Abstract
Given our recent finding that the lymph node (LN) can serve as an in vivo factory to generate complex structures like liver, pancreas, and thymus, we investigated whether LN could also support early development and maturation from several mid-embryonic (E14.5/15.5) mouse tissues including brain, thymus, lung, stomach, and intestine. Here we observed brain maturation in LN by showing the emergence of astrocytes with well-developed branching processes. Thymus maturation in LN was monitored by changes in host immune cells. Finally, newly terminally differentiated mucus-producing cells were identified in ectopic tissues generated by transplantation of lung, stomach and intestine in LN. Thus, we speculate the LN offers a unique approach to study the intrinsic and extrinsic differentiation potential of cells and tissues during early development, and provides a new site for bioengineering complex body parts.
Abbreviations
LN | = | lymph node |
FAH | = | fumarylacetoacetate hydrolase |
mTOR | = | mammalian target of rapamycin |
E14.5/15.5 | = | embryonic day 14.5 to 15.5 |
GFAPδ | = | glial fibrillary acid protein delta |
MAP-2 | = | Microtubule-associated protein 2 |
FACS | = | fluorescence-activated cell sorting |
GM-CSF | = | granulocyte-macrophage colony-stimulating factor |
K8 | = | keratin 8 |
K5 | = | keratin 5 |
EpCAM1 | = | epithelial cell adhesion molecule 1 |
mTEC | = | medullary thymic epithelial cell |
cTEC | = | cortical thymic epithelial cell |
Aire | = | autoimmune regulator |
CgA | = | chromogranin A |
3D | = | 3-dimensional |
2D | = | 2-dimensional |
ECM | = | extracellular matrix |
EmT | = | embryonic thymus |
6wEcT | = | 6-week ectopic thymus |
21wEcT | = | 21-week ectopic thymus |
AdT | = | adult thymus |
EmL | = | embryonic lung |
EmS | = | embryonic stomach |
EmI | = | embryonic intestine |
3wEcL | = | 3-week ectopic lung |
3wEcS | = | 3-week ectopic stomach |
3wEcI | = | 3-week ectopic intestine |
ER-TR7 | = | reticular fibroblasts and reticular fibers |
Introduction
We have previously shown that primary mouse hepatocytes injected intraperitoneally into mice lacking fumarylacetoacetate hydrolase (FAH, a mouse model of tyrosinemia type I) migrate and colonize the host abdominal lymphatics and restore hepatic functions.Citation1 Furthermore, we provided evidence that mouse hepatocytes and pancreatic islets injected directly into a single LN generate functional tissues, rescuing mice from lethal liver failure, and streptozotocin-induced diabetes, respectively.Citation2 Similarly, de novo thymic function could be generated in athymic mice by injecting murine thymic tissues into their LNs.Citation2 More recently, we used the mouse LN to grow human fetal intestinal epithelial cells and assess toxicity of a mammalian target of rapamycin (mTOR) inhibitor against normal cells.Citation3 These 4 independent results support the concept that LN provides a hospitable environment for normal cell engraftment.
Here we investigated LN ability to support growth and maturation of several mouse mid-embryonic (E14.5/15.5) tissues including brain, thyroid, thymus, lung, heart, stomach, intestine, liver, and adrenal gland. While results were promising for brain, thymus, lung, stomach, and intestine, we were less successful to grow thyroid, heart, liver, or adrenal gland. We do not rule out that these tissues could still be effectively grown inside the LN when isolated using a different protocol, or at a different developmental stage as demonstrated previously with adult hepatocytes instead of fetal liver tissues.Citation4 Conversely, we were able to detect terminally differentiated cells in ectopic grafts of brain, thymus, lung, stomach and intestine. Moreover, we provide evidence of host contribution in the maturation of the thymic epithelium within the LN. Our novel findings suggest LN can be used to study stem cell biology in development, maturation, and regeneration.
Results
The lymph node is a permissive site for tissue development
We first investigated LN ability to support engraftment of several mouse mid-embryonic tissues including brain, thyroid, thymus, lung, heart, stomach, intestine, liver, and adrenal gland. Tissues were harvested from embryonic day (E) 14.5 to 15.5 GFP transgenic mice, minced, and injected directly into a single jejunal LN of adult wild-type mice (). Transplant engraftment success into the LN was inconsistent across the various tissues tested. While the brain showed the highest ability to repopulate the LN, in our hands, the thyroid was the only tissue completely unable to engraft (). Although the heart, liver and adrenal gland engrafted occasionally, their grafts were small. We therefore focused our attention on brain, thymus, lung, stomach, and intestine.
Figure 1. Summary of tissue transplantation and astrogenesis of developing brain within the LN. Schematic view of transplantation of multiple E14.5/15.5 tissues into the LN (scale bar, 1mm) (A). Table shows number of LNs repopulated by different tissues (B). Hematoxylin and Eosin (H&E) staining of a paraffin section of donor mid-embryonic brain before transplantation (upper left); whole-mount jejunal LN 3 weeks after brain transplantation (B3, upper right), and pictures of frozen LN sections (B3 and B4, 2 independent samples) with the presence of GFP+ (green) donor cells (lower). Nuclei were counterstained using Hoechst (blue) (C). Pictures of B3 and B4 LN sections stained for GFAPδ (red) with the presence of GFP+ (green) donor cells. Nuclei were counterstained using Hoechst (blue) (D).
