ABSTRACT
Periodontal ligament stem cells (PDLSCs) are tissue-specific mesenchymal stem cells (MSCs), having an important role in regenerative therapy for teeth loss. Interleukin-7 (IL-7) is a key cytokine produced by stromal cells including MSCs, and exhibits specific roles for B and T cell development and osteoblasts differentiation of multiple myeloma. However, the effect of IL-7 on osteogenic differentiation of PDLSCs remains unclear. Therefore, in the present study we determined whether IL-7 affects the proliferation and osteogenic differentiation of PDLSCs in vitro and explored the associated signaling pathways for IL-7-mediated cell differentiation. The results demonstrated that the isolated human PDLSCs possessed MSCs features, highly expressing CD90, CD44, CD105, CD29 and CD73, and almost did not expressed CD34, CD45, CD11b, CD14 and CD117. IL-7 could not significantly affect the proliferation of PDLSCs, but it decreased their osteogenic differentiation and inhibited alkaline phosphatase (ALP) activity. The results of quantitative reverse transcription-polymerase chain reaction (qRT-PCR) and Western blotting exhibited that the expression levels of Runx-2, SP7 and osteocalcin (OCN) were significantly reduced by IL-7. Further studies indicated that IL-7 did not significantly change JNK, ERK1/2 and p38 protein production, but markedly suppressed their phosphorylation levels. These data suggest that IL-7 inhibits the osteogenic differentiation of PDLSCs probably via inactivation of mitogen-activated protein kinase (MAPK) signaling pathway.
INTRODUCTION
Increasing studies have indicated that the cells of periodontal ligament (PDL) tissues have the capacity to form a complete periodontal attachment apparatus that is generally destroyed by periodontitis which is a chronic inflammatory and infectious disease leading to progressive damage including teeth loss if not treated.Citation1-3 PDL, which is a connective tissue between alveolar bone and cementum,Citation4 contains various types of cells including undifferentiated stem cells, fibroblasts, epithelial cells, endothelial cells, and abundance of blood vessels.Citation5-7 Previous studies have indicated that the regenerative capacity of PDL is closely associated with mesenchymal stem cells (MSCs) that have been recognized to reside within human PDL tissue.Citation8,9 PDL stem cells (PDLSCs) represent a population of somatic stem cells of mesenchymal origin, and they were identified as MSCs in 2004.Citation10 PDLSCs are committed to several development lineages, and possess an ability for self-renewal and multipotent differentiation, such as osteoblasts, adipocytes, neurocytes and chondrocytes,Citation11,12 and play an important role in maintaining homeostasis and promote the regeneration of periodontal tissue.Citation5,13 Currently, it is clear that PDLSCs are responsible for the physiological remodeling and therapeutic regeneration of periodontium.Citation6,14 Therefore, PDLSCs are possibly the best and first choice for use in periodontal regenerative therapies.Citation15
Previous studies have indicated that interleukin-7 (IL-7) is a key cytokine during B cell development and is secreted by stromal cells in the bone marrow, fetal liver, thymus and spleen.Citation16 It is established that IL-7 is also required for T cell development in the thymus.Citation17 Additionally, IL-7 produced by bone marrow stromal cells was exhibited to block osteoblast formation by decreasing the activity of Runx-2/Cbfa1 that is a critical transcription factor required for osteoblast differentiation.Citation18-20 The potential involvement of IL-7 in inhibitory effects on osteoblast differentiation has been supported by the decreased osteoblast colony-forming units (CFU-OB) formation in human bone marrow cultures and reduced Runx-2/Cbfal activity in human osteoprogenitor cells.Citation21 The mechanisms responsible for the inhibited osteoblast activity in myeloma are just beginning to be understood.Citation20 However, how IL-7 affects the osteogenic differentiation of PDLSCs remains unclear.
The primary aim of this study was to investigate the ability of IL-7 to modulate the proliferation and osteogenic differentiation of PDLSCs from healthy subjects. We also explored the possible roles of mitogen-activated protein kinase (MAPK) signaling pathway in the IL-7-induced osteoblast differentiation of PDLSCs.
RESULTS
PDLSCs morphology and phenotypic analyses
The cultured human primary PDLSCs demonstrated a spindle-like morphology with elongated cytoplasmic processes (). The results of flow cytometry analysis indicated that the isolated PDLSCs highly expressed CD90 (98.12%), CD44 (95.81%), CD105 (96.37%), CD29 (99.08%) and 73 (97.24%), which all belongs to the surface markers of MSCs, and almost did not express haematopoietic stem cell markers (), such as CD34 (0.34%), CD45 (0.41%), CD11b (0.23%), CD14 (0.46%) and CD117 (0.25%). These findings confirmed the MSCs features of the isolated PDLSCs.
