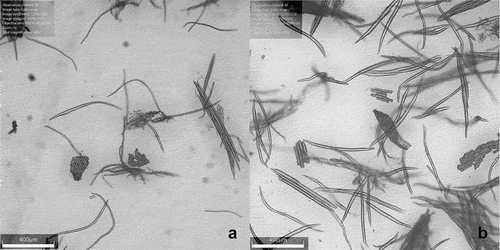
Extractive analysis was conducted by GC-MS to resolve the compounds typical for hulls. Compounds with a match over 80% toward any of the MS libraries used were considered as true (Khakimov, Jespersen, and Engelsen Citation2014). The composition of the hexane extracts of oat and barley hulls is shown in , and the composition of the acetone extracts in
Table 3. Content of selected identified components of oat and barley hulls in hexane extracts, analyzed after silylation by GC-MS. Heneicosanoic acid was used as an internal standard.
Table 4. Content of selected identified components of oat and barley hulls in acetone extracts, analyzed after silylation by GC-MS. Heneicosanoic acid was used as an internal standard.
Hexane extracts of the oat and barley hulls contained mainly oleic, linoleic and palmitic acids (); however, the amount of free fatty acids was small, less than 0.016% of dry weight. Of the fatty acids, 18:1 was dominating in oat hulls, but 18:2 was dominating in barley hulls. Oat hulls contained both cis and trans isomers of 18:1 and 18:2 fatty acids. In contrast, barley hulls had only cis isomers. Only in oats, various derivatives of C14-C26 fatty acids were also identified, including hydroxy acids, aldehydes and alcohols. About 80% of oat hull sterols and 70% of those in barley hulls were identified as β-sitosterol.
By acetone extraction, the fatty acid content of both oat and barley hulls was almost same (), but their fatty acid composition differed. The acetone extract of barley hulls contained 4.7 times more sugars and sugar alcohols than the extract of oat hulls. Of disaccharides, sucrose was the most abundant in both species. Organic acids and phenolic compounds were found in the acetone extracts as well ().
Methanolysis followed by GC-FID was used to analyze the non-cellulosic sugars in oat and barley hulls. The cell wall sugar composition did not differ significantly between the species studied (). The oat hulls had a smaller amount of hemicelluloses than barley hulls (414 and 505 mg/g dry weight, respectively). The hemicellulosic sugars were similar in both species, with the predominance of xylose and glucose (). There were slightly less arabinose and mannose in the oat hulls than in those of barley.
Table 5. Cell wall carbohydrate composition.
The total grain hull lignin and the lignin subunit composition were analyzed with the Klason method and pyrolysis-GC-MS, respectively. The quantity and subunit composition of lignin were observed to be similar in both hulls (). The oat and barley hulls contained similar amounts of lignin (23 and 25% per dry weight, respectively). Guaiacyl (G) units derived from coniferyl alcohol dominated, with p-hydroxyphenyl (H) and syringyl units (S) originating from p-coumaryl alcohol and sinapyl alcohol, respectively, at almost similar amounts.
Table 6. The amount of Klason lignin including acid-soluble lignin in the oat and barley hulls, and the lignin subunit composition as determined by pyrolysis-GC.
The paper technical properties of the laboratory sheets produced in the present work were determined ( and Table S1). For the non-calendered sheets, the bulk increased when the hulls were added to the paper furnish (). Also, as more hulls were added, the more the bulk was increased. After refining the hull materials, they still contained some longer cells attached together as fiber bundles. The bundles created a porous network with pulp fibers, thus yielding a bulky paper. The pulp fibers and fiber bundles from hulls were compressed during the calendering process (). Calendering and compaction of the paper surface and its structure improves the surface properties, the most important of which are smoothness and gloss. The compressed sheets were less bulky and increasing the amount of hulls did not yield bulkier sheets after calendering (). Photographs of the different laboratory sheets are shown in Supplementary Figure S1.
