ABSTRACT
Banana pseudostems are now recognized as a sustainable raw material with a wide range of applications. Plenty of research attention has been paid to banana pseudostem fiber extraction, characterization, modification, and utilization. Mechanical extraction takes precedence over manual extraction. Surface treatments are employed to modify the surface of a fiber to make it suitable for customized applications. Because of its high cellulose content, good to moderate strength, fineness, fiber length-to-breath ratio, and other properties, banana pseudostem fiber is currently used to make nano and microcrystalline cellulose, activated carbon, green composites, and technical textiles. This review article discusses the manual and mechanical extraction processes of banana fiber, as well as its quality evaluation, morphology, chemical composition, physico-mechanical properties, and various surface modification techniques.
摘要
香蕉假茎现在被公认为具有广泛应用的可持续原料. 香蕉假茎纤维的提取、表征、改性和利用已引起人们的广泛关注. 机械提取优先于手动提取. 表面处理用于修饰纤维表面,使其适合定制应用. 由于其纤维素含量高、强度、细度、纤维长度与呼吸比以及其他特性,香蕉假茎纤维目前被用于制造纳米和微晶纤维素、活性炭、绿色复合材料和工业纺织品. 本文综述了香蕉纤维的人工和机械提取工艺,以及香蕉纤维的质量评价、形态、化学成分、物理机械性能和各种表面改性技术.
Introduction
The modern scientific world is moving toward a sustainable research environments for the protection of the ecosystem. Different efforts are going on one of them is the utilization of natural fiber in different ways by extraction from the direct nature so that it could be biodegradable material for preservation of the environment (Khan et al. Citation2021a, Citation2022; Kumar et al. Citation2022). All natural fibers are lower in health risks, environmentally safe, recyclable, and nonabrasive for quick processing. Natural fibers have many advantages over synthetic fibers, including wide availability, significantly reduced density, low cost, outstanding thermal insulation, significant endurance, excellent electrical resistance, improved acoustic qualities, and high specific strength (Khan et al. Citation2021b, Citation2022; Premalatha et al. Citation2021; Sumesh et al. Citation2022).
Banana has seven recorded wild species viz., Musa. lolodensis, M. maclayi, M. peekelii, M. jackeyii, M. textilis, and M. bukensis, while M. fehi is the cultivated species in many counties like Thailand, India, Indonesia, Malaysia, Philippines, Hawaii, and Pacific islands (Uma, Saraswathi, and Durai Citation2019). India is the largest producer of banana fruit. Approximately 31.5% of the total banana plants in the world is grown in India, followed by China (11.5%), Indonesia (8.2%), Brazil (6.6%), Ecuador (6.2%), Philippines (6%), Guatemala (4.4%), Angoa (4.1%), Tanzania (3.4%), Costa Rica (2.5%), and other tropical countries (15.6%) (Evans, Ballen, and Siddiq Citation2020). This tropical crop is grown all year long in an environment with an average annual rainfall of 650–750 mm, temperatures ranging from 15 to 35°C, and relative humidity levels between 75 and 85%.
After harvesting, the pseudostems are generally discarded as waste or burnt after drying, thus causes substantial pollution. In a few places, it is used as a biofertilizer. One hectare of banana farm is estimated to contribute approximately 220 tons of biomass as waste containing pseudostems, peduncles, bulbs, leaf sheaths, and reaches (Ahmad and Danish Citation2018). A fully matured banana pseudostem has 20–25 layers of flesh sheaths. The fibers can be extracted either manually or using a decorticating machine. The extracted fibers may further be subjected to water retting for degumming to enhance the fiber quality. The fibers extracted from banana pseudostem are lignocellulosic in nature, having a good length/fineness ratio, and strength and thus, considered as a textile grade fiber. Banana pseudostem fiber is coarse, resembles jute and kenaf fibers in many of its properties, and is capable of a potential replacement for above said fibers in certain applications. The unmodified and modified banana pseudostem fiber could find applications in nano and micro cellulose, technical textiles, adsorbents, paper and pulp, sanitary napkins, and composites (Benedetto, Gelfuso, and Thomazini Citation2015).
Unlike fiber crops viz., jute, ramie, and kenaf, banana is cultivated not for fiber but for fruit. Thus, the primary focus of the research was given on the banana plant. Though there are many research publications are available in banana pseudostem fiber, very few of the technologies are commercialized. The unavailability of advanced machineries for fiber extraction, lack of a steady supply of the fiber in the market, deficiency of standard machineries for fiber processing, and product development is still constrained. The fiber properties majorly depend on the plant variety, soil used for cultivation, agro-climatic conditions, cultivation treatments, method of extraction, and post-harvest treatments. This review article reports different extraction process of banana pseudostem fiber, its quality evaluation, physical-mechanical properties, and surface modification. In addition, variegated morphological and structural analysis of banana fiber is also reported.
Banana fiber extraction
Manual and mechanical extraction
One of the critical challenges in banana fiber processing is its extraction. Since banana fiber is not a well-established fiber for apparel textiles, the advanced fiber extraction machineries are not developed yet. Various attempts (Basak et al. Citation2016; Jayaprabha, Brahmakumar, and Manilal Citation2011; Paramasivam et al. Citation2020) have been performed to develop different types of extractors; however, very few are commercially available. The primitive and simple method of extraction is manual extraction done by direct extraction which is carried out using sharp objects like a blade or broken crockery. It is common in villages and rural areas. This technique produces good quality fiber, without much debris on the fiber surface; however, it is laborious and production is very low (). Thus, it cannot be adopted for large-scale production (Gañán et al. Citation2004). In general, manual extraction produces superior quality of fiber than machine extraction, since mechanical extraction may cause severe damage to the fibers due to high-speed mechanical beating. The key elements contributing to the mechanical qualities of manually extracted fibers are high cellulose content and low microfibrillar angle, which are not disturbed during manual extraction (Jagadeesh et al. Citation2021).
