ABSTRACT
Fetal cutaneous wounds have the unique ability to completely regenerate wounded skin and heal without scarring. However, adult cutaneous wounds heal via a fibroproliferative response which results in the formation of a scar. Understanding the mechanism(s) of scarless wound healing leads to enormous clinical potential in facilitating an environment conducive to scarless healing in adult cutaneous wounds. This article reviews the embryonic development of the skin and outlines the structural and functional differences in adult and fetal wound healing phenotypes. A review of current developments made towards applying this clinical knowledge to promote scarless healing in adult wounds is addressed.
INTRODUCTION
The adult epidermis plays a vital role in maintaining the health of the human body: it protects the organism from physical or mechanical insults, prevents microbes and other external pathogens from entering the body and simultaneously prevents loss of vital internal fluids.Citation1 The epidermis must therefore continually renew itself to preserve integrity as it faces a constant barrage of assaults from the surrounding environment. Epidermal stem cells, located in the basal layer (stratum basale) of the stratified squamous epithelium (), are essential is maintaining tissue homeostasis, regenerating hair follicles, and repairing damaged epidermis. The normal response to injury in adult skin results in the formation of a scar. Early in gestation, however, wounded fetal skin is able to regenerate without forming a scar.Citation2, Citation3 The exact mechanisms of scarless wound repair in human skin are currently unknown, but greater understanding may provide insight into how to therapeutically recapitulate the fetal healing phenotype in injured human skin. Intensive effort has been dedicated towards this objective. This review: 1) highlights the key points in embryonic skin development and the key anatomical differences between human fetal and adult skin; 2) provides an overview of epidermal maintenance and the fetal and adult regenerative mechanisms; 3) outlines the progress in the application of this knowledge for therapeutic benefit.
Embryonic skin development
Epidermal development in mammals is a multi-step process consisting of: epidermal specification, commitment, stratification, terminal differentiation, and growth of epidermal appendages. Distinct signaling patterns at different developmental stages ensure the growth and progression of the epidermis and its derivatives. Each step of epidermal development is closely tied to the development of the dermis and the underlying mesenchyme, with differences in the dermis contributing to the regional heterogeneities of the overlying epidermis.Citation4, Citation5
Epidermal specification
Embryonic skin development begins after gastrulation,Citation1 one of the earliest stages of embryonic development happening in the third week after fertilization. In gastrulation, there is invagination of the epiblast along the primitive streak and proliferation and downward migration of epiblast cells. Gastrulation results in the formation of three germ layers of distinct cell lineages: the ectoderm, mesoderm, and endodermCitation6 ().
FIGURE 2. Gastrulation and the formation of 3 distinct germ cell layers: ectoderm, mesoderm, and endoderm.
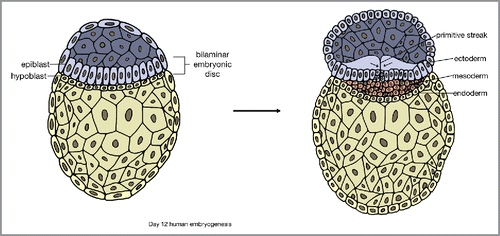
The ectoderm gives rise to both the nervous system and skin epithelium. Neural and epidermal fates of ectodermal cells are based on the effects of Wnt, fibroblast growth factor (FGF), and bone morphogenetic protein (BMP) signaling following gastrulation.Citation7, Citation8 Wnt signaling restricts the ectodermic response to FGFs, a family of polypeptide growth factors required for neural induction.Citation9 This leads to expression of BMPs,Citation10 which also inhibits neural fateCitation7 and commits the cells to differentiate into the epidermis, resulting in a single layer of multipotent epithelial cells.Citation11, Citation12
Epidermal commitment
Keratin is the major structural protein of keratinocytes, and there is a sequence of keratin expression in the developing epidermis. The single layer of multipotent epithelial cells initially express keratins K8/K18. This is replaced by K5/K14 expression prior to stratification, marking the beginning of epidermal commitment.Citation13 Some studies suggest p63 protein, a member of the p53 transcription factor family, plays a significant role in epidermal commitment and K5/K14 induction.Citation14 Isoforms of p63 can induce K14 expression as demonstrated by the strong presence of p63 before any observable significant phenotypic changes towards commitment.Citation15 In addition, ablation of p63 during embryonic development is lethal, preventing the development of stratified epithelia and leading to severe defects in epithelial-mesenchymal-derived structures such as prostate and mammary glands.Citation16
Epidermal stratification and terminal differentiation
The multipotent epithelial cells in the surface ectoderm subsequently become the embryonic epidermal basal layer of the stratifying epidermis, which gives rise to all structures of the future epidermis. The formation of the embryonic basal layer is accompanied by the development of the basement membrane, produced by the basal layer cells.Citation17 The basement membrane separates the epidermis from the dermis and provides epidermal basal cells with growth factors.Citation18 Basal cells attach to the basement membrane through focal adhesions and hemidesmosomes composed of integrins.Citation1
A layer of tightly adhered cells called the periderm transiently overlays the ectoderm during embryonic development (days 9–12 of gestation in mice, and weeks 4–8 of gestation in humans; ).Citation12, Citation19 The periderm is thought to protect the developing epidermis from amniotic fluid,Citation20 and is shed once the epidermis has stratified.Citation12, Citation18
FIGURE 3. Stages of embryonic epidermal development. Adapted from Forni et al. Citation19
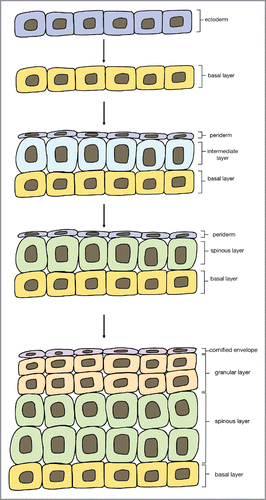
In order to successfully differentiate and stratify, cells must escape the basement layer. Recent studies in mouse embryos have suggested that during stratification, basal cells change mitotic spindle orientation, allowing them to generate both a committed suprabasal cell as well as an undifferentiated basal cell.Citation21 As cells in the basal layer proliferate, they form an ‘intermediate layer’ of cells under the periderm. This intermediate layer is transient and only exists in embryonic skin, it divides and matures into spinous cells.Citation22 Both intermediate layer and spinous cells express K1, whose expression is induced by Notch signaling. Notch signaling directs spinous cells to continue to differentiate, mature, and migrate towards the surface of the skin to form the granular layer and cornified layer.Citation23 In the postnatal epidermis, spinous cells are directly differentiated from proliferating basal cells rather than from intermediate cells. Notch signaling remains important in the postnatal epidermis for inhibiting basal cell proliferation and initiating terminal differentiation.Citation24
The subsequent formation of the granular and cornified layers is predominantly regulated by increases in extracellular Ca2+ concentrations and corresponding intracellular Ca2+ sensing.Citation5 Protein kinase C (PKC) is also thought to contribute to the transition of spinous cells to granular cells.
Growth of epidermal appendages
Development of the epidermis includes the formation of the epidermal appendages such as hair follicles and sweat glands. During development, cells of the basal epidermal layer form buds, which are primitive cells of organ development, and invaginate into the dermis ultimately forming the hair follicle appendages, apocrine, and eccrine glands.
Mammalian hair follicle development has been discovered using murine models, is divided broadly into three stages: 1) hair placode formation; 2) hair follicle organogenesis; 3) cytodifferentiation. These stages are further subdivided into 8 morphologically discrete stages ().Citation25, Citation26
FIGURE 4. The 8 stages of hair development. IRS = inner root sheath, ORS = outer root sheath, SG = sebaceous gland.