FIGURE 1. Morphologic analysis and phenotypic identification of PDLSCs. (A) Morphology of PDLSCs which demonstrated spindle-like shape. Scale bar: 100 µm. (B) Cell surface antigens of PDLSCs at passage 2 were detected by flow cytometry. The percentages of cell surface CD markers were shown in the histograms.
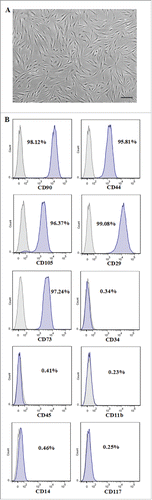
Effect of IL-7 on the proliferation of PDLSCs
The effect of IL-7 treatment on cell proliferation was evaluated by MTT assay. As shown in , the results demonstrated that the addition of 10 or 20 ng/ml IL-7 in culture medium did not significantly affect PDLSCs proliferation after cultivation of 24, 48, 72 and 96 h, when compared to the untreated control.
IL-7 inhibits osteogenic differentiation of PDLSCs
To investigate the effect of IL-7 on osteogenic differentiation of PDLSCs, the cells were cultivated in osteogenic culture medium in the presence of IL-7 (0, 10 and 20 ng/ml). After two weeks of induction, ALP as an early osteogenesis marker, its activity was detected. As shown in , ALP activity was significantly decreased by increasing concentrations of IL-7, which indicates that IL-7 treatment suppressed osteogenic differentiation of PDLSCs. This inhibitory effect was further confirmed by Alizarin red staining which demonstrated that calcium deposition and mineralized nodules of PDLSCs were markedly reduced after induction of 3 weeks when compared to the untreated cells (). Additionally, the sensitivity of the colorimetric method obtained by the extraction of the calcified mineral demonstrated that a decreased absorbance value was observed with increasing concentrations of IL-7 (), which was consistent with the results of Alizarin red staining. On the other hand, the expression of osteogenic related genes and proteins such as Runx-2, SP7 and OCN was determined by qRT-PCR and western blotting. The results exhibited that the expression levels of Runx-2, SP-7 and OCN mRNA and proteins were markedly reduced by IL-7 at a dose-dependent manner (0, 10 and 20 ng/ml) after 2 weeks of osteogenic induction ().
FIGURE 3. IL-7 suppresses osteogenic differentiation of PDLSCs. After osteogenic induction culture for 2 or 3 weeks with or without IL-7 (10 and 20 ng/ml), ALP activity and calcium deposition and extracellular matrix mineralization were evaluated, respectively. (A) ALP activity was quantified and expressed relative to the untreated cells, to which an arbitrary value of 1 was given. (B) Representative images of Alizarin red S staining. (C) Alizarin red S staining (B) was extracted and, the amount of released dye was then measured by a spectrophotometer at 562 nm for absorbance value. Data are presented as mean ± SD, *P < 0.05 and **P < 0.01 compared with the control (0 ng/ml of IL-7). #P < 0.05 compared with the group (10 ng/ml of IL-7).
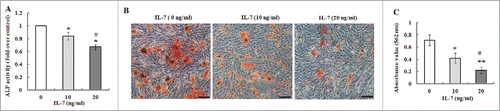
FIGURE 4. Effects of IL-7 on the expression levels of Runx-2, SP7 and OCN. (A-C) Gene expression of Runx-2, SP7 and OCN in PDLSCs was determined by qRT-PCR after osteogenic induction culture with 10 and 20 ng/ml of IL-7 for 2 weeks. (D) Representative Western blot images demonstrated that protein expression of Runx-7, SP7 and OCN in PDLSCs was measured by Western blotting after osteogenic induction with 10 and 20 ng/ml of IL-7 for 2 weeks of culture. (E) Relative protein levels of Runx-2, SP7 and OCN were quantified by the densitometry of each band normalized to β-actin signal. Data are presented as mean ± SD, *P < 0.05 and **P < 0.01 compared with the control (0 ng/ml of IL-7). #P < 0.05 compared with the group (10 ng/ml of IL-7).