Figure 2. Paper technical properties of non-calendered and calendered laboratory sheets. a) Bulk; b) tear strength expressed as a tear index; c) tensile strength expressed as a tensile index; d) burst strength. 100/0 = 100% softwood pulp; 90/10 oats = 90% softwood pulp and 10% oat hulls; 90/10 barley = 90% softwood pulp and 10% barley hulls; 80/20 oats = 80% softwood pulp and 20% oat hulls; 80/20 barley = 80% softwood pulp and 20% barley hulls. Dotted line is the reference paper, CP = commercial paper with similar grammage (Mondi Group Citation2021).
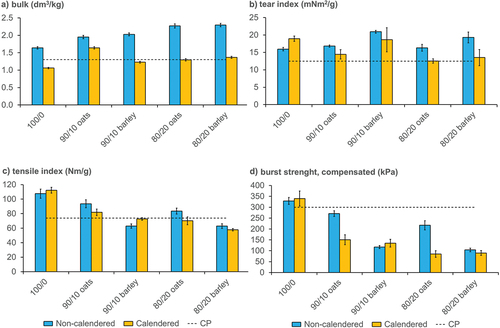
Figure 3. A non-calendered laboratory sheet (a) and a calendered laboratory sheet (b) containing 20% oat hulls. The height difference is expressed in µm. Oat hull bundle is a bundle of fibers which remained attached to each other after refining.
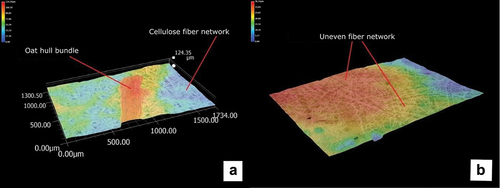
Tear index of the non-calendered sheets increased when the hulls were added to the paper furnish (). Tear index is linked to fiber length and fiber width (Seth and Page Citation1988). The hull bundles acted as long, thick fibers increasing the tear strength. The calendering process crushed the bundles, and most likely the pulp fibers were damaged next to the bundles (), leading to decreased fiber strength and tearing resistance (; Page and MacLeod Citation1992).
Tensile index was decreased when the hulls were added to the paper furnish (). Additionally, increasing the amount of hulls decreased the tensile index. Due to poor fiber-fiber bonding (short fibers and minor fibrillation), damaged pulp fibers after the calendering process, and inflexibility of the hull bundles, the burst strength was greatly reduced by addition of oat and barley hulls ().
Addition of hulls in the paper furnish increased air permeability, opacity, surface roughness and light scattering (Supplementary Table S1). On the other hand, ISO-brightness was decreased significantly due to the color of the hull material.
Mycotoxins are often found in low levels in different cereals and are controlled by food safety legislation. The oat hull batch used in the laboratory sheets in the present study contained 530 µg/kg deoxynivalenol DON and 1200 µg/kg nivalenol (NIV). Of the other trichothecene mycotoxins included in the multi-analyte method (See paragraph 1.2.), 3-acetyl-deoxinivalenol and T-2-toxin + HT-2 toxin were found only in very low levels.
Discussion
The aim of the present work was to test whether available side stream material from the food industry, barley and/or oat hulls, could be used to partially replace the conventional softwood pulp in papermaking. For this purpose, we determined the extractive and mycotoxin contents, cell wall composition and fiber lengths of these cereal hulls.
The extractive content differed to some extent between oat and barley hulls. In the hexane extracts, the most significant differences were noticed in the amounts of oleic acid and sterols (). In addition, cis,trans-isomers of C18 fatty acids were found only in oat hulls, whereas in barley hulls, fatty acids were all cis-isomers. Barley hulls contained more sterols than oat hulls. Acetone extracted efficiently many monosaccharides, disaccharides such as sucrose, and C5 and C6 sugar alcohols (). Out of the latter, xylitol was detected only in the oat hulls, whereas arabinitol was detected in the barley hulls. Our data are in accordance with those of Bach Knudsen (Citation1997), who detected soluble sugars in the hulls of oats and barley with sucrose as the most abundant sugar.