Decorticator is a general-purpose machine used for the extraction of lengthy natural fibers. It has already been reported for the extraction of many bast and leaf fibers like ramie (Lü et al. Citation2013), PALF (Jose, Salim, and Ammayappan Citation2016), and banana (Basak et al. Citation2016). Though the principle of mechanism is the same for all decorticating machines, it may slightly differ in design according to the manufactures and the type of fiber to be extracted (Jayaprabha, Brahmakumar, and Manilal Citation2011; Paramasivam et al. Citation2020). For the fiber extraction, after harvesting the crop, the sheaths of the pseudostems are separated manually. The machine operator holds one end of the long sheaths of pseudostem and inserts it inside the machine. The machine consists of a high-speed rotating drum. Due to high-speed mechanical beating action, the debris associated with the pseudostem is removed and the fibers are getting separated (). The separated fibers are manually combed using a metallic comb to remove the remnants of the pseudostem remains, if any. Finally, the fibers are washed and dried. While inserting the long sheaths inside the decorticator, a major portion of the pseudostem is converted into long fiber (). In addition to this, the decortication also produces short fiber (), pith, and sap. Although the quality of the fiber is marginally inferior to the manual method, it is well accepted in terms of its high production capacity. Since dried sheaths cannot be processed by a machine, decortication must be done as soon as the crop is harvested. One pseudostem sheet can be processed at a time because the majority of decorticators are made for batch processing. However, in some more modern versions, the decorticator uses a conveyor belt rather than hand feeding (Paramasivam et al. Citation2020). The majority of decorators are now portable. displays a comparison of the quality of banana fiber obtained using various extraction techniques.
Table 1. Physical and mechanical properties of banana fiber.
Retting
Retting is the simplest method of extraction of natural fiber. Yet, it cannot be adopted in all kinds of fibers. Water retting is the most common retting technique; However, dew retting, chemical retting, fungal retting, and bacterial retting are also common. Retting facilitates the extraction of fiber by removing the cementitious materials such as pectins matter, hemicellulose, wax, and some lignin (Chanakya and Sreesha Citation2012; Hazarika et al. Citation2017).
In direct water retting, after harvesting the fruit, the pseudostems are individualized and directly immersed in water. It will take a long time for biological degradation to occur and for the fibers to be extracted. This retted fibers again need further cleaning to remove the attached debris from the pseudostem if present any. This process is time-consuming and not eco-friendly, as it will contaminate the water resources, thus it is not a recommended method for fiber extraction. The water retention requirement is highly dependent on the quality of the fiber provided by the decorticator. After decortications and subsequent combing, if the banana fiber consists of fleshy remnants of the pseudostem, the fiber may be subjected to water retting to enhance the fiber quality. Although water retting is a slow degradation process by microorganisms, extreme care should be taken up to avoid over retting of banana fiber, which may otherwise result in degradation of cellulose and ultimately reduce the strength and luster of the fiber. The retting time, temperature, pH of water, and micro-nutrients are having a substantial effect on the quality of banana fiber (Subagyo and Chafidz Citation2018).
Chemical degumming
While facing difficulties for getting individual fibers after decortication or retting, the fiber extraction may be performed using chemicals, and the processing is known as degumming. Since the binding materials (lignin, hemicellulose, fats, and waxes) are acidic, generally mild alkali like soap or sodium carbonate are used for degumming and the process is performed at boiling for 30–60 min (Pandey et al. Citation2021). If extensive removal is required, then sodium hydroxide can be used (Badrinath and Senthilvelan Citation2014). Chemicals like magnesium carbonate, calcium hydroxide, sodium bicarbonate were found to be effective in removing hemicellulose, lignin, and other impurities present on the fiber surface (Siva et al. Citation2019, Citation2020, Citation2020). The selection of degumming chemicals and treatment conditions are determined by the nature and amount of gum present on the fiber extent of the individualization of fiber entities. A combination of hydrogen peroxide and sodium hydroxide may provide better whiteness to the fiber in addition to fiber separation. A mild acidic treatment is preferred after alkali degumming to remove the excess alkali content present if any. This process may either be performed in the open vessel or in an autoclave. The high-temperature high-pressure process (HTHP) in autoclave results in the partial breakdown of the lignocellulose structure, hemicellulose fraction hydrolysis, lignin de-polymerization, and defibrillation and produces better quality fiber. Proper precautions are required to avoid degradation of fiber during chemical degumming.
Microbial retting
Microbial degradation is a well-established method used for the extraction of natural fibers. It may be either aerobic or anaerobic, however; the latter is much preferred. Selected bacteria and funguses (Pseudomonas, Azotobacter, Clostridium, Bacillus, Clostridiaceae, etc.) at favorable environment can attack the lignocellulosic biomass partially and they may be employed for the extraction of fiber. The mechanically extracted banana fibers were polished with A. niger using anaerobic digestion methods and the results were found encouraging (Sarma and Deka Citation2016). They also inferred that Aspergillus niger has effectively been used for the extraction of fiber from decorticated banana fiber. Similarly, Jayaprabha, Brahmakumar, and Manilal (Citation2011) attempted anaerobic extraction of banana fiber. They observed that 6 days of anaerobic digestion may give the fibers having almost at par physico-mechanical properties as compared to the fibers extracted by the sweaty manual method. The process is less laborious and could be adopted for bulk scale production. To overcome certain inevitable drawbacks such as breakage of some β-glucosidic linkages in cellulose and hemicelluloses during mechanical extraction and brown coloration of fiber during retting, alternative anaerobic digestion in a controlled reactor for the extraction of banana fiber is also reported (Chanakya and Sreesha Citation2012).
Enzymatic extraction
The action of enzymes is biochemical specific and so, a single enzyme may not be useful for fiber extraction. It is not possible to remove all the cementing materials like lignin, pectin, and hemicellulose using a single enzyme from the fiber. For this, a combination of enzymes is employed. Enzymes are not much effective for the direct extraction of fiber from raw sources. Instead, it is applied for the removal of post retting residual cementing materials after preliminary extraction of fiber. Commonly used enzymes for the extraction of fiber are cellulase, pectinase, hemicellulase, and lignin peroxidase for removal of cellulose, pectinase, hemicellulose, and lignin respectively. The scope of enzyme treatment for the fiber extraction from banana fiber as well as fiber surface modification has not yet been explored systematically. Jacob et al. (Citation2008) prepared a pectinase enzyme applied upon banana pseudostem fiber. Pectinase helps to rupture the middle lamella resulting in fiber separation and increases the smoothness of the fiber due to the removal of debris on fiber surface.
Physico-mechanical properties of banana fiber
The physico-mechanical properties of the banana fiber greatly depend on the method of extraction. The literature () gives information about the fiber properties. Length and fineness of the fiber depend preliminary on the variety of plant, sheath length, as well as the method of extraction. It is also observed that hand extraction produces fine fibers as compared to mechanical extraction (Vigneswaran et al. Citation2015). In many cases, after mechanical extraction, multiple entities are found to be adhered to themselves. The bundle may be opened by mechanical combing. The quality of the combed fiber is further enhanced through better fiber separation and removal of surface debris. indicates that the length and diameter of the fiber differ considerably. The variation may be due to the differences in the length of the sheath taken by individual researchers as well as the method of extraction employed. The gauge length and the amount of defects in the fiber are the main determinants of the mechanical properties. Additionally, the strength of the fibers diminishes as the microfibrillar angle raises and the cellulose content falls, while it increases with the number of cells that supply strength (Kulkarni et al. Citation1983; Mukherjee and Satyanarayana Citation1984).