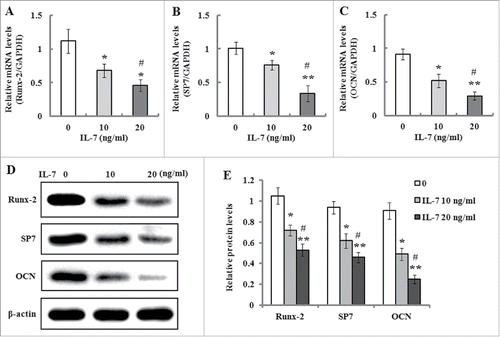
IL-7 inhibits the activation of MAPK signaling
Previous studies have indicated MAPK signal pathways play an important role in the regulation of osteoblasts differentiation.Citation22 Therefore, we detected the protein levels of JNK, ERK1/2, p38 and their phosphorylated forms by western blotting after the cells were incubated in serum-free medium with IL-7 (0, 10 and 20 ng/ml) for 48 h. As shown in , the results demonstrated that IL-7 significantly down-regulated the phosphorylation levels of JNK, ERK1/2 and p38, but it did not change the protein production of JNK, ERK1/2 and p38, which suggests that MAPK signaling probably involved in IL-7-mediated inhibition of osteogenic differentiation of PDLSCs.
FIGURE 5. IL-7 inhibits the MAPK signaling in PDLSCs. PDLSCs were stimulated with IL-7 (0, 10 and 20 ng/ml) for 48 h, and total protein was extracted and subjected to Western blot analysis. (A) Representative western blot images demonstrating a markedly inhibitory role on the phosphorylation levels of JNK, ERK1/2 and p38, but not their protein levels. (B) Relative protein levels of JNK, ERK1/2, p38, and their phosphorylated levels were quantified by the densitometry of each band normalized to β-actin signal. Data are presented as mean ± SD, *P < 0.05 and **P < 0.01 compared with the control (0 ng/ml of IL-7). #P < 0.05 compared with the group (10 ng/ml of IL-7).
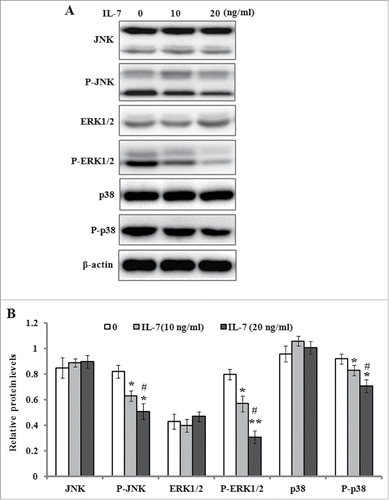
DISCUSSION
Mesenchymal stem cells are multipotent progenitor cells capable of differentiation into osteoblast and maintain the bone homeostasis. Many studies have indicated that PDLSCs are a group of tissue specific MSCs derived from periodontal ligament,Citation23 and play an important role, not only in maintaining the homeostasis of periodontal tissue, but also in promoting periodontal regeneration.Citation24 Previous studies have shown the role of IL-7 in the inhibition of bone formation in multiple myeloma.Citation25 However, how osteogenic differentiation of PDLSCs is regulated by IL-7 still need to be explored. In the present study, we found that IL-7 did not significantly change proliferation ability of PDLSCs, but indeed inhibited their osteogenic differentiation, decreased ALP activity, and downregulated the expression levels of Runx-2, SP7 and OCN, which are osteogenic markers.
In this studies, the PDLSCs were isolated from healthy subjects, and their cell surface markers were detected by flow cytometry. The results demonstrated that these isolated PDLSCs expressed high levels of CD90, CD44, CD105, CD29 and 73, which are MSCs-related surface markers, but they were almost negative for CD34, CD45, CD11b, CD14 and CD117. These findings are in accordance with previous reports about the characteristics of PDLSCs.Citation26,27
It has been shown that PDLSCs are multipotent stem cells with features similar to MSCs, and have capacity to self-renew and differentiate into various cell lineages such as bone, adipose and neural cells.Citation28 In this study, the effect of IL-7 on osteogenic differentiation of PDLSCs was evaluated. Alizarin red staining demonstrated that IL-7 treatment apparently decreased the formation of mineralized nodules and calcium deposition. The activity of ALP, an early osteogenesis marker, was significantly reduced in the PDLSCs after osteogenic induction culture for 2 weeks in the presence of IL-7. Additionally, the expression of osteogenic differentiation related genes and proteins Runx-2, SP7 and OCN was also downregulated after osteogenic induction with IL-7 addition. Runx-2/cbfa1 is a critical transcription factor responsible for regulating the formation and differentiation of osteoblastic cells from bone marrow mesenchymal/stromal cells in physiologic condition.Citation29,30 It has been indicated that in preosteoblastic cells and bone marrow stromal cells, Runx-2/cbfa1 activation induces osteogenic markers expression, such as collagen, alkaline phosphatase, and OCN during the various stages of osteoblast maturation.Citation31 Previous studies have demonstrated that IL-7 reduces the promoter activity of Runx-2/cbfa1 in osteoblastic cells, and may act as an uncoupled factor to stimulate bone resorption or inhibit bone formation.Citation21 In the present studies, Runx-2 expression was significantly reduced by IL-7 at transcriptional and translational levels, which suggests that the osteogenic differentiation of PDLSCs was blocked during the osteogenic induction culture.