Of cell wall carbohydrates, non-cellulosic sugar contents in oat and barley hulls were at the same level (). Similar to that in barley stem (Fincher Citation2009), heteroxylans (arabinoxylans and glucuronoarabinoxylans) are the major hemicelluloses in the barley hulls (Aspinall and Ferrier, Citation1957; Krawczyk et al. Citation2008). Alike that in cereal stems (ca. 5% in barley stem; Fincher Citation2009), β-glucan (1,3;1,4-β-glucan) is reported to be a minor constituent in the hulls of both studied species (1.6 and 1.4% of dry weight in hulls of barley and oats, respectively; Bach Knudsen Citation1997). The relative abundancy of glucose detected in the present study () is probably derived mostly from starch originating from the starch endosperm due to dehulling process.
The total lignin content (Klason+acid-soluble lignin; ca. 24% of dry weight) and the subunit composition were similar in both barley and oat hulls, with G units being the most abundant, and H and S units in comparable amounts (). The lignin subunit composition in the hulls determined by pyrolysis-MS seems to differ from that in oat straw determined by nitrobenzene oxidation, where the S:G ratio was 1.64; however, the latter method releases units from non-condensed linkages only (Hatfield et al. Citation2009). The lignin content now determined with the Klason method was in the same range as determined by Krawczyk et al. (Citation2008) as acid-soluble and -insoluble lignin in oat hulls, whereas the Klason lignin contents determined by Bach Knudsen (Citation1997) for barley and oat hull meal were clearly lower (11.5% and 14.8%). In a review of Isikgor and Becer (Citation2015), lignin content in barley hulls was shown to be higher than that in straw (13.8–19.0% and 6.3–9.8%, respectively; analysis method not mentioned). The total lignin content in the barley straw was determined by the Klason method as 16–20% (Begović et al. Citation2018) and in the oat straw as 17.1% (Hatfield and Fukushima Citation2005). Different lignin analysis methods give different results; hence one should compare results determined with same method (Kärkönen et al. Citation2014). High lignin content of hulls can hinder fibrillation, for example, in mechanical pulping (e.g. Spence et al. Citation2010).
Fibers of oat and barley hulls were found to be relatively short (0.80 ± 0.19 mm and 0.51 ± 0.17, respectively). Good tensile strength, durability, low bulk, easy formability and recyclability are desired qualities for fibers (Bogoeva-Gaceva et al. Citation2007). Short and hard fibers of the hulls are not usually used in papermaking, since with only short fibers the strength of the paper is not acceptable (Glasser, Slupski, and Clark Citation1973). When combined with softwood pulp with long (ca. 2.5–3.5 mm) fibers (Barnett and Jeronimides Citation2003), on the other hand, the short fibers improve opacity, surface smoothness, absorbency, dimension stability and printability (Rantasuo Citation1976). Adding the hull fractions to the paper furnish was successful and the refined hull fractions remained in the fiber network forming the sheets.
shows that the laboratory sheets containing cereal hulls had an adequate bulk and tear strength and met the specifications for a commercial paper except for the burst strength in sheets containing either species’ hulls, and the tensile strength in sheets containing barley hulls (Mondi Group Citation2021). Nevertheless, the calendered sheets were smooth and glossy, and the sheets were visually pleasing. The refining of the hulls did not cause fibrillation, thus, bonding of the pulp fibers with the hulls was not rendered (Kang and Paulapuro Citation2006). Also, hull fiber bundles are not flexile and do not facilitate the formation of fiber–fiber bonds (Karlsson Citation2010). Hull fibers are shorter than pulp fibers yielding lower tensile strength (Seth Citation1990). However, the tensile strength of the laboratory sheets containing cereal hulls met the requirements of the commercial paper, except in the sheets containing barley hulls ()). This is most probably due to the short fibers of barley hulls (0.51 ± 0.17 mm).