A comparative description of the physico-mechanical properties of banana fiber with other lignocellulosic fibers is shown in . It is depicted that the banana fibers resemble roselle fibers in many aspects like finesses, bundle strength, and moisture content. It is inferior to other popular fibers like flax and sisal. The strength of banana fiber is lower in comparison with jute. Fineness of banana fiber ranges between 3 and 7 tex. The difference may be due to the extent of defibrillation depending on different degumming methods adopted. It can be concluded from the physico-mechanical properties that banana fiber can be a potential alternative for the above-said fibers for many applications. Contrary to coir fibers, the structure of banana fibers exhibits an increase in cell number in fibers up to a diameter of 100/m before remaining constant. As a result, so does the strength. There may not be any discernible variations in the mechanical properties with fiber diameter as a result of the lack of considerable variation in the microfibrillar angle and the fraction of cells that renders strength in banana fibers (Geethamma, Joseph, and Thomas Citation1995; Kulkarni et al. Citation1983).
Table 2. Comparison of physico-mechanical properties of banana fiber with other lignocellulosic fibers.
Chemical composition
shows the chemical composition analysis of banana fiber reported by different researchers. The major components of the fiber are cellulose (55–65%), hemicellulose (15–25%), lignin (10–15%), and pectin (3–5%). Cellulose being the main constituent of banana fiber provides structural integrity to the fiber. Lignin also plays a crucial role in the plant’s mechanical strength (Jústiz-Smith, Virgo, and Buchanan Citation2008). The yellowish color of the banana fiber is chiefly due to its lignin content. The chemical composition of banana fiber can differ depending on its species, geographical locations, agro-climatic conditions, soil nutrients, plant age, and conditions of extraction. Other bast fibers such as jute, flax and roselle have higher cellulose and hemicellulose content compared to banana. It may be noted that the lignin content of banana fiber is considerably lower than that of jute and sisal. The presence of trace elements such as sodium, calcium, magnesium, aluminum, and silicon in the fiber may increase the brittleness of the fiber (Jústiz-Smith, Virgo, and Buchanan Citation2008).
Table 3. Comparison of the chemical composition of banana fiber with other lignocellulosic fibers.
Microscopic analysis
The longitudinal and cross-section morphology of the banana fiber has been extensively studied using various microscopic methods. The topography of the fiber greatly depends on the method of extraction. Generally, the surface of the raw fiber seems to be slightly rough () and may contain certain amounts of debris after manual or mechanical extraction (Jannah et al. Citation2009). Among various extraction methods, the chemical degumming produces fibers with a smooth surface () (Kalita et al. Citation2018). The alkali removes the pectins, hemicelluloses, and a certain amount of lignin from the fiber and ultimately results in a smooth surface. In the cross-section, the banana fiber seems to be irregular and non-spherical in shape, whereas the cell wall is thick and the central lumen is elliptical, narrow, and elongated (Guimarães et al. Citation2009). The fiber is multiluminar, as revealed from a high number of collapsed dry lumens.
Figure 3. SEM images of banana fiber (a) raw surface (b) degummed surface (c) fiber cross-section (Guimarães et al. Citation2009).
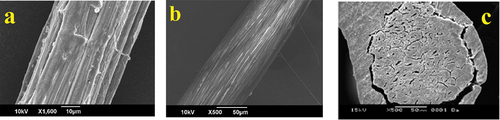
Neelamana, Thomas, and Parameswaranpillai (Citation2013) synthesized macro, micro, and nano fibers through steam explosion. The AFM topography () shows the macro, micro, and nano fibers. Multifibrillar structure is more apparent from AFM analysis. The average nano fiber diameter was estimated at about 30 nm. The middle lamella and primary cell walls are removed more efficiently to form micro and nano fibers. As a result, a more cellulose-rich surface and an effective reduction of the fiber dimension to the nano range have been achieved. Chokshi et al. (Citation2022) reported that the primary factor influencing the inherent fiber strength characteristics is the microfibrillar angle. A higher cellulosic percentage and a lower MFA are reportedly necessary for high fiber strength. The lower microfibrillar angle (11°) and comparatively high percentage of crystallinity [08Muk] in banana fibers should be the primary causes of their low elongation (Gassan, Chate, and Bledzki Citation2001).
Figure 4. AFM topography of (a) macro, (b) micro and, (c) nano fibers derived from banana pseudo stem (Neelamana, Thomas, and Parameswaranpillai Citation2013).
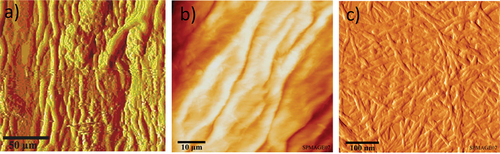
Spectroscopy
X-ray diffractometry
Being lignocellulosic in nature, banana fiber consists of both amorphous and ordered (crystalline) regions. The XRD spectra of raw banana fiber is presented in . The spectra showed a sharp peak at 22.0°Corresponding to the ordered/crystalline region. Though there is a minor difference in the crystallinity of the banana fiber as observed in the reported studies, it is widely accepted as 62–65% crystalline and 35–40% amorphous (Guimarães et al. Citation2009; Madhushani et al. Citation2021) which is close to jute (58:42) (Ghosh, Samanta, and Basu Citation2004), but lower than ramie (63:37) (Song et al. Citation2019), and cotton (71:29) (Liu et al. Citation2012). Kiruthika and Veluraja (Citation2009) observed from parallel fiber x-ray diffractometry that crystalline orientation and crystallite size differs within varieties. It indicates that identification of variety(ies) is essentially needed for selective uses of fiber. Chemical treatments like alkaline degumming and bleaching may enhance the crystallinity of the fiber by the removal of non-crystalline components like pectins, hemicelluloses, and lignin (Basu et al. Citation2015; Taer et al. Citation2021). It is also reported that the nature of chemicals for the surface treatment has a significant role in determining the crystallinity of the banana fiber (Bar et al. Citation2021).