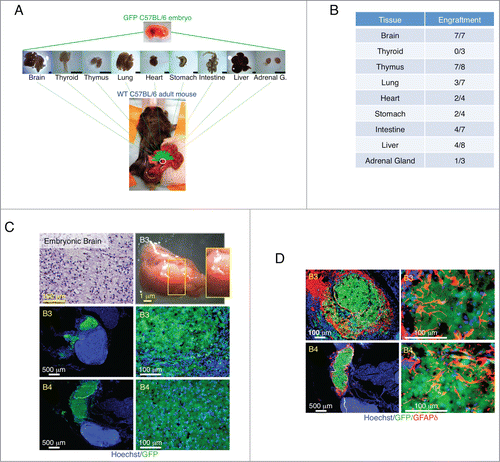
Maturation of the ectopic brain
We found the LN to be a permissive environment for the growth of E14.5/15.5 brain implants (). In order to understand whether maturation had occurred, we assessed glial fibrillary acid protein delta (GFAPδ) expression. In the developing mouse brain, GFAPδ is absent at E15 and begins to be expressed at E18, coinciding with the start of astrogenesis.Citation5 As the animal matures, GFAPδ+ cells lose their bipolar morphology and shift toward a more star-like, mature phenotype.Citation5 Interestingly, GFAPδ+ cells with complex branching and extended processes were observed in ectopic brain grafts as early as 3 weeks after transplantation, indicating brain maturation inside the LN (). Grafted brains also stained positive for Microtubule-associated protein (MAP)-2, a marker of neuronal differentiation that is likewise expressed in embryonic stage by cells committed to the neuronal lineage (Supplementary Fig. 1A). Interestingly, distant from the main brain graft, cells with a branching structure could be found, indicating possible cell detachment from the bulk mass and migration to secondary sites (Supplementary Fig. 1B).
We next sought to investigate whether the LN could provide the environment necessary for intrinsic cues to influence maturation of neural stem and progenitor cells. These cells were derived from E14 C57BL/6-GFP mouse fore- and midbrain, and propagated as floating spheres. 5 d primary neurospheres showed the appearance of microspikes known to appear on young healthy neurospheres (, panel 1), and stained positive for the neural stem cell-type specific markers Nestin and SOX2 and the proliferation marker Ki-67 (, right). Primary neurospheres were therefore injected into the jejunal LN as intact spheres (approximately 100 spheres/mouse; , panel 3) or single cells (5 × 105 cells/mouse; , panel 4). After 3 weeks, mice were sacrificed, but no grafts were found (, left). We repeated the experiment, injecting at least 106 cells/mouse as intact spheres, and sacrificed mice 1 or 3 weeks later. This time, we found grafts in both groups (, right). However, regardless of the time permitted for development, the grafts remained very small and did not display discrete brain regions. Nonetheless, they stained positive for GFAPδ (, left) and MAP-2 (, right), confirming our previous finding of brain cell maturation within the LN. Overall, although the LN supports the expansion of several cell types, it does not provide a permissive environment for neurogenesis from neural stem and progenitor cells.
Figure 2. The LN does not provide a permissive environment for neurogenesis from neural stem and progenitor cells. Appearance of 5 d floating primary neurospheres (panels 1–2); intact spheres (panel 3) or single cells (panel 4) used for LN injections, and immunofluorescence staining for Nestin, SOX2, or Ki-67 (right, red). Nuclei were counterstained using Hoechst (blue) (A). Table shows a summary of LN engraftment outcome in 20 mice injected with neurospheres in 2 separate experiments (B). Immunofluorescence staining for GFAPδ (left, red) or MAP-2 (right, red) with GFP+ donor cells (green). Nuclei were counterstained using Hoechst (blue) (C).