Oats are more susceptible for Fusarium spp. than other cereal grain crops grown in Finland (Hietaniemi et al. Citation2016). The oat hulls were found to contain low mycotoxin levels. This observation suggests that the quality of the hulls is acceptable, and the risks related to mycotoxins in the produced paper are low, because the mycotoxin content is further diluted with the cellulose pulp. Oat and barley hulls used in this study were authentic milling by-products from the Finnish food industry. The initial quality of the crop was thus acceptable for food processing by the cereal company, which controls the quality. Such by-products are often more safe to use in food packaging materials than other by-products.
Conclusions
This study indicates that it is possible to use hull fractions of oats and barley for paper and paperboard production. The laboratory sheets mostly met the same quality requirements as the commercial paper except for the burst strength in sheets containing either species’ hulls, and the tensile strength in sheets containing barley hulls. Instead of combustion, hulls could be used in paperboard and recycled several times with pulp fibers. In the future, oat and barley products can possibly be retailed in their own packaging material.
Supplemental Material
Download JPEG Image (1.2 MB)Supplemental Material
Download MS Word (21.4 KB)Acknowledgments
We thank Päivi Viitaharju and Ulla Häggblom for the permission to use the Tampere University of Applied Sciences (TAMK) laboratory for paper making, and Arto Nikkilä for the help in the use of some equipment. Sari Rämö, Luke Jokioinen, is thanked for performing the mycotoxin analyses and interpretation of the results. The experimental work was partly conducted in a project funded by the European Social Fund (S21361).
Disclosure statement
No potential conflict of interest was reported by the author(s).
Supplementary material
Supplemental data for this article can be accessed online at https://doi.org/10.1080/15440478.2022.2091709
Additional information
Funding
References
- Aspinall, G. O., and R. J. Ferrier. 1957. 840. The constitution of barley husk hemicellulose. Journal of the Chemical Society (Resumed) Jan:4188–94. doi:10.1039/JR9570004188.
- Bach Knudsen, K. E. 1997. Carbohydrate and lignin contents of plant materials used in animal feeding. Animal Feed Science and Technology 67 (4):319–38. doi:10.1016/S0377-8401(97)00009-6.
- Barnett, J. R., and G. Jeronimides, eds. 2003. Wood quality and its biological basis. Oxford: Blackwell Publishing Ltd.
- Begović, L., I. Abičić, A. Lalić, H. Lepeduš, V. Cesar, and D. Leljak-Levanić. 2018. Lignin synthesis and accumulation in barley cultivars differing in their resistance to lodging. Plant Physiology and Biochemistry 133:142–48. doi:10.1016/j.plaphy.2018.10.036.
- Bogoeva-Gaceva, G., M. Avella, M. Malinconico, A. Buzarovska, A. Grozdanov, G. Gentile, and M. E. Errico. 2007. Natural fiber eco-composites. Polymer Composites 28 (1):98–107. doi:10.1002/pc.20270.
- Crosbie, G. B., A. W. Tarr, P. A. Portmann, and J. B. Rowe. 1984. Variation in hull composition and digestibility among oat genotypes 1. Crop Science 25 (4):678–80. doi:10.2135/cropsci1985.0011183X002500040023x.
- Du, L., and P. Yu. 2011. Relationship of physicochemical characteristics and hydrolyzed hydroxycinnamic acid profile of barley varieties and nutrient availability in ruminants. Journal of Cereal Science 53 (2):178–87. doi:10.1016/j.jcs.2010.11.006.
- Emmons, C. L., and D. M. Peterson. 1999. Antioxidant activity and phenolic contents of oat groats and hulls. Cereal Chemistry 76 (6):902–06. doi:10.1094/CCHEM.1999.76.6.902.
- FAO. 2019. Crops and livestock products FAOSTAT Retrieved 25 1 2021 . https://www.fao.org/faostat/en/#data/QC/visualize database search keywords: world total + production + crops primary + oats + barley .
