ABSTRACT
Stem cell research has significantly evolved over the last few years, allowing the differentiation of pluripotent cells into almost any kind of lineage possible. Studies that focus on the liver have considerably taken a leap into this novel technology, and hepatocyte-like cells are being generated that are close to resembling actual hepatocytes both genotypically and phenotypically. The potential of this extends from disease models to bioengineering, and even also innovative therapies for end-stage liver disease. Nonetheless, too few attention has been given to the non-parenchymal cells which are also fundamental for normal liver function. This includes cholangiocytes, the cells of the biliary epithelium, without whose role in bile modification and metabolism would impair hepatocyte survival. Such can be observed in diseases that target them, so called cholangiopathies, for which there is much yet to study so as to improve therapeutical options. Protocols that describe the induction of human induced pluripotent stem cells into cholangiocytes are scarce, although progress is being achieved in this area as well. In order to give the current view on this emerging research field, and in hopes to motivate further advances, we present here a review on the known differentiation strategies with sight into future applications.
INTRODUCTION
Over the last two decades, the advances made in hepatic research have been substantial. Hepatocytes, the main cell line within the liver, have constituted a main target for researchers to study the mechanisms of disease development, regeneration, and recently the developing of functional liver tissue, even also bioengineered organs with the aim of solving many of the current liver transplant issues regarding the lack of donors. Nevertheless, there is still much to explore on the non-parenchymal cells, which might be of benefit considering that their main role concerns the supporting and enhancing of normal hepatic function.Citation1 Cholangiocytes belong to this group of cells and represent only 3% of the total liver cell population,Citation2 nonetheless, their role as the epithelium lining the biliary tree is vital for the processing of bile produced by hepatocytes. Cholangitic diseases not only are responsible for significant morbidity and mortality, but also account for the majority of pediatric liver transplants and a significant percentage of young adult liver transplants; unfortunately, at present there are few models to accurately replicate them and seek new therapeutic goals.Citation3 With the rise of stem cell research, the new ideal cell source to generate cholangiocytes in vitro is being generated both for disease modeling and for potential curative alternatives as well, which involves pluripotent stem cells such as embryonic stem cells (ESCs) and induced pluripotent stem cells (iPSCs) whose continuous proliferation and ability to differentiate into any of the three germ-layers is ideal for this purpose. Due to the lack of limitations that ESCs have shown, including immune rejection after transplantation and ethical concerns, iPSCs have appeared as an alternate and reproducible source of differentiated cells with therapeutic interest, making it even feasible to create patient-matched pluripotent stem cells.Citation4 In this review, we will describe what is currently known regarding specification, maturation of iPSCs derived cholangiocytes and future applications, whose field has been actively growing and will surely become a key component for future hepatic research in the basic and clinic area.
DIFFERENTIATION OF HUMAN IPSCS INTO CHOLANGIOCYTE-LIKE CELLS
Biliary system development
The development of the biliary system starts when the diverticulum, derived from the ventral foregut endoderm whose cranial part forms the intrahepatic biliary trees and the caudal part generates the extrahepatic bile ducts, extends into the septum transversum and develops the liver bud. To initiate the formation of the intrahepatic bile ducts, the bi-potential hepatic fate specified endoderm cells in the liver bud, which are also called hepatoblasts, proliferate and migrate into the septum transversum differentiate into both hepatocytes and cholangiocytes.Citation5 Subsequently, the biliary precursors interact with adjacent mesodermal tissues and transiently form an asymmetrical structure called the ductal plate which, in mouse embryos, is constituted on the portal side by a single layer of SRY-related HMG box transcription factor 9 (Sox9) and cytokeratin (CK)-19 positive cholangiocyte progenitors, whereas on the parenchymal side hepatocyte nuclear factor 4 (HNF4) positive hepatoblasts line next to them,Citation6 thus indicating the unique beginning of tubulogenesis. During the development of the ducts, all cells lining their lumen progressively acquire cholangiocyte morphology and function. Guided by the cell orientation system known as planar cell polarity, the biliary epitheliums uniformly maintain the tubular architecture and proliferate in length following a hilum-periphery axis, surrounded by periportal extracellular matrix and mesenchyme, which leads to postnatal development of hepatic artery branches.Citation7 Some ductal plate cells which do not participate in bile duct formation transdifferentiate and become the periportal hepatocytes, thus building the canals of Hering.Citation8 The formation of the extrahepatic bile duct system, which involves the hepatic ducts, the cystic duct, the gallbladder, and the common bile duct, initiates earlier and may be regulated by several distinct transcription factors and signaling pathways originating from the intrahepatic biliary trees.Citation5 Anatomically, these two ductal systems merge at the level of the hepatic ducts/hilum.
The network and pathways of the growth factors that control early biliary development, from cell fate determination to tubulogenesis, is not fully uncovered. Several essential proteins and signaling pathways which may play important roles in cholangitic specification and bile duct morphogenesis have been demonstrated. Li, et al.Citation9 suggests that by inhibiting the nuclear factor-kappa B-dependent pathway, which is specifically activated by interleukin (IL) −6, can terminate bile duct development through forkhead box A1 (FoxA1) and FoxA2 transcriptional systems in a mice model. The Notch signaling pathway, constituted by four known Notch receptors (Notch 1–4) and five ligands belonging to the Jagged (Jagged1 and 2) and Delta-like (Delta-like ligand [Dll]1, 3, and 4) family, is well known for its power to determine biliary cell fates and guide proper morphogenesis of the developing biliary tree.Citation10 Some previous studies about Alagille syndrome carried out in animal models showed that Jagged1/Notch2 interactions regulated potentially during tubulogenesis of the biliary tree after the formation of the ductal plate and initial lineage commitment of hepatoblasts to biliary cells.Citation11-14 Transforming growth factor beta (TGF-β) can also support cholangiocyte differentiation. TGF-β can stimulate cholangiocytes maturation around portal vein, where there is more expression of the TGF-β receptor type II, without HNF6 and OC2 control.Citation15 It was shown as well that canonical wingless (Wnt)/beta-catenin and its ligands, such as Wnt3a, induce biliary differentiation, since beta-catenin deficient mice develop impaired maturation, expansion, and survival of hepatoblasts.Citation16 Another important morphogenetic cue comes from Hedgehog signaling (Hh), by temporally promoting early hepatoblast proliferation; interestingly, mature cholangiocytes do not respond to these signals.Citation17
During later gestation and postnatally, owing to the maternal feeds, liver metabolism undergoes numerous modifications which may start to act as the main stimuli guiding biliary system maturation until reaching eventual full physiological capacity.Citation18 Due to both increasing levels in the neonate of bilirubin derived from senescent red blood cells and increasing expression levels of the hepatic enzyme uridine diphosphate glucuronyl transferase (UDPGT), from 1% of adult values at 30 to 40 weeks of gestation to adult levels during the first few weeks of postnatal life,Citation19 the level of conjugated bilirubin in the canaliculus and bile is elevated. It is transported via the biliary tract to the small intestine and either excreted, or deconjugated and reabsorbed into blood as part of the bilirubin enterohepatic circulationCitation20,21 all of which may promote maturation of the secretory function of cholangiocytes. The different ratio of the primary bile acid, cholic acid, and chenodeoxycholic acid, as well as the chemical position conjugated demonstrate unique changes of the bile acid synthetic pathway between fetal and adult livers.Citation22-24 Another aspect to consider is the fact that the bile acid pool size goes through a significant expansion at the end of gestation.Citation25 In infants, it becomes comparable to that of adults at the age of 7 weeks after correcting for body surface area,Citation26 and can be explained by the increased activity of enzymes involved in bile acid synthesis among hepatocytes just before birth.Citation27 During the first week of life, the primary bile acids circulating in the serum reach a significantly higher concentration compared to healthy children and adults, which may accelerate the maturation of channels and receptors in cholangiocytes.Citation28 With regard to the neonatal gallbladder, its complete development is necessary for efficient bile flow into the duodenum. Additionally, the sodium bile acid co-transport activity of the terminal ileum also attains full function postnatally, normally by the time of weaning.Citation29