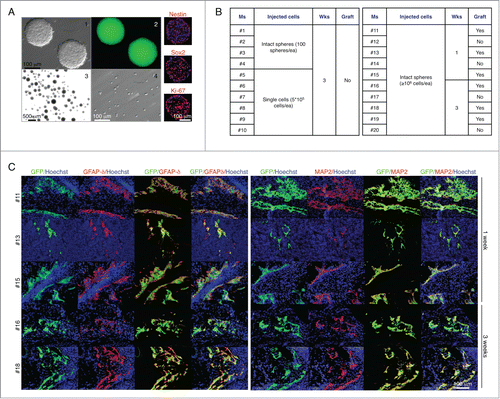
Maturation of the thymic epithelium in an ectopic site: contribution of the host in the generation of the thymic cortex
In order to understand whether E14.5/15.5 thymus could mature inside the LN and become functional, we monitored percentages of granulocytes/myeloid cells and lymphocytes by fluorescence-activated cell sorting (FACS) analysis of peripheral blood of recipient mice up to 21 weeks from transplantation. Gating strategy is indicated in Supplementary Figure 2A. The CD11b+/Ly6G-Ly6C−/low and CD11b+/Ly6G-Ly6Cint cell populations (primarily immature myeloid cells) were dramatically increased 3 weeks after thymus transplantation, to the detriment of the CD11b+/Ly6G-Ly6Chigh cell population (primarily mature myeloid cells). All cell populations returned to control levels by 21 weeks (, Supplementary Fig. 2B). Slight changes were also observed in the lymphocyte population (data not shown). Indeed, thymus transplantation resulted in increased T-cell percentages, identified by expression of CD3. In particular, the CD8 T-cell subset was increased across transplanted mice; however, both total and CD4−/CD8+ T cell percentages were normalized within 6 weeks. Conversely, the CD4+/CD8− cell population remained unchanged in all the time points examined. Overall, these changes reflect ectopic graft functionality. The striking effect on the CD11/Ly6G-Ly6C phenotype possibly indicates maturation of the ectopic thymic epithelium and enhanced transcription of the granulocyte-macrophage colony-stimulating factor (GM-CSF). Production of this cytokine eventually results in expansion of immature myeloid cells, and consequently, granulocyte/macrophage progenitor accumulation. During thymic ontogeny, GM-CSF mRNA reaches its peak level around E19–20. Before this stage, it is undetectable during the early amplification cycles of a semi-quantitative PCR.Citation6,7 We therefore analyzed GM-CSF mRNA levels in the ectopic thymus, using the donor thymus for comparison. While levels of GM-CSF mRNA were hardly detectable in E14.5/15.5 thymus, GM-CSF mRNA was clearly present in 6- as well as in 21-week ectopic grafts, but the levels were lower than in the adult thymus (). We thus hypothesized that successful restoration of physiological levels of all immune cell subsets analyzed within 21 weeks from thymus transplantation could not have been due to ectopic graft degeneration. Ectopic grafts varied in size (, lower). While E14.5/15.5 thymus had a uniform parenchyma with light staining (, upper right), 21-week ectopic thymus comprised a meshwork of epithelial cells reactive to keratin 8 (K8) or keratin 5 (K5) antibodies, indicating a cortical or a medullary identity, respectively (). Nevertheless, a clear corticomedullary compartmentalization was never observed (Supplementary Fig. 2C). Interestingly, most of K8+ cells were of host origin, while most of K5+ cells were of donor origin (). Thymic grafts also showed chimeric blood (CD31+) vessels expressing the CD105 neoangiogenic marker ().
Figure 3. Granulocyte/macrophage progenitor accumulation following E14.5/15.5 thymus transplantation within the LN, and host contribution in the generation of the ectopic thymic cortex. Representative fluorescence intensity histograms of granulocytes/myeloid cells from mouse 2 (M2) analyzed for Ly6G-Ly6C (upper) or CD11b (lower) at 0, 3, 6, 12, or 21 weeks after thymus transplant (A). Dot plots showing frequency of CD11b+/Ly6G-Ly6C−/low, CD11b+/Ly6G-Ly6Cint, and CD11b+/Ly6G-Ly6Chigh at 0, 3, 6, 12, or 21 weeks after thymus transplant. Each symbol represents one mouse, and the horizontal bars represent the median values. * P < 0 .05, ** P < 0 .01, *** P < 0 .001 (B). Agarose gel electrophoresis of PCR products following semi-quantitative RT-PCR analysis for GM-CSF (expected amplicon size of 431 bp) in mid-embryonic thymus (EmT), 6- (6wEcT) or 21-week ectopic thymus (21wEcT), and adult thymus (AdT). Wild type LN (LN) was used as a negative control. Amplification of GAPDH was used as an internal control. The densitometric scanning data from 2 experiments are shown as bar graphs of GM-CSF/GAPDH ratio on the right (6wEcTs were isolated from M4 and M5, while 21wEcTs were isolated from M1 and M3) (C). Picture of a native thymus gland isolated from a C57BL/6 GFP+ embryo (upper left) and H&E staining of a paraffin section of native mid-embryonic thymus (EmT, upper right); whole-mount mouse jejunal LNs 21 weeks after thymus transplantation, showing 3 different engraftments (21wEcT, lower) (D). Immunofluorescence staining for GFP (red), keratin 8 (K8, red), keratin 5 (K5, red), CD31 (red) or CD105 (red) of 21-week ectopic thymus with the presence of GFP+ (green) donor cells. Nuclei were counterstained using Hoechst (blue) (GFP, K8, K5 pictures were taken from a LN isolated from M2, while CD31 and CD105 pictures were taken from a LN isolated from M6) (E).