- Fincher, G. B. 2009. Revolutionary times in our understanding of cell wall biosynthesis and remodeling in the grasses. Plant Physiology 149 (1):27–37. doi:10.1104/pp.108.130096.
- Franklin, G. L. 1945. Preparation of thin sections of synthetic resins and wood-resin composites, and a new macerating method for wood. Nature 155 (3924):51. doi:10.1038/155051a0.
- Gangopadhyay, N., M. B. Hossain, D. K. Rai, and N. P. Brunton. 2015. A review of extraction and analysis of bioactives in oat and barley and scope for use of novel food processing technologies. Molecules 20 (6):10884–909. doi:10.3390/molecules200610884.
- Ganssmann, W., and K. Vorwerck. 1995. Oat milling, processing and storage. In The oat crop, ed. R. W. Welch, Dordrecht: Springer 369–408 . doi:10.1007/978-94-011-0015-1_12.
- Glasser, W. G., R. H. Slupski, and J. P. Clark. 1973. Pulp-and paper-making potential of peanut hull waste in blends with softwood pulp. Wood and Fiber Science 5 (2):98–104. https://wfs.swst.org/index.php/wfs/article/view/2094.
- Group, M. 2021. Technical data sheet: advantage MG white high gloss. Accessed October 29, 2021. https://www.mondigroup.com/media/13278/advantage-mg-white-high-gloss.pdf
- Hatfield, R., and R. S. Fukushima. 2005. Can lignin be accurately measured? Crop Science 45 (3):832–39. doi:10.2135/cropsci2004.0238.
- Hatfield, R. D., J. M. Marita, K. Frost, J. Grabber, J. Ralph, F. Lu, and H. Kim. 2009. Grass lignin acylation: P-coumaroyl transferase activity and cell wall characteristics of C3 and C4 grasses. Planta 229 (6):1253–67. doi:10.1007/s00425-009-0900-z.
- Hietaniemi, V., S. Rämö, T. Yli-Mattila, M. Jestoi, S. Peltonen, M. Kartio, E. Sieviläinen, T. Koivisto, and P. Parikka. 2016. Updated survey of Fusarium species and toxins in finnish cereal grains. Food Additives & Contaminants: Part A 33 (5):831–48. doi:10.1080/19440049.2016.1162112.
- Isikgor, F. H., and C. Becer. 2015. Lignocellulosic biomass: A sustainable platform for the production of bio-based chemicals and polymers. Polymer Chemistry 6 (25):4497–559. doi:10.1039/c5py00263j.
- Kang, T., and H. Paulapuro. 2006. Effect of external fibrillation on paper strength. Pulp & Paper Canada 107 (7/8):51–54.
- Karlsson, H. 2010. “Strenght properties of paper produced from softwood kraft pulp – Pulp mixture, reinforcement and sheet stratification.” PhD diss., Karlstad University, Sweden. https://www.diva-portal.org/smash/get/diva2:317178/FULLTEXT01.pdf
- Khakimov, B., B. M. Jespersen, and S. B. Engelsen. 2014. Comprehensive and comparative metabolomic profiling of wheat,barley,oat and rye using gas chromatography-mass spectrometry and advanced chemometrics. Foods 3 (4):569–85. doi:10.3390/foods3040569.
- Korkalo, P., R. Korpinen, E. Beuger, T. Sarjala, J. Hellström, J. Kaseva, U. Lassi, and T. Jyske. 2020. Clonal variation in the bark chemical properties of hybrid aspen: Potential for added value chemicals. Molecules 25 (19):4403. doi:10.3390/molecules25194403.
- Krawczyk, H., T. Persson, A. Andersson, and A. S. Jönsson. 2008. Isolation of hemicelluloses from barley husks. Food and Bioproducts Processing 86 (1):31–36. doi:10.1016/j.fbp.2007.10.018.