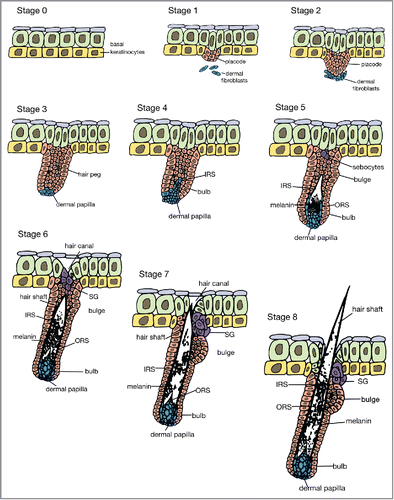
In stage 0, a single basal layer of multipotent epithelial cells is established. In stage 1, continual interactions between mesenchymal cells and the epithelium result in the formation of hair placodes, which first appear in the underlying dermis.Citation1 Placodes are areas of thickening epithelium that will give rise to hair follicles.Citation27 Studies suggest that these early interactions allow the mesenchyme to determine the exact position of the placodes.Citation28, Citation29 In stage 2, the hair placode elongates downwards towards the dermis while dermal fibroblasts that have aggregated under the placode condense and become more evident. In stage 3, the epidermal cells form a hair peg, a multi-layered and elongated column, and the aggregated dermal fibroblasts form a spherical dermal papilla adjacent to the hair peg. In stage 4, the hair peg thickens at the lower end to form a hair bulb, half enclosing the elongated dermal papilla. The inner root sheath forms above the dermal papilla. During stage 5, the inner root sheath extends up the hair follicle and the bulge becomes visible. In stage 6, the downward growth of the hair follicle reaches the subcutis and the inner root sheath forms a hair shaft at the upper end. The dermal papilla becomes thinner and is fully enclosed. The sebaceous gland forms at the upper end of the hair follicle. In stage 7, the tip of the hair shaft leaves the inner root sheath and enters the hair canal. Finally, in stage 8, the hair shaft protrudes above the skin surface and the hair follicle reaches its maximum length.Citation30 The hair follicles express a range of keratins. The outer root sheaths express K5 and K14, the inner root sheaths express K10 and the cortex and medulla express hair-specific keratins.Citation31-33 A sebaceous gland is usually located at the upper part of the hair follicle and helps in the secretion of sebum to lubricate the skin and maintain the waterproof property of hair in mammals. Sebaceous glands develop around week 13–14 of gestation.
In addition to the apocrine sweat glands which are associated with hair follicles, eccrine sweat glands are another epidermal derivative, with ducts that open directly to the skin surface. Apocrine sweat glands are only found in hair regions of the human body. In contrast, eccrine sweat glands are widely distributed across the human body. They are surrounded by adipose tissue and are also closely related to nerve fibers.Citation34 Unlike humans, mice have eccrine sweat glands only in the pads of their paws.Citation35 In humans, sweat glands begin developing around weeks 13–14 of gestation and mature by week 24.Citation36 In mouse embryos, sweat glands emerge as invaginations of epidermal basal cells shortly before birth. During postnatal days P1-5, sweat gland germs develop into single long ducts that extend deeply into the dermis with coiled glands at the tip.Citation37 Formation of sweat gland germs depends on the activation of epidermal Eda signaling, which up-regulates downstream Shh pathway. Shh signaling acts to sustain the coiling stage of sweat gland development and is down-regulated once development completes.Citation37
Migrant cells
There are three types of cells which migrate into the human epidermis at different points of development. Melanocytes are neural crest-derived cells which migrate into the human fetal epidermis by 12 weeks of gestation in humans.Citation38 Langerhans cells are antigen presenting cells of the immune system and appear in human fetal skin at six weeks of gestation, but demonstrate functional activity at around 12–14 weeks of gestation.Citation39 Merkel cells are cells associated with sensory nerve fibers along the dermal-epidermal junction, and appear in human fetal skin at 18 weeks of gestation. The origin of merkel cells is debated, they either arise from within the epidermis or migrate from neural crest cells.Citation40
Adult epidermal maintenance
Mature epidermis is stratified squamous epithelium, composed of layers of cells, with the outermost layer forming the skin surface. In order to guard against dehydration, prevent infection, and re-epithelialize following injury, cells in the innermost or basal layer continuously divide and differentiate, moving outwards towards the skin surface.Citation1 Near the surface of the skin, the cells acquire the barrier function: they become flat and form cornified envelopes consisting of keratin proteins. The resultant layer of cells, called the stratum corneum, creates an impassable seal. This seal is continuously replenished by cells from the basal layer that differentiate as they migrate outwards. Postnatally, the dead cells of the cornified layer are constantly sloughed off and replaced by cells from the basal layer.Citation41 The keratinocyte turnover time, from formation to desquamation, is estimated to be 40–56 days in humansCitation42 and 8–10 days in mice.Citation43
Basal cell proliferation is dependent on the close association between basal cells and the underlying basement membrane. It is a tightly regulated process: excess growth can lead to hyperproliferative disorders, such as psoriasis, whereas insufficient growth can result in thinning of skin.Citation44 When injured, the epidermis produces large quantities of intermediate filaments composed of keratin proteins. As cells from the basal layer migrate to more superficial layers, they switch from expressing keratins K5 and K14 to K1 and K10,Citation45 indicating that the epidermal cells have committed to terminal differentiation.
The first layer of suprabasal cells is the spinous layer consisting of spinous cells, which contain a cytoskeleton of K1/K10 filament bodies connected to desmosomes.Citation1 In hyperproliferative situations, like wound healing, other keratins such as K6, K16, and K17 can be found in suprabasal cells. This keratin network acts to regulate cell growth through stimulation of the Akt/mTOR pathway and remodel the cytoskeleton to allow cellular migration.Citation46
As spinous cells migrate towards the skin surface, they begin to produce keratohyalin granules. Processing of these granules bundles the keratin intermediate filaments to produce macrofibrillar cables. In addition, envelope proteins rich in glutamine and lysine residues are deposited under the plasma membrane of the granular cells. When the cells become permeabilized to calcium, they activate transglutaminase, generating γ-glutamyl ϵ-lysine crosslinks to create an indestructible proteinaceous sac to hold the keratin macrofibrils. The final steps of terminal differentiation involve the destruction of all cellular organelles, including the nucleus, and extrusion of lipid bilayers.
Skin repair versus regeneration
The human epidermis has an impressive ability to heal in response to injury and to restore its structural integrity, barrier, and protective functions. Wound healing occurs in three distinct overlapping phases: inflammation, cell proliferation, and remodeling.Citation47, Citation48 The outcome of healing is dependent upon gestational age, wound site and size, and the developmental age of an organism. Epidermal injuries in embryos in the early stages of development, are capable of healing without scarringCitation49-52; however, at around 24 weeks human fetal skin transitions to heal with a fibrotic scarring response as is observed in adult humans. The ability to heal scarlessly is lost earlier in bigger wounds.Citation53 In adult skin, superficial skin injuries heal through the proliferation of unperturbed basal cells, which are capable of regenerating squamous cells, granular layer, and the stratum corneum. When epidermal damage is extensive and there is disruption to the basal cell layer, adult wounds heal via fibrosis rather than regeneration. The scarring is a result of both the epidermis and dermal compartments.Citation49, Citation54 If distal appendages survive they act as a source of new basal cells, which proliferate out from the surviving appendages and form multiple foci of new epidermis and eventually join. However, when no appendages remain in deep damage, re-epithelialization occurs from the basal cells of the wound margins until a confluent epidermis is achieved. In adult skin, the recovering epidermis loses the ability to develop dermal appendages, unlike in fetal skin where basal cells form dermal appendages.Citation55 Damaged skin is replaced with dysfunctional scar tissue by a proliferative response, that is efficient, but results in skin that is missing dermal appendages, like hair follicles and sebaceous glands, and is comprised of poorly organized collagen.Citation49
There are numerous wound healing differences between human adult and fetal skin, only some of which are relevant in reduced scarring. Experiments involving the transplantation of fetal skin show the ability to heal scarlessly is intrinsic to the skin cells, independent of the intrauterine environment.Citation52 The main cellular causative differences are thought to be related to differences in the inflammatory and cell responses, cytokine and growth factor release, extracellular matrix (ECM) composition, and gene expression.Citation56
Immune responses
A large body of evidence suggests that the ability to heal scarlessly may relate to the attenuated immune response in prenatal life.Citation57 The embryonic immune system is developing, but despite sufficient numbers of immune cells in circulation and a sufficiently developed immune system, human fetal skin at rest contains fewer macrophages, dendritic cells, and Langerhans cells compared to adult skin.Citation58 Likewise, upon wounding fewer inflammatory cells, such as lymphocytes, monocytes, macrophages, mast cells, and neutrophils are attracted to the wounded site. The inflammatory cells present are also less differentiated and have altered behavior. The macrophages present in human fetal skin have an anti-inflammatory M2 phenotype,Citation58 and the mast cells in wounded fetal mouse skin are smaller, less granular, largely immature, and are less able to degranulate in response to injury compared to the mast cells in scarring wounds.Citation59