Generating cholangiocytes from human iPSCs derived hepatoblasts
Given that hepatic specification initiates from hepatoblasts which originate both hepatocytes and cholangiocytes, the derivation of hepatoblasts from pluripotent stem cells has been sought as a main objective by researchers.Citation30 According to the formation of hepatic tissue in vivo, several of these well know stepwise protocols (developed in mouse and human ESCs) employ the formation of embryonic bodies (EBs) and short exposure to TGF-beta superfamily members activin A and fibroblast growth factor 2 (FGF2) to generate definitive endoderm (DE) cells, followed by hepatoblast specification which requires both 1% dimethyl sulfoxide (DMSO) and hepatocyte growth factor (HGF).Citation31,32 After being proven that such differentiation is also possible in mouse iPSCs,Citation33 Hay, et al.Citation34 devised a non-EB method to generate hepatic-like cells from human ESCs that only requires DMSO for hepatoblast formation,Citation34 and proved the important role of Wnt3 during the DE commitment stage; the utility of this method has been proven and applied in several human iPSCs studies.Citation35,36,37 Song, et al.,Citation38 who were the first to report the hepatic differentiation of human iPSCs, reported hepatoblast induction, as a result of the combined stimuli of HGF and keratinocyte growth factor. Si-Tayeb, et al.Citation39 developed an iPSCs hepatic differentiation protocol by using bone morphogenic protein 4 (BMP4) and FGF2. Two different teams which recently established their own differentiation procedures to derive cholangiocyte-like cells used this protocol as hepatoblast induction.Citation40,41,42
Dianat, N. et al. settled for the first time robust conditions to drive differentiation of human pluripotent stem cells (ESCs, iPSCs) derived hepatoblasts into functional cholangiocyte-like cells using feeder-free and defined culture settings, including the use of growth hormone, epidermal growth factor (EGF), IL-6, and sodium taurocholate. The cholangiocytic lineage obtained was found to express cell-specific markers including CK-7 and osteopontin, the transcription factors SOX9 and hepatocyte HNF6 and functional proteins including cystic fibrosis transmembrane conductance regulator (CFTR), secretin receptor (SR), and nuclear receptors; primary cilia and response to hormonal stimulation were observed as well. In a three-dimensional (3D) matrix, those cells developed epithelial/apicobasal polarity and formed functional cysts and biliary ducts.Citation43 Afterwards, two systematic studies were published applying different approaches to generate specific cholangiocyte-like cells: Ogawa, et al. described a protocol that achieved efficient differentiation of cholangiocytes from iPSCs by means of a 3D co-culture of hepatoblasts with OP9 stromal cells and the combination of different growth factors including HGF, EGF and TGF-β,44 while Sampaziotis, et al. emphasized on the pre-cholangiocyte stage, which can be called cholangiocyte progenitor specification, using FGF10, Activin A, and retinoic acid before the 3D condition.Citation45 Both studies accomplished creating functional cells that acquire epithelial functions such as rhodamine efflux and CFTR-mediated fluid secretion, and that yield cystic and/or ductal structures that express mature biliary markers, including apical sodium-dependent bile acid transporter, SR, cilia and CFTR. Ogawa et al. demonstrated the importance of NOTCH signaling in cholangiocytes differentiation, whereas Sampaziotis et al pointed out the functional characteristics of cholangiocytes, which comprises bile acids transfer, alkaline phosphatase activity, gamma-glutamyl-transpeptidase activity and physiological responses to secretin, somatostatin, and vascular endothelial growth factor. Another novel stepwise cholangiocyte differentiation strategy was designed by De Assuncao, et al., who fully characterized the cells in vitro and in vivo, by exposing them to high doses of Jagged1 and TGF-β. In vivo, the cells engrafted within mouse liver following retrograde intrabiliary infusion.Citation46 The most recent study which applies a similar differentiation protocol was carried by Dianat, N. et al., who proved that laminin could promote gene expression levels of the cholangiocyte markers, AQP1, SOX9, CFTR, G protein-coupled bile acid receptor 1 (GPBAR1), JAG1, SCTR, and gama-glutamyl transferase (GGT1).Citation47
Current and potential strategies for maturation of human iPSCs derived cholangiocyte-like cells
The evaluation and control of the transcriptional network and markers for maturation
Defining and controling a unique transcriptome that allows hepatoblasts to enter the biliary lineage and maturate into cholangiocytes instead of hepatocytes, should be the first strategy for optimizing this type of iPSC differentiation process. Such an approach has been already considerably taken advantage of in phenotype characterization. The cholangitic transcriptional network has been found to be closely linked in part to factors that are crucial to define further growth of the endoderm into either the pancreatic or hepatic lineage, since the suppression of the marker genes of the definitive endoderm Sox17 and FoxA2 (needed to specify foregut endoderm and subsequent derivation), results in cholangiocyte hyperplasia associated to an excessive expression of IL-6.Citation48,49 During the hepatoblast stage, T-box transcription factor (Tbx)-3 not only takes part in hepatoblast migration into the septum transversum along with other factors such as prospero-related homeobox (Prox)-1, but is fundamental too in increasing the expression of HNF 6 and HNF1β and decreasing the level of HNF4α. This is an essential process, as HNF4α together with alpha-fetoprotein (AFP) are key markers of hepatocyte fate commitment; consequently, their expression should be repressed in order to facilitate biliary differentiation.Citation50,51 When progenitor cells reach the cholangiocyte stage, an important mediator is HNF-6 whose expression, and that of another related factor, onecut (OC)-2, is much higher in cholangiocytes than in hepatoblasts or hepatocytes, and is related to the modulation of TGF-β gradients.Citation49 Furthermore, the relevance of the liver enriched factors HNF6 and HNF1β concerns not only their embryological role, but their function in normal biliary physiology as well, as both influence bile acid metabolism besides participating in other hepatic pathways. Interestingly, Prox-1 is repressed by the factor liver receptor homolog (LRH)-1, which also contributes to cholangiocytes physiologically.Citation52 Another factor with a significant role is SOX9 which can be observed first supporting normal TGF-β activity in the cells that will constitute the ductal plate that, as mentioned previously, maintains cholangiocyte differentiation. Seemingly, it is downstream of HNF6 and upstream of CAAT/enhancer-binding protein (C/EBP)-α, a hepatocyte enriched transcription factor known previously as HNF2. The latter must be inhibited by SOX9 in order to allow normal biliary-favoring signaling of HNF6 and HNF-1β, even though low C/EBPα expression is also associated with low HNF-6 levels which have been found to induce a hybrid phenotype.Citation53 Cytokeratin 19 is expressed at low levels in hepatoblasts and at increasing levels in differentiating cholangiocytes, nonetheless, it is not a good indicator of cell fate commitment. On the contrary, cytokeratin 7 becomes detectable when cholangiocytes are already committed to a cholangiocyte lineage.Citation54 Much is yet to uncover regarding other pathways for which there is some evidence of biliary participation. The need is clear for better models that will enable the study and precise characterization of the factors that mediate the embryogenesis of the biliary system (Fig. 1).