Previous studies have demonstrated that MAPK activation is necessary for extracellular matrix-dependent stimulation of the bone marker, alkaline phosphatase.Citation32 It is well-known that MAPK signaling pathway mainly consists of JNK, ERK1/2, and p38. To further investigate the mechanism by which IL-7 suppressed the osteogenic differentiation of PDLSCs, we detected the protein levels of JNK, ERK1/2, p38, and their phosphorylated forms. The results indicated that IL-7 did not significantly change the expression levels of JNK, ERK1/2, and p38, but it markedly reduced the phosphorylated levels of JNK, ERK1/2, and p38 after osteogenic induction culture. Recently, it has been demonstrated that ERK1/2 and p38 phosphorylation is involved in the induction and control of transcriptional activity of Runx-2 and Osterix that are required for osteoblast differentiation and bone formation.Citation33 Extracellular matrix plays an important role in cell proliferation, differentiation, and migration, and its stiffness was found to regulate MSCs osteogenic differentiation through JNK or ERK activation.Citation34 It has been reported that the activation of JNK and p38 leads to Runx-2 and Osterix expression, and promotes MSCs osteogenesis.Citation35 In the present study, IL-7 significantly reduced the phosphorylated levels of JNK, ERK1/2, and p38, which indicated IL-7 inhibited osteogenic differentiation of PDLSCs possibly through inactivation of MAPK signaling pathway. It should be noted that there are some limitations in this study. Firstly, we did not detect the effect of IL-7 on bone formation in animals, although we found its inhibitory role in osteogenic differentiation of PDLSCs in vitro. Additionally, we did not determine the levels of IL-7 in the blood sera of patients with periodontal disease. It has been demonstrated that an elevation of IL-7 levels has been observed in circulatory environment of patients with multiple myeloma, and its inhibitory effects on osteoblast differentiation were confirmed in these patients with multiple myeloma.Citation18 On the other hand, it is necessary to detect the expression level of IL-7 receptor and its downstream signaling because the presence of IL-7 receptor has been shown in human bone marrow stromal cells.Citation36 The above mysteries should be further investigated in future work.
In summary, our data indicate that IL-7 has no effect on the proliferation of human PDLSCs, but it markedly inhibits the osteogenic differentiation of human PDLSCs, decreases ALP activity, and downregulates the expression of Runx-2, SP7, and OCN genes and proteins possibly through suppressing the phosphorylation of JNK, ERK1/2, and p38 proteins. This study may represent the starting point for further investigating the role of IL-7 in vivo.
MATERIALS AND METHODS
Isolation and culture of PDLSCs
PDLSCs were isolated from 4 healthy subjects (age 21–28 years) who donated their normal impacted third molars for orthodontic purpose. The informed consent was signed by every donor. The experimental protocol and informed consent were reviewed and approved by the Ethics Committee of PLA General Hospital of Chengdu Military Region (Chengdu, China). The periodontal ligament tissues were washed with sterile phosphate-buffered saline (PBS), and gently scraped from the tooth root surface, and cut into small peaces (1–2 mm3) with scissors, and then transferred to 25 cm2 culture flasks with DMEM culture medium (Gibco, Life Technologies) added with 10% fetal bovine serum (FBS), 100 U/ml penicillin and 100 mg/ml streptomycin. After culture of 2 weeks, the cells migrated from the explant tissues reached about 80% confluence, and their morphology was observed and captured using an inverted phase contrast microscope with a Nikon digital camera (Tokyo, Japan), followed by trypsinization and subculture until passage 6. The passage 2–6 (P2-P6) were used to the following study.
PDLSCs immunophenotype
To confirm the mesenchymal stem cells (MSCs) nature of isolated PDLSCs, we detected the positive markers: CD90, CD44, CD105, CD29, and CD73, and negative markers: CD34, CD45, CD11b, CD14, and CD117 by flow cytometry. Briefly, the cells were harvested, and incubated for 30 min in the dark with the following fluorescein isothiocyanate (FTIC)-conjugated or phycoerythrin (PE)-conjugated monoclonal antibodies: anti-CD90, anti-CD44, anti-CD105, anti-CD29, anti-CD73, anti-CD34, anti-CD45, anti-CD11b, anti-CD14, and anti-CD117 which all obtained from Cell Signal Technology (USA). A nonspecific phycoerythrin-conjugated IgG was used evaluate background fluorescence. After incubation, CD markers of PDLSCs were determined using flow cytometry with CellQestPro acquisition software.