The inflammatory factors orchestrating the honing response of immune cells may be responsible for their diminished presence and activity at the wounded site. Fetal human skin contains lower levels of chemoattractants including CCL17, CCL21 and CCL27 compared to adult skin.Citation58 CCL27 is important for the influx of memory T cells from the circulation.Citation60 Less neutrophil adhesion molecules are expressed in fetal wounds,Citation61 and consequently less neutrophils migrate into the wound.Citation61, Citation62 There are lower levels of histamine, transforming growth factor-β (TGFβ), tumor necrosis factor alpha (TNFα), and vascular endothelial growth factor (VEGF) released by fetal mast cells.Citation59 TGFβs are secreted polypeptide growth factors with multiple functions, important in both regeneration and repair, and are secreted ligands. TGFß exists in 3 isoforms. Overall levels of TGFß are higher in fetal compared to adult wounds, but fetal wounds have less TGFß1 and TGFß2 and more TGFß3 than adult wounds.Citation63-66 TGFß1 is released by monocytes and macrophages, and upon the degranulation of platelets. It is a chemotactic cytokine for monocytes, fibroblasts, and neutrophils to the wound site.Citation67 TGFß3, in contrast, is a skin morphogenic factor synthesized mainly by keratinocytes and fibroblasts. In fetal wounds the high TGFß3 expression is associated with hypoxia and is able to stop the terminal differentiation of cells, which may function to keep cells relatively undifferentiated and blunt their inflammatory response.Citation63, Citation68
In addition to TGFß3, FGF is another morphogenic factor found in higher levels in fetal, compared to adult skin, reflecting the rapid and considerable growth that fetal skin undergoes during development.Citation69 FGFs stimulate the proliferation and migration of a number of cells.Citation70 There are a number of FGFs that do not change in expression in scarless and scarring healing, but FGF7 and FGF10 are downregulated in scarless healingCitation71 and the FGF receptor 2 shows an earlier and more sustained downregulation in scarless wound healing. Epidermal growth factor (EGF) is another mitogen for fibroblasts and keratinocytes, and more EGF mRNA is also found in wounds of a younger gestational age.Citation72
Platelet-derived growth factor (PDGF) is released by degranulating platelets and is pro-inflammatory. Interestingly, gene expression of PDGF is increased in wounds of younger gestational age,Citation72 but PDGF disappears more rapidly in fetal wounds of mice.Citation69 VEGF has been reported to be less abundant in fetal rat wounds,Citation73 however, greater VEGF mRNA has also been found in the early phases of wound healing in fetal rats in scarless repairing versus scarring repair.Citation74 Variations in fetal wound healing models may explain these contradictory findings. Other inflammatory factors are also released in decreased amounts in fetal compared to adult wounds, including pro-inflammatory interleukins (ILs) 6 and 8.Citation57, Citation75 IL-6 and IL-8 are decreased in fetal wounds even when fetal fibroblasts are stimulated with PDGF.Citation57, Citation76 In contrast, there is over-expression of the anti-inflammatory cytokine, IL-10 in fetal wounds. IL-10 suppresses the expression of IL-1, IL-6, IL-8, and TNF-α,Citation77 inhibits the migration of inflammatory cells, and promotes the organization of wound collagen and dermal architecture.Citation78
Adult skin is constantly exposed to pathogens and it may be expected there is a higher basal immune cell presence in adult compared to fetal skin that is surrounded by a semi-sterile environment. Interestingly, excisional wounds in germ-free mice healed faster indicating that macroscopic wound closure is accelerated when there are low levels of commensal microbiota. These wounds had fewer neutrophils but more mast cells and macrophage infiltration. The levels of IL-10, VEGF, and angiogenesis were higher in germ-free mice, and levels of TGFß1 were reduced compared to conventional mice.Citation79
Extracellular matrix (ECM)
The ECM functions to facilitate cell adhesion, differentiation, and proliferation, and is made of collagen, glycosaminoglycans, and adhesion proteins.Citation80 The adult and fetal ECM differ widely. Collagen deposition is different in fetal and postnatal skin. Fetal skin is composed primarily of type III collagen, and when wounded the newly synthesized collagen is organized into fine, reticular patterns, comparable to collagen organization in uninjured skin.Citation81-83 Adult skin, however, is composed mainly of type 1 collagen. In adult wound repair the collagen is deposited parallel to the wound surface, and extensive cross-linking between collagen fibers leads to a dense, rigid matrix characteristic of scarring.Citation78 This gives skin strength and rigidity but is less conducive to cell migration. The shift from type III to I collagen in fetal development correlates with transition from scarless regeneration to scar formation. Consistent with this finding, fetal fibroblasts, responsible for synthesizing collagen, have decreased pro-collagen 1α1 activity, and increased pro-collagen 3 expression compared to adult fibroblasts. Fetal fibroblasts have also been found to synthesize more total collagen than adult fibroblasts, and have greater propyl hydroxylase activity (the rate limiting enzyme in collagen synthesis). They migrate faster to the wound site and are able to simultaneously synthesize collagen and proliferate, unlike adult fibroblasts where the synthesis of collagen is delayed while fibroblasts proliferate.Citation84
Hyaluronic acid (HA) and chondroitin sulfate are important and abundant glycosaminoglycans in the ECM, composed of repeating disaccharide units of glucuronic acid and N-acetyl glucosamine which form unbranched polysaccharides found on cell surfaces or in the ECM.Citation85, Citation86 HA has a net negative charge that traps water and resists motion of cells. HA is found in higher levels and at earlier time points following wounding in fetal skin compared to adult skin,Citation87 which has led many investigators to propose a role in scarless healing.Citation88, Citation89 Fetal wounds have higher levels of HA synthase expression in response to inflammatory cytokines,Citation90 as well as reduced activity of hyaluronidase in the fetal skin.Citation91 Fetal fibroblasts have more HA receptors (CD44) compared to adult fibroblastsCitation92 facilitating fibroblast migration and deposition of collagen and non-collagen ECM proteins.Citation83, Citation89
Cell adhesion proteins, such as the glycoproteins fibronectin, laminin, and tenascin C, are important in the attachment of fibroblasts, keratinocytes, and endothelial cells to the ECM. A rabbit model revealed fibronectin is expressed earlier in fetal wounds.Citation93 Tenascin C is also deposited earlier in fetal, compared to adult wounds.Citation94 Increased expression of these cell adhesion proteins is thought to facilitate the rapid epithelialization seen in fetal wounds.Citation94-96 Fetal keratinocytes located at the wound edge express more integrins than adult keratinocytes, including receptors for laminin and collagen (α2, α3, α6, β4) and receptors for fibronectin and tenascin C (α5, αV, β6), which may promote keratinocyte proliferation, migration and consequently epithelialization.Citation97
The proteoglycans of the ECM, such as decorin and fibromodulin, regulate collagen fibrillogenesis, cell proliferation, and growth factor activity, including the activity of TGFß. Fetal fibroblasts in mice have reduced decorin expression, and decorin expression increases with gestational age.Citation98
Finally, the proportion of matrix metalloproteinases (MMPs) and tissue-derived inhibitors of metalloproteinases (TIMPs) in fetal wounds may be linked to scarless healing.Citation99 MMPs degrade the ECM, whereas inhibitors of the MMPs stop this matrix degradation. Higher levels of MMP-1, MMP-9, and MMP-14 mRNA has been reported in fetal scarless compared to fetal scarring wounds, whereas fibrotic wounds have decreased MMP-2 and higher levels of TIMPs.Citation99 The MMP:TIMP ratio in fetal wounds is conducive to an environment of remodeling over collagen accumulation.
Wound closure
Epidermal repair occurs through different methods in adult and fetal wounds. The basal cells comprising the epidermal edge of healing wounds in chick embryos are connected by a cable of filamentous actin running continuously through these basal cells, which forms within minutes of wounding.Citation100 Contraction of this actin akin to a ‘purse string’ is responsible for wound closure.Citation101 In contrast, the epidermal edge of adult wounds extends over the exposed dermis to close the wound site. The fibroblasts which migrate to the wound site are able to contract to bring opposed wound edges together through contractile forces, but myofibroblasts, a specialized contractile fibroblast, are the main cell responsible for supplying the tensile strength able to close adult wounds.Citation102 Myofibroblasts are almost absent in fetal wounds, but populate adult wounds in early phases of healing and, likely through the generation of contractile forces, alter collagen fibril orientation and contribute wound contraction and scarring.Citation103
Tissue engineering and regenerative medicine
An increased understanding of the process of regeneration of fetal cutaneous wounds has led to a number of efforts to promote scarless regeneration of adult cutaneous wounds through the manipulation of various processes involved in wound healing. Current guidelines on preventing or treating scars, however, are focused on the management of severe scars, such as hypertrophic scars and keloids.Citation104, Citation105 Most of the treatments currently available for scarring treat scars that have already formed, and there is no single treatment universally accepted as the gold standard for clinical practice. Many of the available treatments have variable and unpredictable effectiveness, unclear mechanisms, and require long-term use or result in unwanted side effects. Furthermore, there are no treatments currently marketed for prophylactic treatment to improve the appearance of scars.Citation105-108 Clinical treatment of scars has been hindered by a lack of the exact processes contributing to scarring, and a lack of randomized clinical trials to evaluate scar treatments.Citation109 A number of companies are currently exploring a range of scar-reduction therapies, outlined here.