Functional maturation stimulus from the bile acids
Physiologically, cholangiocytes carry out the alkalinization of the bile produced by hepatocytes, and contribute to the modification of this fluid with other secretory and absorptive functions, all of which accounts an approximate 30% of total bile production.Citation55 The processing of bile acids is certainly essential to digestion, nonetheless, it also represents an important signaling system which can guide proliferation and growth in cholangiocytes. This has been shown in the case of taurocholic, taurolithocholic, and glycodeoxycholic acid, while ursodeoxycholic acid has the opposite effect of inhibiting proliferation.Citation56 Interestingly, some of these bile acids, namely tauroursodeoxycholic acid and ursodeoxycholic acid, additionally indirectly stimulate cholangiocytic secretion of fluid and electrolytes through changes in cytosolic calcium, which are caused by ATP released into bile by induced hepatocytes.Citation57 Furthermore, taurocholate when infused in rats was found to induce structural changes of the bile ducts, including an increase in canalicular branching and redundancy of canalicular microvilli.Citation58
Since patients with primary biliary cholangitis, a disease that targets the bile ducts, display histologically hyperplasia of cholangiocytes at early stages, it can be inferred that bile acids exert favoring activity on the biliary epithelium.Citation59 This has been replicated with the so-called bile duct ligation models which have demonstrated an increase in the number of cholangiocytes due to ductular proliferation.Citation60 Experiments on hepatocyte transplantation have even shown that when transplanted in the spleen and by ligating the bile duct, hepatocytes develop bile canaliculi resembling Hering's canals onto which they cluster.Citation61 In a contradictory manner, bile stasis is an important factor leading to cytoxicity, oxidative stress, and apoptosis, as is seen for example in cirrhotic livers, nonetheless, in cholangiocytes it appears to have a carcinogenic effect by promoting proliferation and inflammation.Citation62 In addition, bile acids bring about the benefits mentioned above concerning secretion, which in the case of taurocholic and taurolithocholic acid include an increase in gene expression of the secretin receptor, cAMP levels, and Cl−/HCO3− exchanger activity.Citation63
Figure 1. During generation of iPSCs derived cholangiocytes, the key transcription factors that guide the specification of definitive endoderm cells (DE) and hepatoblasts stages are pointed out in the figure, which should be well evaluated. Those factors that favor commitment of hepatoblasts to the hepatocytic lineage should be down regulated during the cholangiocyte differentiation. Several markers are showed in the figure that can be used to recognize mature cholangiocytes, and thus evaluate the differentiation process; these also include cell ultrastructural components and transporters that are essential for normal cholangitic function.
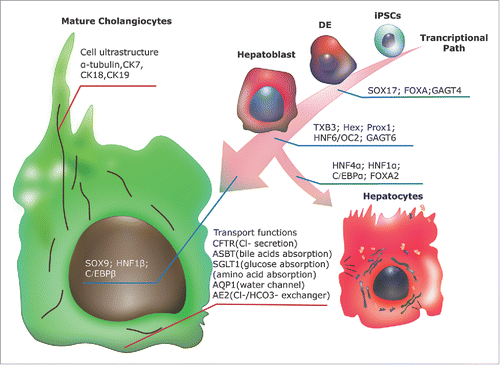
As to the molecular factors activated through bile acid signaling, it appears that the key initial triggers are their binding to the nuclear receptor farnesoid X-receptor (Fxr) and/or the plasma membrane G protein-coupled bile acid receptor TGR5.Citation56 In hepatocytes, for instance, Fxr activation by bile salts causes the short heterodimer protein (Shp) to block retinoid-X nuclear receptor/retinoic acid receptor (RXR/RAR) from binding to the promoter of the sodium taurocholate-cotransporting polypeptide (NTCP), a bile acid transporter protein expressed in the basolateral membrane of hepatocytes.Citation55 In cholangiocytes, the knockout of Tgr5 prevented their proliferation in mice bile duct ligation models, and on the other hand, bile salt stimulation of this receptor was shown to result in an increase in cAMP, biliary bicarbonate secretion, and secretin receptors in the human gallbladder epithelium.Citation64 Regarding Fxr activation in cholangiocytes, it is linked to cytoprotective and anti-cholestatic properties, which is attributed indirectly to the inhibition of CYP27 expression, a rate determining enzyme of bile acid synthesis.Citation65
Maturation in extracellular matrix
A key component that is also relevant and should be taken into account when culturing and differentiating pluripotent cell lines, is the extracellular matrix. The use of specific basal lamina components such as collagen or fibronectin, and not only Matrigel whose conformation isn't completely known and derives from mouse Engelbreth–Holm–Swarm tumors, has proved to favor cell-specific morphologic changes and gene expression as well.Citation66 In regard to this, the team of Tanimizu et al. demonstrated that by culturing a liver progenitor cell line with laminin- 111 (α1β1γ1), one of the major components of Matrigel, cyst formation was promoted and apicobasal polarity that is typical for cholangiocytes could be observed.Citation67 Laminin is a heterotrimer glycoprotein composed of α, β, and γ chains, of which five, four and three variants exist respectively, and a total of 18 isoforms can be found in mammals; laminin receptors include syndecans and dystroglycans, but the main adhesion receptor of human embryonic stem cells are integrins which are made of α and β chains.Citation68 In a further study, it was shown that β1 integrins are the main ones that activate the signaling system from laminins that leads to cyst formation in hepatic progenitor cells, being α6β1 the most characterized. Moreover, α−1 containing laminin is an important but dispensable extracellular matrix component needed to promote differentiation into cholangiocytes, while α−5 containing laminin is not only necessary for cyst formation, but for bile duct formation as well since knockout livers of this laminin presented a decrease in mature ductal structures and a reduced size of the luminal space of the remaining ducts.Citation68 This can be expected, considering that α−1 containing laminins are relevant to the regenerating liver, as was shown with the transient expression of laminin 111 in the liver lobules of mice after two-thirds partial hepatectomy, and α−5 containing laminins (specifically laminins 511 and 521) are expressed in the basal lamina of mature epithelial tissues including bile ducts, central veins, and hepatic arteries.Citation69 Taking into account that the extracellular matrix is equally relevant to growth factors and the culture media for the differentiation of iPSCs into cholangiocytes, Tanimizu et al.Citation68 set out to prove the utility of various laminin isoforms. Due that the mass production of intact, full-length laminins is technically difficult and requires weeks for purification, shorter recombinant E8 fragments were used as reported by Mizayaki et al. which can be purified in days, have a higher yield, and retain full α6β1 integrin-binding capacity; in addition, E8 laminin fragments promote a greater adhesion of human iPSCs than substrates like Matrigel and intact laminin 511.Citation66 Thus, it was proved that culture with laminins 411 E8 and 511 E8 supported the cholangiocyte differentiation from hepatoblast-like cells, and on the contrary, laminin 111 E8 and also 121 E8, 211 E8, and 221 E8 inhibit this transition. Further studies will be needed to characterize the pathways activated by these laminins and to optimize culture conditions.