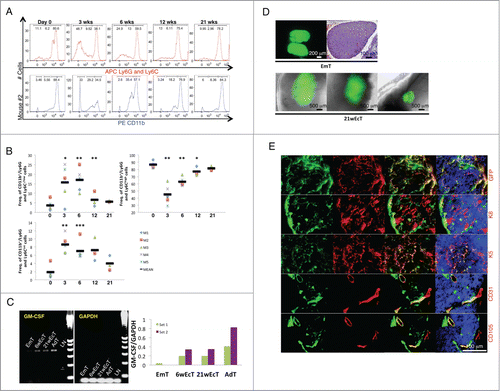
While previous studies of thymus development suggested that cortex and medulla derive from ectoderm and endoderm, respectively, a more recent study has provided clear evidence of a single endodermal origin for the thymic epithelium.Citation8 Other important evidence has indicated that both cortical and medullary descendants arise from a single E12 progenitor expressing Epithelial Cell Adhesion Molecule 1 (EpCAM1/CD326).Citation9 Around E14/15, 2 different cell subsets can be distinguished within the EpCAM1+ population, possessing the CD40+CD205− or the CD40−CD205+ signature, identifying putative medullary (mTECs) and cortical (cTEC) thymic epithelial progenitors, respectively.Citation10 Although some controversy exists in the literature, it is likely that further maturation of CD205+ cTECs until they express MHC class II and CD40 requires the presence of CD4−/CD8− thymocytes.Citation10 Thus, it is conceivable that within the LN, where a normal program of T cell development is absent, further maturation of donor cTECs is hindered to the point that host cells may need to be recruited to the developing graft to ensure function. Differently from cTECs, lineage-restricted medullary progenitors might diverge earlier, along the stratification process of the anlage.Citation11 The autoimmune regulator (Aire), modulating the mTEC differentiation program, begins to be expressed at E14.5.Citation12 Thus, it is likely that mTECs are already committed to their fate around this stage, and do not need any further extrinsic signal to complete their maturation. This might explain why donor mTECs are better able to acquire a mature phenotype with long-term retention by the graft than the donor cTECs. However, although most of K5+ cells are of donor origin, some are not, indicating that mTECs might also be recruited from the host. Alternatively, host-derived cTECs might differentiate into mTECs, as suggested by very recent studies.Citation13-15 Nevertheless, in our study, the origin of these cells is not clear but could come from the host thymus. Whether fully mature cortical or uncommitted bipotent thymic epithelial cells are recruited to the ectopic graft remains to be investigated. Overall, our findings reveal that the LN might be exploited to understand how and when cortical and medullary lineages diverge.
Presence of terminally differentiated, mucus-producing cells in ectopic lung, stomach and intestinal tissues
E14.5/15.5 lung fragments arranged in lobe-like structures within the LN, and showed signs of differentiation from a pseudoglandular to mixed saccular/alveolar morphology 3 weeks after transplantation. Importantly, ectopic lung comprised a glandular epithelium with MUC5AC-producing goblet cells 10 weeks after transplantation, indicating that it is possible to achieve a postnatal stage of lung development within the LN ().
Figure 4. Presence of terminally differentiated, mucus-producing cells in ectopic lung, stomach and intestinal tissues. Each panel shows an H&E staining of a paraffin section of donor mid-embryonic lung (EmL), stomach (EmS) or intestine (EmI); whole-mount jejunal LN 3 weeks after transplantation of lung (3wEcL), stomach (3wEcS) or intestine (3wEcI), and pictures of frozen LN sections stained with specific markers (red) with the presence of GFP+ (green) donor cells. Nuclei were counterstained using Hoechst (blue) (ER-TR7, Reticular Fibroblasts and Reticular Fibers; CgA, chromogranin A) (A-C).
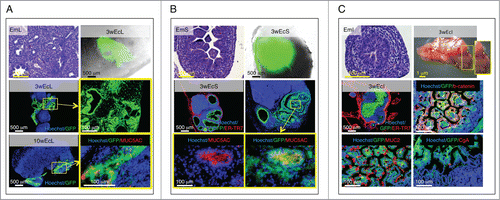
Similarly, E14.5/15.5 stomach fragments engrafted and expanded nicely within the LN. This ectopic stomach also comprised MUC5AC-producing goblet cells 3 weeks after transplantation ().
While intestinal development occurs quite early during mammalian embryogenesis, intestinal maturation takes place during the post-embryonic period.Citation16 Crypt-like structures developed following injection of intestinal fragments into LNs and membrane-localized β-catenin was detected all along these crypts. Activity of the Wnt/β-catenin pathway is required for the emergence of secretory cell types in the intestinal epithelium.Citation17 Since both MUC2-reactive goblet-like cells and chromogranin A (CgA)-producing enteroendocrine cells could be detected in intestinal grafts 3 weeks after transplantation, our results likely indicate that Wnt signals were active and supported intestinal epithelium terminal differentiation within the LN ().
Discussion
In vivo, stem cells reside in a highly specialized 3-dimensional (3D) structure, the so-called stem cell niche.Citation18 Not only does this niche preserve the stem cell pool, but it also promotes progenitor cell expansion and mobilization. Reproducing this dynamic and complex microenvironment in culture is challenging, either because the mechanisms that control stem cell fate in vivo have not yet been fully elucidated, or because of ethical and technical issues.
Conventional two-dimensional (2D) cell cultures poorly mimic the physiological environment. Key events like cell-to-cell and cell-extracellular matrix (ECM) interactions, cell polarization, adhesion, and migration cannot be fully recreated in a monolayer cell culture. Simple 3D systems like cellular spheroidsCitation19 and other scaffold-free platforms using ECM hydrogelsCitation20 or hydrogel-grown stromal cellsCitation21 were introduced as a way to overcome some of the limitations of traditional 2D cell culture. Synthetic and natural (decellularized) scaffolds that provide a defined 3D structure for tissue development and bio-degrade at a controlled rate were also created.Citation22 However, these scaffolds are unable to supply adequate oxygen and nutrients unless combined with endothelial cells.Citation23 Unfortunately, an estimation of how many vessels are required for a particular tissue remains critical. Mechanical means have also been exploited to modulate scaffold properties, leading to the development of more or less sophisticated bioreactor systems with improved oxygen and nutrient delivery. Nevertheless, drawbacks to currently available bioreactors include difficulty in assembly/disassembly, prolonged culture times, risk of cell contamination, and low cell output.