Scaffolds
The growing awareness of the importance of the ECM in scarless wound healing has led to the development of tissue-engineered dermal scaffolds to deliver therapeutic cells and provide a supportive environment for tissue regeneration.Citation110 Different materials are being explored in terms of their biocompatibility, toxicity, ability to integrate with the host tissue, and promote regeneration and cellular incorporation. Scaffolds may be composed of naturally occurring substances, like collagen, HA, fibrin, or Chitosan, or constructed as acellular matrices, or made from synthetic biodegradable polymers, which are strong, degradable and have adjustable physical properties.Citation111 HA formed into three-dimensional strands was found to produce a fetal-like environment in rat wounds, with reduced TGFß1.Citation112 However, attempts to manipulate matrices through the addition of proteins have suffered from poor incorporation and rapid degradation, meaning the desired temporospatial presentation of matrix components has not been achieved.
Cell-based therapy
One such strategy with potential for promoting scar-free healing is cell-based therapy. In some forms of cell-based therapy, cells are transplanted into wound sites in the hope of stimulating regeneration and wound repair.Citation113 The cells do not necessarily need to survive for prolonged periods of time, but to survive long enough to promote scar-free healing of the endogenous cells. Cell-based therapy in combination with dermal scaffolds can provide the supportive niche and improve cell survival. Most of the current research into cell-based therapies, however, has been conducted in animal models, with few reports on the use of stem cells in human scars. One study has shown that injection of bone marrow-derived mesenchymal stem cells (MSCs) in both healthy and diabetic mice significantly promotes wound healing in excisional wounds by enhancing both re-epithelialization and cell migration to the injury.Citation114 These results have also been replicated in healthy humans, where topically delivered MSCs facilitated the closure of chronic, non-healing wounds.Citation115 Injection of human amniotic fluid and membrane matrix cells into a painful postsurgical scar was reported to be effective in decreasing the amount of scar tissue and associated pain.Citation116 MSCs and adipose-derived stem cells (ASCs) have also been shown to increase scar quality and reduce associated pain in 13 patients with neuropathic and hypertrophic scars.Citation117 Fat grafts have been shown to improve the appearance of existing scars in five well-designed controlled studies.Citation118-122 The mechanism of improvement is often reported to be due to the presence of ASCs, however there is a lack of evidence to draw definitive conclusions.Citation123 More clinical studies are required before cell-based therapy is used clinically.
Gene therapy
Advances in the understanding of gene expression have made it possible to transfer genetic material of interest into somatic cells. Stem cells genetically modified to alter the expression of certain genes that are differentially expressed in fetal wound healing have been shown to enhance and expedite the wound healing process.Citation124, Citation125 In one such study, transplanted ASCs engineered to over-express VEGF, caused increased blood vessel development which contributed to quicker wound healing in mice when compared to wounds injected with genetically unaltered stem cells or no stem cells.Citation124 A second study found that deletion of IL-10 led to scar formation in fetal wounds, suggesting that over-expression of the anti-inflammatory cytokine IL-10 may constitute another promising target for gene therapy.Citation126
Mechanical forces
The importance of mechanical tension to scar formation has long been underscored by a number of clinical observations: a healing wound placed under mechanical stress leads to the formation of a hypertrophic scar and surgical techniques used to reduce scar size often involve reorienting the direction of the wound to decrease mechanical stress. Adult human skin is inherently taut and is therefore much more likely to heal via scarring than human fetal skin, which has relatively low resting tension.Citation127, Citation128 Mechanical offloading of epithelial wounds to reduce this internal tension has been shown to mitigate fibrosis, whereas increased mechanical tension leads to hypertrophic scarring of wounds. Certain studies have demonstrated the effectiveness of silicone sheets or even tape to passively reduce intrinsic mechanical forces of human skin, and new dynamic polymers are currently being developed to actively reduce the mechanical stress on wounds.Citation129 One such stress-shielding device that mechanically offloads tension has been shown in two randomized controlled clinical trials to significantly reduce scar formation.Citation130, Citation131
Small molecule regulation
One key aspect in recreating the embryonic wound healing process lies in the regulation of signaling molecules to decrease levels of inflammation in healing skin.Citation49 There has been significant investment into developing new pharmaceuticals to minimize scarring on a molecular level. Of the wide array of growth factors and cytokines involved in fetal healing, TGFβ is the most widely studied and has advanced most in clinical development. TGFβ has been implicated in all phases of both adult and fetal epidermal wounds and certain isoforms have a markedly profibrotic effect when added exogenously to healing wounds.Citation132 Studies observing TGFβ isoforms, β3 upregulation and β1 downregulation, in nonscarring fetal healing suggest that there is an optimum ratio of these small molecules required for scarless regeneration.Citation113 In one study, Ferguson et al. was able to promote scarless healing by increasing the ratio of TGFβ3 to TGFβ1 through knockdown of to TGFβ1 in conjunction with exogenous application of TGFβ3.Citation56 As a result, many topical agents such as chitosan, and TGFβ1 antibodies have been developed to inhibit TGFβ1 in an attempt to minimize scar formation. Avotermin was developed as a human recombinant TGFß (‘Juvista’) and in Phase I/II human clinical trials in healthy volunteers in full-thickness excisions significantly reduced scarring compared to placebo, with collagen deposition resembling uninjured skin.Citation56, Citation109 Despite aiming to be the first biopharmaceutical company to develop a drug that reduced scarring, Renovo halted all further pharmaceutical development after Avotermin failed to meet primary and secondary end points in a phase III clinical trial.
The role of other anti-inflammatory agents has also been investigated. Celecoxib is a selective cyclo-oxygenase-2 (COX-2) inhibitor that prevents the conversion of arachidonic acid to prostaglandin precursors. When topically applied to incisional wounds celecoxib reduced the inflammatory phase of healing and treated wounds had a reduction in the scar tissue produced.Citation133 Additionally, lodecakin (recombinant human IL-10) has been shown to reduce scar formation and demonstrated safety in a prospective randomized clinical trials administered to full-thickness excisions in healthy human volunteers.Citation134 Currently, there is no licensed drug in clinical use with a proven consistent success in minimizing scarring.
Conclusion
The adult epidermis has important barrier and protective functions. It is formed from the embryonic ectoderm via a multi-step process, involving distinct signaling patterns, and constant communication with the underlying dermis. In order to fulfill its important functions and maintain tissue homeostasis, stem cells in the basal layer of the epidermis continually reproduce to replace cell loss during turnover or after trauma. Adult skin is able to repair itself but through a process of fibrosis which results in scarring and loss of dermal appendages. The fetal epidermis has a unique ability to heal without scarring, and differences in extracellular proteins, signaling, and inflammatory and cell responses underlie this different healing capacity in fetal and adult skin. A greater understanding of the fetal tissue regeneration process has stimulated a variety of tissue engineering approaches to recapitulate this environment. However, more high-quality randomized controlled trials are required to demonstrate the clinical efficacy of many of the therapies in development for effectiveness in reducing or preventing scarring in human skin.
DISCLOSURE OF POTENTIAL CONFLICTS OF INTEREST
No potential conflicts of interest were disclosed.