Future applications of human iPSCs cholangiocytes in basic and clinical research
Modeling of cholangiocyte disease
Cholangiopathies are a set of disorders of the epithelial cells that line the bile ducts, commonly known as cholangiocytes, apparently caused by genetic and environmental factors and that can progress to end-stage liver disease, or even suffer malignant transformation.Citation3 Their significance resides in the fact that they are the leading cause in up to a third of all liver transplants done on adults, with primary biliary cholangitis and primary sclerosing cholangitis being the principal leading cholangiopathies. In children, biliary atresia represents the main reason for liver transplant.Citation70 The progressive developments of generating mature cholangiocyte from human iPSCs are opening new possibilities in the field of cholangiopathies, encouraging the conception of experiments that more closely resemble pathology under human conditions and holds great promise for new and precise human models that will enable a better understanding of disease and pathophysiology, as virtually any type of anomaly can be recreated, either artificially by gene manipulation or by obtaining and reprogramming patient cells. The two recent studies of genarating iPSCs derived cholangiocytes also explored this area. Ogawa et al. developed cholangiocytes from patients with cystic fibrosis (CF) carrying the characteristic F508del mutation of this disorder, which interestingly had delayed cyst formation even after the addition of the folding defect corrector molecule VX-809 (now FDA approved as Lumacaftor). Nonetheless, treated cysts that were potentiated with VX-770 (also FDA approved, Ivacaftor) showed significant swelling compared to untreated cysts after induction with forskolin/IBMX.Citation44 In a similar manner, the team of Sampaziotis et al. decreased CFTR function with improvement and increased organoid size after the addition of corrector molecules on CF patient derived cholangiocytes.Citation45 Such an effect is achieved clinically in the polycystic liver disease (PLD) cholangiopathy, characterized by cystic lesions due to benign cholangiocyte hyperproliferation, but with octreotide, a synthetic somatostatin analog.Citation3 Of worth noting, both disease models have shown valid applications of this iPSCs not only in the proving of pathophysiological interactions, but in biliary-specific pharmacological screening as well. In the close future, functional grafts all from iPSCs are also expected, potentially serving as treatment for diseases with no other cure available.
In present literature, no other cholangiopathy has been modeled with iPSCs technology, though sufficient examples exist that could serve as a basis. The advances that have been made with CF and PLD prove that innovative experiments can be designed, as compared to earlier models, which relied only on animals with induced deficiency or mutation of characteristic genes, Cftr or ΔF508 in CF, and sec 63, prkcsh, pkd1, or pkhd1 in PLD.Citation71-73 Modeling with iPSCs permits the direct introduction of disease-specific genes in the target cells, with consequent observation and follow up until pathology settles. This also will allow the testing of new drugs before introducing them on animal models, which may be easier to achieve given that pathophysiology can be inferred with more detail. In biliary atresia, perinatal obstructive fibrosis of the bile ducts due to unexplained phenomena occurs, though attributed to both the environment and genetics. Currently, models for this disease have found that sensitization to viral particles, including rotavirus, reovirus or cytomegalovirus can lead to biliary obstruction. Nonetheless, certain susceptibility yet to decipher triggers an exaggerated immune response that induces fibrosis, perhaps related to autoimmunity although patients don't benefit from corticosteroid use.Citation74,75 Thus, it will be interesting to confirm such an etiological hypothesis with iPSCs cholangiocytes derived from biliary atresia cases, and genetic alteration may define potential therapeutic targets. Primary biliary cholangitis and primary sclerosing cholangitis are similar cholangiopathies in the sense that both are characterized by chronic immune-mediated inflammation and destruction of the bile ducts, although their differences are evident including a female preponderance and distinctive antimitochondrial antibodies in the former, and male preponderance and probable HLA antigen-B locus related susceptibility in the latter.Citation3,76 As with the other cholangiopathies discussed, their etiology is still not clearly elucidated, in part because of the complex interaction between environment and genes. Current animal models have recurred mainly to genetic alterations, xenobiotic immunization, infectious agents, and chemical induction, though neither one can fully show all disease-specific characteristics.Citation76,77 The use of iPSCs technology will allow the possibility of directly studying pathological mechanisms with patient-specific cholangiocytes, and perhaps solve what determines each clinical condition. The idea of personalized medicine is also an attractive one, as for example, not all patients with primary sclerosing cholangitis respond to ursodeoxycholic acid therapy, and long-term consequences of the use of this drug in patients with primary biliary cholangitis are unknown.Citation3 In the case of cholangiocarcinoma, no specific etiology can be attributed to the disease, although chronic inflammation with concomitant malignant transformation is an important factor considering the fact that primary sclerosing cholangitis and polycystic liver disease are the main risk factors involved.Citation3,78 It appears that cholangiocarcinoma is the result of genetic alterations, induced by any of the risks factors, as was proven in a hamster model where infection with the helminth parasite Clonorchis sinensis in hamsters caused upregulation of oncogenes and downregulation of tumor suppressor genes.Citation79 Accordingly, it is tantalizing to recreate an inflammatory microenvironment in iPS cholangiocyte cultures that favors dysplasia. Multiple ways to achieve this setting are to be found, cholestasis already being a common tested variable in bile duct obstruction models, including direct exposure to inflammatory cytokines or IL-33, a biliary mitogen.Citation80,81
iPSCs cholangiocytes in cell therapy, scaffolding, and bioprinting
As the generation of functional differentiated cholangiocytes is becoming an easier goal to reach, it is an attractive idea to use them as part of cell therapy, as has been intensively researched with hepatocytes. Although non-parenchymal cells represent only a small fraction of the total liver mass (6.5%), they are an essential component with functions that benefit and enhance overall hepatocyte metabolism, thus possibly favoring their survival which in regard to cell therapy could represent reduced hepatocyte amounts needed to achieve a therapeutic effect.Citation82,83 In the case of cholangiocytes, a conceivable advantage is the complementing of bile canaliculi with concomitant elimination of hepatocyte end products of metabolism.Citation84 The extent to which cholangiocyte transplant has been explored is short, reflecting the broader focus on hepatocytes. After finding that intrasplenic transplantation of autologous adult hepatocytes in Lewis rat recipients formed hepatocyte cords but not bile canaliculi when pretreated with a mixture of irradiation, intravenous anti-asialo GM1 antiserum, and syngeneic bone marrow cell reconstitution, Olszewski et al.Citation84 added to their protocol the ligation of the common bile duct, which resulted in the development of these biliary structures. Interestingly, it was found that transplanted hepatocytes and not autologous cholangiocytes responded to an unknown signal in the bile stasis model, creating canaliculi with CK9 and CK19 and gamma-glutamyl transpeptidase positive cells. Thus, it appeared that hepatocyte intrasplenic transplantation was sufficient for laying out the basis of the biliary architecture as long as there is ligation of the common bile duct, probably due to a fraction of hepatocytes with cholangiocyte progenitor cell properties, while cholangiocyte transplantation did not lead to either hepatocyte or canaliculi development, probably indicating a more mature and differentiated phenotype.Citation84 Considering the seeding of scaffolds for bioengineered organs using both iPSCs derived cholangiocytes and hepatocytes, the question arises if cholangiocytes would react to the bile canaliculi formed by the latter and establish a functional biliary system that would promote even more hepatocyte growth.
In regard to liver engineering, the team of Baptista et al. set out a method for making liver 3D scaffolds by decellularization and attempted to recellularize them.Citation85 Although they used human fetal hepatocytes and human umbilical vein endothelial cells for the recellularization of the hepatic parenchyma, they prove that reepithelization of the bile ducts occurs as immunostaining for CK-19 was positive in biliary tubular structures, and surrounding them were cells that either stained for CK18, a marker of hepatocytes, or both CK-19 and CK-18, indicating also immature cells with hepatoblast properties.Citation85 Their results are the only ones in current literature that point out to the possibility of biliary reseeding, and in this case show the importance of the extracellular matrix provided by the scaffold in the engraftment of specific cells which react to the cues given by the various matrix molecules. Coinciding in a way with the results of Olszewski,Citation84 it is clear that hepatocytes remain an essential factor in cell therapy and bioengineering, due to their plasticity when either in their adult or fetal forms. Thus, for future experiments that involve iPSCs cholangiocytes, it might be useful to include both mature hepatocytes and hepatoblasts, in an effort to set up a basis of biliary architecture that will promote cholangiocyte engraftment and growth.