Fourier transform infrared spectroscopy
The functional groups present in the banana fiber may be interpreted using FTIR analysis. shows the FTIR analysis of banana fiber. The spectra showed a wide band near the 3335 cm−1 region due to the presence of the – OH group of absorbed water (Pandey, Jose, and Sinha Citation2020; Rodríguez, Álvarez-láinez, and Orrego Citation2022). A small band at 2900 cm−1 shows the presence of C-H stretching in alkanes (Pandey et al. Citation2021). The conjugated >CO stretching of ester and aldehyde groups in hemicelluloses and lignin is observed around 1740 cm−1 (Basu et al. Citation2015). A slightly wider band present near 1650 cm−1 is the indication of C = O stretching vibration in conjugated carbonyl of lignin (Jose et al. Citation2019). A small bust sharp band at 1314 cm−1 indicates C=C aromatic symmetrical stretching of lignin. The band at 832 cm−1 is attributed to the C – H out-of-plane deformation of lignin in banana fiber (Kalita et al. Citation2019). The peak at 1160 cm−1 attributes to the C-O-C asymmetric stretching in cellulose I and cellulose II (Singh et al. Citation2020). The strong and sharp band present at 1050 cm−1 is the indication of C-O stretching of hemicelluloses and lignin (Jose et al. Citation2017). A broad region of overlapping bands of absorption (2000–1400 cm−1) due to aromatic linkages of C – C, C=C, OH, CO, C – O–C, C – H. (Bar et al. Citation2021; Rahman et al. Citation2022).
Fiber modification techniques
Most natural fibers are inherently hydrophilic in nature due to the existence of the – OH group. The hydrophilic nature of these fibers enables better moisture management properties. However, in certain applications, the connatural hydrophilic nature of the natural fiber is not desired and some hydrophobic properties are required. In this context, meticulous research attempts have been made to impart hydrophobicity to the fibers. Physical methods viz., plasma treatment, gamma, and UV radiation, and chemical methods viz., grafting, enzyme treatment, polymer coating are commonly employed for surface modification. The studies on the physical and chemical fiber modification of banana fiber are described below.
Plasma treatment
When a suitable gas like argon, nitrogen, or oxygen is exposed to the electromagnetic field, plasma is generated. The surface modification largely depends on the nature of the gas used for plasma treatment and it may induce hydrophilicity or hydrophobicity on the fiber surface. This process opens up a scope of eco-friendly surface modification of natural fibers with a short duration of time. Depending on the type and nature of the gases utilized, plasma treatment may be used to create a wide range of surface modification by successively introducing surface crosslinking (Gupta et al. Citation2021, Citation2022). Whilst this process is effective, however, the plasma-treated fiber gradually loses its acquired surface characteristics after keeping for a prolonged time.
Dielectric Barrier Discharge (DBD) assisted plasma treatment was used for the modification of banana fiber. The treatment improved the elastic modulus, tensile strength, surface roughness, and hydrophilicity of the fiber (Oliveira et al. Citation2012). Hrabě, Müller, and Mizera (Citation2016) modified the fiber with surface plasma treatment. The samples were evaluated immediately for tensile strength soon after treatment and found to be greater as compared to the untreated ones. Vajpayee et al. (Citation2020) modified the surface of the banana fabric with DBD air plasma after coating it with natural leaf extracts. The findings inferred that the treatment improved the hydrophilicity of the fiber due to the enhancement of the polar functional groups. The treated fabric exhibits great tolerance to gram + ve and – ve bacteria.
UV-radiation treatment
Radiation treatments are other important tools for surface modification of natural fibers. The UV rays are having low energy to that of gamma and X-rays. Thus, they cannot diffuse inside the fiber and the interaction remains on the surface only. Very little information is available on the radiation treatments on banana fiber. Zaman, Khan, and Khan (Citation2011) reported the chemical modification of banana fiber under UV radiation for the development of banana/LDPE composites. The results showed improved mechanical properties for modified fiber composites compared to untreated composites. In another attempt, the same group studied the effect of UV radiation on Polypropylene (PP) composite banana fibers. They reported improved mechanical properties of UV irradiated banana fiber and PP composites compared to untreated counterparts. It is also reported that UV radiation treatment improves the tensile strength and elastic modulus of banana fibers (Benedetto, Gelfuso, and Thomazini Citation2015)
Chemical modification of banana fiber
Chemical modification is a well-established and simple method of modification since it does not need many sophisticated instruments and therefore cost-effective. In most of the chemical modifications of banana fiber, the – OH functional groups of the cellulose are coupled with external chemicals, thus blocks the active reaction site. The common chemical methods of treatments are shown below ().
Alkali treatment
Alkali treatment is the most common and wildly adopted chemical treatment. Alkali treatment may be performed either to remove the fiber impurities to make the fiber clean and smooth or to augment the functional performance. Generally, in the first case, mild alkali like soap or sodium carbonate is used, whereas, in the second case, caustic soda is used. Since, non-cellulosic materials viz., hemicelluloses, lignin (partially), pectins are soluble in hot alkaline condition, the method enhances the crystallinity of the fiber. The NaOH treatment at high temperature enhances the whiteness of the fiber by the removal of lignin, and also increases the dye uptake due to the formation of soda cellulose. Benítez et al. (Citation2013) reported that treatment of banana fiber with hot alkali removes the surface impurities and enhances the mechanical strength of the fiber. The alkali treatment also enhances the thermal stability, thermal conductivity, and diffusivity of the fiber (Paul et al. Citation2008; Twebaze et al. Citation2022).
Silane treatment
Silane coupling is generally performed to replace the – OH group of natural fiber with the organosiloxy group, as a result, the fiber becomes hydrophobic. The most common silane coupling agents are tetraethyl orthosilicate (TEOS), 3-amino propyltriethoxysilane, and vinyl triethoxysilane. In general, the coupling agents hydrolyze to silanol and form an effective ester bond/linkage with carboxylic groups of fiber. Apart from this, there might be chances of the formation of other bonds like hydrogen or ester linkage, which highly dependent upon the availability of functional groups such as -NH2 or -OH. The silane treatment increases the surface energy and lowers the surface wettability. Silane modifications are mainly performed on banana fibers before the preparation of composites. It is reported that the treatment of banana fiber with 3-amino propyltriethoxysilan and vinyl triethoxysilane enhances the hydrophobicity of fiber (Jandas et al. Citation2011; Kannan and Thangaraju Citation2022; Pothan, George, and Thomas Citation2002).
Acetylation
An alternative method of imparting hydrophobicity to natural fiber is grafting with acetic anhydride and the process is known as acetylation. In this process, the – OH group of the fiber is replaced with the acetyl group. This process improves the hydrophobicity of banana fiber. Although the acetylation of some natural fibers has been reported, attempts on the banana fiber are scanty. Teli and Valia (Citation2013) used acetic anhydride and N-Bromosuccinimide (NBS) for solvent-free acetylation on banana fiber to improve its oil absorbency. Acetylation on banana fiber contributed to a notable increase in acetyl groups. Therefore, the modified fiber was found to have better hydrophobicity and thermal stability. The acetylated banana fiber may have better adhesion with synthetic resins for composite preparation and it can also find application in oil spilled water bodies.