Cell proliferation assay
MTT (3-(3,4-Dimethylthiazol-2-yi)-2,5-diphenyltetrasolium bromide) assay was used to detect the effect of IL-7 on cell proliferation. Briefly, PDLSCs were seeded into 96-well culture plates at a density of 1 × 104 cells/well, and incubated for 24 h in the culture medium. Recombinant human IL-7 (R&D System, Abingdon, UK) at increasing concentrations (0, 10, 20 ng/ml) was then added to the culture for 0, 24, 48, 72, and 96 h, followed by addition of MTT (5 mg/ml) into each well for 4 h incubation. Then, culture medium was removed, and 150 µl of dimethyl sulfoxide were added into each well for dissolution of formazan crystals. Absorbance value at 570 nm was then determined using automatic reader for microtiter plates (PerkinElmer, USA). The tests were performed at least 3 times. Data are denoted as a percentage of the value for the corresponding control cultured without IL-7 treatment (0 ng/ml of IL-7).
Osteogenic differentiation assays
The effect of IL-7 on osteogenic differentiation of PDLSCs was evaluated. Briefly, The cells at P3 were seeded into 6-well plates at a density of 1 × 105 cells/well, and cultured in osteogenic induction medium containing DMEM culture medium, 10% FBS, 50 mM ascorbate-2-phosphate (Sigma-Aldrich, USA), 10 mM β-glycerophosphate (Sigma-Aldrich), 100 nM dexamethasone (Sigma-Aldrich). After 24 h incubation, IL-7 (0, 10 and 20 ng/ml) was added into the osteogenic induction medium for continuous culture of 2 or 3 weeks. The osteogenic differentiation was separately assessed by the detection of alkaline phosphatase (ALP) activity, Alizarin red staining assay, and quantitative reverse transcription polymerase chain reaction (RT-PCR) analyses and Western blot analyses.
ALP activity detection and Alizarin red staining assay
ALP activity was determined using a commercially available assay kit (Zhongshan, Beijing, China) according to the manufacturer's instructions. Briefly, after 2 weeks of induction culture, PDLSCs were washed 3 times with PBS, followed by addition with pnitrophenol phosphate substrate solution (100 µl/well in 96-well plates) and incubation for 30 min at 37°C. The reaction was then stopped by the addition of 2 M NaOH to reaction mixture. Enzyme activity was quantified by absorbance at 405 nm using a microplate reader. In addition, to evaluate calcium nodules deposition and extracellular matrix mineralization, the cells were fixed with 4% paraformaldehyde after 3 weeks of induction culture, and stained 2% Alizarin red for 20 min at room temperature after 3 weeks of osteogenic induction. For Alizarin red quantification, 1 ml of 10% cetylpyridinium chloride was added to each well as described previously.Citation37 Light absorbance of the extracted dye was determined at 562 nm using a spectrophotometer (NanoDrop Technologies, DE, USA). The experiments were performed 3 times.
Quantitative RT-PCR
After 14 d of induction culture, the cells were harvested, and the total RNA from each sample was extracted using Trizol reagent (Invitrogen, CA, USA) following the manufacturer's instructions. cDNA was reversely synthesized using OligodT primer and the Superscript First-Strand Synthesis System (Invitrogen, CA, USA) for RT-PCR according to the manufacturer's instructions. The specific primers of Runx-2, SP7 OCN, and GAPDH were as follows: Runx-2 forward5′-CCCGTGGCCTTCAAGGT
T-3′, and reverse 5′-ATGACAGTACCGCCCATTGC-3′; SP7 forward5′-GCCAGAA
GCTGTGAAACCTC-3′, and reverse 5′-GCTGCAAGCTCTCCATAACC-3′; OCN forward 5′-CACTCCTCGCCCTATTGGC-3′, and reverse 5′-CCCTCCTGCTTGGAC
ACAAAG-3′; GAPDH forward 5′-ACCCAGTCCATGCCATCAC-3′, and reverse 5′-TCCACCACCCTGTTGCTGTA-3′. Quantitative RT-PCR was performed with SYBR® Premix Ex Taq™ (Takara, Japan) on a LightCycler® (Bio-Rad, CA, USA). The relative expression levels of targeted genes were calculated by normalizing to the GAPDH. Quantitative RT-PCR was performed in 3 independent experiments.