An alternative to decellularization methods in order to obtain hepatic scaffolds is to recur to bioprinting, an emerging area of research that promises the creation of 3D tissue structures that resemble those in the normal organ of origin. Despite the technical difficulties that this method implies, including cell damage due to biomechanical forces, the maintaining of the cell culture in the printed structure, and preserving normal cell metabolism,Citation86 bioprinting might perhaps become a faster and more accurate procedure to generate disease models than organic scaffolds. Whole organ manufacture is one possibility, nonetheless, small scale samples (associated with the concept of “liver on a chip”) are more accessible and may mimic the tissue's normal physiology.Citation87 Few publications are at present focused specifically on liver tissue bioprinting, and as is seen with existing reports on liver scaffolds, the biliary system is for now left out. An early indication on the incorporation of cholangiocytes is mentioned briefly in a recent article that reports the use of digital light processing-based 3D printing to recreate liver lobules which supported iPSCs hepatic progenitor cells, endothelial cells and adipose-derived stem cells; although biliary epithelium wasn't characterized in this model, the authors suggest the hepatoblasts printed on the lobules may have been favored to differentiate into this tissue as well, which would indicate maturation and functionality of the 3D structures.Citation88 Certainly, the addition of iPSCs derived cholangiocytes in bioprinting may help maintaining the threatened hepatocyte mass on the manufactured scaffolds, though their interaction with printed biliary ducts and the rest of the cells will be an interesting aspect to demonstrate.
As emphasized by Mubbach et al, there are three important goals to accomplish, if a true liver is to be produced by bioengineering methods: the reseeding of the parenchyma, vascular endothelization, and biliary endothelization.Citation89 Unfortunately, too few attention has been given to the latter goal, perhaps owed to the feat of obtaining a sufficient amount of cholangiocytes with a stable phenotype. Until recently, the creation of iPSCs derived cholangiocytes through high efficiency protocols should represent a means to curve this problem. Nonetheless, once this is settled, the problem of cell mass production will soon be needed to solve. The advances accomplished in these fields of research will be in much need not only for cholangiopathies, but for many other liver diseases with no other hope than liver transplant.
CONCLUSION
As we have mentioned throughout, cholangiocyte-specific research is scarce at present, though it's clear that a greater interest is now being directed toward their generation and application in regenerative medicine and toward the creation of better and improved models of disease. While hepatocytes may be the fundamental piece when aiming for any of these objectives, one cannot leave behind the rest of the cells that also conform the liver mass, and whose function is clearly essential in many house-keeping tasks. Cholangiocytes may be the second most important cell in this organ, with bile ducts forming part of the portal triads that demarcate the hepatocyte-rich lobules. Bile is a toxic substance with corrosive properties when not processed adequately, as is evident in patients with cholangiopathies, thus, the inclusion of biliary epithelial cells should be pursued if a true liver graft is to be bioengineered; this also points out to the importance of giving continuity to the models already made for these pathologies, so as to find new therapeutic targets that can be altered either pharmacologically or with an innovative regenerative approach. The review we have presented should inspire the developing of better cholangiocyte differentiation and culture methods, which will be of much need in this continually expanding stem cell study field.
DISCLOSURE OF POTENTIAL CONFLICTS OF INTEREST
No potential conflicts of interest were disclosed.
FUNDING
This work was supported by the Natural Science Foundation of China grant # 81470874, 81470874 for the stipend of Y.W.
REFERENCES
- Collin de l'Hortet A, Takeishi K, Guzman-Lepe J, Handa K, Matsubara K, Fukumitsu K, Dorko K, Presnell SC, Yagi H, Soto-Gutierrez A. Liver-Regenerative Transplantation: Regrow and Reset. Am J Transplant 2016; 16:1688-1696; PMID:26699680; http://dx.doi.org/10.1111/ajt.13678
- Gordillo M, Evans T, Gouon-Evans V. Orchestrating liver development. Development 2015; 142:2094-2108; PMID:26081571; http://dx.doi.org/10.1242/dev.114215
- Lazaridis KN, LaRusso NF. The Cholangiopathies. Mayo Clin Proc 2015; 90:791-800; PMID:25957621; http://dx.doi.org/10.1016/j.mayocp.2015.03.017
- Aoi T. 10th anniversary of iPS cells: the challenges that lie ahead. J Biochem 2016; 160:121-129; PMID:27387749; http://dx.doi.org/10.1093/jb/mvw044
- Roskams T, Desmet V. Embryology of extra- and intrahepatic bile ducts, the ductal plate. Anat Rec (Hoboken) 2008; 291:628-635; PMID:18484608; http://dx.doi.org/10.1002/ar.20710
- Antoniou A, Raynaud P, Cordi S, Zong Y, Tronche F, Stanger BZ, Jacquemin P, Pierreux CE, Clotman F, Lemaigre FP. Intrahepatic bile ducts develop according to a new mode of tubulogenesis regulated by the transcription factor SOX9. Gastroenterology 2009; 136:2325-2333; PMID:19403103; http://dx.doi.org/10.1053/j.gastro.2009.02.051
- Raynaud P, Carpentier R, Antoniou A, Lemaigre FP. Biliary differentiation and bile duct morphogenesis in development and disease. Int J Biochem Cell Biol 2011; 43:245-256; PMID:19735739; http://dx.doi.org/10.1016/j.biocel.2009.07.020
- Carpentier R, Suñer RE, van Hul N, Kopp JL, Beaudry JB, Cordi S, Antoniou A, Raynaud P, Lepreux S, Jacquemin P, et al. Embryonic ductal plate cells give rise to cholangiocytes, periportal hepatocytes, and adult liver progenitor cells. Gastroenterology 2011; 141:1432-1438, 1438 e1431–1434; PMID:21708104; http://dx.doi.org/10.1053/j.gastro.2011.06.049
- Li Z, White P, Tuteja G, Rubins N, Sackett S, Kaestner KH. Foxa1 and Foxa2 regulate bile duct development in mice. J Clin Invest 2009; 119:1537-1545; PMID:19436110; http://dx.doi.org/10.1172/JCI38201