Additional information
Funding
REFERENCES
- Fuchs E. Scratching the surface of skin development. Nature. 2007; 445(7130):834-42. doi:10.1038/nature05659. PMID:17314969
- Rowlatt U. Intrauterine wound healing in a 20 week human fetus. Virchows Archiv. 1979; 381(3):353-361. doi:10.1007/BF00432477. PMID:155931
- Adzick N, Longaker M. Characteristics of fetal repair. Fetal Wound Healing. New York, NY: Elsevier; 1992: p. 53-70
- Rinn JL, Wang JK, Allen N, Brugmann SA, Mikels AJ, Liu H, Ridky TW, Stadler HS, Nusse R, Helms JA, et al. A dermal HOX transcriptional program regulates site-specific epidermal fate. Genes Dev. 2008; 22(3):303-7. doi:10.1101/gad.1610508. PMID:18245445
- Koster MI, Roop DR. Mechanisms regulating epithelial stratification. Annu Rev Cell Dev Biol. 2007; 23:93-113. doi:10.1146/annurev.cellbio.23.090506.123357. PMID:17489688
- Arnold SJ, Robertson EJ. Making a commitment: cell lineage allocation and axis patterning in the early mouse embryo. Nat Rev Mol Cell Biol. 2009; 10(2):91-103. doi:10.1038/nrm2618. PMID:19129791
- Stern CD. Neural induction: old problem, new findings, yet more questions. Development. 2005; 132(9):2007-21. doi:10.1242/dev.01794. PMID:15829523
- MacDonald BT, Tamai K, He X. Wnt/beta-catenin signaling: components, mechanisms, and diseases. Dev Cell. 2009; 17(1):9-26. doi:10.1016/j.devcel.2009.06.016. PMID:19619488
- Bottcher RT, Niehrs C. Fibroblast growth factor signaling during early vertebrate development. Endocr Rev. 2005; 26(1):63-77. doi:10.1210/er.2003-0040. PMID:15689573
- Bleuming SA, He XC, Kodach LL, Hardwick JC, Koopman FA, Ten Kate FJ, van Deventer SJ, Hommes DW, Peppelenbosch MP, Offerhaus GJ, et al. Bone morphogenetic protein signaling suppresses tumorigenesis at gastric epithelial transition zones in mice. Cancer Res. 2007; 67(17):8149-55. doi:10.1158/0008-5472.CAN-06-4659. PMID:17804727
- Moll R, Moll I, Wiest W. Changes in the pattern of cytokeratin polypeptides in epidermis and hair follicles during skin development in human fetuses. Differentiation. 1982; 23(2):170-8. doi:10.1111/j.1432-0436.1982.tb01280.x. PMID:6187618
- M'Boneko V, Merker HJ. Development and morphology of the periderm of mouse embryos (days 9–12 of gestation). Acta Anatomica. 1988; 133(4):325-36. doi:10.1159/000146662. PMID:3227794
- Byrne C, Tainsky M, Fuchs E. Programming gene expression in developing epidermis. Development. 1994; 120(9):2369-83. PMID:7525178
- Yang A, Schweitzer R, Sun D, Kaghad M, Walker N, Bronson RT, Tabin C, Sharpe A, Caput D, Crum C, et al. p63 is essential for regenerative proliferation in limb, craniofacial and epithelial development. Nature. 1999; 398(6729):714-8. doi:10.1038/19539. PMID:10227294
- Romano RA, Birkaya B, Sinha S. A functional enhancer of keratin14 is a direct transcriptional target of deltaNp63. J Invest Dermatol. 2007; 127(5):1175-86. doi:10.1038/sj.jid.5700652. PMID:17159913
- Morasso MI, Tomic-Canic M. Epidermal stem cells: the cradle of epidermal determination, differentiation and wound healing. Biologie Cellulaire. 2005; 97(3):173-83. doi:10.1042/BC20040098
- Mikkola ML. Genetic basis of skin appendage development. in Seminars in cell & developmental biology. Amsterdam, Netherlands: Elsevier; 2007
- Liu S, Zhang H, Duan E. Epidermal development in mammals: key regulators, signals from beneath, and stem cells. Int J Mol Sci. 2013; 14(6):10869-95. doi:10.3390/ijms140610869. PMID:23708093
- Forni MF, Trombetta-Lima M, Sogayar MC. Stem cells in embryonic skin development. Biol Res. 2012; 45(3):215-22. doi:10.4067/S0716-97602012000300003. PMID:23283431
- Benitah SA, Frye M. Stem cells in ectodermal development. Journal of molecular medicine. 2012; 90(7):783-790. doi:10.1007/s00109-012-0908-x. PMID:22570240
- Lechler T, Fuchs E. Asymmetric cell divisions promote stratification and differentiation of mammalian skin. Nature. 2005; 437(7056):275-80. doi:10.1038/nature03922. PMID:16094321
- Smart IH. Variation in the plane of cell cleavage during the process of stratification in the mouse epidermis. British Journal of Dermatology, 1970; 82(3):276-82. doi:10.1111/j.1365-2133.1970.tb12437.x. PMID:5441760
- Koster MI, Dai D, Marinari B, Sano Y, Costanzo A, Karin M, Roop DR. p63 induces key target genes required for epidermal morphogenesis. Proc Natl Acad Sci USA. 2007; 104(9):3255-60. doi:10.1073/pnas.0611376104. PMID:17360634
- Blanpain C, Lowry WE, Pasolli HA, Fuchs E. Canonical notch signaling functions as a commitment switch in the epidermal lineage. Genes and Development. 2006; 20(21):3022-35. doi:10.1101/gad.1477606. PMID:17079689
- Schmidt‐Ullrich R, Paus R. Molecular principles of hair follicle induction and morphogenesis. Bioessays. 2005; 27(3):247-261. doi:10.1002/bies.20184. PMID:15714560
- Stenn K, Paus R. What controls hair follicle cycling? Exp Dermatol. 1999; 8(4):229-236. doi:10.1111/j.1600-0625.1999.tb00376.x. PMID:10439219
- Park BY, Saint-Jeannet JP. in Induction and Segregation of the Vertebrate Cranial Placodes. Williston, Vermont, US: Morgan & Claypool Life Sciences; 2010
- Hardy MH. The secret life of the hair follicle. Trends in Genetics. 1992; 8(2):55-61. doi:10.1016/0168-9525(92)90350-D. PMID:1566372
- Olivera-Martinez I, Thelu J, Dhouailly D. Molecular mechanisms controlling dorsal dermis generation from the somitic dermomyotome. Int J Dev Biol. 2004; 48(2–3):93-101. doi:10.1387/ijdb.15272374. PMID:15272374
- Paus R, Müller-Röver S, Van Der Veen C MM, Eichmüller S, Ling G, Hofmann U, Foitzik K, Mecklenburg L, et al. A comprehensive guide for the recognition and classification of distinct stages of hair follicle morphogenesis. J Invest Dermatol. 1999; 113(4):523-32. doi:10.1046/j.1523-1747.1999.00740.x. PMID:10504436
- Heid HW, Werner E, Franke WW. The complement of native α-keratin polypeptides of hair-forming cells: a subset of eight polypeptides that differ from epithelial cytokeratins. Differentiation, 1986; 32(2):101-119. doi:10.1111/j.1432-0436.1986.tb00562.x. PMID:2431943
- Lynch MH, O'Guin WM, Hardy C, Mak L, Sun TT. Acidic and basic hair/nail (“ hard”) keratins: their colocalization in upper cortical and cuticle cells of the human hair follicle and their relationship to” soft” keratins. J Cell Biol. 1986; 103(6):2593-2606. doi:10.1083/jcb.103.6.2593. PMID:2432071
- Stark H, Breitkreutz D, Limat A, Ryle CM, Roop D, Leigh I, Fusenig N. Keratins 1 and 10 or homologues as regular constituents of inner root sheath and cuticle cells in the human hair follicle. Eur J Cell Biol. 1990; 52(2):359-372. PMID:1706998
- Sato K, et al. Biology of sweat glands and their disorders. I. Normal sweat gland function. J Am Acad Dermatol. 1989; 20(4):537-563
- Lu C, Fuchs E. Sweat gland progenitors in development, homeostasis, and wound repair. Cold Spring Harbor perspectives in medicine. 2014; 4(2):a015222. doi:10.1101/cshperspect.a015222. PMID:24492848
- Fu X, Li J, Sun X, Sun T, Sheng Z. Epidermal stem cells are the source of sweat glands in human fetal skin: evidence of synergetic development of stem cells, sweat glands, growth factors, and matrix metalloproteinases. Wound Repair and Regeneration. 2005; 13(1):102-8. doi:10.1111/j.1067-1927.2005.130113.x. PMID:15659042
- Kunisada M, Cui CY, Piao Y, Ko MS, Schlessinger D. Requirement for Shh and Fox family genes at different stages in sweat gland development. Human Molecular Genetics. 2009; 18(10):1769-78. doi:10.1093/hmg/ddp089. PMID:19270025
- Holbrook KA. Structure and function of the developing human skin. Biochemistry and Physiology of the Skin, 1983; 1:64-101
- Foster CA, Holbrook KA, Farr AG. Ontogeny of Langerhans cells in human embryonic and fetal skin: expression of HLA-DR and OKT-6 determinants. J Invest Dermatol. 1986; 86(3):240-243. doi:10.1111/1523-1747.ep12285201. PMID:2427603
- Moll R, Moll I, Franke WW. Identification of Merkel cells in human skin by specific cytokeratin antibodies:: Changes of cell density and distribution in fetal and adult plantar epidermis. Differentiation, 1984; 28(2):136-154. doi:10.1111/j.1432-0436.1984.tb00277.x. PMID:6084624
- Mascré G, Dekoninck S, Drogat B, Youssef KK, Broheé S, Sotiropoulou PA, Simons BD, Blanpain C. Distinct contribution of stem and progenitor cells to epidermal maintenance. Nature. 2012; 489(7415):257-62. doi:10.1038/nature11393. PMID:22940863
- Halprin K. Epidermal” turnover time”–a re-examination. The British journal of dermatology, 1972; 86(1):14. doi:10.1111/j.1365-2133.1972.tb01886.x. PMID:4551262
- Potten C, Saffhill R, Maibach H. Measurement of the transit time for cells through the epidermis and stratum corneum of the mouse and guinea‐pig. Cell proliferation, 1987; 20(5):461-472. doi:10.1111/j.1365-2184.1987.tb01355.x.