All these different approaches have diminished the gap between cell culture and living tissue. Nevertheless, while success has been achieved with bone,Citation24 cartilage,Citation25 and heart valveCitation26 engineering, the reconstruction of more complicated 3D tissues is still challenging. A major limitation remains the vascularization of the transplanted tissue.Citation27 Our previous studies indicated the LN guarantees optimal engraftment with long-term function by providing a highly vascularized environment.Citation1–3 Accordingly, here we showed the ability of mouse LN to support development of different tissues including brain, thymus, lung, stomach and intestine. Although we did not assess the presence of all mature cell types in ectopic grafts, we do not rule out that all different cell lineages could be present in the tissues developed. Further investigations will address this hypothesis. For lung, intestine, and stomach, we monitored goblet cell mucus secretion within the columnar epithelium as readout of terminal differentiation and maturation of the tissues injected. Indeed, mucus production mostly takes place postnatally. For the intestine, for example, not only is mucus mainly secreted postnatally, but also during the first 2 weeks after birth, its production is negligible, due to a low number of goblet cells.Citation28 Therefore, the finding of several MUC2-reactive cells in our grafts, only 3 weeks after transplantation, indicates the LN offered a favorable environment for maturation of the ectopic intestine. Among others, enteroendocrine cells represent less than 1% of intestinal epithelial cells,Citation29 making hard their identification in tissue specimens. Nevertheless, we found a quite few enteroendocrine cells in our grafts, as indicated by reactivity to the CgA marker. Conversely, although we previously showed that direct injection of adult mouse hepatocytes into a single mouse LN generates enough ectopic liver mass to rescue the survival of mice with lethal metabolic disease,Citation2 growth of E14.5/15.5 liver within the LN was not robust. There are at least 3 reasons why fetal liver fragments are not one of the most successful tissues to grow in the LN when compared to our previous experiments. First, we used fetal, not adult, liver. Fetal hepatocytes have a significantly reduced engraftment potential compared to adult hepatocytes, both in mouse and human settings.Citation4 Second, we used liver fragments and not a single cell suspension of hepatocytes. Fragments of liver tissue may have a harder time to survive compared to cells. Third, we used a wild type mouse and not a mouse model of liver disease (FAH-/-) as a recipient. A need for a selective environment to regenerate hepatic functions (and an ectopic liver) is undoubtedly necessary. Differently from the liver, although the LN efficiently supported the expansion of E14.5/15.5 brain fragments, it did not provide a permissive environment for neurogenesis from single neural stem and progenitor cell suspensions, indicating transplant engraftment success into the LN is inconsistent across different tissues.
In conclusion, with the mouse being the preferred model for mammalian development, the transplantation of mouse embryonic tissue inside the LN might provide the developmental biologist a powerful tool to unravel the cellular and molecular mechanisms governing proliferation, specification, differentiation, and function of several cell types during normal development. Mechanisms like stem cell-niche interactions, and chromatin and epigenetic regulation of early development might be investigated, and linked to physiological processes like stem cell maintenance, and regeneration and repair, as well as pathological processes such as tumor growth and metastasis. Importantly, our previous, current, and unpublished observations indicate that different tissues in the LN can sense a stimulus and respond appropriately.Citation2 On the other hand, the host senses the graft by providing new cells, as here suggested by host contribution in the maturation of the thymic cortex. Although further studies are needed to understand how donor cells communicate with neighboring and distant host cells, this study suggests the LN might have future research applications if exploited as an in vivo bioreactor. The LN may provide an exclusive site for organogenesis for therapeutic purposes. It is reasonable to think that transplanting cells orthotopically would provide the best environment for cell survival and function. However, cell therapy approaches to restore loss of tissue function or damaged tissue may not be possible when the native environment is not hospitable or readily accessible. Ectopic organogenesis may be a useful approach to provide compensatory function for the damaged or diseased native organ. Renal capsule grafting is a well-established method of growing rudimentary organs in vivo for extended periods. However, whereas ectopic transplantation under the kidney capsule is suitable in rodents, it is clinically unrealistic as the human kidney capsule and parenchyma cannot be easily separated to permit cell or tissue transplantation.Citation30 The omentum, a more accessible site, also provides a favorable environment for implant revascularization.Citation31 Nevertheless, a major disadvantage using omentum in the clinic is that repeated transplantation is not allowed, as well as laparotomy for any reason. Furthermore, omental manipulation may result in postoperative adhesions and intestinal obstruction.Citation32,33 For cell-based therapy to be effective, a hospitable site for transplantation must be relatively accessible, provide sufficient space, offer access to vasculature, and support long-term engraftment.Citation34 There are over 500 LNs in the human body, many of which are relatively easily accessible. Although a single LN structurally limits the number of donor cells that can be transplanted, it is technically feasible to transplant more than one LN to gain sufficient organ or tissue function from the transplanted cells. The potential loss of function in a few LNs does not seem to compromise the overall function of the lymphatic system.Citation35 Moreover, LNs have ready access to the bloodstream, and can therefore foster cell growth by providing nutrients as well as hormones and growth factors. Importantly, new angiogenesis occurs fast enough in this site to sustain cell survival and engraftment of normal tissues. We therefore suggest LN meets all criteria for being considered a valuable candidate transplantation site. Eventually, studies in large animals will show just how effective transplantation into the LNs could be. Overall, although the LN is not a common location for transplantation, it is not such a crazy idea to consider it as an alternative site for cell transplantation, at least for some tissues.Citation36
Materials and Methods
Tissue collection, cell culture, and transplantation
Embryonic day (E) 14.5 to 15.5 tissues were retrieved from timed pregnant GFP+ C57BL/6 black mice under a dissecting microscope (embryos were considered 0.5 d old when the vaginal plug was detected in the morning). All tissue were minced in PBS and kept on ice until injection.