Western blot analysis
Total proteins were extracted from the cells with lysis buffer (1% sodium dodecyl sulfate, 10 nM tris-HCl, 1% Nonidet P-40, 1 nM ethylenediaminetetraacetic acid (EDTA), 50 Nm β-glycerophosphate, 1:100 proteinase inhibitor cocktail, 50 nM sodium fluoride) (Roche Applied Science, Monza, Italy). After the protein concentration was determined with BCA assay kit (Beyotime, China, 20 µg protein from each sample were separated on 12% sodium dodecyl sulfate polyacrylamide gel (SDS-PAGE) and electrotransferred onto polyvinylidene difluoride (PVDF) membranes, followed by blocking in 5% fat-free dry milk in TBST (0.1 M Tris-buffered saline-0.1% Tween-20) buffer for 2 h. Then, the membranes were incubated with the following primary antibodies: anti-Runx-2 (dilution,1:500) (Catalog#= ab76956, abcam, Cambridge, UK), anti-SP7 (dilution, 1: 500) (Catalog#= ab22552, abcam, Cambridge, UK), anti-OCN (dilution, 1: 500) (Catalog#= ab13421, abcam, Cambridge, UK), and β-actin (dilution, 1:1000) (Catalog#= ab8226, abcam, Cambridge,UK) overnight at 4°C, followed by incubation with horseradish peroxidase-conjugated secondary antibody for 1 h. Protein bands were visualized with an enhanced chemiluminescence reagent (Amersham Biosciences, NJ, USA), and quantified by densitometric scanning and analyses using an ImageQuant LAS 4000 (Fujifilm, Tokyo, Japan), and expressed as a relative ratio to β-actin protein.
Statistical analysis
Data were presented as the mean ± SD. The comparisons among different groups were performed by one-way ANOVA followed by post-hoc analysis the Scheffe test using a software SPSS (version 17.0, Chicago, USA). P value < 0.05 was considered statistically significant.
DISCLOSURE OF POTENTIAL CONFLICTS OF INTEREST
No potential conflicts of interest were disclosed.
ACKNOWLEDGMENT
We would like to thank Dr. Kunlas Karsenty for English proof reading of this manuscript.
Funding
This work was supported by China Postdoctoral Science Foundation (2015M582908).
REFERENCES
- Catón J, Bostanci N, Remboutsika E, De Bari C, Mitsiadis TA. Future dentistry: cell therapy meets tooth and periodontal repair and regeneration. J Cell Mol Med 2011; 15:1054-65; http://dx.doi.org/10.1111/j.1582-4934.2010.01251.x
- Zhu B, Liu Y, Li D, Jin Y. Somatic stem cell biology and periodontal regeneration. Int J Oral Maxillofac Implants 2013; 28:e494-502; PMID:24278958; http://dx.doi.org/10.11607/jomi.te30
- Lu H, Xie C, Zhao YM, Chen FM. Translational research and therapeutic application of stem cell transplantation in periodontal regenerative medicine. Cell Transplant 2013; 22:205-29; PMID:23031442; http://dx.doi.org/10.3727/096368912X656171
- Fujita T, Iwata T, Shiba H, Igarashi A, Hirata R, Takeda K, Mizuno N, Tsuji K, Kawaguchi H, Kato Y, Kurihara H. Identification of marker genes distinguishing human periodontal ligament cells from human mesenchymal stem cells and human gingival fibroblasts. J Periodontal Res 2007; 42:283-6; PMID:17451549; http://dx.doi.org/10.1111/j.1600-0765.2006.00944.x
- Feng F, Akiyama K, Liu Y, Yamaza T, Wang TM, Chen JH, Wang BB, Huang GT, Wang S, Shi S. Utility of PDL progenitors for in vivo tissue regeneration: a report of 3 cases. Oral Dis 2010; 16:20-8; PMID:20355278; http://dx.doi.org/10.1111/j.1601-0825.2009.01593.x
- Bright R, Hynes K, Gronthos S, Bartold PM. Periodontal ligament-derived cells for periodontal regeneration in animal models: a systematic review. J Periodontal Res 2015; 50:160-72; PMID:24965968; http://dx.doi.org/10.1111/jre.12205
- Maeda H, Tomokiyo A, Koori K, Monnouchi S, Fujii S, Wada N, Kono K, Yamamoto N, Saito T, Akamine A. An in vitro evaluation of two resin-based sealers on proliferation and differentiation of human periodontal ligament cells. Int Endod J 2011; 44:425-31; PMID:21255042; http://dx.doi.org/10.1111/j.1365-2591.2010.01845.x