Enzyme treatment
Enzymes are biological molecules and are having specific activity. In the textile industry enzymes are employed for various stages of wet processing viz., desizing, scouring, bio finishing, etc. Pectinase, cellulase, laccase, transglutaminase, protease, xylanase, lipase, amylase, and peroxidase are the most commonly used enzymes for textiles. The main objective of enzyme treatment on natural fiber is to remove the surface impurities like fat and waxes, and in certain cases, the enzymes can be used to target lignin and cellulose. The process is eco-friendly and it ultimately results in the smooth fiber surface and enhancement of dye uptake. In a reported study, biopectinase has been employed for the surface modification of banana fiber. The prolonged duration of enzyme treatment more than 6 h was not found effective. With the removal of pectins, and hemicelluloses, the biopectinase treatment enhanced the surface smoothness and thermal stability of banana fiber; however, a marginal decrease in the mechanical properties was observed (Ortega et al. Citation2016). The treatment of banana fiber with laccase resulted in the removal of lignin, while xylanase was found to be effective in the removal of hemicellulose (Vishnu Vardhini and Murugan Citation2017). The higher concentrations of enzyme treatment may adversely affect the mechanical strength of the fabric.
Conclusion
This review article discussed the significance of banana pseudostem fiber, extraction procedures, characteristics, and various surface modification methods. Banana fiber is primarily composed of cellulose, hemicellulose, lignin, and pectin, with cellulose serving as the primary component. The banana fiber exhibits structural and physico-mechanical characteristics that are very similar to jute and roselle. The mechanical extraction through decortications method is preferred for fiber extraction than manual methods. Retting/degumming is an optional operation to improve the quality of the fiber. The fibers are often modified with chemicals or enzymes as per the requirement such as for the preparation of composites or getting better process ability. The surface treatments remove the non-cellulosic materials from the fiber as a result the fiber achieved improved properties.
Highlights
Various methods of fiber extraction from banana pseudostem are reviewed.
Various surface modification techniques of banana pseudostem fiber are discussed.
Microscopic, spectroscopic, and thermal characterization of the fiber are described.
The chemical composition and physico-mechanical properties of the fiber are enumerated.
Disclosure statement
No potential conflict of interest was reported by the author(s).
References
- Ahmad, T., and M. Danish. 2018. Prospects of banana waste utilization in wastewater treatment: A review. Journal of environmental management 206:330–15. doi:10.1016/j.jenvman.2017.10.061.
- Alavudeen, A., N. Rajini, S. Karthikeyan, M. Thiruchitrambalam, and N. Venkateshwaren.2015.Mechanical properties of banana/kenaf fiber-reinforced hybrid polyester composites: Effect of woven fabric and random orientation.Materials & Design.66:246–57.10.1016/j:matdes.
- Badrinath, R., and T. Senthilvelan. 2014. Comparative investigation on mechanical properties of banana and sisal reinforced polymer based composites. Procedia Materials Science 5:2263–72. doi:10.1016/j.mspro.2014.07.444.
- Bar, M., H. Belay, A. Ramasamy, A. Das, and P. Ouagne. 2021. Refining of banana fiber for load bearing application through emulsion treatment and its comparison with other traditional methods. Journal of Natural Fibers 19:1–18. doi:10.1080/15440478.2021.1902901.
- Basak, S., S. Saxena, S. K. Chattopadhyay, R. Narkar, and R. Mahangade. 2016. Banana pseudostem sap: A waste plant resource for making thermally stable cellulosic substrate. Journal of Industrial Textiles 46 (4):1003–23. doi:10.1177/1528083715591580.
- Basu, G., L. Mishra, S. Jose, and A. K. Samanta. 2015. Accelerated retting cum softening of coconut fibre. Industrial Crops and Products 77:66–73. doi:10.1016/j.indcrop.2015.08.012.
- Basu, G., A. N. Roy, K. K. Satapathy, S. M. J. Abbas, L. Mishra, and R. Chakraborty. 2012. Potentiality for value-added technical use of Indian sisal. Industrial Crops and Products 36 (1):33–40. doi:10.1016/j.indcrop.2011.08.001.
- Benedetto, R. M. D., M. V. Gelfuso, and D. Thomazini. 2015. Influence of UV radiation on the physical-chemical and mechanical properties of banana fiber. Materials Research 18 (2):265–72. doi:10.1590/1516-1439.371414.
- Benítez, A. N., M. D. Monzón, I. Angulo, Z. Ortega, P. M. Hernández, and M. D. Marrero. 2013. Treatment of banana fiber for use in the reinforcement of polymeric matrices. Measurement 46 (3):1065–73. doi:10.1016/j.measurement.2012.11.021.
- Bhatnagar, R., G. Gupta, and S. Yadav. 2015. A review on composition and properties of banana fibers. International Journal of Scientific & Engineering 6 (5):49–52.
- Binoj, J. S., R. E. Raj, and B. S. S. Daniel. 2017. Comprehensive characterization of industrially discarded fruit fiber, Tamarindus indica L. as a potential eco-friendly bio-reinforcement for polymer composite. Journal of Cleaner Production 142:1321–31. doi:10.1016/j.jclepro.2016.09.179.
- Biswal, M., S. Mohanty, and S. K. Nayak. 2011. Mechanical, thermal and dynamic-mechanical behavior of banana fiber reinforced polypropylene nanocomposites. Polymer Composites 32 (8):1190–201. doi:10.1002/pc.21138.
- Chanakya, H. N., and M. Sreesha. 2012. Anaerobic retting of banana and arecanut wastes in a plug flow digester for recovery of fiber, biogas and compost. Energy for Sustainable Development 16 (2):231–35. doi:10.1016/j.esd.2012.01.003.
- Chokshi, S., V. Parmar, P. Gohil, and V. Chaudhary. 2022. Chemical composition and mechanical properties of natural fibers. Journal of Natural Fibers 19 (10):3942–53. doi:10.1080/15440478.2020.1848738.
- Evans, E. A., F. H. Ballen, and M. Siddiq. 2020. Banana production, global trade, consumption trends, postharvest handling, and processing. In Handbook of banana production, postharvest science, processing technology, and nutrition, ed. G. Siddiq Jasim Ahmed and L. M. Gloria, 1–18.
- Gañán, P., J. Cruz, S. Garbizu, A. Arbelaiz, and I. Mondragon. 2004. Stem and bunch banana fibers from cultivation wastes: Effect of treatments on physico-chemical behavior. Journal of Applied Polymer Science 94 (4):1489–95. doi:10.1002/app.21061.