- Geisler F, Strazzabosco M. Emerging roles of Notch signaling in liver disease. Hepatology 2015; 61:382-392; PMID:24930574; http://dx.doi.org/10.1002/hep.27268
- McCright B, Lozier J, Gridley T. A mouse model of Alagille syndrome: Notch2 as a genetic modifier of Jag1 haploinsufficiency. Development 2002; 129:1075-1082; PMID:11861489
- Kodama Y, Hijikata M, Kageyama R, Shimotohno K, Chiba T. The role of notch signaling in the development of intrahepatic bile ducts. Gastroenterology 2004; 127:1775-1786; PMID:15578515
- Geisler F, Nagl F, Mazur PK, Lee M, Zimber-Strobl U, Strobl LJ, Radtke F, Schmid RM, Siveke JT. Liver-specific inactivation of Notch2, but not Notch1, compromises intrahepatic bile duct development in mice. Hepatology 2008; 48:607-616; PMID:18666240; http://dx.doi.org/10.1002/hep.22381
- Sparks EE, Huppert KA, Brown MA, Washington MK, Huppert SS. Notch signaling regulates formation of the three-dimensional architecture of intrahepatic bile ducts in mice. Hepatology 2010; 51:1391-1400; http://dx.doi.org/10.1002/hep.23431
- Clotman F, Lannoy VJ, Reber M, Cereghini S, Cassiman D, Jacquemin P, Roskams T, Rousseau GG, Lemaigre FP. The onecut transcription factor HNF6 is required for normal development of the biliary tract. Development 2002; 129:1819-1828; PMID:11934848
- Tan X, Yuan Y, Zeng G, Apte U, Thompson MD, Cieply B, Stolz DB, Michalopoulos GK, Kaestner KH, Monga SP. Beta-catenin deletion in hepatoblasts disrupts hepatic morphogenesis and survival during mouse development. Hepatology 2008; 47:1667-1679; PMID:18393386; http://dx.doi.org/10.1002/hep.22225
- Hirose Y, Itoh T, Miyajima A. Hedgehog signal activation coordinates proliferation and differentiation of fetal liver progenitor cells. Exp Cell Res 2009; 315:2648-2657; PMID:19559697; http://dx.doi.org/10.1016/j.yexcr.2009.06.018
- Grijalva J, Vakili K. Neonatal liver physiology. Semin Pediatr Surg 2013; 22:185-189; PMID:24331092; http://dx.doi.org/10.1053/j.sempedsurg.2013.10.006
- Watchko JF, Lin Z. Exploring the genetic architecture of neonatal hyperbilirubinemia. Semin Fetal Neonatal Med 2010; 15:169-175; PMID:20022574; http://dx.doi.org/10.1016/j.siny.2009.11.003
- Dennery PA, Seidman DS, Stevenson DK. Neonatal hyperbilirubinemia. N Engl J Med 2001; 344:581-590; PMID:11207355; http://dx.doi.org/10.1056/NEJM200102223440807
- Watchko JF, Maisels MJ. Jaundice in low birthweight infants: pathobiology and outcome. Arch Dis Child Fetal Neonatal Ed 2003; 88:F455-458; PMID:14602689
- Lester R, St Pyrek J, Little JM, Adcock EW. Diversity of bile acids in the fetus and newborn infant. J Pediatr Gastroenterol Nutr 1983; 2:355-364; PMID:6348233
- Setchell KD, Dumaswala R, Colombo C, Ronchi M. Hepatic bile acid metabolism during early development revealed from the analysis of human fetal gallbladder bile. J Biol Chem 1988; 263:16637-16644; PMID:3182806
- Gustafsson J. Bile acid biosynthesis during development: hydroxylation of C27-sterols in human fetal liver. J Lipid Res 1986; 27:801-806.
- Little JM, Richey JE, Van Thiel DH, Lester R. Taurocholate pool size and distribution in the fetal rat. J Clin Invest 1979; 63:1042-1049; PMID:3772248; http://dx.doi.org/10.1172/JCI109373
- Heubi JE, Balistreri WF, Suchy FJ. Bile salt metabolism in the first year of life. J Lab Clin Med 1982; 100:127-136; PMID:7201000
- Henning SJ. Postnatal development: coordination of feeding, digestion, and metabolism. Am J Physiol 1981; 241:G199-214; PMID:7025659
- Suchy FJ, Balistreri WF, Heubi JE, Searcy JE, Levin RS. Physiologic cholestasis: elevation of the primary serum bile acid concentrations in normal infants. Gastroenterology 1981; 80:1037-1041; PMID:7202962
- Barnard JA, Ghishan FK, Wilson FA. Ontogenesis of taurocholate transport by rat ileal brush border membrane vesicles. J Clin Invest 1985; 75:869-873; PMID:2579978; http://dx.doi.org/10.1172/JCI111785
- Roy-Chowdhury N, Wang X, Guha C, Roy-Chowdhury J. Hepatocyte-like cells derived from induced pluripotent stem cells. Hepatol Int 2016; 1-16; http://dx.doi.org/10.1007/s12072-016-9757-y
- Soto-Gutierrez A, Kobayashi N, Rivas-Carrillo JD, Navarro-Alvarez N, Zhao D, Okitsu T, Noguchi H, Basma H, Tabata Y, Chen Y, et al. Reversal of mouse hepatic failure using an implanted liver-assist device containing ES cell-derived hepatocytes. Nat Biotechnol 2006; 24:1412-1419; PMID:17086173; http://dx.doi.org/10.1038/nbt1257
- IBasma H, Soto-Gutiérrez A, Yannam GR, Liu L, Ito R, Yamamoto T, Ellis E, Carson SD, Sato S, Chen Y, et al. Differentiation and transplantation of human embryonic stem cell-derived hepatocytes. Gastroenterology 2009; 136:990-999; PMID:19026649; http://dx.doi.org/10.1053/j.gastro.2008.10.047
- Gai H, Nguyen DM, Moon YJ, Aguila JR, Fink LM, Ward DC, Ma Y. Generation of murine hepatic lineage cells from induced pluripotent stem cells. Differentiation 2010; 79:171-181; PMID:20106584; http://dx.doi.org/10.1016/j.diff.2010.01.002
- Hay DC, Zhao D, Ross A, Mandalam R, Lebkowski J, Cui W. Direct differentiation of human embryonic stem cells to hepatocyte-like cells exhibiting functional activities. Cloning Stem Cells 2007; 9:51-62; PMID:17386014; http://dx.doi.org/10.1089/clo.2006.0045
- Chen YF, Tseng CY, Wang HW, Kuo HC, Yang VW, Lee OK. Rapid generation of mature hepatocyte-like cells from human induced pluripotent stem cells by an efficient three-step protocol. Hepatology 2012; 55:1193-1203; PMID:22095466; http://dx.doi.org/10.1002/hep.24790
- Sullivan GJ, Hay DC, Park IH, Fletcher J, Hannoun Z, Payne CM, Dalgetty D, Black JR, Ross JA, Samuel K, et al. Generation of functional human hepatic endoderm from human induced pluripotent stem cells. Hepatology 2010; 51:329-335; PMID: 19877180; http://dx.doi.org/10.1002/hep.23335
- Hay DC, Fletcher J, Payne C, Terrace JD, Gallagher RC, Snoeys J, Black JR, Wojtacha D, Samuel K, Hannoun Z, et al. Highly efficient differentiation of hESCs to functional hepatic endoderm requires ActivinA and Wnt3a signaling. Proc Natl Acad Sci U S A 2008; 105:12301-12306; PMID:18719101; http://dx.doi.org/10.1073/pnas.0806522105
- Song Z, Cai J, Liu Y, Zhao D, Yong J, Duo S, Song X, Guo Y, Zhao Y, Qin H, et al. Efficient generation of hepatocyte-like cells from human induced pluripotent stem cells. Cell Res 2009; 19:1233-1242; PMID:19736565; http://dx.doi.org/10.1038/cr.2009.107