- Kärkönen, A., T. Tapanila, T. Laakso, M. Seppänen, M. Isolahti, M. Hyrkäs, P. Virkajärvi, and P. Saranpää. 2014. Effect of lignin content and subunit composition on digestibility in clones of timothy (Phleum pratense L.). Journal of Agricultural and Food Chemistry 62 (26):6091–99. doi:10.1021/jf5016494.
- Mahesh, S., P. Kumar, and S. A. Ansari. 2015. A rapid and economical method for the maceration of wood fibers in Boswellia serrata roxb. Tropical Plant Research 2 (2):108–11. https://www.tropicalplantresearch.com/archives/2015/vol2issue2/7.pdf.
- Olkku, J., E. Kotaviita, M. Salmenkallio-Marttila, H. Sweins, and S. Home. 2005. Connection between structure and quality of barley husk. Journal of the American Society of Brewing Chemists 63 (1):17–22. doi:10.1094/ASBCJ-63-0017.
- Page, D. H., and J. M. MacLeod. 1992. Fiber strength and its impact on tear strength. Tappi Journal 75 (1):172–74.
- Raitanen, J.-E., E. Järvenpää, R. Korpinen, S. Mäkinen, J. Hellström, P. Kilpeläinen, J. Liimatainen, A. Ora, T. Tupasela, and T. Jyske. 2020. Tannins of conifer bark as nordic Piquancy—Sustainable preservative and aroma? Molecules 25 (3):567. doi:10.3390/molecules25030567.
- Rantasuo, P. 1976. Framställning av sågspånscellulosa med Enso-Bauer M&D-kokare [The production of sawdust kraft pulp with Enso-Bauer M&D-digester]. Norsk Skogindustri 30 (6):186–88.
- Saake, B., N. Erasmy, E. S. Th. Kruse, and J. Puls. 2004. Isolation and characterization of arabinoxylan from oat spelts. In Hemicellulose: Science and technology, ACS symposium series 864, ed., P. Gatenholm and M. Tenkanen, 52–65. Washington: American Chemical Society. doi:10.1021/bk-2004-0864.ch004.
- Salo, M.-L., and K. Kotilainen. 1970. On the carbohydrate composition and nutritive value of some cereals. Agricultural and Food Science 42 (2):21–29. doi:10.23986/afsci.71752.
- Seth, R. S., and D. H. Page. 1988 February. Fiber properties and tearing resistance. Tappi Journal 71:103–07.
- Seth, R. S. 1990. Fibre quality factors in papermaking–II. The importance of fibre coarseness. In MRS Symposium Proceedings Vol. 197, 143–61. Pittsburgh, PA: Materials Research Society.
- Spence, K. L., R. A. Venditti, Y. Habibi, O. J. Rojas, and J. J. Pawlak. 2010. The effect of chemical composition on microfibrillar cellulose films from wood pulps: Mechanical processing and physical properties. Bioresource Technology 101 (15):5961–68. doi:10.1016/j.biortech.2010.02.104.
- Thompson, B. K., A. F. Mustafa, J. J. McKinnon, D. Maenz, and B. Rossnagel. 2000. Genotypic differences in chemical composition and ruminal degradability of oat hulls. Canadian Journal of Animal Science 80 (2):377–80. doi:10.4141/A99-132.
- Viljamaa, S., E. Dikareva, J. Tolonen, J. Edesi, K. Nickolov, T. Laitinen, T. Laakso, R. Korpinen, P. Saranpää, S. Jokipii‑Lukkari, et al. 2018. Cryopreservation of the Norway spruce tissue culture line able to produce extracellular lignin. Plant Cell, Tissue and Organ Culture 133 (2):225–35. doi:10.1007/s11240-017-1375-4.
- Welch, R. W. 2005. Cereal grains. In Encyclopedia of Human Nutrition, ed. Caballero, B., l. Allen, and A. Prentice, 346–356. 2nd ed. New York: Academic Press.