- Fuchs E, Horsley V. More than one way to skin. Genes and Development. 2008; 22(8):976-85. doi:10.1101/gad.1645908. PMID:18413712
- Fuchs E, Green H. Changes in keratin gene expression during terminal differentiation of the keratinocyte. Cell, 1980; 19(4): p. 1033-42. doi:10.1016/0092-8674(80)90094-X. PMID:6155214
- Kim S, Wong P, Coulombe PA. A keratin cytoskeletal protein regulates protein synthesis and epithelial cell growth. Nature. 2006; 441(7091):362-5. doi:10.1038/nature04659. PMID:16710422
- Singer AJ, Clark RA. Cutaneous wound healing. New England journal of medicine, 1999; 341(10):738-746. doi:10.1056/NEJM199909023411006. PMID:10471461
- Longaker MT, Gurtner GC. Introduction: wound repair. in Seminars in cell & developmental biology. Cambridge, Massachusetts, US: Academic Press; 2012
- Gurtner GC, Werner S, Barrandon Y, Longaker MT. Wound repair and regeneration. Nature. 2008; 453(7193):314-21. doi:10.1038/nature07039. PMID:18480812
- Adzick NS, Longaker MT. Animal models for the study of fetal tissue repair. J Surg Res. 1991; 51(3):216-222. doi:10.1016/0022-4804(91)90097-6. PMID:1908924
- Adzick NS, Longaker MT. Scarless fetal healing. Therapeutic implications. Annals of surgery, 1992; 215(1):3. doi:10.1097/00000658-199201000-00004. PMID:1731647
- Lorenz HP, et al. Scarless wound repair: a human fetal skin model. Development, 1992; 114(1):253-259. PMID:1576963
- Cass DL, Bullard KM, Sylvester KG, Yang EY, Longaker MT, Adzick NS. Wound size and gestational age modulate scar formation in fetal wound repair. J Pediatr Surg. 1997; 32(3):411-415. doi:10.1016/S0022-3468(97)90593-5. PMID:9094005
- Longaker MT, et al. Adult skin wounds in the fetal environment heal with scar formation. Annals of surgery, 1994; 219(1):65. doi:10.1097/00000658-199401000-00011. PMID:8297179
- Lane AT. Human fetal skin development. Pediatric dermatology, 1986; 3(6):487-491. doi:10.1111/j.1525-1470.1986.tb00656.x. PMID:3562363
- Ferguson MW, O'Kane S. Scar-free healing: from embryonic mechanisms to adult therapeutic intervention. Philos Trans R Soc Lond B Biol Sci. 2004; 359(1445):839-50. doi:10.1098/rstb.2004.1475. PMID:15293811
- Liechty KW, Adzick NS, Crombleholme TM. Diminished interleukin 6 (IL-6) production during scarless human fetal wound repair. Cytokine. 2000; 12(6):671-676. doi:10.1006/cyto.1999.0598. PMID:10843743
- Walraven M, Talhout W, Beelen RH, van Egmond M, Ulrich MM. Healthy human second‐trimester fetal skin is deficient in leukocytes and associated homing chemokines. Wound Repair and Regeneration. 2016; 24(3):533-541. doi:10.1111/wrr.12421. PMID:26873861
- Wulff BC, Parent AE, Meleski MA, DiPietro LA, Schrementi ME, Wilgus TA. Mast cells contribute to scar formation during fetal wound healing. J Invest Dermatol. 2012; 132(2):458-465. doi:10.1038/jid.2011.324. PMID:21993557
- Morales J, Homey B, Vicari AP, Hudak S, Oldham E, Hedrick J, Orozco R, Copeland NG, Jenkins NA, McEvoy LM, et al. CTACK, a skin-associated chemokine that preferentially attracts skin-homing memory T cells. Proceedings of the National Academy of Sciences, 1999; 96(25):14470-14475. doi:10.1073/pnas.96.25.14470.
- Naik-Mathuria B, Gay AN, Zhu X, Yu L, Cass DL, Olutoye OO. Age-dependent recruitment of neutrophils by fetal endothelial cells: implications in scarless wound healing. J Pediatr Surg. 2007; 42(1):166-171. doi:10.1016/j.jpedsurg.2006.09.058. PMID:17208559
- Olutoye OO, Zhu X, Cass DL, Smith CW. Neutrophil recruitment by fetal porcine endothelial cells: implications in scarless fetal wound healing. Pediatric research. 2005; 58(6):1290-1294. doi:10.1203/01.pdr.0000184326.01884.bc. PMID:16306210
- Barrientos S, Stojadinovic O, Golinko MS, Brem H, Tomic-Canic M. Growth factors and cytokines in wound healing. Wound Repair Regen. 2008; 16(5):585-601. doi:10.1111/j.1524-475X.2008.00410.x. PMID:19128254
- Eslami A, Gallant-Behm CL, Hart DA, Wiebe C, Honardoust D, Gardner H, Häkkinen L, Larjava HS. Expression of integrin αvβ6 and TGF-β in scarless vs scar-forming wound healing. Journal of Histochemistry & Cytochemistry. 2009; 57(6):543-557. doi:10.1369/jhc.2009.952572.
- Hsu M, Peled ZM, Chin GS, Liu W, Longaker MT. Ontogeny of expression of transforming growth factor-beta 1 (TGF-beta 1), TGF-beta 3, and TGF-beta receptors I and II in fetal rat fibroblasts and skin. Plastic and reconstructive surgery. 2001; 107(7):1787-94; discussion 1795–6. doi:10.1097/00006534-200106000-00023. PMID:11391201
- Longaker MT, Bouhana KS, Harrison MR, Danielpour D, Roberts AB, Banda MJ. Wound healing in the fetus. Wound Repair and Regeneration, 1994; 2(2):104-112. doi:10.1046/j.1524-475X.1994.20204.x. PMID:17134379
- Soo C, Hu FY, Zhang X, Wang Y, Beanes SR, Lorenz HP, Hedrick MH, Mackool RJ, Plaas A, Kim SJ, et al. Differential expression of fibromodulin, a transforming growth factor-β modulator, in fetal skin development and scarless repair. The American journal of pathology. 2000; 157(2):423-433. doi:10.1016/S0002-9440(10)64555-5. PMID:10934147
- Caniggia I, Mostachfi H, Winter J, Gassmann M, Lye SJ, Kuliszewski M, Post M. Hypoxia-inducible factor-1 mediates the biological effects of oxygen on human trophoblast differentiation through TGFβ;3. J Clin Invest. 2000; 105(5):577. doi:10.1172/JCI8316. PMID:10712429
- Whitby DJ, Ferguson MW. Immunohistochemical localization of growth factors in fetal wound healing. Developmental biology, 1991; 147(1):207-215. doi:10.1016/S0012-1606(05)80018-1. PMID:1879607
- Ornitz DM, Itoh N. Fibroblast growth factors. Genome biology. 2001; 2(3):reviews3005. 1. doi:10.1186/gb-2001-2-3-reviews3005. PMID:11276432
- Dang CM, Beanes SR, Soo C, Ting K, Benhaim P, Hedrick MH, Lorenz HP. Decreased expression of fibroblast and keratinocyte growth factor isoforms and receptors during scarless repair. Plastic and reconstructive surgery. 2003; 111(6):1969-1979. doi:10.1097/01.PRS.0000054837.47432.E7. PMID:12711959
- Peled ZM, Rhee SJ, Hsu M, Chang J, Krummel TM, Longaker MT. The ontogeny of scarless healing II: EGF and PDGF-B gene expression in fetal rat skin and fibroblasts as a function of gestational age. Ann Plast Surg. 2001; 47(4):417-424. doi:10.1097/00000637-200110000-00010. PMID:11601578
- Ihara S, Motobayashi Y, Nagao E, Kistler A. Ontogenetic transition of wound healing pattern in rat skin occurring at the fetal stage. Development. 1990; 110(3):671-680. PMID:2088714
- Colwell AS, Beanes SR, Soo C, Dang C, Ting K, Longaker MT, Atkinson JB, Lorenz HP. Increased angiogenesis and expression of vascular endothelial growth factor during scarless repair. Plast Reconstr Surg. 2005; 115(1):204-212. PMID:15622252
- Satish L, Kathju S. Cellular and molecular characteristics of scarless versus fibrotic wound healing. Dermatol Res Pract. 2010; 2010. doi:10.1155/2010/790234. PMID:21253544