- Si-Tayeb K, Noto FK, Nagaoka M, Li J, Battle MA, Duris C, North PE, Dalton S, Duncan SA. Highly efficient generation of human hepatocyte-like cells from induced pluripotent stem cells. Hepatology 2010; 51:297-305; PMID:19998274; http://dx.doi.org/10.1002/hep.23354
- Ogawa S, Surapisitchat J, Virtanen C, Ogawa M, Niapour M, Sugamori KS, Wang S, Tamblyn L, Guillemette C, Hoffmann E, et al. Three-dimensional culture and cAMP signaling promote the maturation of human pluripotent stem cell-derived hepatocytes. Development 2013; 140:3285-3296; PMID:23861064; http://dx.doi.org/10.1242/dev.090266
- Hannan NR, Fordham RP, Syed YA, Moignard V, Berry A, Bautista R, Hanley NA, Jensen KB, Vallier L. Generation of multipotent foregut stem cells from human pluripotent stem cells. Stem Cell Reports 2013; 1:293-306; PMID:24319665; http://dx.doi.org/10.1016/j.stemcr.2013.09.003
- Touboul T, Hannan NR, Corbineau S, Martinez A, Martinet C, Branchereau S, Mainot S, Strick-Marchand H, Pedersen R, Di Santo J, et al. Generation of functional hepatocytes from human embryonic stem cells under chemically defined conditions that recapitulate liver development. Hepatology 2010; 51:1754-1765; PMID:20301097; http://dx.doi.org/10.1002/hep.23506
- Dianat N, Dubois-Pot-Schneider H, Steichen C, Desterke C, Leclerc P, Raveux A, Combettes L, Weber A, Corlu A, Dubart-Kupperschmitt A. Generation of functional cholangiocyte-like cells from human pluripotent stem cells and HepaRG cells. Hepatology 2014; 60:700-714; PMID:24715669; http://dx.doi.org/10.1002/hep.27165
- Ogawa M, Ogawa S, Bear CE, Ahmadi S, Chin S, Li B, Grompe M, Keller G, Kamath BM, Ghanekar A. Directed differentiation of cholangiocytes from human pluripotent stem cells. Nat Biotechnol 2015; 33:853-861; PMID:26167630; http://dx.doi.org/10.1038/nbt.3294
- Sampaziotis F, Cardoso de Brito M, Madrigal P, Bertero A, Saeb-Parsy K, Soares FA, Schrumpf E, Melum E, Karlsen TH, Bradley JA, et al. Cholangiocytes derived from human induced pluripotent stem cells for disease modeling and drug validation. Nat Biotechnol 2015; 33:845-852; PMID:26167629; http://dx.doi.org/10.1038/nbt.3275
- De Assuncao TM, Sun Y, Jalan-Sakrikar N, Drinane MC, Huang BQ, Li Y, Davila JI, Wang R, O'Hara SP, Lomberk GA, et al. Development and characterization of human-induced pluripotent stem cell-derived cholangiocytes. Lab Invest 2015; 95:684-696; PMID:25867762; http://dx.doi.org/10.1038/labinvest.2015.51
- Takayama K, Mitani S, Nagamoto Y, Sakurai F, Tachibana M, Taniguchi Y, Sekiguchi K, Mizuguchi H. Laminin 411 and 511 promote the cholangiocyte differentiation of human induced pluripotent stem cells. Biochem Biophys Res Commun 2016; 474:91-96; PMID:27103433; http://dx.doi.org/10.1016/j.bbrc.2016.04.075
- Norrman K, Strombeck A, Semb H, Stahlberg A. Distinct gene expression signatures in human embryonic stem cells differentiated towards definitive endoderm at single-cell level. Methods 2013; 59:59-70; PMID:22503774; http://dx.doi.org/10.1016/j.ymeth.2012.03.030
- Lemaigre FP. Mechanisms of liver development: concepts for understanding liver disorders and design of novel therapies. Gastroenterology 2009; 137:62-79; http://dx.doi.org/10.1053/j.gastro.2009.03.035; PMID:19328801
- Ludtke TH, Christoffels VM, Petry M, Kispert A. Tbx3 promotes liver bud expansion during mouse development by suppression of cholangiocyte differentiation. Hepatology 2009; 49:969-978; PMID:19140222; http://dx.doi.org/10.1002/hep.22700
- Gualdi R, Bossard P, Zheng M, Hamada Y, Coleman JR, Zaret KS. Hepatic specification of the gut endoderm in vitro: cell signaling and transcriptional control. Genes Dev 1996; 10:1670-1682; PMID:8682297
- Kamiya A, Kakinuma S, Onodera M, Miyajima A, Nakauchi H. Prospero-related homeobox 1 and liver receptor homolog 1 coordinately regulate long-term proliferation of murine fetal hepatoblasts. Hepatology 2008; 48:252-264; PMID:18571787; http://dx.doi.org/10.1002/hep.22303
- Suzuki A, Iwama A, Miyashita H, Nakauchi H, Taniguchi H. Role for growth factors and extracellular matrix in controlling differentiation of prospectively isolated hepatic stem cells. Development 2003; 130:2513-2524; PMID:12702664
- Van Eyken P, Sciot R, Callea F, Van der Steen K, Moerman P, Desmet VJ. The development of the intrahepatic bile ducts in man: a keratin-immunohistochemical study. Hepatology 1988; 8:1586-1595; PMID:2461337
- Boyer JL. Bile formation and secretion. Compr Physiol 2013; 3:1035-1078; http://dx.doi.org/10.1002/cphy.c120027
- Marin JJ, Macias RI, Briz O, Banales JM, Monte MJ. Bile acids in physiology, pathology and pharmacology. Curr Drug Metab 2015; 17:4-29; PMID:26526836
- Feranchak AP, Fitz JG. Thinking outside the cell: the role of extracellular adenosine triphosphate in bile formation. Gastroenterology 2007; 133:1726-1728; PMID:17983816; http://dx.doi.org/10.1053/j.gastro.2007.09.050
- Layden TJ, Boyer JL. Influence of bile acids on bile canalicular membrane morphology and the lobular gradient in canalicular size. Lab Invest 1978; 39:110-119; PMID:23897680
- Alpini G, Baiocchi L, Glaser S, Ueno Y, Marzioni M, Francis H, Phinizy JL, Angelico M, Lesage G. Ursodeoxycholate and tauroursodeoxycholate inhibit cholangiocyte growth and secretion of BDL rats through activation of PKC alpha. Hepatology 2002; 35:1041-1052; PMID:11981754; http://dx.doi.org/10.1053/jhep.2002.32712
- Tabibian JH, Masyuk AI, Masyuk TV, O'Hara SP, LaRusso NF. Physiology of cholangiocytes. Compr Physiol 2013; 3:541-565; PMID:23720296; http://dx.doi.org/10.1002/cphy.c120019
- Gewartowska M, Olszewski WL. Hepatocyte transplantation-biology and application. Ann Transplant 2007; 12:27-36; PMID:17953140
- Lozano E, Sanchez-Vicente L, Monte MJ, Herraez E, Briz O, Banales JM, Marin JJ, Macias RI. Cocarcinogenic effects of intrahepatic bile acid accumulation in cholangiocarcinoma development. Mol Cancer Res 2014; 12:91-100; http://dx.doi.org/10.1158/1541-7786.MCR-13-0503;PMID: 24255171
- Alpini G, Ueno Y, Glaser SS, Marzioni M, Phinizy JL, Francis H, Lesage G. Bile acid feeding increased proliferative activity and apical bile acid transporter expression in both small and large rat cholangiocytes. Hepatology 2001; 34:868-876; PMID:11679956; http://dx.doi.org/10.1053/jhep.2001.28884