Neural stem and progenitor cells were derived from E14 C57/B6-GFP mouse fore- and midbrain and propagated as floating spheres in NeuroCult™ NSC Basal Medium (Catalog #05700) supplemented with NeuroCult™ NSC Proliferation Supplement (Catalog #05701) and rhEGF (Catalog #02633). NeuroCult™ Chemical Dissociation Kit was used for the non-mechanical and non-enzymatic dissociation of neurospheres (Catalog #05707).
For LN transplantation, recipient mice (wild-type C57BL/6 black mice, n = 71) were anesthetized with 1–3% isoflurane. A small incision was made in the abdomen to expose jejunal LNs. Tissue fragments were slowly injected with a 1000 μL threaded plunger syringe (Hamilton, 81341) and 20 gauge removable needle. Neural stem and progenitor cells were injected using a 100 μL gas-tight syringe (Hamilton, 7656–01) and 27 gauge removable needle (Hamilton, 7803–01). Incisions were cauterized and sutured. Ketoprofen (2mg/kg, IM) was then administered for 2 d to relieve postoperative pain. Mice were euthanized for analysis at pre-defined time points.
Mice were purchased from Charles River, and bred and housed in the Division of Laboratory Animal Resources facility at the University of Pittsburgh Center for Biotechnology and Bioengineering. Experimental protocols followed US National Institutes of Health guidelines for animal care and were approved by the Institutional Animal Care and Use Committee at the University of Pittsburgh.
Histology and immunofluorescence/immunohistochemistry
Repopulated jejunal LNs were fixed 2 hours in 4% PFA, and embedded in Optimal Cutting Temperature (OCT) following overnight infiltration with 30% sucrose. Sections were stained with antibodies against GFAPδ (Bioss, bs-11016R), MAP2 (Abcam, ab32454), GFP (Abcam, ab6556), Keratin-8 (DSHB, TROMA-1), Keratin-5 (Covance, PRB-160P), CD31 (BD, 550274), CD105 (BD, 550546), ER-TR7 (Abcam, ab51824), MUC5AC (Abcam, ab79082), β-catenin (CST, 8480), MUC2 (SCT, sc-15334), or chromogranin A (SCT, sc-18232). Whole intact neurospheres were fixed 30 min in 4% PFA, and embedded in OCT for cryostat sectioning. Frozen sections of neurospheres were successively stained using the following antibodies: Nestin (Ebioscience, 14–5843–82), SOX2 (Abcam, ab97959), Ki-67 (Abcam, ab15580). Alexa Fluor 594 (Invitrogen) secondary antibodies were used for all primary antibodies. Nuclei were counterstained using Hoechst. Donor organs were embedded in paraffin and stained with hematoxylin and eosin (H&E) as described elsewhere.
Flow cytometry
Whole blood was collected in K2EDTA collection tubes (Terumo Medical). One hundred microliters of blood was added to cold fluorescence-activated cell sorting (FACS) tubes. Antibodies were added at a dilution of 1/50 in blood and mixed by gentle pipetting. Antibodies used were as follows: PerCP-Cy5.5 CD45 (BD, 550994), PE CD11b (BD, 553311), and APC Ly6G-Ly6C (BD, 553129). Reactions were incubated in the dark in an ice slurry bath for one hour. Three milliliters of Red Blood Cell Lysing Buffer (Sigma) was added to each tube, lightly vortexed and incubated for an additional 15 min. Two milliliters of flow buffer (2% FBS in HBSS) was added to the tubes, mixed and centrifuged at 500g for 5 min. The supernatant was aspirated. The red blood cell lysis and centrifuge were repeated as described. The final cell pellet was resuspended in 400 μl of flow buffer with Sytox Blue dye. Cells were analyzed using a Miltenyi MACSQuant and FlowJo software (Tree Star).
RNA extraction, cDNA synthesis, RT-PCR
Total RNA was isolated from tissues stored in RNAlater reagent (QIAGEN, 76104) using RNeasy Mini kit (QIAGEN, 74104), according to the manufacturer's instructions. Potentially contaminating genomic DNA was digested using DNase (QIAGEN, 79254). cDNA was synthesized using the iScript™ Reverse Transcription Supermix for RT-qPCR (Bio-Rad, 170–8841). PCR was performed using the iTaq DNA Polymerase kit (Bio-Rad, 170–8870). GAPDH transcript levels served as the housekeeping control target. Sequences of primers were as follows: GM-CSF, Fwd, 5′- TTCCTGGGCATTGTGGTCT-3’, Rev, 5′-TGGATTCAGAGCTGGCCTGG-3′; GAPDH, Fwd, 5′-GGCATCCTGGGCTACACTGA-3′, Rev, 5′-GGAGTGG-GTGTCGCTGTTG-3′. For GM-CSF, PCR mixtures were subjected to different numbers of amplification cycles; 23 cycles were eventually chosen to quantify gene expression.