- Yan XZ, Yang F, Jansen JA, de Vries RB, van den Beucken JJ. Cell-based approaches in periodontal regeneration: a systematic review and meta-analysis of periodontal defect models in animal experimental work. Tissue Eng Part B Rev 2015; 21:411-26; PMID:25929285; http://dx.doi.org/10.1089/ten.teb.2015.0049
- Chen FM, Sun HH, Lu H, Yu Q. Stem cell-delivery therapeutics for periodontal tissue regeneration. Biomaterials 2012; 33:6320-44; PMID:22695066; http://dx.doi.org/10.1016/j.biomaterials.2012.05.048
- Seo BM, Miura M, Gronthos S, Bartold PM, Batouli S, Brahim J, Young M, Robey PG, Wang CY, Shi S. Investigation of multipotent postnatal stem cells from human periodontal ligament. Lancet 2004; 364:149-55; PMID:15246727; http://dx.doi.org/10.1016/S0140-6736(04)16627-0
- Cha Y, Jeon M, Lee HS, Kim S, Kim SO, Lee JH, Song JS. Effects of in vitro osteogenic induction on in vivo tissue regeneration by dental pulp and periodontal ligament stem cells. J Endod 2015; 41:1462-8; PMID:26001856; http://dx.doi.org/10.1016/j.joen.2015.04.010
- Park JC, Kim JM, Jung IH, Kim JC, Choi SH, Cho KS, Kim CS. Isolation and characterization of human periodontal ligament (PDL) stem cells (PDLSCs) from the inflamed PDLtissue: in vitro and in vivo evaluations. J Clin Periodontol 2011; 38:721-31; PMID:21449989; http://dx.doi.org/10.1111/j.1600-051X.2011.01716.x
- Chen FM, Gao LN, Tian BM, Zhang XY, Zhang YJ, Dong GY, Lu H, Chu Q, Xu J, Yu Y, Wu RX, Yin Y, Shi S, Jin Y. Treatment of periodontal intrabony defects using autologous periodontal ligament stem cells: a randomized clinical trial. Stem Cell Res Ther 2016; 7:33; PMID:26895633; http://dx.doi.org/10.1186/s13287-016-0288-1
- Nuñez J, Sanz-Blasco S, Vignoletti F, Muñoz F, Arzate H, Villalobos C, Nuñez L, Caffesse RG, Sanz M. Periodontal regeneration following implantation of cementum and periodontal ligament-derived cells. J Periodontal Res 2012; 47:33-44; http://dx.doi.org/10.1111/j.1600-0765.2011.01402.x
- Trubiani O, Orsini G, Zini N, Di Iorio D, Piccirilli M, Piattelli A, Caputi S. Regenerative potential of human periodontal ligament derived stem cells on three-dimensional biomaterials: a morphological report. J Biomed Mater Res A 2008; 87:986-93; PMID:18257082; http://dx.doi.org/10.1002/jbm.a.31837
- Parrish YK, Baez I, Milford TA, Benitez A, Galloway N, Rogerio JW, Sahakian E, Kagoda M, Huang G, Hao QL, et al. IL-7 Dependence in human B lymphopoiesis increases during progression of ontogeny from cord blood to bone marrow. J Immunol 2009; 182:4255-66; PMID:19299724; http://dx.doi.org/10.4049/jimmunol.0800489
- Chen X, Fang L, Song S, Guo TB, Liu A, Zhang JZ. Thymic regulation of autoimmune disease by accelerated differentiation of Foxp3+ regulatory T cells through IL-7 signaling pathway. J Immunol 2009; 183:6135-44; PMID:19841165; http://dx.doi.org/10.4049/jimmunol.0901576
- Nierste BA, Glackin CA, Kirshner J. Dkk-1 and IL-7 in plasma of patients with multiple myeloma prevent differentiation of mesenchymal stem cells into osteoblasts. Am J Blood Res 2014; 4:73-85; PMID:25755907
- Giuliani N, Rizzoli V, Roodman GD. Multiple myeloma bone disease: pathophysiology of osteoblast inhibition. Blood 2006; 108:3992-6; PMID:16917004; http://dx.doi.org/10.1182/blood-2006-05-026112
- Silbermann R, Roodman GD. Myeloma bone disease: pathophysiology and management. J Bone Oncol 2013; 2:59-69; PMID:26909272; http://dx.doi.org/10.1016/j.jbo.2013.04.001
- Weitzmann MN, Roggia C, Toraldo G, Weitzmann L, Pacifici R. Increased production of IL-7 uncouples bone formation from bone resorption during estrogen deficiency. J Clin Invest 2002; 110:1643-50; PMID:12464669; http://dx.doi.org/10.1172/JCI0215687
- Xiao G, Jiang D, Thomas P, Benson MD, Guan K, Karsenty G, Franceschi RT. MAPK pathways activate and phosphorylate the osteoblast-specific transcription factor, Cbfa1. J Biol Chem 2000; 275:4453-9; PMID:10660618; http://dx.doi.org/10.1074/jbc.275.6.4453