- Gassan, J., A. Chate, and A. K. Bledzki. 2001. Calculation of elastic properties of natural fibers. Journal of Materials Science 36 (15):3715–20. doi:10.1023/A:1017969615925.
- Geethamma, V. G., R. Joseph, and S. Thomas. 1995. Short coir fiber-reinforced natural rubber composites: Effects of fiber length, orientation, and alkali treatment. Journal of Applied Polymer Science 55 (4):583–94. doi:10.1002/app.1995.070550405.
- Ghosh, P., A. K. Samanta, and G. Basu. 2004. Effect of selective chemical treatments of jute fibre aimed at improved textile related properties and processibility. Indian Journal of Fibre and Textile Research 29 (3):85–99.
- Guimarães, J. L., E. Frollini, C. G. Da Silva, F. Wypych, and K. G. Satyanarayana. 2009. Characterization of banana, sugarcane bagasse and sponge gourd fibers of Brazil. Industrial Crops and Products 30 (3):407–15. doi:10.1016/j.indcrop.2009.07.013.
- Gupta, U. S., M. Dhamarikar, A. Dharkar, S. Chaturvedi, A. Kumrawat, N. Giri, S. Tiwari, and R. Namdeo. 2021. Plasma modification of natural fiber: A review. Materials Today: Proceedings 43:451–57. doi:10.1016/j.matpr.2020.11.973.
- Gupta, U. S., S. Tiwari, U. Sharma, and K. Viliya. 2022. Cold glow discharge nitrogen plasma pretreatment of banana fibre for improving the mechanical characterisation of banana/epoxy composites. Journal of the Institution of Engineers D:1–13. doi:10.1007/s40033-022-00356-8.
- Hazarika, D., N. Gogoi, S. Jose, R. Das, and G. Basu. 2017. Exploration of future prospects of Indian pineapple leaf, an agro waste for textile application. Journal of Cleaner Production 141:580–86. doi:10.1016/j.jclepro.2016.09.092.
- Hrabě, P., M. Müller, and Č. Mizera. 2016. The effect of plasma treatment on tensile strength of enseteventricosum fibres. Manufacturing Technology 16 (5):928–33. doi:10.21062/ujep/x.2016/a/1213-2489/MT/16/5/928.
- Huang, J., and C. Yu. 2019. Determination of cellulose, hemicellulose and lignin content using near-infrared spectroscopy in flax fiber. Textile Research Journal 89 (23–24):4875–4883. doi:10.1177/0040517519843464.
- Jacob, N., K. N. Niladevi, G. S. Anisha, and P. Prema. 2008. Hydrolysis of pectin: An enzymatic approach and its application in banana fiber processing. Microbiology Research 163 (5):538–44. doi:10.1016/j.micres.2006.07.016.
- Jagadeesh, P., M. Puttegowda, S. Mavinkere Rangappa, and S. Siengchin. 2021. A review on extraction, chemical treatment, characterization of natural fibers and its composites for potential applications. Polymer Composites 42 (12):6239–64. doi:10.1002/pc.26312.
- Jandas, P. J., S. Mohanty, S. K. Nayak, and H. Srivastava. 2011. Effect of surface treatments of banana fiber on mechanical, thermal, and biodegradability properties of PLA/banana fiber biocomposites. Polymer Composites 32 (11):1689–700. doi:10.1002/pc.21165.
- Jannah, M., M. Mariatti, A. Abu Bakar, and H. P. S. Abdul Khalil. 2009. Effect of chemical surface modifications on the properties of woven banana-reinforced unsaturated polyester composites. Journal of Reinforced Plastic Composites 28 (12):1519–32. doi:10.1177/0731684408090366.
- Jayaprabha, J. S., M. Brahmakumar, and V. B. Manilal. 2011. Banana pseudostem characterization and its fiber property evaluation on physical and bioextraction. Journal of Natural Fibers 8 (3):149–60. doi:10.1080/15440478.2011.601614.
- Jose, S., L. Mishra, G. Basu, and A. K. Samanta. 2017. Study on reuse of coconut fiber chemical retting bath. Part II—Recovery and characterization of lignin. Journal of Natural Fibers 14 (4):510–18. doi:10.1080/15440478.2016.1212772.
- Jose, S., L. Mishra, S. Debnath, S. Pal, P. K. Munda, and G. Basu. 2019. Improvement of water quality of remnant from chemical retting of coconut fibre through electrocoagulation and activated carbon treatment. Journal of Cleaner Production 210:630–37. doi:10.1016/j.jclepro.2018.11011.
- Jose, S., R. Salim, and L. Ammayappan. 2016. An overview on production, properties, and value addition of pineapple leaf fibers (PALF). Journal of Natural Fibers 13 (3):362–73. doi:10.1080/15440478.2015.1029194.
- Jústiz-Smith, N. G., G. J. Virgo, and V. E. Buchanan. 2008. Potential of Jamaican banana, coconut coir and bagasse fibres as composite materials. Materials Characterization 59 (9):1273–78. doi:10.1016/j.matchar.2007.10.011.
- Kalita, H., A. Hazarika, R. Kandimalla, S. Kalita, and R. Devi. 2018. Development of banana (Musa balbisiana) pseudostem fiber as a surgical bio-tool to avert post-operative wound infections. RSC advances 8 (64):36791–801. doi:10.1039/C8RA04470H.
- Kalita, B. B., S. Jose, S. Baruah, S. Kalita, and S. R. Saikia. 2019. Hibiscus sabdariffa (Roselle): A potential source of bast fiber. Journal of Natural Fibers 16 (1):49–57. doi:10.1080/15440478.2017.1401504.
- Kannan, G., and R. Thangaraju. 2022. Effect of industrial waste fly ash on the drilling characteristics of banana fiber residue reinforced polymer composites. Journal of Industrial Textiles 15280837221102641. doi:10.1177/15280837221102.
- Kathirselvam, M., A. Kumaravel, V. P. Arthanarieswaran, and S. S. Saravanakumar. 2019. Isolation and characterization of cellulose fibers from Thespesia populnea barks: A study on physicochemical and structural properties. International Journal of Biological Macromolecules 129:396–406. doi:10.1016/j.ijbiomac.2019.02.044.
- Khan, A., R. Vijay, D. L. Singaravelu, M. R. Sanjay, S. Siengchin, M. Jawaid, K. A. Alamry, and A. M. Asiri. 2022. Extraction and characterization of natural fibers from Citrullus lanatus climber. Journal of Natural Fibers 19 (2):621–29. doi:10.1080/15440478.2020.1758281.
- Khan, A., R. Vijay, D. L. Singaravelu, M. R. Sanjay, S. Siengchin, F. Verpoort, K. A. Alamry, and A. M. Asiri. 2021a. Characterization of natural fibers from Cortaderia selloana grass (pampas) as reinforcement material for the production of the composites. Journal of Natural Fibers 18 (11):1893–901. doi:10.1080/15440478.2019.1709110.