Statistical analysis
Data are presented as means ±SD. Statistical analysis was performed using Student's t test (P < 0.05 was considered significant).
Disclosure of Potential Conflicts of Interest
No potential conflicts of interest were disclosed.
Supplementary_Figures.pptx
Download MS Power Point (192.9 MB)Acknowledgments
We thank Lynda Guzik for proofreading and editing.
Funding
This work was supported by Ri.MED Foundation (MGF), and by the NIH grant R01 DK085711 (EL).
Supplemental Material
Supplemental data for this article can be accessed on the publisher's website.
References
- Hoppo T, Komori J, Manohar R, Stolz DB, Lagasse E. Rescue of lethal hepatic failure by hepatized lymph nodes in mice. Gastroenterology 2011; 140:656-66.e2; PMID:21070777; http://dx.doi.org/10.1053/j.gastro.2010.11.006
- Komori J, Boone L, DeWard A, Hoppo T, Lagasse E. The mouse lymph node as an ectopic transplantation site for multiple tissues. Nat Biotechnol 2012; 30:976-83; PMID:23000933; http://dx.doi.org/10.1038/nbt.2379
- Francipane MG, Lagasse E. Selective targeting of human colon cancer stem-like cells by the mTOR inhibitor Torin-1. Oncotarget 2013; 4:1948-62; PMID:24185040
- Komori J, Deward AD, Gramignoli R, Strom SC, Fontes P, Lagasse E. Potential barriers to human hepatocyte transplantation in MUP-uPARag2gammaC mice. Cell Transplantation 2013; PMID:23998208
- Mamber C, Kamphuis W, Haring NL, Peprah N, Middeldorp J, Hol EM. GFAPdelta expression in glia of the developmental and adolescent mouse brain. PLoS One 2012; 7:e52659; PMID:23285135; http://dx.doi.org/10.1371/journal.pone.0052659
- Montgomery RA, Dallman MJ. Analysis of cytokine gene expression during fetal thymic ontogeny using the polymerase chain reaction. J Immunol 1991; 147:554-60; PMID:1649220
- Montgomery RA, Dallman MJ. Semi-quantitative polymerase chain reaction analysis of cytokine and cytokine receptor gene expression during thymic ontogeny. Cytokine 1997; 9:717-26; PMID:9344502; http://dx.doi.org/10.1006/cyto.1997.0227
- Gordon J, Wilson VA, Blair NF, Sheridan J, Farley A, Wilson L, Manley NR, Blackburn CC. Functional evidence for a single endodermal origin for the thymic epithelium. Nat Immunol 2004; 5:546-53; PMID:15098031; http://dx.doi.org/10.1038/ni1064
- Rossi SW, Jenkinson WE, Anderson G, Jenkinson EJ. Clonal analysis reveals a common progenitor for thymic cortical and medullary epithelium. Nature 2006; 441:988-91; PMID:16791197; http://dx.doi.org/10.1038/nature04813
- Shakib S, Desanti GE, Jenkinson WE, Parnell SM, Jenkinson EJ, Anderson G. Checkpoints in the development of thymic cortical epithelial cells. J Immunol 2009; 182:130-7; PMID:19109143; http://dx.doi.org/10.4049/jimmunol.182.1.130
- Hamazaki Y, Fujita H, Kobayashi T, Choi Y, Scott HS, Matsumoto M, Minato N. Medullary thymic epithelial cells expressing Aire represent a unique lineage derived from cells expressing claudin. Nat Immunol 2007; 8:304-11; PMID:17277780; http://dx.doi.org/10.1038/ni1438
- Zuklys S, Balciunaite G, Agarwal A, Fasler-Kan E, Palmer E, Hollander GA. Normal thymic architecture and negative selection are associated with Aire expression, the gene defective in the autoimmune-polyendocrinopathy-candidiasis-ectodermal dystrophy (APECED). J Immunol 2000; 165:1976-83; PMID:10925280; http://dx.doi.org/10.4049/jimmunol.165.4.1976
- Baik S, Jenkinson EJ, Lane PJ, Anderson G, Jenkinson WE. Generation of both cortical and Aire(+) medullary thymic epithelial compartments from CD205(+) progenitors. Eur J Immunol 2013; 43:589-94; PMID:23299414; http://dx.doi.org/10.1002/eji.201243209
- Ribeiro AR, Rodrigues PM, Meireles C, Di Santo JP, Alves NL. Thymocyte selection regulates the homeostasis of IL-7-expressing thymic cortical epithelial cells in vivo. J Immunol 2013; 191:1200-9; PMID:23794633; http://dx.doi.org/10.4049/jimmunol.1203042
- Ohigashi I, Zuklys S, Sakata M, Mayer CE, Zhanybekova S, Murata S, Tanaka K, Hollander GA, Takahama Y. Aire-expressing thymic medullary epithelial cells originate from beta5t-expressing progenitor cells. Proc Natl Acad Sci U S A 2013; 110:9885-90; PMID:23720310; http://dx.doi.org/10.1073/pnas.1301799110
- Sun G, Shi YB. Thyroid hormone regulation of adult intestinal stem cell development: mechanisms and evolutionary conservations. Int J Biol Sci 2012; 8:1217-24; PMID:23136549; http://dx.doi.org/10.7150/ijbs.5109