- Keitel V, Haussinger D. TGR5 in the biliary tree. Dig Dis 2011; 29:45-47; PMID:21691103; http://dx.doi.org/10.1159/000324127
- Jung D, York JP, Wang L, Yang C, Zhang A, Francis HL, Webb P, McKeehan WL, Alpini G, Lesage GD, et al. FXR-induced secretion of FGF15/19 inhibits CYP27 expression in cholangiocytes through p38 kinase pathway. Pflugers Arch 2014; 466:1011-1019; PMID:24068255; http://dx.doi.org/10.1007/s00424-013-1364-3
- Miyazaki T, Futaki S, Suemori H, Taniguchi Y, Yamada M, Kawasaki M, Hayashi M, Kumagai H, Nakatsuji N, Sekiguchi K, et al. Laminin E8 fragments support efficient adhesion and expansion of dissociated human pluripotent stem cells. Nat Commun 2012; 3:1236; PMID:23212365; http://dx.doi.org/10.1038/ncomms2231
- Tanimizu N, Miyajima A, Mostov KE. Liver progenitor cells develop cholangiocyte-type epithelial polarity in three-dimensional culture. Mol Biol Cell 2007; 18:1472-1479; PMID:17314404; http://dx.doi.org/10.1091/mbc.E06-09-0848
- Tanimizu N, Kikkawa Y, Mitaka T, Miyajima A. alpha1- and alpha5-containing laminins regulate the development of bile ducts via beta1 integrin signals. J Biol Chem 2012; 287:28586-28597; PMID:22761447; http://dx.doi.org/10.1074/jbc.M112.350488
- Shiojiri N, Sugiyama Y. Immunolocalization of extracellular matrix components and integrins during mouse liver development. Hepatology 2004; 40:346-355; PMID:15368439; http://dx.doi.org/10.1002/hep.20303
- Pisarello MJ, Loarca L, Ivanics T, Morton L, LaRusso N. MicroRNAs in the Cholangiopathies: Pathogenesis, Diagnosis, and Treatment. J Clin Med 2015; 4:1688-1712; PMID:26343736; http://dx.doi.org/10.3390/jcm4091688
- Cohen TS, Prince A. Cystic fibrosis: a mucosal immunodeficiency syndrome. Nat Med 2012; 18:509-519; PMID:22481418; http://dx.doi.org/10.1038/nm.2715
- Tietz Bogert PS, Huang BQ, Gradilone SA, Masyuk TV, Moulder GL, Ekker SC, Larusso NF. The zebrafish as a model to study polycystic liver disease. Zebrafish 2013; 10:211-217; PMID:23668934; http://dx.doi.org/10.1089/zeb.2012.0825
- Munoz-Garrido P, Marin JJ, Perugorria MJ, Urribarri AD, Erice O, Sáez E, Úriz M, Sarvide S, Portu A, Concepcion AR, et al. Ursodeoxycholic acid inhibits hepatic cystogenesis in experimental models of polycystic liver disease. J Hepatol 2015; 63:952-961; PMID:26044126; http://dx.doi.org/10.1016/j.jhep.2015.05.023
- Ningappa M, Min J, Higgs BW, Ashokkumar C, Ranganathan S, Sindhi R. Genome-wide association studies in biliary atresia. Wiley Interdiscip Rev Syst Biol Med 2015; 7:267-273; PMID:25963027; http://dx.doi.org/10.1002/wsbm.1303
- Mezina A, Karpen SJ. Genetic contributors and modifiers of biliary atresia. Dig Dis 2015; 33:408-414; PMID:26045276; http://dx.doi.org/10.1159/000371694
- Pollheimer MJ, Fickert P. Animal models in primary biliary cirrhosis and primary sclerosing cholangitis. Clin Rev Allergy Immunol 2015; 48:207-217; PMID:25172178; http://dx.doi.org/10.1007/s12016-014-8442-y
- Katsumi T, Tomita K, Leung PS, Yang GX, Gershwin ME, Ueno Y. Animal models of primary biliary cirrhosis. Clin Rev Allergy Immunol 2015; 48:142-153; PMID:25771770; http://dx.doi.org/10.1007/s12016-015-8482-y
- Lowe R, Afdhal N, Anderson C. Epidemiology, pathogenesis, and classification of cholangiocarcinoma, <http://www.uptodate.com/contents/epidemiology-pathogenesis-and-classification-of-cholangiocarcinoma 2016
- Uddin MH, Choi MH, Kim WH, Jang JJ, Hong ST. Involvement of PSMD10, CDK4, and tumor suppressors in development of intrahepatic cholangiocarcinoma of syrian golden hamsters induced by clonorchis sinensis and n-Nitrosodimethylamine. PLoS Negl Trop Dis 2015; 9:e0004008; http://dx.doi.org/10.1371/journal.pntd.0004008
- Yamada D, Rizvi S, Razumilava N, Bronk SF, Davila JI, Champion MD, Borad MJ, Bezerra JA, Chen X, Gores GJ. IL-33 facilitates oncogene-induced cholangiocarcinoma in mice by an interleukin-6-sensitive mechanism. Hepatology 2015; 61:1627-1642; http://dx.doi.org/10.1002/hep.27687
- Mu X, Pradere JP, Affò S, Dapito DH, Friedman R, Lefkovitch JH, Schwabe RF. Epithelial transforming growth factor-beta signaling does not contribute to liver fibrosis but protects mice from cholangiocarcinoma. Gastroenterology 2016; 150:720-733; PMID:26627606; http://dx.doi.org/10.1053/j.gastro.2015.11.039
- Gerbal-Chaloin S, Funakoshi N, Caillaud A, Gondeau C, Champon B, Si-Tayeb K. Human induced pluripotent stem cells in hepatology: beyond the proof of concept. Am J Pathol 2014; 184:332-347; PMID:24269594; http://dx.doi.org/10.1016/j.ajpath.2013.09.026
- Navarro-Alvarez N, Soto-Gutierrez A, Chen Y, Caballero-Corbalan J, Hassan W, Kobayashi S, Kondo Y, Iwamuro M, Yamamoto K, Kondo E, et al. Intramuscular transplantation of engineered hepatic tissue constructs corrects acute and chronic liver failure in mice. J Hepatol 2010; 52:211-219; PMID:20022655; http://dx.doi.org/10.1016/j.jhep.2009.11.019
- Olszewski WL, Charysz A, Gewartowska M, Nagui ME. Intrasplenic transplanted adult rat isolated hepatocyte fraction but not cholangiocytes forms bile canaliculi. Transplant Proc 2014; 46:2894-2896; PMID:25380945; http://dx.doi.org/10.1016/j.transproceed.2014.09.067
- Baptista PM, Siddiqui MM, Lozier G, Rodriguez SR, Atala A, Soker S. The use of whole organ decellularization for the generation of a vascularized liver organoid. Hepatology 2011; 53:604-617; PMID:21274881; http://dx.doi.org/10.1002/hep.24067
- Soto-Gutierrez A, Zhang L, Medberry C, Fukumitsu K, Faulk D, Jiang H, Reing J, Gramignoli R, Komori J, Ross M, et al. A whole-organ regenerative medicine approach for liver replacement. Tissue Eng Part C Methods 2011; 17:677-686; PMID:21375407; http://dx.doi.org/10.1089/ten.tec.2010.0698
- Alkhouri N, Zein NN. Three-dimensional printing and pediatric liver disease. Curr Opin Pediatr 2016; 28:626-630; PMID:27328182; http://dx.doi.org/10.1097/MOP.0000000000000395
- Ma X, Qu X, Zhu W, Li YS, Yuan S, Zhang H, Liu J, Wang P, Lai CS, Zanella F, et al. Deterministically patterned biomimetic human iPSC-derived hepatic model via rapid 3D bioprinting. Proc Natl Acad Sci U S A 2016; 113:2206-2211; PMID:26858399; http://dx.doi.org/10.1073/pnas.1524510113
- Mussbach F, Dahmen U, Dirsch O, Settmacher U. [Liver engineering as a new source of donor organs: A systematic review]. Chirurg 20'16; 87:504-513; PMID:25986672; http://dx.doi.org/10.1007/s00104-015-0015-y