- Khan, A., R. Vijay, D. L. Singaravelu, M. R. Sanjay, S. Siengchin, F. Verpoort, K. A. Alamry, and A. M. Asiri. 2021b. Extraction and characterization of natural fiber from Eleusine indica grass as reinforcement of sustainable fiber reinforced polymer composites. Journal of Natural Fibers 18 (11):1742–50. doi:10.1080/15440478.2019.1697993.
- Kiruthika, A. V., and K. Veluraja. 2009. Experimental studies on the physico-chemical properties of banana fibre from various varieties. Fibers and Polymers 10 (2):193–99. doi:10.1007/s12221-009-0193-7.
- Kulkarni, A. G., K. G. Satyanarayana, P. K. Rohatgi, and K. Vijayan. 1983. Mechanical properties of banana fibres (Musa sepientum). Journal of Materials Science 18 (8):2290–96. doi:10.1007/bf00541832.
- Kumar, R., S. Sivaganesan, P. Senthamaraikannan, S. S. Saravanakumar, A. Khan, S. Ajith Arul Daniel, and L. Loganathan. 2022. Characterization of new cellulosic fiber from the bark of Acacia nilotica L. plant. Journal of Natural Fibers 19 (1):199–208. doi:10.1080/15440478.2020.1738305.
- Li, X., L. G. Tabil, and S. Panigrahi. 2007. Chemical treatments of natural fiber for use in natural fiber-reinforced composites: A review. Journal of Polymers and the Environment 15 (1):25–33. doi:10.1007/s10924-006-0042-3.
- Liu, Y., D. Thibodeaux, G. Gamble, P. Bauer, and D. VanDerveer. 2012. Comparative investigation of Fourier transform infrared (FT-IR) spectroscopy and X-ray diffraction (XRD) in the determination of cotton fiber crystallinity. Applied spectroscopy 66 (8):983–86. doi:10.1366/12-06611.
- Lü, J., C. Long, J. Zhao, L. Ma, H. Lü, J. Liu, and H. He. 2013. Design and experiment of transverse-feeding ramie decorticator. Transactions of the Chinese Society of Agricultural Engineering 29 (16):16–21.
- Madhushani, W. H., R. W. I. B. Priyadarshana, S. R. W. M. C. J. K. Ranawana, K. G. C. Senarathna, and P. E. Kaliyadasa. 2021. Determining the crystallinity index of cellulose in chemically and mechanically extracted banana fiber for the synthesis of nanocellulose. Journal of Natural Fibers 19:1–9. doi:10.1080/15440478.2021.1958428.
- Majhi, S. K., S. K. Nayak, S. Mohanty, and L. Unnikrishnan. 2010. Mechanical and fracture behavior of banana fiber reinforced Polylactic acid biocomposites. International Journal of Plastics Technology 14 (1):57–75. doi:10.1007/s12588-010-0010-6.
- Mishra, S., A. K. Mohanty, L. T. Drzal, M. Misra, and G. Hinrichsen. 2004. A review on pineapple leaf fibers, sisal fibers and their biocomposites. Macromolecular Materials and Engineering 289 (11):955–74. doi:10.1002/mame.200400132.
- Muensri, P., T. Kunanopparat, P. Menut, and S. Siriwattanayotin. 2011. Effect of lignin removal on the properties of coconut coir fiber/wheat gluten biocomposite. Composites. Part A, Applied Science and Manufacturing 42 (2):173–79. doi:10.1016/j.compositesa.2010.11.002.
- Mukherjee, P. S., and K. G. Satyanarayana. 1984. Structure and properties of some vegetable fibres. Journal of Materials Science 19 (12):3925–34. doi:10.1007/BF00980755.
- Mumthas, A. C. S. I., G. L. D. Wickramasinghe, and U. S. Gunasekera. 2019. Effect of physical, chemical and biological extraction methods on the physical behaviour of banana pseudo-stem fibres: Based on fibres extracted from five common Sri Lankan cultivars. Journal of Engineered Fibers and Fabrics 14. 1558925019865697. doi:10.1177/1558925019865697.
- Neelamana, I. K., S. Thomas, and J. Parameswaranpillai. 2013. Characteristics of banana fibers and banana fiber reinforced phenol formaldehyde composites-macroscale to nanoscale. Journal of Applied Polymer Science 130 (2):1239–46. doi:10.1002/app.39220.
- Oliveira, F. R., L. Erkens, R. Fangueiro, and A. P. Souto. 2012. Surface modification of banana fibers by DBD plasma treatment. Plasma Chemistry and Plasma Processing 32 (2):259–73. doi:10.1007/s11090-012-9354-3.
- Ortega, Z., M. Morón, M. D. Monzón, P. Badalló, and R. Paz. 2016. Production of banana fiber yarns for technical textile reinforced composites. Materials 9 (5):370–79. doi:10.3390/ma9050370.
- Pandey, R., S. Jose, G. Basu, and M. K. Sinha. 2021. Novel methods of degumming and bleaching of Indian flax variety Tiara. Journal of Natural Fibers 18 (8):1140–50. doi:10.1080/15440478.2019.1687067.
- Pandey, R., S. Jose, and M. K. Sinha. 2020. Fibre extraction and characterisation from typha domingensis. Journal of Natural Fibers 1–11. 1821285. doi:10.1080/15440478.2020.
- Paramasivam, S. K., D. Panneerselvam, D. Sundaram, K. N. Shiva, and U. Subbaraya. 2020. Extraction, characterization and enzymatic degumming of banana fiber. Journal of Natural Fibers 19:1–10. doi:10.1080/15440478.2020.1764456.
- Paul, S. A., A. Boudenne, L. Ibos, Y. Candau, K. Joseph, and S. Thomas. 2008. Effect of fiber loading and chemical treatments on thermophysical properties of banana fiber/polypropylene commingled composite materials. Composites Part A, Applied Science and Manufacturing 39 (9):1582–88. doi:10.1016/j.compositesa.2008.06.004.
- Pokharel, A., K. J. Falua, A. Babaei-Ghazvini, and B. Acharya. 2022. Biobased polymer composites: A review. Journal of Composites Science 6 (9):255. doi:10.3390/jcs6090255.
- Pothan, L. A., J. George, and S. Thomas. 2002. Effect of fiber surface treatments on the fiber–matrix interaction in banana fiber reinforced polyester composites. Composite Interfaces 9 (4):335–53. doi:10.1163/156855402760194692.