- Pinto D, Gregorieff A, Begthel H, Clevers H. Canonical Wnt signals are essential for homeostasis of the intestinal epithelium. Genes Dev 2003; 17:1709-13; PMID:12865297; http://dx.doi.org/10.1101/gad.267103
- Spradling A, Drummond-Barbosa D, Kai T. Stem cells find their niche. Nature 2001; 414:98-104; PMID:11689954; http://dx.doi.org/10.1038/35102160
- Baraniak PR, McDevitt TC. Scaffold-free culture of mesenchymal stem cell spheroids in suspension preserves multilineage potential. Cell Tissue Res 2012; 347:701-11; PMID:21833761; http://dx.doi.org/10.1007/s00441-011-1215-5
- Langenbach F, Naujoks C, Smeets R, Berr K, Depprich R, Kubler N, Handschel J. Scaffold-free microtissues: differences from monolayer cultures and their potential in bone tissue engineering. Clin Oral Investig 2013; 17:9-17; PMID:22695872; http://dx.doi.org/10.1007/s00784-012-0763-8
- Sharma MB, Limaye LS, Kale VP. Mimicking the functional hematopoietic stem cell niche in vitro: recapitulation of marrow physiology by hydrogel-based three-dimensional cultures of mesenchymal stromal cells. Haematologica 2012; 97:651-60; PMID:22058199; http://dx.doi.org/10.3324/haematol.2011.050500
- Willerth SM, Sakiyama-Elbert SE. Combining stem cells and biomaterial scaffolds for constructing tissues and cell delivery. StemBook, ed. The Stem Cell Research Community. Cambridge (MA), 2008
- Liu X, Zhang G, Hou C, Wang H, Yang Y, Guan G, Dong W, Gao H, Feng Q. Vascularized bone tissue formation induced by fiber-reinforced scaffolds cultured with osteoblasts and endothelial cells. Biomed Res Int 2013; 2013:854917; PMID:24369019; http://dx.doi.org/10.1155/2013/854917
- Rauh J, Milan F, Gunther KP, Stiehler M. Bioreactor systems for bone tissue engineering. Tissue Eng Part B Rev 2011; 17:263-80; PMID:21495897; http://dx.doi.org/10.1089/ten.teb.2010.0612
- Mabvuure N, Hindocha S, Khan WS. The role of bioreactors in cartilage tissue engineering. Curr Stem Cell Res Ther 2012; 7:287-92; PMID:22563665; http://dx.doi.org/10.2174/157488812800793018
- Berry JL, Steen JA, Koudy Williams J, Jordan JE, Atala A, Yoo JJ. Bioreactors for development of tissue engineered heart valves. Ann Biomed Eng 2010; 38:3272-9; PMID:20820920; http://dx.doi.org/10.1007/s10439-010-0148-6
- Auger FA, Gibot L, Lacroix D. The pivotal role of vascularization in tissue engineering. Annu Rev Biomed Eng 2013; 15:177-200; PMID:23642245; http://dx.doi.org/10.1146/annurev-bioeng-071812-152428
- Tourneur E, Chassin C. Neonatal immune adaptation of the gut and its role during infections. Clin Dev Immunol 2013; 2013:270301; PMID:23737810; http://dx.doi.org/10.1155/2013/270301
- Tsubouchi S, Leblond CP. Migration and turnover of entero-endocrine and caveolated cells in the epithelium of the descending colon, as shown by radioautography after continuous infusion of 3H-thymidine into mice. Am J Anat 1979; 156:431-51; PMID:525623; http://dx.doi.org/10.1002/aja.1001560403
- Merani S, Toso C, Emamaullee J, Shapiro AM. Optimal implantation site for pancreatic islet transplantation. Br J Surg 2008; 95:1449-61; PMID:18991254; http://dx.doi.org/10.1002/bjs.6391
- Bartholomeus K, Jacobs-Tulleneers-Thevissen D, Shouyue S, Suenens K, In't Veld PA, Pipeleers-Marichal M, Pipeleers DG, Hellemans K. Omentum is better site than kidney capsule for growth, differentiation, and vascularization of immature porcine beta-cell implants in immunodeficient Rats. Transplantation 2013; 96(12):1026-33; PMID:24056625; http://dx.doi.org/10.1097/TP.0b013e3182a6ee41
- Kim HI, Yu JE, Park CG, Kim SJ. Comparison of four pancreatic islet implantation sites. J Korean Med Sci 2010; 25:203-10; PMID:20119571; http://dx.doi.org/10.3346/jkms.2010.25.2.203
- Ellis H. The clinical significance of adhesions: focus on intestinal obstruction. Eur J Surg Suppl 1997:5-9
- DeWard AD, Komori J, Lagasse E. Ectopic transplantation sites for cell-based therapy. Current opinion in organ transplantation 2014; 19:169-74; PMID:24480968; http://dx.doi.org/10.1097/MOT.0000000000000050
- Lawenda BD, Mondry TE, Johnstone PA. Lymphedema: a primer on the identification and management of a chronic condition in oncologic treatment. CA Cancer J Clin 2009; 59:8-24; PMID:19147865; http://dx.doi.org/10.3322/caac.20001
- Weir GC. Cellular transplantation into lymph nodes may not be such a crazy idea. Cell Stem Cell 2012; 11:587-8; PMID:23122285; http://dx.doi.org/10.1016/j.stem.2012.10.004