- Li B, Sun J, Dong Z, Xue P, He X, Liao L, Yuan L, Jin Y. GCN5 modulates osteogenic differentiation of periodontal ligament stem cells through DKK1 acetylation in inflammatory microenvironment. Sci Rep 2016; 6:26542; PMID:27216891; http://dx.doi.org/10.1038/srep26542
- Zhang C, Lu Y, Zhang L, Liu Y, Zhou Y, Chen Y, Yu H. Influence of different intensities of vibration on proliferation and differentiation of human periodontal ligament stem cells. Arch Med Sci 2015; 11:638-46; PMID:26170859; http://dx.doi.org/10.5114/aoms.2015.52370
- Giuliani N, Colla S, Morandi F, Lazzaretti M, Sala R, Bonomini S, Grano M, Colucci S, Svaldi M, Rizzoli V. Myeloma cells block RUNX2/CBFA1 activity in human bone marrow osteoblast progenitors and inhibit osteoblast formation and differentiation. Blood 2005; 106:2472-83; PMID:15933061; http://dx.doi.org/10.1182/blood-2004-12-4986
- Li B, Jung HJ, Kim SM, Kim MJ, Jahng JW, Lee JH. Human periodontal ligament stem cells repair mental nerve injury. Neural Regen Res 2013; 8:2827-37; PMID:25206604
- Wei F, Liu D, Feng C, Zhang F, Yang S, Hu Y, Ding G, Wang S. microRNA-21 mediates stretch-induced osteogenic differentiation in human periodontal ligament stem cells. Stem Cells Dev 2015; 24:312-9; PMID:25203845; http://dx.doi.org/10.1089/scd.2014.0191
- Trubiani O, Orsini G, Zini N, Di Iorio D, Piccirilli M, Piattelli A, Caputi S. Regenerative potential of human periodontal ligament derived stem cells on three-dimensional biomaterials: a morphological report. J Biomed Mater Res A 2008; 87:986-93; PMID:18257082; http://dx.doi.org/10.1002/jbm.a.31837
- Franceschi RT, Xiao G, Jiang D, Gopalakrishnan R, Yang S, Reith E. Multiple signaling pathways converge on the Cbfa1/Runx2 transcription factor to regulate osteoblast differentiation. Connect Tissue Res 2003; 44(suppl 1):109-116; PMID:12952183; http://dx.doi.org/10.1080/03008200390152188
- Komori T. Runx2, a multifunctional transcription factor in skeletal development. J Cell Biochem 2002; 87:1-8; PMID:12210716; http://dx.doi.org/10.1002/jcb.10276
- Shui C, Spelsberg TC, Riggs BL, Khosla S. Changes in Runx2/Cbfa1 expression and activity during osteoblastic differentiation of human bone marrow stromal cells. J Bone Miner Res 2003; 18:213-21; PMID:12568398; http://dx.doi.org/10.1359/jbmr.2003.18.2.213
- Artigas N, Ureña C, Rodríguez-Carballo E, Rosa JL, Ventura F. Mitogen-activated protein kinase (MAPK)-regulated interactions between Osterix and Runx2 are critical for the transcriptional osteogenic program. J Biol Chem 2014; 289:27105-17; PMID:25122769; http://dx.doi.org/10.1074/jbc.M114.576793
- Sinha KM, Yasuda H, Zhou X, deCrombrugghe B. Osterix and NO66 histone demethylase control the chromatin of Osterix target genes during osteoblast differentiation. J Bone Miner Res 2014; 29:855-65; PMID:24115157; http://dx.doi.org/10.1002/jbmr.2103
- Hwang JH, Byun MR, Kim AR, Kim KM, Cho HJ, Lee YH, Kim J, Jeong MG, Hwang ES, Hong JH. Extracellular matrix stiffness regulates osteogenic differentiation through MAPK activation. PLoS One 2015; 10:e0135519; PMID:26262877; http://dx.doi.org/10.1371/journal.pone.0135519
- Zhang X, Zhou C, Zha X, Xu Z, Li L, Liu Y, Xu L, Cui L, Xu D, Zhu B. Apigenin promotes osteogenic differentiation of human mesenchymal stem cells through JNK and p38 MAPK pathways. Mol Cell Biochem 2015; 407:41-50; PMID:25994505; http://dx.doi.org/10.1007/s11010-015-2452-9
- Iwata M, Graf L, Awaya N, Torok-Storb B. Functional interleukin-7 receptors (IL-7Rs) are expressed by marrow stromal cells: binding of IL-7 increases levels of IL-6 mRNA and secreted protein. Blood 2002; 100:1318-25; PMID:12149213; http://dx.doi.org/10.1182/blood-2002-01-0062
- Manescu A, Giuliani A, Mohammadi S, Tromba G, Mazzoni S, Diomede F, Zini N, Piattelli A, Trubiani O. Osteogenic potential of dual blocks cultured with human periodontal ligament stem cells: in vitro and synchrotron microtomography study. J Periodontal Res 2016; 51:112-24; PMID:26094874; http://dx.doi.org/10.1111/jre.12289