- Pothan, L. A., Z. Oommen, and S. Thomas. 2003. Dynamic mechanical analysis of banana fiber reinforced polyester composites. Composites Science and Technology 63 (2):283–93. doi:10.1016/s0266-3538(02)00254-3.
- Premalatha, N., S. S. Saravanakumar, M. R. Sanjay, S. Siengchin, and A. Khan. 2021. Structural and thermal properties of chemically modified luffa cylindrica fibers. Journal of Natural Fibers 18 (7):1038–44. doi:10.1080/15440478.2019.1678546.
- Rahman, M., S. T. Nipa, S. Z. Rima, M. Hasan, R. Saha, M. Halim, Y. Ali, and A. Deb. 2022. Pseudo-stem banana fiber as a potential low-cost adsorbent to remove methylene blue from synthetic wastewater. Applied Water Science 12 (10):1–16. doi:10.1007/s13201-022-01769-2.
- Ramesh, M., R. Logesh, M. Manikandan, N. S. Kumar, and D. V. Pratap. 2017. Mechanical and water intake properties of banana-carbon hybrid fiber reinforced polymer composites. Materials Research 20:365–76. doi:10.1590/1980-5373-mr-2016-0760.
- Rana, P., and S. Chopra. 2021. Extraction and characterization of inherently antimicrobial fibres from aerial roots of banyan tree. Journal of Natural Fibers 19:1–18. doi:10.1080/15440478.2021.1905586.
- Rodríguez, L. J., M. L. Álvarez-láinez, and C. E. Orrego. 2022. Optimization of processing conditions and mechanical properties of banana fiber-reinforced polylactic acid/high-density polyethylene biocomposites. Journal of Applied Polymer Science 139 (3):51501. doi:10.1002/app.51501.
- Sarma, I., and A. C. Deka. 2016. Banana fibre extraction by mycogenic pectinase enzyme (S)-an eco-friendly approach. Imperial Journal of Interdisciplinary Research 2 (10):997–1006.
- Singh, G., S. Jose, D. Kaur, and B. Soun. 2020. Extraction and characterization of corn leaf fiber. Journal of Natural Fibers 1–11. doi:10.1080/15440478.2020.1787914.
- Siva, R., T. N. Valarmathi, K. Palanikumar, and A. V. Samrot. 2020. Study on a novel natural cellulosic fiber from Kigelia africana fruit: Characterization and analysis. Carbohydrate Polymers 244:116494. doi:10.1016/j.carbpol.2020.116494.
- Siva, R., T. N. Valarmathi, A. V. Samrot, and J. J. Jeevahan. 2021. Surface-modified and untreated Cissus quadrangularis reinforced polylactic composite. Current Research in Green and Sustainable Chemistry 4:100121. doi:10.1016/j.crgsc.2021.100121.
- Siva, R., T. N. Valarmathi, B. Siddardha, K. Sanjana, and B. Dakshin. 2019. Processing and evaluation of mechanical properties of sisal and bamboo chemically treated hybrid composite. In Innovative design, analysis and development practices in aerospace and automotive engineering, ed. U. Chandrasekhar, L. J. Yang, and S. Gowthaman, 435–41. Singapore: Springer.
- Song, Y., W. Jiang, K. Nie, Y. Zhang, H. Ben, G. Han, and A. J. Ragauskas. 2019. An alkali-free method to manufacture ramie fiber. Textile Research Journal 89 (17):3653–59. doi:10.1177/0040517518811946.
- Subagyo, A., and A. Chafidz. 2018. Banana pseudo-stem fiber: Preparation, characteristics, and applications. In Banana nutrition-function and processing kinetics, ed. I. O. J. Afam and T. A. Anyasi, 47–65. Intech Open. doi: 10.5772/intechopen.82204.
- Sumesh, K. R., V. Kavimani, G. Rajeshkumar, S. Indran, and A. Khan. 2022. Mechanical, water absorption and wear characteristics of novel polymeric composites: Impact of hybrid natural fibers and oil cake filler addition. Journal of Industrial Textiles 51 (4):5910S–37. doi:10.1177/1528083720971344.
- Taer, E., D. A. Yusra, A. Amri, A. Taslim, R. Putri. 2021. The synthesis of activated carbon made from banana stem fibers as the supercapacitor electrodes. Materials Today: Proceedings 44:3346–49. doi:10.1016/j.matpr.2020.11.645.
- Teli, M. D., and S. P. Valia. 2013. Acetylation of banana fibre to improve oil absorbency. Carbohydrate Polymers 92 (1):328–33. doi:10.1016/j.carbpol.2012.09.019.
- Twebaze, C., M. Zhang, X. Zhuang, M. Kimani, G. Zheng, and Z. Wang. 2022. Banana fiber degumming by alkali treatment and ultrasonic methods. Journal of Natural Fibers 19:1–13. doi:10.1080/15440478.2022.2079581.
- Udaya Kiran, C., G. Ramachandra Reddy, B. M. Dabade, and S. Rajesham. 2007. Tensile properties of sun hemp, banana and sisal fiber reinforced polyester composites. Journal of Reinforced Plastics and Composites 26 (10):1043–50. doi:10.1177/0731684407079423.
- Uma, S., M. S. Saraswathi, and P. Durai. 2019. Banana genetic resources. In Conservation and utilization of horticultural genetic resources, ed. P. Rajasekharan and V. Rao, 321–61. Singapore: Springer. doi:10.1007/978-981-13-3669-0_10.
- Vajpayee, M., M. Singh, L. Ledwani, R. Prakash, and S. K. Nema. 2020. Investigation of antimicrobial activity of DBD air plasma-treated banana fabric coated with natural leaf extracts. ACS Omega 5 (30):19034–49. doi:10.1021/acsomega.0c02380.
- Venkateshwaran, N., and A. Elayaperumal. 2010. Banana fiber reinforced polymer composites-a review. Journal of Reinforced Plastics and Composites 29 (15):2387–96. doi:10.1177/0731684409360578.
- Vigneswaran, C., V. Pavithra, V. Gayathri, and K. Mythili. 2015. Banana fiber: Scope and value added product development. Journal of Textile and Apparel, Technology and Management 9 (2):1–7.
- Vishnu Vardhini, K. J., and R. Murugan. 2017. Effect of laccase and xylanase enzyme treatment on chemical and mechanical properties of banana fiber. Journal of Natural Fibers 14 (2):217–27. doi:10.1080/15440478.2016.1193086.
- Zaman, H. U., M. A. Khan, and R. A. Khan. 2011. Physico-mechanical and degradation properties of banana fiber/LDPE composites: Effect of acrylic monomer and starch. Composite Interfaces 18 (8):685–700. doi:10.1163/156855412x626261.