- Liechty KW, Crombleholme TM, Cass DL, Martin B, Adzick NS. Diminished interleukin-8 (IL-8) production in the fetal wound healing response. J Surg Res. 1998; 77(1):80-84. doi:10.1006/jsre.1998.5345. PMID:9698538
- Couper KN, Blount DG, Riley EM. IL-10: the master regulator of immunity to infection. J Immunol. 2008; 180(9):5771-5777. doi:10.4049/jimmunol.180.9.5771. PMID:18424693
- Peranteau WH, Zhang L, Muvarak N, Badillo AT, Radu A, Zoltick PW, Liechty KW. IL-10 overexpression decreases inflammatory mediators and promotes regenerative healing in an adult model of scar formation. J Invest Dermatol. 2008; 128(7):1852-1860. doi:10.1038/sj.jid.5701232. PMID:18200061
- Canesso MC, Vieira AT, Castro TB, Schirmer BG, Cisalpino D, Martins FS, Rachid MA, Nicoli JR, Teixeira MM, Barcelos LS. Skin wound healing is accelerated and scarless in the absence of commensal microbiota. J Immunol. 2014; 193(10):5171-5180. doi:10.4049/jimmunol.1400625. PMID:25326026
- Schultz GS, Wysocki A. Interactions between extracellular matrix and growth factors in wound healing. Wound repair and regeneration. 2009; 17(2):153-162. doi:10.1111/j.1524-475X.2009.00466.x. PMID:19320882
- Merkel JR, DiPaolo BR, Hallock GG, Rice DC. Type I and type III collagen content of healing wounds in fetal and adult rats. Proc Soc Exp Biol Med. 1988; 187(4):493-497. doi:10.3181/00379727-187-42694. PMID:3353398
- Burd DA, Longaker MT, Adzick NS, Harrison MR, Ehrlich HP. Foetal wound healing in a large animal model: the deposition of collagen is confirmed. British journal of plastic surgery, 1990; 43(5):571-577. doi:10.1016/0007-1226(90)90122-G. PMID:2224353
- Longaker MT, Whitby DJ, Adzick NS, Crombleholme TM, Langer JC, Duncan BW, Bradley SM, Stern R, Ferguson MW, Harrison MR. Studies in fetal wound healing VI. Second and early third trimester fetal wounds demonstrate rapid collagen deposition without scar formation. J Pediatr Surg. 1990; 25(1):63-69. doi:10.1016/S0022-3468(05)80165-4. PMID:2299547
- Larson BJ, Longaker MT, Lorenz HP. Scarless fetal wound healing: a basic science review. Plastic and reconstructive surgery. 2010; 126(4):1172. doi:10.1097/PRS.0b013e3181eae781. PMID:20885241
- Oerther S, Payan E, Lapicque F, Presle N, Hubert P, Muller S, Netter P. Hyaluronate-alginate combination for the preparation of new biomaterials: investigation of the behaviour in aqueous solutions. Biochimica et Biophysica Acta (BBA)-General Subjects, 1999; 1426(1):185-194. doi:10.1016/S0304-4165(98)00155-X.
- Shepard S, Becker H, Hartmann JX. Using hyaluronic acid to create a fetal-like environment in vitro. Annals of plastic surgery, 1996; 36(1):65-69. doi:10.1097/00000637-199601000-00013. PMID:8722987
- Longaker MT, Chiu ES, Adzick NS, Stern M, Harrison MR, Stern R. Studies in fetal wound healing. V. A prolonged presence of hyaluronic acid characterizes fetal wound fluid. Annals of surgery, 1991; 213(4):292.
- Mast BA, Flood LC, Haynes JH, DePalma RL, Cohen IK, Diegelmann RF, Krummel TM. Hyaluronic acid is a major component of the matrix of fetal rabbit skin and wounds: implications for healing by regeneration. Matrix, 1991; 11(1):63-68. doi:10.1016/S0934-8832(11)80228-3. PMID:2027330
- Mast BA, Diegelmann RF, Krummel TM, Cohen IK. Hyaluronic acid modulates proliferation, collagen and protein synthesis of cultured fetal fibroblasts. Matrix, 1993; 13(6):441-446. doi:10.1016/S0934-8832(11)80110-1. PMID:8309423
- Kennedy CI, Diegelmann RF, Haynes JH, Yager DR. Proinflammatory cytokines differentially regulate hyaluronan synthase isoforms in fetal and adult fibroblasts. J Pediatr Surg. 2000; 35(6):874-879. doi:10.1053/jpsu.2000.6869. PMID:10873029
- West DC, Shaw DM, Lorenz P, Adzick NS, Longaker MT. Fibrotic healing of adult and late gestation fetal wounds correlates with increased hyaluronidase activity and removal of hyaluronan. The international journal of biochemistry & cell biology, 1997; 29(1):201-210. doi:10.1016/S1357-2725(96)00133-1.
- Alaish SM, Yager D, Diegelmann RF, Cohen IK. Biology of fetal wound healing: hyaluronate receptor expression in fetal fibroblasts. J Pediatr Surg. 1994; 29(8):1040-1043. doi:10.1016/0022-3468(94)90275-5. PMID:7525917
- Longaker MT, Whitby DJ, Ferguson MW, Harrison MR, Crombleholme TM, Langer JC, Cochrum KC, Verrier ED, Stern R. Studies in fetal wound healing: III. Early deposition of fibronectin distinguishes fetal from adult wound healing. J Pediatr Surg. 1989; 24(8):799-805
- Whitby DJ, Longaker MT, Harrison MR, Adzick NS, Ferguson MW. Rapid epithelialisation of fetal wounds is associated with the early deposition of tenascin. J Cell Sci. 1991; 99(3):583-586. PMID:1719005
- Whitby D, Ferguson M. The extracellular matrix of lip wounds in fetal, neonatal and adult mice. Development, 1991; 112(2):651-668. PMID:1724421
- Longaker MT, Whitby DJ, Jennings RW, Duncan BW, Ferguson MW, Harrison MR, Adzick NS. Fetal diaphragmatic wounds heal with scar formation. J Surg Res. 1991; 50(4):375-385. doi:10.1016/0022-4804(91)90206-2. PMID:2020189
- Cass DL, Bullard KM, Sylvester KG, Yang EY, Sheppard D, Herlyn M, Adzick NS. Epidermal integrin expression is upregulated rapidly in human fetal wound repair. J Pediatr Surg. 1998; 33(2):312-316. doi:10.1016/S0022-3468(98)90453-5. PMID:9498408
- Beanes SR, Dang C, Soo C, Wang Y, Urata M, Ting K, Fonkalsrud EW, Benhaim P, Hedrick MH, Atkinson JB. Down-regulation of decorin, a transforming growth factor–beta modulator, is associated with scarless fetal wound healing. J Pediatr Surg. 2001; 36(11):1666-1671. doi:10.1053/jpsu.2001.27946. PMID:11685698
- Dang CM, Beanes SR, Lee H, Zhang X, Soo C, Ting K. Scarless fetal wounds are associated with an increased matrix metalloproteinase–to–tissue-derived inhibitor of metalloproteinase ratio. Plastic and reconstructive surgery. 2003; 111(7):2273-2285. doi:10.1097/01.PRS.0000060102.57809.DA. PMID:12794470
- Brock J, Midwinter K, Lewis J, Martin P. Healing of incisional wounds in the embryonic chick wing bud: characterization of the actin purse-string and demonstration of a requirement for Rho activation. J Cell Biol. 1996; 135(4):1097-1107. doi:10.1083/jcb.135.4.1097. PMID:8922389
- Martin P, Lewis J. Actin cables and epidermal movement in embryonic wound healing. Nature. 1992; 360(6400):179-183. doi:10.1038/360179a0. PMID:1436096
- Nodder S, Martin P. Wound healing in embryos:a review. Anatomy and embryology. 1997; 195(3):215-228. doi:10.1007/s004290050041. PMID:9084820
- Estes JM, Vande Berg JS, Adzick NS, MacGillivray TE, Desmoulière A, Gabbiani G. Phenotypic and functional features of myofibroblasts in sheep fetal wounds. Differentiation. 1994; 56(3):173-181. doi:10.1046/j.1432-0436.1994.5630173.x. PMID:8034132
- Bayat A, McGrouther DA. Clinical management of skin scarring. SKINmed: Dermatology for the Clinician. 2005; 4(3):165-173. doi:10.1111/j.1540-9740.2005.02507.x.
- Mustoe TA, Cooter RD, Gold MH, Hobbs FD, Ramelet AA, Shakespeare PG, Stella M, Téot L, Wood FM, Ziegler UE, et al. International clinical recommendations on scar management. Plastic and reconstructive surgery. 2002; 110(2):560-571. doi:10.1097/00006534-200208000-00031. PMID:12142678
- Meier K, Nanney LB. Emerging new drugs for scar reduction. Expert opinion on emerging drugs. 2006; 11(1):39-47. doi:10.1517/14728214.11.1.39. PMID:16503825
- Franz MG, Steed DL, Robson MC. Optimizing healing of the acute wound by minimizing complications. Current problems in surgery. 2007; 44(11):691-763. doi:10.1067/j.cpsurg.2007.07.001. PMID:18036992
- Batra R. Surgical techniques for scar revision. Skin therapy letter. 2005; 10(4):4-7. PMID:15986081
- Occleston NL, O'Kane S, Goldspink N, Ferguson MW. New therapeutics for the prevention and reduction of scarring. Drug discovery today. 2008; 13(21):973-981. doi:10.1016/j.drudis.2008.08.009. PMID:18824245
- Yates CC, Bodnar R, Wells A. Matrix control of scarring. Cell Mol Life Sci. 2011; 68(11):1871-1881. doi:10.1007/s00018-011-0663-0. PMID:21390544
- Debels H, Hamdi M, Abberton K, Morrison W. Dermal matrices and bioengineered skin substitutes: a critical review of current options. Plastic and reconstructive surgery Global open. 2015; 3(1). doi:10.1097/GOX.0000000000000219. PMID:25674365
- Hu M, Sabelman EE, Cao Y, Chang J, Hentz VR. Three‐dimensional hyaluronic acid grafts promote healing and reduce scar formation in skin incision wounds. Journal of Biomedical Materials Research Part B: Applied Biomaterials. 2003; 67(1):586-592. doi:10.1002/jbm.b.20001.
- Degen KE, Gourdie RG. Embryonic wound healing: a primer for engineering novel therapies for tissue repair. Birth Defects Res C Embryo Today. 2012; 96(3):258-70. doi:10.1002/bdrc.21019. PMID:23109321
- Wu Y, Chen L, Scott PG, Tredget EE. Mesenchymal stem cells enhance wound healing through differentiation and angiogenesis. Stem Cells. 2007; 25(10):2648-59. doi:10.1634/stemcells.2007-0226. PMID:17615264
- Falanga V, Iwamoto S, Chartier M, Yufit T, Butmarc J, Kouttab N, Shrayer D, Carson P. Autologous bone marrow-derived cultured mesenchymal stem cells delivered in a fibrin spray accelerate healing in murine and human cutaneous wounds. Tissue Engineering. 2007; 13(6):1299-312. doi:10.1089/ten.2006.0278. PMID:17518741
- Hemphill C, Stavoe K, Khalpey Z. First in man: amniotic stem cell injection promotes scar remodeling and healing processes in late-stage fibrosis. Int J Cardiol. 2014; 174(2):442. doi:10.1016/j.ijcard.2014.04.023. PMID:24768371
- Huang SH, Wu SH, Chang KP, Lin CH, Chang CH, Wu YC, Lee SS, Lin SD, Lai CS. Alleviation of neuropathic scar pain using autologous fat grafting. Annals of plastic surgery. 2015; 74:S99-S104. doi:10.1097/SAP.0000000000000462. PMID:25695456
- Balkin DM, Samra S, Steinbacher DM. Immediate fat grafting in primary cleft lip repair. Journal of Plastic, Reconstructive & Aesthetic Surgery. 2014; 67(12):1644-1650. doi:10.1016/j.bjps.2014.08.049.
- Ribuffo D, Atzeni M, Guerra M, Bucher S, Politi C, Deidda M, Atzori F, Dessi M, Madeddu C, Lay G. Treatment of irradiated expanders: protective lipofilling allows immediate prosthetic breast reconstruction in the setting of postoperative radiotherapy. Aesthetic plastic surgery. 2013; 37(6):1146-1152. doi:10.1007/s00266-013-0221-2. PMID:24114295
- Zellner EG, Pfaff MJ, Steinbacher DM. Fat grafting in primary cleft lip repair. Plastic and reconstructive surgery. 2015; 135(5):1449-1453. doi:10.1097/PRS.0000000000001187. PMID:25919258
- Bruno A, Delli Santi G, Fasciani L, Cempanari M, Palombo M, Palombo P. Burn scar lipofilling: immunohistochemical and clinical outcomes. J Craniofac Surg. 2013; 24(5):1806-1814. doi:10.1097/SCS.0b013e3182a148b9. PMID:24036785
- Maione L, Memeo A, Pedretti L, Verdoni F, Lisa A, Bandi V, Giannasi S, Vinci V, Mambretti A, Klinger M. Autologous fat graft as treatment of post short stature surgical correction scars. Injury. 2014; 45:S126-S132. doi:10.1016/j.injury.2014.10.036. PMID:25457332
- Spiekman M, van Dongen JA, Willemsen JC, Hoppe DL, van der Lei B, Harmsen MC. The power of fat and its adipose‐derived stromal cells: emerging concepts for fibrotic scar treatment. Journal of Tissue Engineering and Regenerative Medicine. 2017; 11(11):3220–3235. doi:10.1002/term.2213
- Nauta A, Seidel C, Deveza L, Montoro D, Grova M, Ko SH, Hyun J, Gurtner GC, Longaker MT, Yang F. Adipose-derived stromal cells overexpressing vascular endothelial growth factor accelerate mouse excisional wound healing. Mol Ther. 2013; 21(2):445-55. doi:10.1038/mt.2012.234. PMID:23164936
- Liu W, Cao Y, Longaker MT. Gene therapy of scarring: a lesson learned from fetal scarless wound healing. Yonsei Med J. 2001; 42(6):634-45. doi:10.3349/ymj.2001.42.6.634. PMID:11754147
- Liechty KW, Kim HB, Adzick NS, Crombleholme TM. Fetal wound repair results in scar formation in interleukin-10–deficient mice in a syngeneic murine model of scarless fetal wound repair. J Pediatr Surg. 2000; 35(6):866-873. doi:10.1053/jpsu.2000.6868. PMID:10873028
- Aarabi S, Bhatt KA, Shi Y, Paterno J, Chang EI, Loh SA, Holmes JW, Longaker MT, Yee H, Gurtner GC. Mechanical load initiates hypertrophic scar formation through decreased cellular apoptosis. Faseb J. 2007; 21(12):3250-61. doi:10.1096/fj.07-8218com. PMID:17504973
- Derderian CA, Bastidas N, Lerman OZ, Bhatt KA, Lin SE, Voss J, Holmes JW, Levine JP, Gurtner GC. Mechanical strain alters gene expression in an in vitro model of hypertrophic scarring. Ann Plast Surg. 2005; 55(1):69-75; discussion 75. doi:10.1097/01.sap.0000168160.86221.e9. PMID:15985794
- Wong VW, Akaishi S, Longaker MT, Gurtner GC. Pushing back: wound mechanotransduction in repair and regeneration. J Invest Dermatol. 2011; 131(11):2186-96. doi:10.1038/jid.2011.212. PMID:21776006
- Longaker MT, Rohrich RJ, Greenberg L, Furnas H, Wald R, Bansal V, Seify H, Tran A, Weston J, Korman JM, et al. A randomized controlled trial of the embrace advanced scar therapy device to reduce incisional scar formation. Plast Reconstr Surg. 2014; 134(3):536-46. doi:10.1097/PRS.0000000000000417. PMID:24804638
- Lim AF, Weintraub J, Kaplan EN, Januszyk M, Cowley C, McLaughlin P, Beasley B, Gurtner GC, Longaker MT. The embrace device significantly decreases scarring following scar revision surgery in a randomized controlled trial. Plast Reconstr Surg. 2014; 133(2):398-405. doi:10.1097/01.prs.0000436526.64046.d0. PMID:24105084
- Bullard KM, Longaker MT, Lorenz HP. Fetal wound healing: current biology. World J Surg. 2003; 27(1):54-61. doi:10.1007/s00268-002-6737-2. PMID:12557038
- Wilgus TA, Vodovotz Y, Vittadini E, Clubbs EA, Oberyszyn TM. Reduction of scar formation in full‐thickness wounds with topical celecoxib treatment. Wound Repair Regen. 2003; 11(1):25-34. doi:10.1046/j.1524-475X.2003.11106.x. PMID:12581424
- Kieran I, Knock A, Bush J, So K, Metcalfe A, Hobson R, Mason T, O'Kane S, Ferguson M. Interleukin‐10 reduces scar formation in both animal and human cutaneous wounds: Results of two preclinical and phase II randomized control studies. Wound Repair and Regeneration. 2013; 21(3):428-436. doi:10.1111/wrr.12043. PMID:23627460\